Nitrogen positional scanning in tetramines active against HIV-1 as potential CXCR4 inhibitors†
Received
24th November 2015
, Accepted 10th December 2015
First published on 10th December 2015
Abstract
The paradigm, derived from bicyclams and other cyclams, by which it is necessary to use the p-phenylene moiety as the central core in order to achieve high HIV-1 antiviral activities has been reexamined for the more flexible and less bulky structures 4, previously described by our group as potent HIV-1 inhibitors. The symmetrical compounds 7{x,x} and the non-symmetrical compounds 8{x,y} were designed, synthesized and biologically evaluated in order to explore the impact on the biological activity of the distance between the phenyl ring and the first nitrogen atom of the side chains. EC50 exactly followed the order 7{x,x} < 8{x,x} < 4{x,x} indicating that, for such flexible tetramines, the presence of two methylene units on each side of the central phenyl ring increases the biological activity contrary to AMD3100. A computational study of the interactions of 4{3,3}, 7{3,3} and 8{3,3} with CXCR4 revealed interactions in the same pocket region with similar binding modes for 4{3,3} and 7{3,3} but a different one for 8{3,3}.
Introduction
Since the discovery of the bicyclam AMD3100 (1, plerixafor, EC50 = 0.002 μM),1,2 the first small molecule CXCR4 inhibitor, a lot of efforts have been devoted to develop more potent, selective and less toxic inhibitors. Such research has included the synthesis of monocyclam derivatives (AMD3465,3 AMD3451,4…) and non-cyclam derivatives (AMD070,52,63,7…) (Fig. 1).
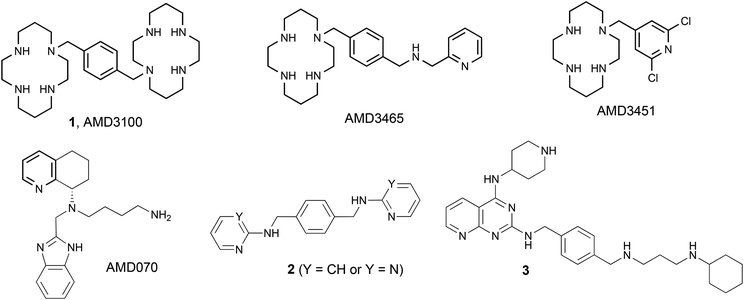 |
| Fig. 1 Structures of the bicyclam AMD3100 (1), monocyclams AMD3465 and AMD3451, and non-cyclam CXCR4 inhibitors AMD070, 2, and 3. | |
The reason for such interest was, initially, the use by the human immunodeficiency virus (HIV) of the chemokine receptor CXCR4 as a coreceptor during the HIV cell fusion and entry, which starts with the interaction of the virus envelop glycoprotein gp120 with the primary receptor CD4.8 However, more recently, CXCR4 has been connected with the regulation of the trafficking and homing of various cell types including stem cells and cancer stem cells. Among others, the CXCL12/CXCR4 pathway9 promotes tumor vasculogenesis and angiogenesis in tumor stem-like glioma cells.10 In fact plerixafor (1, AMD3100) has been commercialized (trade name Mozobil) as an immunostimulant used to mobilize stem cells to the peripheral blood for collection and subsequent autologous stem-cell transplantation.2
In this context, our work in the field of anti-HIV entry/fusion inhibitors11–13 identified novel potent CXCR4 coreceptor inhibitors of the general structure 4 (Fig. 2). Among these tetramines, compound 5 was selected as the lead compound because it shows almost the same level of activity (5, EC50 = 0.019 μM) as the reference compound 1 and shows no cell toxicity at concentrations up to 25 μg mL−1.11 More recently, we have synthesized three stereoisomers of compound 5 (5(R,R), 5(S,S), and the meso form 5(S,R)) and evaluated their effect on glioma initiating cells (GICs) in comparison with the prototype compound AMD3100.14
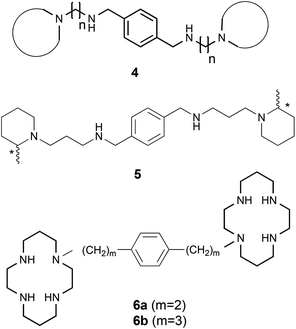 |
| Fig. 2 General structures of tetramines 4, compound 5, and AMD3100 analogues 6. | |
Through our research and previous ones, a paradigm has been widely accepted: to use the p-phenylene moiety as the central core for the connection of nitrogen heterocycles linked to two nitrogen atoms on both sides of such a core. This paradigm, the presence of a single carbon atom between the central phenyl ring and the first nitrogen of the side chain, comes from the study of Bridger et al.15 who showed that, in the case of analogues 6 (Fig. 2), the HIV-1 antiviral activity of 6a (m = 2; EC50 = 14.8 μM) was 3–4 orders of magnitude less effective than AMD3100 although in the case of 6b (m = 3; EC50 = 0.4 μM) the diminution was not so clear.
