DOI:
10.1039/C5QO00311C
(Research Article)
Org. Chem. Front., 2016,
3, 66-70
Copper-catalyzed domino synthesis of benzo[b]thiophene/imidazo[1,2-a]pyridines by sequential Ullmann-type coupling and intramolecular C(sp2)–H thiolation†
Received
10th October 2015
, Accepted 14th November 2015
First published on 17th November 2015
Abstract
The copper-catalyzed double C–S bond formation via Ullmann-type S-arylation and C–H thiolation using K2S as a sulfur source is described. This novel one-step sulfur-incorporation method provides a straightforward avenue to benzo[b]thiophene and imidazo[1,2-a]pyridine frameworks.
Seeking efficient and convenient methods for the construction of C–S bonds is of fundamental research interest in organic chemistry, since sulfur-containing architectures are prevalent in natural products, drugs, bioactive molecules, and materials.1 Generally, cross-coupling reactions are established to be very useful tools for the formation of C–S bonds. In the past few years, with the renaissance of Ullmann-type reactions,2 the copper-catalyzed cross-couplings of aryl halides with thiols have been demonstrated to be a versatile method for constructing C(sp2)–S bonds.3 Meanwhile, metal sulfides as abundant inorganic substances are also used as a sustainable thiol source, and have been widely used for introducing sulfur atoms into organic molecules.4 In 2010, Xi and co-workers reported an elegant copper-catalyzed one-pot synthesis of thiophenes from 1,4-diiodo-1,3-dienes and potassium sulphide (Scheme 1a).5 In the same year, Li's group developed an efficient CuI-catalyzed double thiolation reaction of 1,4-dihalides with sulfides leading to 2-trifluoromethyl benzothiophenes under mild conditions (Scheme 1b).6 Although great achievements have been made using these methods, the substrates involved in these transformations could be mainly limited to aryl halides. Over the past few decades, direct transformation of inert C–H bonds has emerged as an economical and environmentally friendly alternative to traditional synthetic methods.7 However, a literature survey indicates that such a synthetic strategy for the formation of C–S bonds remains rather limited,1d,g,8 and especially the substrates were mainly electron-rich arenas. In this respect, several examples using thiols, diaryl disulfides, 1-(substituted phenylthio)pyrrolidine-2,5-dione, and sulfonyl hydrazide as thiolation reagents under Cu,9 Fe,10 Pd,11 and metal-free12 conditions have been reported. Very recently, Shi and co-workers developed an elegant copper-mediated C–S/N–S bond-forming reaction via C–H activation using elemental sulfur as a sulfuration agent (Scheme 1c).13 From these wonderful studies, it is thereby expected that combining the two coupling partners C(sp2)–X and C(sp2)–H to access the C–S bonds using metal sulfides under copper-catalytic conditions might be more practical and economical (Scheme 1d).
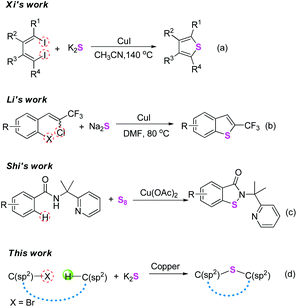 |
| Scheme 1 Strategies for the construction of C–S bonds. | |
The benzo[b]thiophene skeleton is the core unit of natural products, and its derivatives show remarkable biological and medicinal properties.14 For example, they are found in numerous clinically important drugs, such as raloxifene,15 arzoxifene,16 zileuton,17 and clopidogrel.18 In addition, benzo[b]thiophene derivatives are also widely applied in the field of materials science because of their excellent optical properties.19 On the other hand, imidazo[1,2-a]pyridine fragments widely exist in many commercially available drugs, such as zolimidin (to treat peptic ulcer),20 minodronic acid (to treat osteoporosis),21 zolpidem (to treat insomnia),22 and olprinone (to treat heart failure).23 However, synthesis of the combined motifs of benzo[b]thiophene and imidazo[1,2-a]pyridine frameworks (Fig. 1) has not been explored thus far. Therefore, we wish to synthesize this new kind of fused sulfur-containing N-heterocycle which could possibly possess biological activity and optical properties. With our growing interest in sulfur-containing organic compounds synthesis,24 we herein report a novel and efficient copper-catalyzed one-pot synthesis of benzo[b]thiophene/imidazo[1,2-a]pyridines by sequential Ullmann-type coupling and aerobic oxidative intramolecular C–H thiolation. To the best of our knowledge, this method is the first example of copper-catalyzed direct double C–S bond formation in one step via Ullmann-type S-arylation and C–H thiolation using metal sulfides as a sulfur source.
