DOI:
10.1039/C6OB00023A
(Communication)
Org. Biomol. Chem., 2016,
14, 1930-1933
Synthesis and evaluation of a desymmetrised synthetic lectin: an approach to carbohydrate receptors with improved versatility†
Received
6th January 2016
, Accepted 14th January 2016
First published on 14th January 2016
Abstract
A new synthetic lectin features apolar surfaces provided by two different aromatic components (biphenyl and pyrenyl). Affinities up to 260 M−1 are recorded for carbohydrates in water. The desymmetrised design has potential for variation to give receptors with a broadened range of capabilities.
Carbohydrates are versatile biomolecules employed for diverse purposes ranging from energy management and large-scale construction to protein labelling and cell–cell signalling.1 Carbohydrate-binding proteins (lectins) are widely used to help investigate these processes. However the natural lectins are not always ideal as research tools, and there is potential for synthetic analogues to play complementary roles.1a,e Carbohydrate recognition in water is challenging due to the hydromimetic exterior of carbohydrates;1e,2 even natural lectins tend to bind their substrates quite weakly.3 Nonetheless, if the problem can be solved, synthetic carbohydrate receptors4 (“synthetic lectins”) could be valuable in glycobiological research, and may ultimately be exploited in medicine.4b,5
In recent years we have reported a series of synthetic lectins based on the “temple” design strategy illustrated in Fig. 1a.2,4a,6 Amphiphilic cavities have been constructed from two aromatic surfaces held apart by isophthalamide pillars. The aromatic surfaces can help to bind a carbohydrate substrate through hydrophobic and CH–π interactions, while the isophthalamide pillars provide amide groups which can hydrogen bond to polar groups on the substrate. Variations have included tricyclic, bicyclic and monocyclic frameworks (e.g.3–5),6a,f,j but all have featured two identical apolar surfaces. This arrangement is complementary to all-equatorial carbohydrates, such as β-glucosyl (1) and β-N-acetylglucosaminyl (β-GlcNAc) (2), which possess two roughly similar hydrophobic faces. Accordingly, the receptors have shown good affinities and excellent selectivities for this family of substrates.
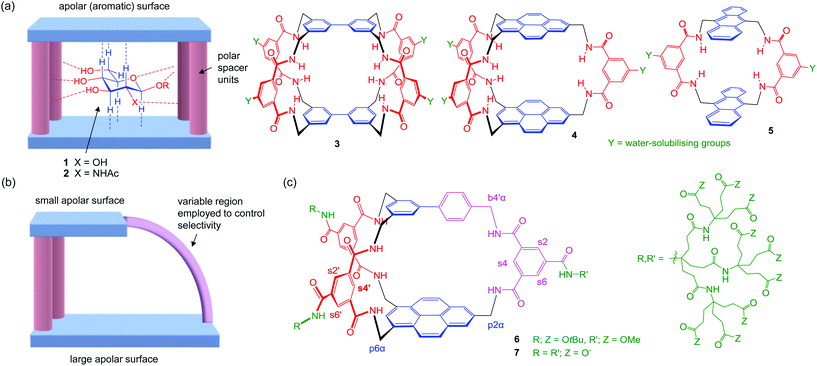 |
| Fig. 1 (a) Design strategy for “temple” receptors targeting all-equatorial carbohydrates. Left: cartoon representation, shown binding β-glucosyl 1 and β-GlcNAc 2. Right: reported examples 3–5. (b) Asymmetric architecture with potential for greater versatility. The variable region may be used to accommodate and/or bond to axial carbohydrate substituents. (c) Prototype 7 and protected precursor 6, showing labels for selected protons (p = pyrenyl, b = biphenyl, s = spacer). The complete labelling system is given in the ESI.† | |
Having succeeded with the all-equatorial saccharides, we would like to expand the scope of these “synthetic lectins” so that a wider range of targets can be addressed. Selectivity for other carbohydrates requires that axial polar substituents should be accommodated. In most cases this will disturb the balance between the faces of the carbohydrate, one becoming more polar while the other gains hydrophobicity. Cavities with matching apolar surfaces are no longer appropriate, but receptors built from two different apolar units (e.g. one large, one small) have potential to succeed. Here we report the first examples of a new generation of desymmetrised carbohydrate receptors with differing roof and floor. This architecture promises greater versatility than the original temple receptors, and could eventually form a family of synthetic lectins with a range of complementary selectivities.
