DOI:
10.1039/C6PY01019A
(Paper)
Polym. Chem., 2016,
7, 5501-5506
N-Ferrocenylsulfonyl-2-methylaziridine: the first ferrocene monomer for the anionic (co)polymerization of aziridines†
Received
13th June 2016
, Accepted 20th July 2016
First published on 21st July 2016
Abstract
N-Ferrocenylsulfonyl-2-methylaziridine (fcMAz) is synthesized in a convenient three-step protocol from ferrocene. It represents the first aziridine-based monomer suitable for the anionic polymerization, carrying a ferrocenyl-substituent. Sulfonamide-activated aziridines can undergo (mostly living) anionic polymerization and fcMAz is also the first example of a functional sulfonamide-based aziridine monomer. It can be polymerized with different initiators to homo and copolymers (block or statistical) with adjustable molecular weights. The homopolymer is insoluble in common organic solvents, while the copolymers with other aziridines are soluble and exhibit molecular weight dispersities of 1.1–1.5. FcMAz thus broadens the scope of ferrocenyl-containing polymers and may be combined with other anionic polymerization techniques in the future.
Introduction
Ferrocene (fc)-containing polymers have been studied for several decades, due to their reversible redox-properties, self-assembly and related properties.1–3 To date, the major monomers that are found in the literature, concerning fc-containing materials, are vinyl ferrocene4 and strained silicon-bridged [1]-ferrocenophanes5 (mainly ferrocenyldimethyl silane, Fig. 1), besides several others.1,3 They can be combined with controlled polymerization techniques, like controlled radical or living anionic polymerization. Our group recently introduced the first fc-containing epoxides, which can undergo oxyanionic polymerization and allow the production of fc-containing polyethers.6–8
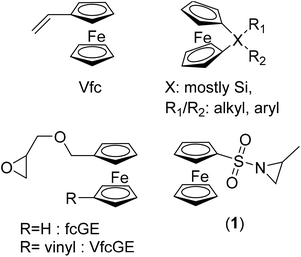 |
| Fig. 1 fc-containing monomers. | |
Herein, we expand the scope of fc-containing monomers to the first fc-aziridine that can undergo anionic polymerization, namely N-ferrocenylsulfonyl-2-methylaziridine (fcMAz, 1). Aziridines have been studied for decades as reactive intermediates9,10 or as monomers for the mostly uncontrolled cationic ring-opening polymerization to poly(ethylene imine)-derivatives.11,12 Earlier work on fc-containing aziridines involved attaching the organometallic moiety to the ring-carbons,13 which were for example used for ring-expansion of the three-membered aziridine to fc-containing oxazolines.14 Wang et al. reported the synthesis of chiral ferrocenyl aziridino alcohols prepared from L-serine and ferrocenecarboxaldehyde; the authors used them as chiral catalysts for the asymmetric addition of diethylzinc to aldehydes.15,16 In contrast, we attached fc via the activating sulfonamide group to the ring-nitrogen of aziridine, allowing subsequent nucleophilic ring-opening17 to perform anionic homo-18 and copolymerization with other sulfonylaziridines.9
Earlier work on fc-modified poly(ethylene imine) derivatives involved attaching the fc-groups via the amines or the carbon atoms in the polymer backbone. These polymers were used as molecular wires to connect the redox centers of enzymes to an electrode surface. Amperometric biosensors for glucose and hydrogen peroxide have been constructed via the immobilization of enzymes in crosslinked polymer films.19–21
FcMAz was investigated with respect to polymerization control, comonomer incorporation rates in statistical copolymers and thermal and electrochemical properties. Besides the fact that poly(1) is insoluble as a homopolymer, it produces soluble and rather well-defined copolymers, allowing the combination with other anionic polymerization techniques for future applications.
Experimental
Materials/methods and additional experimental procedures can be found in the ESI.†
Synthesis of N-ferrocenylsulfonyl-2-methylaziridine (fcMAz, 1)
Ammonium ferrocenyl sulfonate22.
Ferrocene (40 g, 0.22 mol) was suspended in 700 mL acetic anhydride and 15 mL chlorosulfonic acid was added over a period of ca. 30 min. After stirring overnight at room temperature the mixture was poured onto 800 mL ice water, concentrated at reduced pressure and extracted overnight with a Soxhlet-setup with methanol. The extract was concentrated to ca. 250 mL and ca. 100 mL conc. aq. ammonia was added. A brown precipitate was removed by filtration; the filtrate was dried in vacuo to yield 26 g (86 mmol, 40%) of ammonium ferrocenyl sulfonate (as monohydrate).