Our convincement that such a paradigm could not be necessarily valid for structures less hindered than AMD3100 led us to carry out a positional scanning of the nitrogen atoms present on each side of the central phenyl ring of structures 4 in order to explore the impact on the biological activity of the distance between the phenyl ring and the first nitrogen atom of the side chains.
The present paper describes the design, synthesis and biological evaluation of two families of compounds: the symmetrical compounds 7 (in which m = 2 on both sides) and the non-symmetrical compounds 8 (in which m = 1, on one side, and m = 2, on the other side) (Fig. 3).
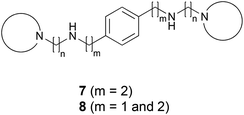 |
| Fig. 3 General structures of the new tetramines 7 and 8. | |
Results and discussion
Compounds 4 containing the p-phenylene moiety as the central core were synthesized using reductive amination starting from terephthalaldehyde, to afford symmetrical compounds using two equivalents of the same primary amine, or 4-(diethoxymethyl)benzaldehyde which allows the stepwise introduction of two different primary amines to afford non-symmetrical compounds.11 However, in the case of compounds 7 and 8 it was not possible to use the same synthetic strategy because the corresponding required dialdehydes, 1,4-phenylendiacetaldehyde and 4-(formylmethyl)benzaldehyde, respectively, were not commercially available. Moreover, it was not possible to synthesize them because of their described instability that was also observed in our hands.16,17 Consequently, it was necessary to develop alternative synthetic routes for 7 and 8.
For the synthesis of the symmetrical tetramines 7{x,x}, we decided to start from 1,4-benzenediacetic acid (9) (Scheme 1) which was transformed to the diacyl dichloride 11 in two steps. Therefore, compound 9 was converted into the bis(trimethylsilyl) 1,4-benzenediacetate (10) upon treatment with hexamethyldisilazane (HMDS)/Me3SiCl/1,2-dichloroethane as previously described by Falck-Pedersen et al.18 The subsequent treatment of 10 with oxalyl chloride/DMF/CH2Cl2 yielded 1,4-benzenediacetyl chloride (11) which was converted to the corresponding diamides 13{x,x} upon treatment with two equivalents of the respective amines 12{x}. Finally, the reduction of amides 13{x,x} with BH3·SMe2 in THF yielded the desired symmetrical tetramines 7{x,x} (Scheme 1).
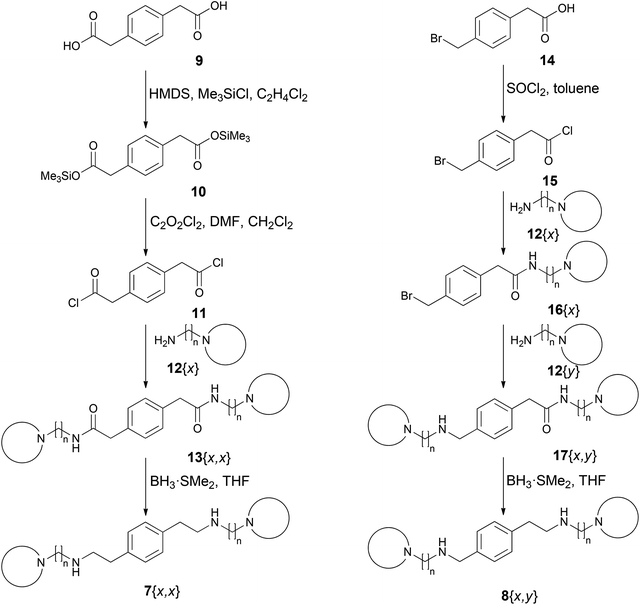 |
| Scheme 1 Synthetic protocols for the preparation of symmetrical and non-symmetrical diamines 7{x,x} and 8{x,y}, respectively. | |
In order to obtain the non-symmetrical compounds 8{x,y}, we selected 2-(4-(bromomethyl)phenyl)acetic acid (14) as the starting material (Scheme 1) due to the different reactivity of the two ends of the molecule which allows the derivatization of both positions with the same or different amines 12{x} to afford compounds 8{x,x} and 8{x,y}, respectively. Thus, the first amine 12{x} was introduced by acylation with acid chloride 15, prepared by treating 14 with SOCl2/toluene. Subsequently, a second amine unit 12{x} or 12{y} was introduced by nucleophilic substitution of the benzyl bromine atom of the intermediates 16{x}. Finally, the reduction of the resulting compounds 17{x,x} or 17{x,y} with BH3·SMe2 yielded the desired non-symmetrical compounds 8{x,x} or 8{x,y}, respectively (Scheme 1).