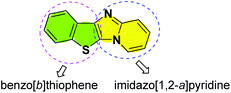 |
| Fig. 1 Structure of a conjugate containing benzo[b]thiophene and imidazo[1,2-a]pyridine frameworks. | |
We commenced our study by examining the reaction between 2-(2-bromophenyl)imidazo[1,2-a]pyridine 1a and K2S 2 to investigate experimental conditions including the optimization of catalysts, ligands, solvents and temperature under an air atmosphere. As shown in Table 1, eight copper catalysts (entries 1–8) were examined at 120 °C in the presence of 0.1 equiv. of 1,10-phenanthroline (A) as the ligand (relative to the amount of 1a) in DMF, and CuI showed the highest reaction activity (entry 3). Only trace amounts of the target product 3a were observed in the absence of catalyst (entry 9). Furthermore, different ligands were tested (entries 3, 10–13), and 1,10-phenanthroline (A) exhibited the highest efficiency (entry 3). We also tested various solvents (entries 3, 14–19), and DMF showed the best result (entry 3). The effect of temperature was also investigated (entries 20–22), and the yields reached the maximum when the temperature was raised from 110 °C to 130 °C. Interestingly, when Na2S was used as the partner of 1a, only 14% of yield was obtained (entry 23). Notably, only 12% yield of the desired product was obtained under a nitrogen atmosphere, indicating that dioxygen is essential in the present transformation (entry 24).
Table 1 Optimization of the conditions a
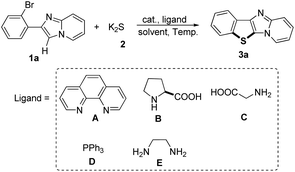
|
Entry |
Cat. |
Ligand |
Solvent |
Yieldb [%] |
Reaction conditions: 2-(2-bromophenyl)imidazo[1,2-a]pyridine (1a) (0.3 mmol), K2S (2) (0.6 mmol), catalyst (0.03 mmol), ligand (0.03 mmol), solvent (2 mL), 120 °C, reaction time (24 h), under air.
Isolated yield.
110 °C.
120 °C.
130 °C.
Na2S was used.
Under a nitrogen atmosphere (extrusion of air).
|
1 |
CuCl |
A |
DMF |
69 |
2 |
CuBr |
A |
DMF |
72 |
3
|
CuI
|
A
|
DMF
|
81
|
4 |
CuSO4 |
A |
DMF |
67 |
5 |
Cu(OAc)2 |
A |
DMF |
74 |
6 |
Cu(NO3)2 |
A |
DMF |
66 |
7 |
Cu(OTf)2 |
A |
DMF |
63 |
8 |
Cu2O |
A |
DMF |
69 |
9 |
None |
A |
DMF |
Trace |
10 |
CuI |
B |
DMF |
Trace |
11 |
CuI |
C |
DMF |
Trace |
12 |
CuI |
D |
DMF |
63 |
13 |
CuI |
E |
DMF |
57 |
14 |
CuI |
A |
DMSO |
66 |
15 |
CuI |
A |
NMP |
Trace |
16 |
CuI |
A |
1,4-Dioxane |
26 |
17 |
CuI |
A |
DCE |
Trace |
18 |
CuI |
A |
CH3CN |
11 |
19 |
CuI |
A |
H2O |
0 |
20 |
CuI |
A |
DMF |
78c |
21 |
CuI |
A |
DMF |
72d |
22 |
CuI |
A |
DMF |
81e |
23 |
CuI |
A |
DMF |
14f |
24 |
CuI |
A |
DMF |
12g |
Next, the substrate scope for the copper-catalyzed synthesis of benzo[b]thiophene/imidazo[1,2-a]pyridines (3) was investigated under the optimized conditions (using 10 mol% CuI as the catalyst, 10 mol% 1,10-phenanthroline as the ligand, two equiv. of K2S as the thiol source, DMF as the solvent at 120 °C under air). As shown in Table 2, the corresponding benzo[b]thiophene/imidazo[1,2-a]pyridines were obtained in moderate to good yields for the examined substrates at 120 °C. Generally, for R1 and R2 substituents, the substrates bearing electron-donating or electron-withdrawing groups were found to show no obvious difference in the transformation. However, a strong electron-withdrawing group such as nitro was not tolerated under the standard conditions (3s). The reason should be that the weak coordination of Cu(I) with sulfur made Cu(I) species unreactive in the present transformation owing to the much more stronger