Our approach to designing these new receptors is illustrated in Fig. 1b. A bicyclic structure was chosen to favour a preorganised cavity while avoiding the synthetic challenge of higher connectivity. Preorganisation would be assisted by employing a rigid, condensed aromatic unit as the larger apolar surface. At one end of the cavity two pillars would connect the large and small apolar surfaces, while the third linkage would be variable. This could allow space to accommodate axial substituents in the substrate and otherwise tune selectivity. Our prototype design 7 is shown in Fig. 1c. The rigid floor is provided by the trisubstituted pyrene unit employed in 4.6j The spacers are isophthalamides with water-solubilising substituents as employed in earlier designs, and the smaller apolar unit is a benzene ring. For this initial work we chose a variable region that would limit flexibility while maintaining ease of synthesis, i.e. a p-phenylene linked to a third isophthalamide. The required biphenyl unit was readily accessible, having previously been used in symmetrical receptor 16.6h
Macrobicycle 7 was synthesised as shown in Scheme 1.7 Biphenyl 10 was obtained by Suzuki coupling of 88 and 9,6g followed by a Staudinger reaction with trimethylphosphine. The linear intermediate 14 was then constructed by treating activated ester 116j first with 10 and then with pyrenyl amine 13.6j Methyl ester protection was employed in 11 to allow the use of acid-labile NHBoc groups in 10 and 13. Removal of the Boc groups was followed by macrocyclisation under high dilution with activated ester 126i to give protected receptor 6. As discussed later, macrobicycle 6 was accompanied by a side product which necessitated careful separation by HPLC, resulting in a low isolated yield of 5%. However, sufficient material was obtained for characterisation and further studies. Treatment of 6 with TFA, removing O-tBu protection, was followed by basic hydrolysis of the methyl ester groups with NaOH. Receptor 7 was isolated from excess salt by acidification with ion exchange resin to give the heptacosa-carboxylic acid. The acid was then dissolved in D2O by adjusting the pH to ∼7 with NaOD. The solutions gave well-resolved 1H NMR spectra which showed some concentration-dependence but were largely unchanged below 0.35 mM.7 Application of 2D NOESY, COSY and TOCSY methods allowed a full assignment of all aromatic and benzylic proton signals.
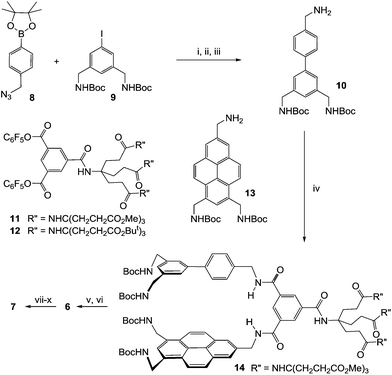 |
| Scheme 1 (i) K2CO3, Pd(PPh3)4, THF/H2O, 12 h, 60 °C; (ii) PMe3, THF, 2 h, 60 °C; (iii) H2O, THF, 12 h, 60 °C; 33% over steps i-iii; (iv) 10 + 11 + DIPEA, THF, RT, 30 min, then 13, RT, 30 min, 55%; (v) TFA, DCM, 0 °C; 3 h; (vi) DIPEA + 12, THF (high dilution), RT, 120 h, 5% over steps v and vi after HPLC separation from side products; (vii) TFA, DCM, 0 → 25 °C, 12 h; (viii) NaOH aq. (5 M); (ix) amberlyst 15 resin H-form, to pH < 3; (x) H2O, NaOH aq. to pH 7.1; ∼100% over steps vii–x. | |
The binding properties of 7 towards carbohydrate substrates were investigated using 1H NMR titrations.7 Addition of carbohydrates to solutions of 7 in D2O produced changes implying complex formation with fast exchange on the NMR chemical shift timescale. A series of spectra from the addition of cellobiose 15 to 7 are shown in Fig. 2. Signal movements were observed for receptor protons from all the aromatic units (pyrene, biphenyl and isophthaloyl spacer). The signal due to internally directed spacer protons s4′ showed especially large movements and could be followed throughout the titration. Analysis of the chemical shift changes (Δδ) according to a 1
:
1 binding model yielded a binding constant of 260 M−1. Similar analyses were performed for mannose, galactose, methyl β-glucoside and methyl β-N-acetylglucosaminide (GlcNAc–β-OMe). The results are summarised in Table 1, where they are compared to those obtained from tricyclic biphenyl-based 3, bicyclic pyrene-based 4 and bicyclic biphenyl-based 16.6h As might be expected, the affinities of 7 for all-equatorial substrates such as 15 are intermediate between bis-biphenyl 16 and bis-pyrenyl 4. The asymmetry of 7 has had no clear effect on the selectivity, as substrates with axial OH groups (mannose and galactose) are still bound only weakly. However, this is not unexpected as the variable region of 7 was not specifically designed to accommodate a hydroxyl group.