A second and important part of our positional scanning of the nitrogen atoms present on each side of the central phenyl ring was the selection of the amines 12{x} (Fig. 4). Such amines are constituted by a nitrogen-containing heterocyclic system (pipecoline, piperidine, piperazine, morpholine, pyrrolidine, and imidazole) linked to a –(CH2)n– spacer (where n = 2, 3 or 4) which ends in an NH2 group. Some of these compounds are commercially available, others were previously obtained by our group for the synthesis of tetramines 4{x,y}11 but some of them have been synthesized specially for this work. This was the case of 12{5} that was obtained using the same protocol previously described by our group.11 Amines 12{10} and 12{11} in which n = 4 were synthesized by alkylation of the corresponding nitrogenated heterocycle, 2-methylpiperidine (19{10}) and piperidine (19{11}), with the bromoalkyl phthalimide 18 to afford the intermediates 20{10} and 20{11}. The subsequent acid hydrolysis of the phthalimido protecting group yielded the desired amines 12{10} and 12{11} (Scheme 2).
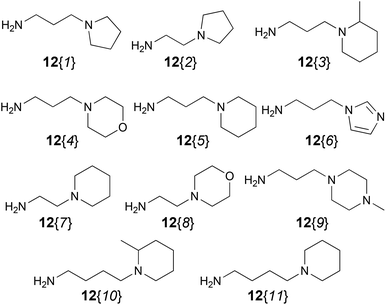 |
| Fig. 4 Amines 12{x} used for the synthesis of compounds 7{x,x} and 8{x,y}. | |
 |
| Scheme 2 Synthesis of the amines 12{10} and 12{11}. | |
Once the symmetrical compounds 7{x,x} and the non-symmetrical compounds 8{x,y} were synthesized, they were tested together with the amide intermediates 13{x,x} and 17{x,y} for anti-HIV activity (EC50) and cytotoxicity (CC50) (Table 1).
Table 1 EC50 and CC50 of amides 13{x,x} and 17{x,y}, and tetramines 4{x,y}, 7{x,x} and 8{x,y}
Compound |

|

|
EC50 (μg mL−1) |
CC50 (μg mL−1) |
EC50 (μM) |
CC50 (μM) |
SI |
Compounds previously described by our group.11 EC50: effective concentration 50 or concentration needed to inhibit by 50% HIV-induced cell death evaluated with the MTT method in MT-4 cells. CC50: cytotoxic concentration 50 or concentration needed to induce 50% death of non-infected MT-4 cells with the MTT method. Reference compound: AMD3100: EC50 = 0.004 μM, CC50 > 10 μM. |
13{1,1} |
12{1} |
12{1} |
> 64.6 |
> 64.6 |
>155.82 |
> 155.82 |
— |
13{2,2} |
12{2} |
12{2} |
4.2 |
>125 |
10.87 |
>323.39 |
>29.8 |
13{3,3} |
12{3} |
12{3} |
68 |
>125 |
144.47 |
>265.57 |
>1.8 |
13{4,4} |
12{4} |
12{4} |
>125 |
>125 |
>279.91 |
>279.91 |
— |
13{5,5} |
12{5} |
12{5} |
>125 |
>125 |
>282.39 |
>282.39 |
— |
13{6,6} |
12{6} |
12{6} |
>125 |
>125 |
>306 |
>306 |
— |
13{7,7} |
12{7} |
12{7} |
2.0 |
>125 |
4.82 |
>301.51 |
>62.5 |
13{8,8} |
12{8} |
12{8} |
>125 |
>125 |
>298.66 |
>298.66 |
— |
13{9,9} |
12{9} |
12{9} |
>125 |
>125 |
>264.46 |
>264.46 |
— |
7{1,1} |
12{1} |
12{1} |