1H NMR (300 MHz, DMSO-d6): δ [ppm] = 7.15 (br, 4H, NH4+), 4.18 (2H, Cpsubst.), 3.35 (s, 5H, Cp), 2.50 (2H, Cpsubst.)
Ferrocenyl sulfonic acid chloride23.
10.2 g (34 mmol) of ammonium ferrocene sulfonate monohydrate were suspended in 50 mL dry dichloromethane and cooled in an ice-bath. A few drops of dimethylformamide and 5.8 mL oxalyl chloride were added dropwise. The reaction mixture was stirred for 30 min at 0 °C. A gas evolution was noted during this time. The cooling bath was then removed and the solution was allowed to warm up to room-temperature and stirring was continued for additional 12 h. After evaporation of all volatiles, the product was extracted with hexane to yield ferrocenyl sulfonic acid chloride as a red-orange powder (4.3 g, 15 mmol, 45%).
1H NMR (300 MHz, CDCl3): δ [ppm] = 4.87 (m, 2H, cp.), 4.62 (m, 2H, cp), 4.47 (s, 5H, cp).
N-Ferrocenylsulfonyl-2-methylaziridine (fcMAz, 1).
rac-2-Methylaziridine (0.4 mL, 7 mmol) and 1.8 mL (18.8 mmol) N,N-diisopropylethylamine were dissolved in 10 mL dry dichloromethane and cooled to −30 °C. Ferrocenyl sulfonic acid chloride (2 g, 7 mmol) was dissolved in 10 mL dry dichloromethane and added dropwise over a period of ca. 20 min to the reaction mixture. The mixture was stirred for additional 90 min at −30 °C and allowed to warm up to 23 °C and stirred overnight. Then 20 mL saturated sodium hydrogen carbonate solution was added and stirred for 30 min. The aqueous phase was removed and the organic layer was washed again with brine, dried over magnesium sulfate and evaporated to dryness. Purification of the crude mixture by silica column chromatography (PE/EA 4
:
3, Rf = 0.7) yielded 1 as orange needles (2 g, 6.5 mmol, 94%).
1H NMR (300 MHz, 298 K, CDCl3): δ [ppm] = 4.74–4.68 (m, 2H, g), 4.48–4.44 (t, 2 H, f), 4.43 (s, 5H, e), 2.86–2.75 (m, 1 H, d), 2.50 (d, 1H, c), 2.00 (d, 1H, b), 1.27 (d, 1H, a). 13C NMR (176 MHz, 298 K, CDCl3): δ [ppm] = 149.81; 135.90; 124.04; 35.67; 34.72; 16.88. 15N NMR (71 MHz, 298 K, CDCl3): δ [ppm] = 81.80. ESI MS: theor: 305.173 g mol−1, found (m/z): 328.00 (M + Na+), 633.01 (2M + Na+) (Fig. S12†). Elemental analysis: calc.: C13H15NO2SFe = C: 51.16%, H: 4.95%, N: 4.59%, O: 10.49%, S: 10.51%, Fe: 18.3%. Found: C: 51.12%, H: 5.04%, N: 4.58%, S: 10.54%. R: ṽ: 3451 (b), 3105 (s), 2974 (m), 2932 (w), 1730 (b), 1449 (s), 1439 (s), 1411 (w), 1394 (s), 1362 (s), 1330 (ss), 1233 (s), 1196 (s), 1155 (s), 1130 (s), 1031 (s), 987 (s), 906 (s), 845 (ss), 773 (s), 691 (s), 636 (s), 618 (s) cm−1.
Results and discussion
Monomer synthesis
Substitution of the acidic proton at the aziridine is essential to enable its anionic polymerization and typically an electron-withdrawing group, such as a sulfonamide, is attached.9,18,24–26 For the first time, we demonstrate that a functional and stimuli-responsive group, i.e. a ferrocenyl substituent, can be coupled via a sulfonamide to the aziridine, allowing us to conduct an anionic polymerization. The synthesis starts with the preparation of ferrocenyl sulfonic acid chloride,22,23 which is then reacted with rac-2-methyl aziridine (Scheme 1), similar to a previously published procedure for tosyl- or mesyl-substituted aziridines.9 After purification by column chromatography (petroleum ether
:
ethyl acetate = 4
:
3, Rf = 0.7), N-ferrocenylsulfonyl-2-methylaziridine (1, fcMAz) is obtained in 94% yield (last step) as orange crystals (a photograph of 1 and poly(1) is shown in the ESI†).