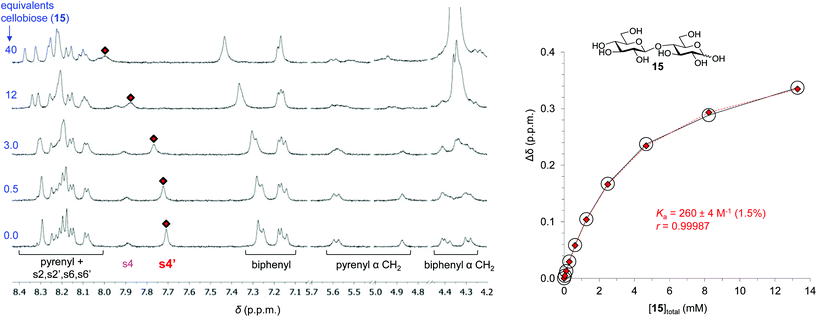 |
| Fig. 2 Left: partial 1H NMR spectra from the addition of D-cellobiose 15 to receptor 7 in D2O. For proton labelling see Fig. 1c. Right: binding analysis curve for the signal due to s4′. The red diamonds ( ) and white circles (○) represent the observed and calculated data based on a 1 : 1 binding model. | |
Table 1 Association constants measured for 7 and some published receptors to carbohydrates in D2O
Carbohydrate |
K
a a [M−1] |
3
6e
|
16
6h
|
7
|
4
6j
|
Measured by NMR titration at 298 K. Estimated errors typically <2–5% for values obtained in the present work.
|
D-Mannose |
∼0 |
∼0 |
4 |
5 |
D-Galactose |
2 |
∼0 |
4 |
18 |
Methyl β-D-glucoside (1, R = Me) |
28 |
5 |
20 |
240 |
Methyl β-D-N-acetylglucosaminide (2, R = Me) |
630 |
19 |
38 |
270 |
D-Cellobiose 15 |
17 |
52 |
260 |
3900 |
Finally, as mentioned earlier, the synthesis of
7 was complicated by a side-product which appeared in the macrocyclisation step leading to
O-protected precursor
6. Separation from
6 was challenging but could be achieved using an HPLC stationary phase bearing C
18 chains terminated with pentafluorophenyl groups.
7 The side-product was obtained in a similar yield to
6 (∼6%). NMR spectra were consistent with a bicyclic cage structure closely related to
6 but lacking a proton at C1 of the pyrene nucleus. Mass spectroscopy revealed a molecular weight greater than
6 by 32 mass units, and we concluded that the most likely structure was hydroperoxide
17. We presume that the hydroperoxide group was introduced
via autoxidation, although there seem to be no previous examples for this process in pyrenes and very few in other condensed aromatics.
9 However there is precedent to suggest that molecular oxygen might show enhanced reactivity within certain macrocyclic cavities,
10 and this may represent a further example.
O-Deprotection of
17 as for
6 gave a water-soluble cage which behaved similarly to
7, giving well-resolved NMR spectra in D
2O and serving as a carbohydrate receptor.
11 Indeed affinities for some carbohydrates were slightly higher than for
7.