0.068 |
37 |
0.18 |
95.70 |
544 |
7{2,2} |
12{2} |
12{2} |
0.51 |
70 |
1.42 |
195.22 |
137 |
7{3,3} |
12{3} |
12{3} |
0.032 |
52 |
0.072 |
117.45 |
1625 |
7{4,4} |
12{4} |
12{4} |
20.73 |
101 |
49.52 |
241.27 |
5 |
7{5,5} |
12{5} |
12{5} |
0.042 |
47 |
0.101 |
113.34 |
1119 |
7{6,6} |
12{6} |
12{6} |
>125 |
>125 |
>328.49 |
>328.49 |
— |
7{7,7} |
12{7} |
12{7} |
0.1 |
5.0 |
0.26 |
129.33 |
500 |
7{8,8} |
12{8} |
12{8} |
>125 |
>125 |
>320.1 |
>320.1 |
— |
7{9,9} |
12{9} |
12{9} |
>36.03 |
36 |
>81.02 |
81.02 |
— |
4{1,1}a |
12{1} |
12{1} |
0.9 |
32.4 |
2.51 |
90.4 |
36 |
4{2,2}a |
12{2} |
12{2} |
10.2 |
>25 |
30.9 |
>75.6 |
>2.5 |
4{3,3}a |
12{3} |
12{3} |
0.050 |
>25 |
0.12 |
>60.3 |
>500 |
4{4,4}a |
12{4} |
12{4} |
>125 |
>125 |
>320.1 |
>320.1 |
— |
4{5,5}a |
12{5} |
12{5} |
0.14 |
>25 |
0.362 |
>64.65 |
>179 |
4{6,6}a |
12{6} |
12{6} |
>59.5 |
59.5 |
>168.8 |
168.8 |
— |
4{7,7}a |
12{7} |
12{7} |
>11.7 |
11.7 |
>32.6 |
32.6 |
— |
4{8,8}a |
12{8} |
12{8} |
>85.7 |
85.7 |
>236.4 |
236.4 |
— |
4{9,9}a |
12{9} |
12{9} |
9.5 |
>125 |
22.8 |
>300 |
>13 |
4{10,10}a |
12{10} |
12{10} |
0.336 |
>25 |
0.76 |
>56.47 |
>74 |
4{11,11}a |
12{11} |
12{11} |
0.298 |
>25 |
0.72 |
>60.29 |
>84 |
4{3,4}a |
12{3} |
12{4} |
0.5 |
>25 |
1.242 |
>62.1 |
>50 |
4{1,4}a |
12{1} |
12{4} |
1.6 |
>25 |
4.271 |
>66.7 |
>16 |
17{3,3} |
12{3} |
12{3} |
0.448 |
>25 |
1.012 |
>56.47 |
>56 |
17{4,4} |
12{4} |
12{4} |
>25 |
>25 |
>59.73 |
>59.73 |
— |
17{4,1} |
12{4} |
12{1} |
>25 |
>25 |
>62.10 |
>62.10 |
— |
17{4,3} |
12{4} |
12{3} |
2.894 |
>25 |
6.720 |
>58.05 |
>9 |
17{5,5} |
12{5} |
12{5} |
1.053 |
>25 |
2.539 |
>60.29 |
>24 |
17{5,3} |
12{5} |
12{3} |
0.596 |
>25 |
1.390 |
>58.32 |
>42 |
8{3,3} |
12{3} |
12{3} |
0.049 |
>25 |
0.114 |
>58.32 |
>510 |
8{4,4} |
12{4} |
12{4} |
>25 |
>25 |
61.79 |
>61.79 |
— |
8{4,1} |
12{4} |
12{1} |
3.779 |
>25 |
9.725 |
>64.34 |
>7 |
8{4,3} |
12{4} |
12{3} |
0.706 |
>25 |
1.695 |
>60.00 |
>35 |
8{5,5} |
12{5} |
12{5} |
0.130 |
>25 |
0.325 |
>62.40 |
>192 |
8{5,3} |
12{5} |
12{3} |
0.036 |
>25 |
0.087 |
>60.29 |
>694 |
From the values obtained some initial conclusions can be drawn: the presence of amide groups dramatically reduces the activity as it can be seen by comparing the EC50 of compounds 13{x,x} and 17{x,y} with those of compounds 7{x,x} and 8{x,y}, respectively. Moreover, monoamides 17{x,y} showed better activity than diamides 13{x,x}. This fact is easily observable by the direct comparison of the EC50 of compounds containing the same amine building blocks: 17{3,3} (EC50 = 1.012 μM) and 17{5,5} (EC50 = 2.539 μM) against 13{3,3} (EC50 = 144.47 μM) and 13{5,5} (EC50 > 282.39 μM), respectively.