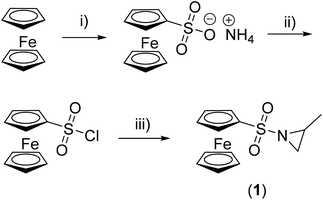 |
| Scheme 1 Synthesis of N-ferrocenylsulfonyl-2-methylaziridine (1) (i) acetic anhydride, chlorosulfonic acid, 20 h, room temperature, followed by NH4OH; (ii) oxalyl chloride, DCM, 0 °C; (iii) rac-2-methylaziridine, diisopropyl ethyl amine, DCM, −30 °C. | |
The 1H NMR spectrum of 1 (Fig. 2) shows the characteristic resonances of a substituted aziridine: three doublets at 1.27 ppm (a), 2.00 ppm (b), and 2.50 ppm (c) and a multiplet at 2.86–2.75 ppm (d) are indicative of the aziridine derivative. The resonance of the unsubstituted ring-methylenes splits into two signals due to coupling with the neighboring methine group at a stereo center. The resonances for the ferrocene group are detected at 4.4 ppm (singlet for the unsubstituted cp ring) and between 4.5 and 4.7 ppm (substituted cp ring). 15N NMR spectroscopy reveals a singlet at 81.8 ppm.
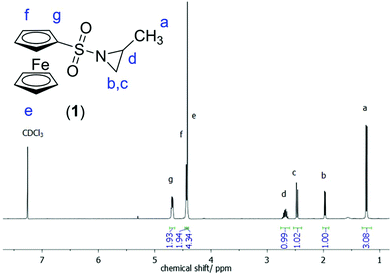 |
| Fig. 2
1H NMR spectrum of fcMAz (1) (CDCl3, 300 MHz, 298 K). | |
Polymerization
The aza-anionic polymerization of aziridines has been previously initiated by sulfonamide anions, such as potassium N-benzyl-mesyl amide. The initiator is generated in the reaction flask prior to the polymerization by deprotonation of the respective sulfonamide with potassium hexamethyldisilazide (KHMDS).18 Alternatively KHMDS can be used as the initiator itself. Also butyl lithium can be used as an initiator, as it was previously shown that aza-anions propagate with lithium counter ions.25 The polymerization of 1 was conducted under different conditions: polar solvents (DMF, THF, and DMSO) have been used, and the temperature, time (18–72 h) and initiators have been varied (Table 1, Scheme 2a).
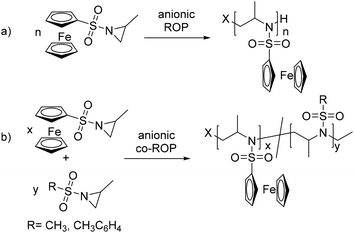 |
| Scheme 2 (a) Anionic ring-opening polymerization of PfcMAz (1) and its copolymerization (b) with MsMAz and TsMAz. | |
Table 1 Overview of the reaction conditions for the polymerization of 1
# |
DPa |
Solvent |
Initiatorb |
t/h |
Degree of polymerization (DP) theoretical.
Initiator system: 1: KHMDS, 2: potassium N-benzyl-mesyl amide, 3: n-BuLi, temperature 50 °C.
|
1 |
7 |
DMF |
2 |
18 |
2 |
16 |
DMF |
2 |
72 |
3 |
33 |
DMSO |
2 |
18 |
4 |
37 |
DMF |
2 |
72 |
5 |
7 |
DMF/DMSO 2 : 1 |
1 |
18 |
6 |
25 |
DMSO |
1 |
18 |
7 |
22 |
THF |
3 |
18 |
8 |
30 |
DMF |
3 |
72 |
In all cases, the polymer precipitated during the reaction from solution. The resulting P(fcMAz) was obtained as an orange powder in quantitative yields, but it was insoluble in all common solvents (DMF, DMSO, acetonitrile, n-hexane or THF, etc.). Also its oxidation with common oxidants, such as silver triflate or hydrogen peroxide, did not produce a soluble polymer. Monomer: initiator ratios of 1
:
7 to 1
:
40 were targeted and in all cases almost full monomer conversion was achieved (as no residual monomer was detectable in the reaction mixture); no higher degrees of polymerization were targeted, due to the formation of an insoluble polymer.