electron-withdrawing properties of the nitro group (see Scheme 3, formation mechanism, the intermediate V). In addition, various functional groups such as methyl, ether, halogen, and trifluoromethyl were well-tolerated under the optimized conditions. Reaction of 6-bromo-2-(2-bromophenyl)imidazo[1,2-a]pyridines with K2S only took place on the ortho-site C–Br bond of the imidazole group, whereas the 6-site C–Br bond remained intact, thus showing the ortho-substituent effect of the imidazole group during S-arylations (3h and 3r). Furthermore, the application of our present protocol for thiolation of other heterocyclic compounds was explored. To our delight, substituted 6-(2-bromophenyl)imidazo[2,1-b]thiazoles also gave moderate yields of the thiolation products in 62–67% yields (3t–3v).
Table 2 Scope of 2-(2-bromophenyl)imidazo[1,2-a]pyridines for the synthesis of benzo[b]thiophene/imidazo[1,2-a]pyridines (3)a,b
Reaction conditions: 2-(2-bromophenyl)imidazo[1,2-a]pyridines (1) (0.3 mmol), K2S (2) (0.6 mmol), CuI (0.03 mmol), 1,10-phen (0.03 mmol), solvent (2 mL), reaction temperature (120 °C) under air.
Isolated yield.
|
|
Although this transformation was efficient, unfortunately not all the N-heterocycles were compatible with K2S under the standard conditions. For example, when 2-(2-bromophenyl)-1-methyl-1H-indole and 1-(2-bromophenyl)-1H-pyrrole were used as the substrates under the optimal reaction conditions, no desired product was obtained (Scheme 2). Thus, further investigations to explore more powerful catalysts and ligands are required.
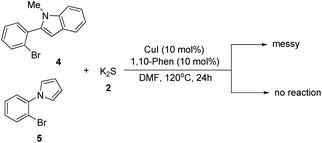 |
| Scheme 2 Substrate scope of heterocyclic compounds. | |
It is interesting to know the optical properties of the synthesized 3a and derivatives. Therefore, 3a and some selected derivatives were analyzed by UV–vis and photoluminescence (PL) spectroscopy in solution. As shown in Fig. 2, the UV–vis spectra of naked 3a and substituted derivatives 3c, 3l, 3j and 3r have high-intensity absorption between 240 and 270 nm, and lower-intensity bands between 320 and 370 nm. Their emission maxima are observed within the range of 380–430 nm. Compared to naked 3a, substituted derivatives 3c, 3l, 3j and 3r have bathochromic shifts or hypochromatic shifts in both absorption and emission spectra to some extent. Apparently, when there is an electron-donating group attached to the pyridine ring or an electron withdrawing group attached to the benzene ring, a hypochromatic shift could occur.
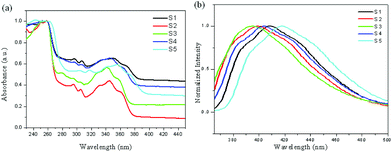 |
| Fig. 2 Normalized UV–vis (a) and photoluminescence (PL) (b) spectra of selected derivatives 3 in DCM (5.0 × 10−5 M); (S1: 3a; S2: 3c; S3: 3l; S4: 3j; S5: 3r). | |
According to the results above and the related literature,25 a possible mechanism for this domino thiolation is thus outlined in Scheme 3. Reaction of CuX with a ligand produces a chelated Cu(I) complex (I), and the subsequent oxidative addition of the chelate with 1 provides the intermediate (II), in which the nitrogen of the imidazole group may coordinate to Cu to provide additional stabilization. Treatment of K2S (2) with (II) forms the complex (III), and then reductive elimination of (III) leads to the S-arylation product (IV). Reaction of (IV) with LCuX gives the “S–Cu–L” complex (V), then (V) furnishes (VI) under air (O2). Reductive elimination of (VI) leads to the target product 3 and regenerates the catalyst, LCuX.