On the other hand, the nine new tetramines 7{x,x} and six tetramines 8{x,y} covered a huge range of biological activities (0.072 μM > EC50 > 328 μM). Seven of these compounds presented EC50 ≤ 1 μM, the most potent being 7{3,3} (EC50 = 0.072 μM).
Among the different amines 12{x} used, 12{3}, 12{5} and 12{1} gave the most active compounds in the three families of compounds 4{x,y}, 7{x,x} and 8{x,y} when the same amine is present on both ends of the compound. These three amines have in common a propylene spacer (–(CH2)3–) connecting the heterocyclic ring and the nitrogen atom closer to the phenyl ring and contain only one nitrogen atom in the heterocyclic ring.
The comparison of the EC50 of compounds 7{x,x} with those of compounds 4{x,y} prepared using the same amine 12{x} clearly showed that higher activities were obtained for compounds 7{x,x} in eight out of nine cases. With the sole exception of compounds 7{9,9} and 4{9,9} prepared with amine 12{9}, better activity values and less toxicity were showed in cell tests when the central core is a 1,4-phenylenbis-(ethylene) moiety (compounds 7{x,x}) instead of a 1,4-phenylenebis-(methylene) one (compounds 4{x,y}).
This trend is also observed when the EC50 of compounds 8{x,y} are considered. These compounds present a central core with a methylene subunit on one side of the central phenyl ring and an ethylene subunit on the other side. When we compare the EC50 values of 7{3,3} (0.072 μM), 8{3,3} (0.114 μM) and 4{3,3} (0.121 μM) or those of 7{5,5} (0.101 μM), 8{5,5} (0.325 μM) and 4{5,5} (0.362 μM), we can observe that the EC50 exactly follow the order 7{x,x} < 8{x,x} < 4{x,x}. This observation let us conclude that for the kind of tetramines studied by our group as potential anti-HIV compounds, the presence of two methylene units on each side of the central phenyl ring increases the biological activity contrary to what has been observed in the case of AMD3100.
In the case of the non-symmetrical structures 4{x,y} and 8{x,y}, prepared using different amines 12{x} on each end of the molecule and in contrast to the symmetrical structures, at least in two cases compounds 4 (4{3,4}, EC50 = 1.242 μM; 4{1,4}, EC50 = 4.271 μM) are more active than the corresponding compounds 8 (8{4,3}, EC50 = 1.695 μM; 8{4,1}, EC50 = 9.725 μM). Among these compounds, 8{5,3} showed better anti-HIV activity (EC50 = 0.087 μM).
Another interesting comparison arises from the m + n value in symmetrical structures 4{x,x} and 7{x,x}. Thus, in Table 2 are included those structures in which m + n = 5 and the EC50 values clearly show that compounds 7{x,x} with m = 2 (EC50 of 7{5,5} and 7{3,3} are 0.101 and 0.072 μM respectively) are nearly 10-fold more active than compounds 4{x,x} with m = 1 (EC50 of 4{11,11} and 4{10,10} are 0.72 and 0.76 μM respectively). On the other hand, the comparison of the EC50 values in symmetrical structures 4{x,x} and 7{x,x} when m + n = 4 (Table 3) helps to conclude that in this case the value of m is not so important in molecules of this length, as compounds with the same terminal cyclic amine have nearly the same EC50.