P(1) is the first reported insoluble racemic polymer prepared by anionic polymerization from a sulfonamide-substituted aziridine. Characterization of the homopolymers was conducted by solid state NMR spectroscopy (Fig. 2). The spectra indicate the successful formation of PAz. Due to the inherently low resolution of the 1H-SS-NMR spectrum, a clear signal assignment is not possible; however, the signals of the polymer backbone (1–3, in Fig. 2), of the fc moieties (4–13), and of the aromatic initiator (14) can be assumed. The 13C-SS-NMR reveals more details of the polymer structure: the initiator (14) can be detected at ca. 130 ppm; the signal of the sulfonamide-bound carbon atom of fc (4) occurs at ca. 90 ppm, with the remaining cp signals between 75–65 ppm; in addition, the backbone resonances between 60–45 ppm and the pendant methyl groups at 20–10 ppm are clearly separated (Fig. 3).
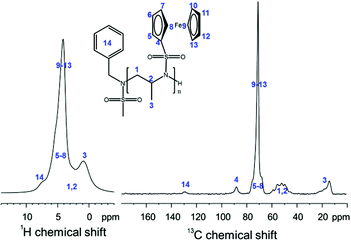 |
| Fig. 3 Solid state NMR of poly(1): 1H (left) and 13C NMR (right) spectra of PfcMAz33. | |
MALDI-TOF mass spectrometry proves the formation of the envisioned PfcMAz. The main distribution can be calculated to contain the mass of the initiator and the monomer mass (cf. Fig. 4) with a distance of 305 g mol−1, the molar mass of 1, up to a detectable molecular weight of ca. 7500 g mol−1. The maximum of the distribution corresponds to the molecular weight of 15 repeating units, the initiator and potassium as a counter ion.
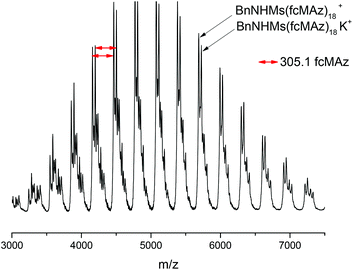 |
| Fig. 4 MALDI-TOF mass spectrum of PfcMAz33. | |
Block copolymer synthesis
In addition to the homopolymerization of 1, copolymers with N-mesyl-2-methylaziridine (MsMAz, 2) and N-tosyl-2-methylaziridine (TsMAz, 3) have been prepared, in order to produce soluble fc-containing PAz structures. Chain extension of living P(2) or P(3) with fcMAz to block copolymers was achieved; however, only short segments of PfcMAz could be realized, probably due to aggregation of the chain ends, leading to inactivation of the propagation. The 1H NMR spectra reveal the successful formation of a copolymer, with the resonances of both monomers detectable (Fig. 5 and S1†). In Fig. 5 the 1H NMR spectrum of P(TsMAz)22-b-P(fcMAz)5 initiated by KHMDS is shown: the tosyl resonances are detected between 8.31 and 7.16 ppm (h, g) and 2.74–2.1 (c), ferrocene signals (f) appear from 5.25 to 4.22 ppm, the polyaziridine backbone (e, d) at 4.22–2.79 ppm, and the pendant methyl groups (b) from 1.42 to 0.75 ppm. The SEC elugrams for all block copolymers prepared in this study show narrow molecular weight dispersities only for polymers with short fcMAz-segments detected from 1H NMR spectroscopy. For copolymers with several fcMAz units, the dispersity increases to ca. 1.4 with a bimodal shape of the elugram; this can be either rationalized by side reactions during the chain extension reaction, or – more probable – due to interactions or micellization during the polymerization and also the SEC measurement (Fig. S3†). MALDI-TOF mass spectrometry further proves the successful incorporation of both monomers into the polymer as the molecular weights of both monomers can be detected with peak distances of 305 g mol−1 (for 1) and 211 g mol−1 (for 3) from the spectrum (Fig. S2†) (Table 2).