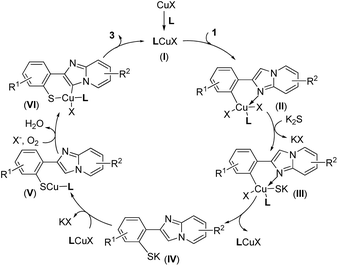 |
| Scheme 3 A proposed mechanism for the direct transformation. | |
In summary, we have developed a novel and efficient copper-catalyzed one-pot method for the synthesis of benzo[b]thiophene/imidazo[1,2-a]pyridines. The corresponding products were obtained in moderate to good yields with excellent functional group tolerance. Some important features of the present protocol involve the use of inexpensive CuI/1,10-phen as the catalyst/ligand system, readily available substituted 2-(2-bromophenyl)imidazo[1,2-a]pyridines and K2S as the starting materials, and environmentally friendly air (O2) as the sole oxidant. Further investigations on the practical application of this method are ongoing in our laboratory.
The authors gratefully acknowledge the financial support from the National Natural Science Foundation of China (No. 21302110, 21302109 and 21375075), the Scientific Research Foundation of Qufu Normal University (BSQD 2012021), the Taishan Scholar Foundation of Shandong Province, the Natural Science Foundation of Shandong Province (ZR2013BQ017 and ZR2013M007), and the Project of Shandong Province Higher Educational Science and Technology Program (J13LD14). We thank Pengfei Sun in this group for reproducing the results of 3a and 3u.
Notes and references
-
(a) T. Kondo and T.-A. Mitsudo, Chem. Rev., 2000, 100, 3205 CrossRef CAS PubMed;
(b) A. R. Murphy and J. M. J. Fréchet, Chem. Rev., 2007, 107, 1066 CrossRef CAS PubMed;
(c) M. Mellah, A. Voituriez and E. Schulz, Chem. Rev., 2007, 107, 5133 CrossRef CAS PubMed;
(d) H. Haruki, M. G. Pedersen, K. I. Gorska, F. Pojer and K. Johnsson, Science, 2013, 340, 987 CrossRef CAS PubMed;
(e) H. Liu and X. Jiang, Chem. – Asian J., 2013, 8, 2546 CrossRef CAS PubMed;
(f) M. D. McReynolds, J. M. Dougherty and P. R. Hanson, Chem. Rev., 2004, 104, 2239 CrossRef CAS PubMed;
(g) I. P. Beletskaya and V. P. Ananikov, Chem. Rev., 2011, 111, 1596 CrossRef CAS PubMed;
(h) C. Shen, P. Zhang, Q. Sun, S. Bai, T. S. Andy Hor and X. Liu, Chem. Soc. Rev., 2015, 44, 291 RSC;
(i) D. Wu, Z. Chen, Y. Zhang, J. Zhang, S. Liu and J. Yin, J. Org. Chem., 2015, 80, 8443 CrossRef CAS PubMed;
(j) X.-D. Xiong, C.-L. Deng, X.-S. Peng, Q. Miao and H. N. C. Wong, Org. Lett., 2014, 16, 3252 CrossRef CAS PubMed.
- For reviews, see:
(a) G. Evano, N. Blanchard and M. Toumi, Chem. Rev., 2008, 108, 3054 CrossRef CAS PubMed;
(b) D. S. Surry and S. L. Buchwald, Chem. Sci., 2010, 1, 13 RSC;
(c) H. Rao and H. Fu, Synlett, 2011, 745 CAS;
(d) D. Ma and Q. Cai, Acc. Chem. Res., 2008, 41, 1450 CrossRef CAS PubMed;
(e) S. R. Chemler and P. H. Fuller, Chem. Soc. Rev., 2007, 36, 1153 RSC;
(f) J. R. Dehli, J. Legros and C. Bolm, Chem. Commun., 2005, 973 RSC;
(g) P. Zhao, H. Yin, H. Gao and C. Xi, J. Org. Chem., 2013, 78, 5001 CrossRef CAS PubMed.