Table 2 EC50 and CC50 of compounds 4{x,x} and 7{x,x} in which m + n = 5
Structure |
m
|
n
|
EC50 (μM) |
CC50 (μM) |
|
4{11,11} |
1 |
4 |
0.72 |
>60.29 |
7{5,5} |
2 |
3 |
0.101 |
113.34 |
|
4{10,10} |
1 |
4 |
0.76 |
>56.47 |
7{3,3} |
2 |
3 |
0.072 |
117.45 |
Table 3 EC50 and CC50 of compounds 4{x,x} and 7{x,x} in which m + n = 4
Structure |
m
|
n
|
EC50 (μM) |
CC50 (μM) |
|
4{1,1} |
1 |
3 |
2.51 |
90.4 |
7{2,2} |
2 |
2 |
1.42 |
195.22 |
|
4{5,5} |
1 |
3 |
0.362 |
>64.65 |
7{7,7} |
2 |
2 |
0.26 |
129.33 |
|
4{4,4} |
1 |
3 |
>320.1 |
>320.1 |
7{8,8} |
2 |
2 |
>320.1 |
>320.1 |
Table 3 also shows that the nature of the terminal heterocyclic ring plays an important role in the biological activity. Thus, symmetrical compounds 4{x,x} and 7{x,x} prepared with an amine 12{x} bearing a pyrrolidine (12{1} or 12{2}) or a piperidine (12{5} or 12{7}) present better anti-HIV activities (EC50 = 2.51, 1.42, 0.362, and 0.26 μM, respectively) while those prepared with an amine 12{x} bearing a morpholine (12{4}, 12{8}) gave EC50 > 320.1 μM.
Finally, in order to globally evaluate the results obtained, the EC50 and CC50 values of AMD3100 were determined (EC50 = 0.004 μM; CC50 > 10 μM) by following the same methodology as for our compounds. As it can be seen, compounds 7{3,3} (EC50 = 0.072 μM) and 8{5,3} (EC50 = 0.087 μM) present nearly the same level of activity as the reference and also show a good therapeutic index (SI = 1625 and >694, respectively).
In order to understand the results obtained, a computational study was carried out. This study consisted of the analysis of the interactions of CXCR4 with three different molecules: 4{3,3}, 7{3,3} and 8{3,3} (Fig. 5). In our previous work,19 we evaluated the docking-based virtual screening performance of CXCR4 crystal structures. We showed how PDB 3OE6 achieved the highest virtual screening performance using the docking function Ligandfit20 and the scoring function DockScore.21
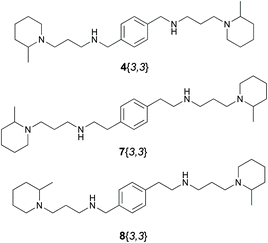 |
| Fig. 5 Structures of compounds 4{3,3}, 7{3,3} and 8{3,3}. | |
In the present work, structures 4{3,3}, 7{3,3} and 8{3,3} were prepared using the protocol LigPrep22 from Schrödinger. The force field OPLS 2005 was applied to generate possible tautomers, stereoisomers and ionization states at pH 7 ± 2 with an ionizer. Protein–ligand interactions established for the best poses obtained for each structure are shown in Fig. 6. All three compounds 4{3,3}, 7{3,3} and 8{3,3} bind CXCR4 in the same region of the extracellular pocket of CXCR4 because, in general, they have similar interactions with the same residues of CXCR4. Nevertheless, the binding modes and some of the interactions are different depending on each compound. In the best binding pose of 4{3,3} three side chain hydrogen bond donors were found between the two hydrogens of the protonated secondary amine and Asp187 and between the protonated pipecoline amine on the other side of the central aromatic ring and Asp97 oxygen, two ionic interactions were also found between the same protonated amines and Asp187 and Asp97 oxygens as before.