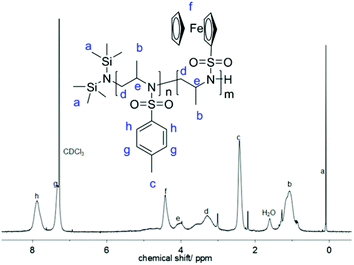 |
| Fig. 5
1H NMR spectrum of P(TsMAz)22-block-P(fcMAz)5 (CDCl3, 300 MHz, 298 K). | |
Table 2 Characterization of the chain extension experiments conducted with 1
# |
Polymer |
DPa |
M
n a |
M
n b |
Đ
|
M
n c |
DPc |
Degree of polymerization (DP) theoretical and theoretical molecular weight (Mn in g mol−1).
Determined by SEC in DMF.
Degree of polymerization (DP) and molecular weight (Mn in g mol−1) determined from 1H NMR.
Not determined.
|
1 |
P(2)37-b-P(1)3 |
54/6 |
9300 |
2700 |
1.07 |
5500 |
37/1 |
2 |
P(2)28-b-P(1)4 |
39/5 |
7000 |
2300 |
1.16 |
4600 |
28/2 |
3 |
P(3)22-b-P(1)5 |
22/5 |
6400 |
3000 |
1.25 |
|
|
4 |
P(3)25-b-P(1)6 |
25/6 |
7300 |
3400 |
1.36 |
|
|
Statistical copolymerization
The statistical copolymerization of 1 with 2 or 3 has been conducted, leading to higher fc-fractions (up to 58 wt% fc) in the final polymers (see below). In both cases, successful copolymerization with quantitative monomer incorporation is achieved; the 1H NMR spectra match the ones for the block copolymers. As the initiator signals are overlapping with the polymer signals, only the comonomer incorporation ratio was calculated, which is in agreement with the theoretical values. The molecular weight distributions for the statistical copolymers range between 1.3 and 1.4 with no significant correlation with the fcMAz content (Fig. S4†) (Table 3).
Table 3 Characterization data for statistical copolymers of 1 with 2 or 3
# |
Initiatora |
DPb |
M
n b |
M
n c |
Đ
|
M
n d |
DPd |
Ratioe |
Initiator system: 1: KHMDS, 2: KBnMs, 3: n-BuLi.
Degree of polymerization (DP) theoretical and theoretical molecular weight (Mn in g mol−1).
Determined by SEC in DMF.
Degree of polymerization (DP) and molecular weight (Mn in g mol−1) determined from 1H NMR.
Monomer ratio determined from 1H NMR.
Not determined.
|
P(329-co-19) |
1 |
29/9 |
9000 |
3400 |
1.3 |
|
|
3 : 1 |
P(332-co-118) |
2 |
32/18 |
12 500 |
4500 |
1.3 |
|
|
2 : 1 |
P(321-co-120) |
3 |
21/20 |
10 500 |
2500 |
1.3 |
|
|
5 : 4 |
P(253-co-118) |
3 |
53/18 |
12 700 |
2600 |
1.3 |
|
|
4 : 1 |
P(221-co-111) |
2 |
21/11 |
6400 |
3300 |
1.4 |
7200 |
27/11 |
5 : 2 |
The bimodal shape of the molecular weight distributions could be rationalized due to aggregation phenomena during the polymerization or side reactions with KHMDS as the initiator. 1H NMR spectra do not indicate side reactions, also the monomers are fully consumed at the end of the polymerization.
The Fe-content was also analyzed by UV/Vis spectroscopy monitoring the absorbance band at ca. 430 nm (Fig. S5†), matching the values from the NMR spectra.
Real-time 1H NMR kinetic studies of the copolymerization of 1 and 3 in DMSO were conducted. Monomer incorporation over time is measured by integration of the methylene resonances for both monomers (Fig. 6, 2.3 ppm for 1, and 2.16 ppm for 3). The integration revealed that the polymerization of 3 is faster than that of 1, indicating the formation of a gradient copolymer after the copolymerization process. From these spectra the monomer incorporation rates during the copolymerization can be calculated (Fig. 6 and S6–S8†).