7 For example the
Ka to cellobiose
15 was measured at 335 M
−1, perhaps reflecting the advantage of an electron-donating oxygen substituent.
12
In conclusion we have demonstrated a synthetic strategy for desymmetrised carbohydrate receptors with potential for greater scope than presently available synthetic lectins. The yield of prototype
7 was limited by autoxidation, but this problem is unlikely to be general and should be controllable where necessary. The structure of
7 should be variable through incorporation of different roof units, perhaps in library format. This should allow tuning of the binding site to accept a greater range of carbohydrates. A further option is the construction of functionalised binding sites capable of stabilising transition states (
e.g. for glycoside hydrolysis), leading to enzyme-like catalysis. Future work will be aimed at realising these possibilities.
Acknowledgements
This research was supported by the Engineering and Physical Sciences Research Council through grant number EP/I028501/1.
Notes and references
-
(a) D. Solis, N. V. Bovin, A. P. Davis, J. Jiménez-Barbero, A. Romero, R. Roy, K. Smetana and H. J. Gabius, Biochim. Biophys. Acta, Gen. Subj., 2015, 1850, 186–235 CrossRef CAS PubMed;
(b)
H. J. Gabius, The Sugar Code: Fundamentals of Glycosciences, Wiley-VCH, Weinheim, 2009 Search PubMed;
(c) C. A. Aarnoudse, J. J. G. Vallejo, E. Saeland and Y. van Kooyk, Curr. Opin. Immunol., 2006, 18, 105–111 CrossRef CAS PubMed;
(d) C. R. Bertozzi and L. L. Kiessling, Science, 2001, 291, 2357–2364 CrossRef CAS PubMed;
(e)
B. Wang and G. J. Boons, Carbohydrate Recognition: Biological Problems, Methods, and Applications, John Wiley & Sons Ltd., New Jersey, 2011 Search PubMed.
- A. P. Davis, Org. Biomol. Chem., 2009, 7, 3629–3638 CAS.
- E. J. Toone, Curr. Opin. Struct. Biol., 1994, 4, 719–728 CrossRef CAS.
- For reviews on synthetic carbohydrate receptors see:
(a) A. P. Davis and R. S. Wareham, Angew. Chem., Int. Ed., 1999, 38, 2978–2996 CrossRef;
(b) S. Jin, Y. F. Cheng, S. Reid, M. Y. Li and B. H. Wang, Med. Res. Rev., 2010, 30, 171–257 CAS;
(c) M. Mazik, Chem. Soc. Rev., 2009, 38, 935–956 RSC;
(d) Y. Nakagawa and Y. Ito, Trends Glycosci. Glycotechnol., 2012, 24, 1–12 CrossRef CAS;
(e) C. E. Miron and A. Petitjean, ChemBioChem, 2015, 16, 365–379 CrossRef CAS PubMed. Recent examples:
(f) N. Chandramouli, Y. Ferrand, G. Lautrette, B. Kauffmann, C. D. Mackereth, M. Laguerre, D. Dubreuil and I. Huc, Nat. Chem., 2015, 7, 334–341 CrossRef CAS PubMed;
(g) Y. Jang, R. Natarajan, Y. H. Ko and K. Kim, Angew. Chem., Int. Ed., 2014, 53, 1003–1007 CrossRef CAS PubMed;
(h) J. Lippe and M. Mazik, J. Org. Chem., 2015, 80, 1427–1439 CrossRef CAS PubMed;
(i) O. Francesconi, M. Gentili, C. Nativi, A. Arda, F. J. Canada, J. Jiménez-Barbero and S. Roelens, Chem. – Eur. J., 2014, 20, 6081–6091 CrossRef CAS PubMed;
(j) M. Rauschenberg, S. Bandaru, M. P. Waller and B. J. Ravoo, Chem. – Eur. J., 2014, 20, 2770–2782 CrossRef CAS PubMed;
(k) A. Schmitt, O. Perraud, E. Payet, B. Chatelet, B. Bousquet, M. Valls, D. Padula, L. Di Bari, J. P. Dutasta and A. Martinez, Org. Biomol. Chem., 2014, 12, 4211–4217 RSC;
(l) S. M. Bromfield, P. Posocco, C. W. Chan, M. Calderon, S. E. Guimond, J. E. Turnbull, S. Pricl and D. K. Smith, Chem. Sci., 2014, 5, 1484–1492 RSC;
(m) S. Rieth, M. R. Miner, C. M. Chang, B. Hurlocker and A. B. Braunschweig, Chem. Sci., 2013, 4, 357–367 RSC.