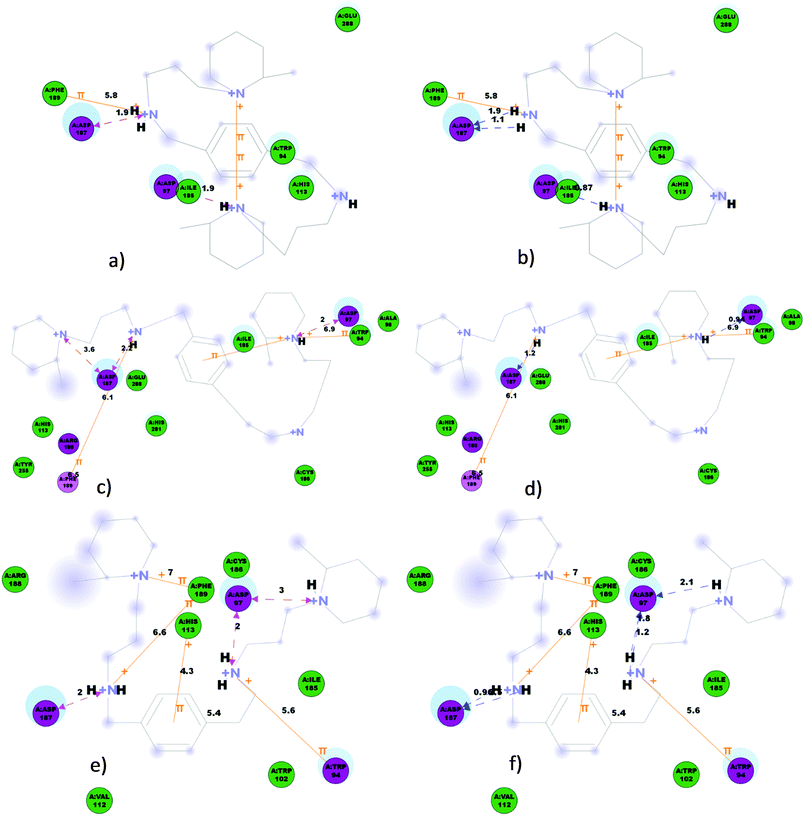 |
| Fig. 6 Docking binding modes of 4{3,3}, 7{3,3} and 8{3,3}. The diagrams show, separately and for a question of clarity, charge interactions (left diagrams) and hydrogen bond interactions (right diagrams) as well as the corresponding distances established between 4{3}, 7{3} and 8{3} and CXCR4. (a) and (b) correspond to the CXCR4-4{3,3} interactions, (c) and (d) to the CXCR4-7{3,3} interactions and (e) and (f) to the CXCR4-8{3,3} interactions found for the best binding poses obtained. The diagrams depict hydrogen bonds (blue arrows), charge interactions (pink arrows), π interactions (orange lines), ionic interactions (magenta circles), solvent (blue halo) and van der Waals interactions (green circles). | |
Three π interactions were also found, one between the same protonated secondary amine and Phe189 and two intramolecular π interactions between both protonated pipecoline amines and the central aromatic ring. Also, polar interactions were found between 4{3,3} and Asp97, Arg183, Asp187, Arg188 and His281 and van der Waals interactions with residues Leu41, Tyr45, Trp94, Ala98, Trp102, His113, Tyr116, Ile185, Cys186, Phe189, Tyr190, Tyr255 and Glu288 (Fig. 6).
The best binding pose of 7{3,3} shows two possible side chain hydrogen bond donors between the protonated secondary amine and Asp187 oxygen and between the protonated pipecoline amine on the other side of the central aromatic ring and Asp97 oxygen. Furthermore, three ionic interactions were also found between Asp187 oxygen and the protonated pipecoline and secondary amine, both on the same side of the structure, and between Asp97 oxygen and the protonated pipecoline on the other side of the central aromatic ring. Three possible π interactions were found, as above for 4{3,3}, one between the protonated secondary amine and Phe189 and two between the protonated pipecoline amine on the other side of the central aromatic ring and Trp94. Polar interactions were also found between 7{3,3} and Asp97, Asp187, Arg188 and Phe189 and van der Waals interactions with residues Asn37, Leu41, Tyr45, Trp94, Ala98, Trp102, His113, Tyr116, Arg183, Ile185, Cys186, Tyr190, Tyr255, His281, Ser285 and Glu288 (Fig. 6).
The best binding conformation of 8{3,3} shows five possible side chain hydrogen bond donors, two between the protonated secondary amine, which is linked to the central aromatic ring by a methylene, and Asp187, and three possible ones between Asp97 and the protonated pipecoline amine and the protonated secondary amine, both in the same amino building block which is linked to the central aromatic ring by an ethylene group. Three possible ionic interactions were also found between Asp187 and the protonated secondary amine linked to the central aromatic ring with a methylene, and between Asp97 and the protonated pipecoline and/or the secondary amine situated in the same amino building block linked with the central aromatic ring by an ethylene. Four possible π interactions were found, two between both the protonated secondary amine and/or with the pipecoline situated in the same building block, which is linked to the central aromatic ring by a methylene, and Phe189, another between the protonated secondary amine linked to the central aromatic ring by an ethylene and Trp94 and between the central aromatic ring and His113. Polar interactions were found between 8{3,3}and Trp94, Asp97 and Asp 187 while van der Waals interactions were established with residues Ala98, Trp102, Val112, His113, Arg183, Ile185, Cys186, Arg188 and Phe189 (Fig. 6).