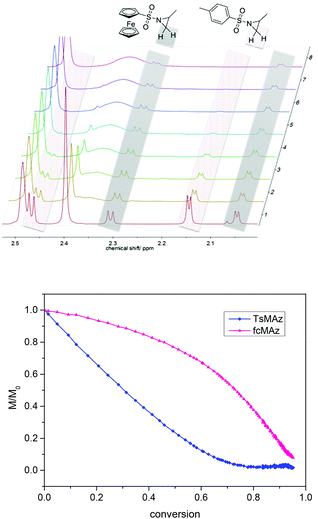 |
| Fig. 6 Top: Real-time 1H-NMR-kinetics for P(TsMAz30-co-fcMAz12) (time interval between the spectra 40 min). Bottom: Monomer conversion determined from NMR for TsMAz and fcMAz in Poly(TsMAz30-co-fcMAz12). | |
Thermal analysis
The thermal properties of the fc-containing PAz were analyzed by DSC and TGA (cf. Fig. S9 and S10†). All polymers are amorphous materials with glass transition temperatures in the range of 123 and 144 °C which rises with the increasing content of fc in the copolymers (both for copolymers with 2 and 3, Table 4). For comparison also homopolymers P(1) (Tg = 56 °C), P(2) (Tg = 137 °C), and P(3) (Tg = 144 °C) have been prepared.
Table 4 Thermal data of fc-containing PAz
# |
Wt% fc-monomera |
T
g b/°C |
Weight % 1 incorporated.
Glass transition temperature, determined from DSC.
|
P(1) |
100 |
56 |
P(244) |
0 |
137 |
P(253-co-118) |
43 |
123 |
P(227-co-111) |
52 |
133 |
P(374) |
0 |
144 |
P(329-co-19) |
30 |
123 |
P(330-co-113) |
37 |
139 |
P(332-co-118) |
44 |
143 |
P(321-co-120) |
58 |
144 |
P(322-b-P15) |
19 |
134 |
All polymers exhibit a similar thermal stability with a beginning mass loss at ca. 350 °C of 53–68 wt%. This weight loss is probably due to the release of the sulfonamide groups. We assume that ferrocene is not eliminated as the polymers with higher iron-content reveal a higher char residue, indicating the formation of iron-containing char. The char residue is 24–36% (36% for the homopolymers poly(1)) rather high, making them also interesting candidates for fc-containing performance materials.
Electrochemical behavior
The redox-profiles of all fc-containing PAz were analyzed by cyclic voltammetry (CV) in dichloromethane with tetrabutylammonium hexafluorophosphate as the conducting salt. All polymers exhibit the reversible oxidation of fc, regardless of their microstructure. Fig. 7 exemplarily shows the CV curves at different scan rates of P(TsMAz32-co-fcMAz18) proving the ideal reversibility of the redox profile.
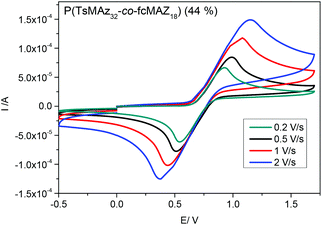 |
| Fig. 7 Cyclic voltammograms of P(TsMAz32-co-fcMAz18) at different scan rates. | |
Conclusions
This work presents the synthesis and polymerization of the first N-sulfonyl-substituted ferrocene-containing aziridine: N-ferrocenylsulfonyl-2-methylaziridine. The monomer is accessible in high yield from ferrocenyl sulfonyl chloride and rac-2-methyl aziridine as an orange powder. An insoluble organometallic polymer is prepared via the anionic polymerization, which was characterized by solid-state NMR and mass spectrometry, indicating the formation of the desired polysulfonamide. Copolymers with other aziridine monomers were prepared in order to produce soluble materials. For the statistical copolymerization of N-ferrocenylsulfonyl-2-methylaziridine with N-tosyl-2-methylaziridine real-time 1H NMR kinetics proved the formation of gradient copolymers with the tosyl-aziridine being incorporated as the first segment. Thermal data reveal the high stability of the polymers (Tonca. 350 °C) with a rather high Tg (120–140 °C) depending on the fc-ratio; electrochemical data reveal the reversible redox profile of fc.
These results broaden the scope of fc-containing monomers, which may be combined with other anionic polymerization techniques in the future to prepare redox-responsive materials.
Acknowledgements
The authors thank the Deutsche Forschungsgemeinschaft (DFG-WU 750/7-1) for funding.
References
- R. D. A. Hudson, J. Organomet. Chem., 2001, 637–639, 47–69 CrossRef CAS
.
- P. Nguyen, P. Gómez-Elipe and I. Manners, Chem. Rev., 1999, 99, 1515–1548 CrossRef CAS PubMed
.