- J. Balzarini, Nat. Rev. Microbiol., 2007, 5, 583–597 CrossRef CAS PubMed.
-
(a) E. Klein, M. P. Crump and A. P. Davis, Angew. Chem., Int. Ed., 2005, 44, 298–302 CrossRef CAS PubMed;
(b) Y. Ferrand, M. P. Crump and A. P. Davis, Science, 2007, 318, 619–622 CrossRef CAS PubMed;
(c) E. Klein, Y. Ferrand, E. K. Auty and A. P. Davis, Chem. Commun., 2007, 2390–2392 RSC;
(d) N. P. Barwell, M. P. Crump and A. P. Davis, Angew. Chem., Int. Ed., 2009, 48, 7673–7676 CrossRef CAS PubMed;
(e) Y. Ferrand, E. Klein, N. P. Barwell, M. P. Crump, J. Jiménez-Barbero, C. Vicent, G.-J. Boons, S. Ingale and A. P. Davis, Angew. Chem., Int. Ed., 2009, 48, 1775–1779 CrossRef CAS PubMed;
(f) C. Ke, H. Destecroix, M. P. Crump and A. P. Davis, Nat. Chem., 2012, 4, 718–723 CrossRef CAS PubMed;
(g) B. Sookcharoenpinyo, E. Klein, Y. Ferrand, D. B. Walker, P. R. Brotherhood, C. Ke, M. P. Crump and A. P. Davis, Angew. Chem., Int. Ed., 2012, 51, 4586–4590 CrossRef CAS PubMed;
(h) J. D. Howgego, C. P. Butts, M. P. Crump and A. P. Davis, Chem. Commun., 2013, 49, 3110–3112 RSC;
(i) H. Destecroix, C. M. Renney, T. J. Mooibroek, T. S. Carter, P. F. N. Stewart, M. P. Crump and A. P. Davis, Angew. Chem., Int. Ed., 2015, 54, 2057–2061 CrossRef CAS PubMed;
(j) T. J. Mooibroek, J. M. Casas-Solvas, R. L. Harniman, C. M. Renney, T. S. Carter, M. P. Crump and A. P. Davis, Nat. Chem., 2016, 8, 69–74 CrossRef CAS PubMed.
- For details see ESI.†.
- J. R. White, G. J. Price, S. Schiffers, P. R. Raithby, P. K. Plucinski and C. G. Frost, Tetrahedron Lett., 2010, 51, 3913–3917 CrossRef CAS.
- Only two examples were found in Reaxys, both relating to electron-rich tricyclic aromatic systems. See: J. Rigaudy and G. Izoret, C. R. Acad. Sci., 1954, 238, 824–826 CAS; R. Knoesel, B. Gebus and J. Parrod, Bull. Soc. Chim. Fr., 1971, 4471 Search PubMed.
- J. J. Gassensmith, J. M. Baumes, J. Eberhard and B. D. Smith, Chem. Commun., 2009, 2517–2519 RSC.
- The identity of this water-soluble receptor is uncertain, as the hydroperoxide unit may not have survived the deprotection step. Unfortunately we were unable to obtain MS data for the deprotected cage, a common problem with our polycarboxylic-substituted receptors. For further discussion see ESI.†.
- N. P. Barwell and A. P. Davis, J. Org. Chem., 2011, 76, 6548–6557 CrossRef CAS PubMed; K. L. Hudson, G. J. Bartlett, R. C. Diehl, J. Agirre, T. Gallagher, L. L. Kiessling and D. N. Woolfson, J. Am. Chem. Soc., 2015, 137, 15152–15160 CrossRef PubMed.
Footnote |
† Electronic supplementary information (ESI) available: Experimental details for syntheses and measurements of binding constants. See DOI: 10.1039/c6ob00023a |
|
This journal is © The Royal Society of Chemistry 2016 |