- For selected papers, see:
(a) L. Rout, T. K. Sen and T. Punniyamurthy, Angew. Chem., Int. Ed., 2007, 46, 5583 CrossRef CAS PubMed;
(b) L. Rout, P. Saha, S. Jammi and T. Punniyamurthy, Eur. J. Org. Chem., 2008, 640 CrossRef CAS;
(c) X. Lv and W. Bao, J. Org. Chem., 2007, 72, 3863 CrossRef CAS PubMed;
(d) C. G. Bates, R. K. Gujadhur and D. Venkataraman, Org. Lett., 2002, 4, 2803 CrossRef CAS PubMed;
(e) Y.-J. Chen and H.-H. Chen, Org. Lett., 2006, 8, 5609 CrossRef CAS PubMed;
(f) D. J. C. Prasad and G. Sekar, Synthesis, 2010, 1, 79 Search PubMed;
(g) A. K. Verma, J. Singh and R. Chaudhary, Tetrahedron Lett., 2007, 48, 7199 CrossRef CAS;
(h) K. Su, Y. Qiu, Y. Yao, D. Zhang and S. Jiang, Synlett, 2012, 2853 CAS;
(i) Y.-J. Chen and H.-H. Chen, Org. Lett., 2006, 8, 5609 CrossRef CAS PubMed.
-
(a) T. Kashiki, S. Shinamura, M. Kohara, E. Miyazaki, K. Takimiya, M. Ikeda and H. Kuwabara, Org. Lett., 2009, 11, 2473 CrossRef CAS PubMed;
(b) D. Ma, S. Xie, P. Xue, X. Zhang, J. Dong and Y. Jiang, Angew. Chem., Int. Ed., 2009, 48, 4222 CrossRef CAS PubMed;
(c) Y. Liu, J.-L. Zhang, R.-J. Song and J.-H. Li, Org. Lett., 2014, 16, 5838 CrossRef CAS PubMed;
(d) N. Azizi, E. Akbari, F. Ebrahimi and M. R. Saidi, Monatsh. Chem., 2010, 141, 323 CrossRef CAS;
(e) Z. Qiao, J. Wei and X. Jiang, Org. Lett., 2014, 16, 1212 CrossRef CAS PubMed;
(f) Y. Li, J. Pu and X. Jiang, Org. Lett., 2014, 16, 2692 CrossRef CAS PubMed;
(g) Z. Qiao, H. Liu, X. Xiao, Y. Fu, J. Wei and X. Jiang, Org. Lett., 2013, 15, 2594 CrossRef CAS PubMed;
(h) Q. Liao, W. Youa, Z.-B. Lou, L.-R. Wen and C. Xi, Tetrahedron Lett., 2013, 54, 1475 CrossRef CAS;
(i) F. Wang, C. Chen, G. Deng and C. Xi, J. Org. Chem., 2012, 77, 4148 CrossRef CAS PubMed.
- W. You, X. Yan, Q. Liao and C. Xi, Org. Lett., 2010, 12, 3930 CrossRef CAS PubMed.
- C. Li, X. Zhang, R. Tang, P. Zhong and J. Li, J. Org. Chem., 2010, 75, 7037 CrossRef CAS PubMed.
- For recent reviews, see:
(a) K. M. Engle, T.-S. Mei, M. Wasa and J.-Q. Yu, Acc. Chem. Res., 2012, 45, 788 CrossRef CAS PubMed;
(b) J. C. Lewis, R. G. Bergman and J. A. Ellman, Acc. Chem. Res., 2008, 41, 1013 CrossRef CAS PubMed;
(c) D. A. Colby, A. S. Tsai, R. G. Bergman and J. A. Ellman, Acc. Chem. Res., 2012, 45, 814 CrossRef CAS PubMed;
(d) C.-L. Sun, B.-J. Li and Z.-J. Shi, Chem. Rev., 2011, 111, 1293 CrossRef CAS PubMed;
(e) I. A. I. Mkhalid, J. H. Barnard, T. B. Marder, J. M. Murphy and J. F. Hartwig, Chem. Rev., 2010, 110, 890 CrossRef CAS PubMed;
(f) S. R. Neufeldt and M. S. Sanford, Acc. Chem. Res., 2012, 45, 936 CrossRef CAS PubMed.