-
J. E. Sheats, C. E. Carraher Jr. and C. U. Pittman, Metal-containing polymeric systems, Springer Science & Business Media, 2012 Search PubMed
.
- M. G. Baldwin and K. E. Johnson, J. Polym. Sci., Part A-1: Polym. Chem., 1967, 5, 2091–2098 CrossRef CAS
.
- A. D. Russell, R. A. Musgrave, L. K. Stoll, P. Choi, H. Qiu and I. Manners, J. Organomet. Chem., 2015, 784, 24–30 CrossRef CAS
.
- A. Alkan, L. Thomi, T. Gleede and F. R. Wurm, Polym. Chem., 2015, 6, 3617–3624 RSC
.
- A. Alkan, C. Steinmetz, K. Landfester and F. R. Wurm, ACS Appl. Mater. Interfaces, 2015, 7, 26137–26144 CAS
.
- C. Tonhauser, A. Alkan, M. Schoemer, C. Dingels, S. Ritz, V. Mailaender, H. Frey and F. R. Wurm, Macromolecules, 2013, 46, 647–655 CrossRef CAS
.
- E. Rieger, A. Alkan, A. Manhart, M. Wagner and F. R. Wurm, Macromol. Rapid Commun., 2016, 37, 833–839 CrossRef CAS PubMed
.
- A. Padwa and S. S. Murphree, ARKIVOC, 2006, 3, 6–33 Search PubMed
.
- M. Jäger, S. Schubert, S. Ochrimenko, D. Fischer and U. S. Schubert, Chem. Soc. Rev., 2012, 41, 4755–4767 RSC
.
- B. D. Monnery and R. Hoogenboom, Cationic Polym. Regener. Med., 2014, 30–61 Search PubMed
.
- Ö. Doğan, S. Zeytinci and A. Bulut, Synth. Commun., 2005, 35, 1067–1076 CrossRef
.
- B. F. Bonini, M. Fochi, M. Comes-Franchini, A. Ricci, L. Thijs and B. Zwanenburg, Tetrahedron: Asymmetry, 2003, 14, 3321–3327 CrossRef CAS
.
- M.-C. Wang, D.-K. Wang, Y. Zhu, L.-T. Liu and Y.-F. Guo, Tetrahedron: Asymmetry, 2004, 15, 1289–1294 CrossRef CAS
.
- M.-C. Wang, L.-T. Liu, J.-S. Zhang, Y.-Y. Shi and D.-K. Wang, Tetrahedron: Asymmetry, 2004, 15, 3853–3859 CrossRef CAS
.
- J. B. Sweeney, Chem. Soc. Rev., 2002, 31, 247–258 RSC
.
- I. C. Stewart, C. C. Lee, R. G. Bergman and F. D. Toste, J. Am. Chem. Soc., 2005, 127, 17616–17617 CrossRef CAS PubMed
.
- S. A. Merchant, D. T. Glatzhofer and D. W. Schmidtke, Langmuir, 2007, 23, 11295–11302 CrossRef CAS PubMed
.
- M. T. Meredith, D.-Y. Kao, D. Hickey, D. W. Schmidtke and D. T. Glatzhofer, J. Electrochem. Soc., 2011, 158, B166–B174 CrossRef CAS
.
- S. A. Merchant, T. O. Tran, M. T. Meredith, T. C. Cline, D. T. Glatzhofer and D. W. Schmidtke, Langmuir, 2009, 25, 7736–7742 CrossRef CAS PubMed
.
- M. Herberhold, O. Nuyken and T. P. Öhlmann, J. Organomet. Chem., 1995, 501, 13–22 CrossRef CAS
.
- M. Jonek, A. Makhloufi, P. Rech, W. Frank and C. Ganter, J. Organomet. Chem., 2014, 750, 140–149 CrossRef CAS
.
- L. Thomi and F. R. Wurm, Macromol. Symp., 2015, 349, 51–56 CrossRef CAS
.
- L. Thomi and F. R. Wurm, Macromol. Rapid Commun., 2014, 35, 585–589 CrossRef CAS PubMed
.
- E. Rieger, A. Manhart and F. R. Wurm, ACS Macro Lett., 2016, 5, 195–198 CrossRef CAS
.
Footnote |
† Electronic supplementary information (ESI) available. See DOI: 10.1039/c6py01019a |
|
This journal is © The Royal Society of Chemistry 2016 |