- P. Anbarasan, H. Neumann and M. Beller, Chem. Commun., 2011, 47, 3233 RSC.
-
(a) S. Zhang, P. Qian, M. Zhang, M. Hu and J. Cheng, J. Org. Chem., 2010, 75, 6732 CrossRef CAS PubMed;
(b) X. Chen, X.-S. Hao, C. E. Goodhue and J.-Q. Yu, J. Am. Chem. Soc., 2006, 128, 6790 CrossRef CAS PubMed;
(c) D. Alves, R. G. Lara, M. E. Contreira, C. S. Radatz, L. F. B. Duarte and G. Perin, Tetrahedron Lett., 2012, 53, 3364 CrossRef CAS;
(d) A. Zhou, X. Liu, K. Yang, S. Zhao and Y. Liang, Org. Biomol. Chem., 2011, 9, 5456 RSC;
(e) H. Deng, Z. Li, F. Ke and X. Zhou, Chem. – Eur. J., 2012, 18, 4840 CrossRef CAS PubMed;
(f) L. D. Tran, I. Popov and O. Daugulis, J. Am. Chem. Soc., 2012, 134, 18237 CrossRef CAS PubMed.
-
(a) H. Wang, L. Wang, J. Shang, X. Li, H. Wang, J. Gui and A. Lei, Chem. Commun., 2012, 48, 76 RSC;
(b) M. Zhang, S. Zhang, C. Pan and F. Chen, Synth. Commun., 2012, 42, 2844 CrossRef CAS.
-
(a) K. Inamoto, Y. Arai, K. Hiroya and T. Doi, Chem. Commun., 2008, 5529 RSC;
(b) L. L. Joyce and R. A. Batey, Org. Lett., 2009, 11, 2792 CrossRef CAS PubMed;
(c) M. Iwasaki, M. Iyanaga, Y. Tsuchiya, Y. Nishimura, W.-J. Li, Z.-P. Li and Y. Nishihara, Chem. – Eur. J., 2014, 20, 2459 CrossRef CAS PubMed;
(d) S. K. Sahoo, A. Banerjee, S. Chakraborty and B. K. Patel, ACS Catal., 2012, 2, 544 CrossRef CAS.
-
(a) R. Tang, Y. Xie, Y. Xie, J. Xiang and J. Li, Chem. Commun., 2011, 47, 12867 RSC;
(b) S.-R. Guo, Y.-Q. Yuan and J.-N. Xiang, Org. Lett., 2014, 15, 4654 CrossRef PubMed;
(c) W. Ge and Y. Wei, Green Chem., 2012, 14, 2066 RSC;
(d) Y.-F. Liao, P.-C. Jiang, S.-P. Chen, H.-R. Qi and G.-J. Deng, Green Chem., 2013, 15, 3302 RSC;
(e) L. Zou, J. Reball, J. Mottweiler and C. Bolm, Chem. Commun., 2012, 48, 11307 RSC;
(f) C. D. Prasad, S. J. Balkrishna, A. Kumar, B. S. Bhakuni, K. Shrimali, S. Biswas and S. Kumar, J. Org. Chem., 2013, 78, 1434 CrossRef CAS PubMed;
(g) P. Sang, Z.-K. Chen, J.-W. Zou and Y.-H. Zhang, Green Chem., 2013, 15, 2096 RSC;
(h) W. Zhao, P. Xie, Z. Bian, A. Zhou, H. Ge, M. Zhang, Y. Ding and L. Zheng, J. Org. Chem., 2015, 80, 9167 CrossRef CAS PubMed;
(i) T. Hostier, V. Ferey, G. Ricci, D. G. Pardo and J. Cossy, Org. Lett., 2015, 17, 3898 CrossRef CAS PubMed.
- F.-J. Chen, G. Liao, X. Li, J. Wu and B.-F. Shi, Org. Lett., 2014, 16, 5644 CrossRef CAS PubMed.
- D. A. Horton, G. T. Bourne and M. L. Smythe, Chem. Rev., 2003, 103, 893 CrossRef CAS PubMed , and references cited therein.
- Z. Qin, I. Kasrati, E. P. Chandrasena, H. Liu, P. Yao, P. A. Petukhov, J. L. Bolton and G. R. J. Thatcher, J. Med. Chem., 2007, 50, 2682 CrossRef CAS PubMed.
- B. L. Flynn, E. Hamel and M. K. Jung, J. Med. Chem., 2002, 45, 2670 CrossRef CAS PubMed.
- B. L. Mylari, E. R. Larson, T. A. Beyer, W. J. Zembrowski, C. E. Aldinger, M. F. Dee, T. W. Siegel and D. H. Singleton, J. Med. Chem., 1991, 34, 108 CrossRef CAS PubMed.
- E. Rogers, H. Araki, L. A. Batory, C. E. McInnis and J. T. Njardarson, J. Am. Chem. Soc., 2007, 129, 2768 CrossRef CAS PubMed.
- K. Takimiya, I. Osaka, T. Mori and M. Nakano, Acc. Chem. Res., 2014, 47, 1493 CrossRef CAS PubMed , and references cited therein.
- L. Almirante, L. Polo, A. Mugnaini, E. Provinciali, P. Rugarli, A. Biancotti, A. Gamba and W. Murmann, J. Med. Chem., 1965, 8, 305 CrossRef CAS PubMed.
- L. A. Sorbera, J. Castaner and P. A. Leeson, Drugs Future, 2002, 27, 935 CrossRef CAS.
- H. T. Swainston and G. M. Keating, CNS Drugs, 2005, 19, 65 CrossRef.
- T. Ueda and K. Mizushige, Curr. Vasc. Pharmacol., 2006, 4, 1 CrossRef CAS PubMed.
-
(a)
D. Yang, K. Yan, W. Wei, J. Zhao, M. Zhang, X. Sheng, G. Li, S. Lu and H. Wang, J. Org. Chem, 2015, 80, 6083 Search PubMed;
(b) K. Yan, D. Yang, W. Wei, J. Zhao, Y. Shuai, L. Tian and H. Wang, Org. Biomol. Chem., 2015, 13, 732 Search PubMed;
(c) K. Yan, D. Yang, P. Sun, W. Wei, Y. Liu, G. Li, S. Lu and H. Wang, Tetrahedron Lett., 2015, 56, 4792 CrossRef CAS;
(d) D. Yang, K. Yan, W. Wei, L. T. ian, Q. Li, J. You and H. Wang, RSC Adv., 2014, 4, 48547 RSC;
(e) W. Wei, J. Li, D. Yang, J. Wen, Y. Jiao, J. You and H. Wang, Org. Biomol. Chem., 2014, 12, 1861 RSC;
(f) W. Wei, J. Wen, D. Yang, J. Du, J. You and H. Wang, Green Chem., 2014, 16, 2988 RSC;
(g) D. Yang, K. Yan, W. Wei, G. Li, S. Lu, C. Zhao, L. Tian and H. Wang, J. Org. Chem., 2015, 80, 11073 CrossRef CAS PubMed.
-
(a) S. Fukuzawa, E. Shimizu, Y. Atsuumi, M. Haga and K. Ogata, Tetrahedron Lett., 2009, 50, 2374 CrossRef CAS;
(b) A. R. Rosario, K. K. Casola, C. E. S. Oliveira and G. Zeni, Adv. Synth. Catal., 2013, 355, 2960 CrossRef CAS;
(c) H. Xu and H. Fu, Chem. – Eur. J., 2012, 18, 1180 CrossRef CAS PubMed;
(d) D. Ma, S. Xie, P. Xue, X. Zhang, J. Dong and Y. Jiang, Angew. Chem., Int. Ed., 2009, 48, 4222 CrossRef CAS PubMed;
(e) H. Xu, S. Ma, Y. Xu, L. Bian, T. Ding, X. Fang, W. Zhang and Y. Ren, J. Org. Chem., 2015, 80, 789 Search PubMed.
Footnote |
† Electronic supplementary information (ESI) available. See DOI: 10.1039/c5qo00311c |
|
This journal is © the Partner Organisations 2016 |
Click here to see how this site uses Cookies. View our privacy policy here.