Protein–ligand interactions can be represented from the protein point of view and also from the ligand point of view. Fig. 7 shows the stronger interactions and the corresponding distances established between structures 4{3,3}, 7{3,3} and 8{3,3} with different residues of CXCR4. Structure 4{3,3} shows less number of interactions than structures 7{3,3} and 8{3,3} and the interactions established are located at one end of the molecule. This observation could explain why this structure showed the highest EC50 of the three analogues. Despite this 8{3,3} shows more interactions than the other analogues, chiefly the π ones, and it showed higher EC50 than 7{3,3}. This result could be explained by the fact that 7{3,3} is the only compound that has shown charge interactions at each end of the molecule, at both nitrogens of the pipecolines. Charge interactions are considered the most determinant interactions established by CXCR423 and by HIV when they enter a host cell.24 These charge interactions could block the overall structure of 7{3,3} in the binding site, reducing global movements of the structure and therefore stabilizing interactions with CXCR4 inhibiting more effectively the HIV activity.
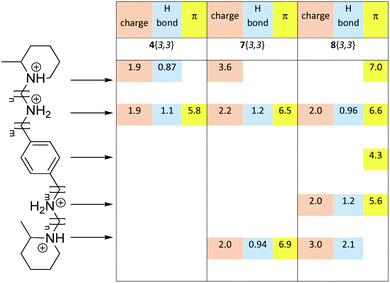 |
| Fig. 7 Representation of the main protein–ligand interactions (charge interactions in pink colour, hydrogen bonding in blue, and π interactions in yellow) and the interaction distances established by structures 4{3,3}, 7{3,3} and 8{3,3}. | |
In addition, the representation in Table 4 of the protein–ligand interactions, considering the protein point of view, shows that 8{3} was the ligand which presented fewer interactions (13) with CXCR4 while 7{3,3} was the one which presented more (20). 4{3,3} shows 18 interactions, all of them with the same amino acids as 7{3,3}. These different interactions could be explained by the study of the binding conformations of 4{3,3}, 7{3} and 8{3,3} which are superposed in Fig. 8. It seems as if all three compounds interacted with CXCR4 in the same pocket region, but if we compare the docked poses of each compound, it is noticeable that compounds 4{3,3} and 7{3,3} present a very similar binding mode (Fig. 8a and b), whilst compound 8{3,3} shows a different one (Fig. 8c), establishing accordingly different interactions with CXCR4.
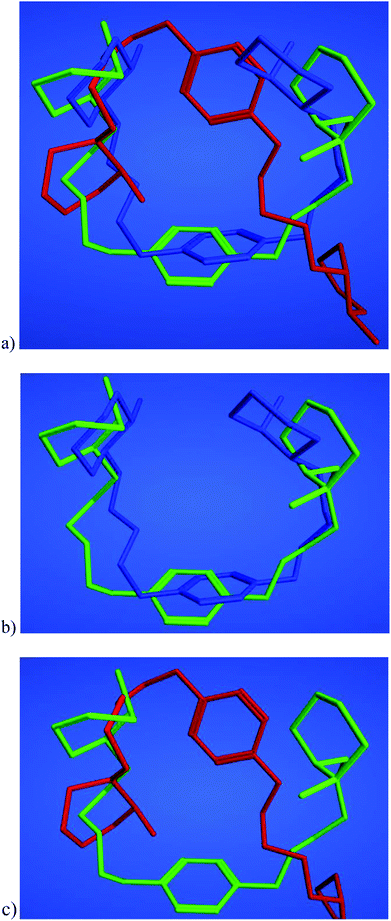 |
| Fig. 8 Superposition of docked structures of 4{3,3} (blue), 7{3,3} (green) and 8{3,3} (red). | |
Table 4 Amino acids involved in the interaction between CXCR4 and 4{3,3}, 7{3,3} and 8{3,3}, respectively
Conclusions
The present paper has shown that, contrary to AMD3100 and other cyclams, the paradigm by which it is necessary to use the p-phenylene moiety as the central core in order to achieve a high HIV-1 antiviral activity is not necessarily valid for structures less hindered than AMD3100.
Starting from structures 4, previously described by our group as potent CXCR4 coreceptor inhibitors, we carried out a positional scanning of the nitrogen atoms present on each side of the central phenyl ring in order to explore the impact on the biological activity of the distance between the phenyl ring and the first nitrogen atom of the side chains. Consequently, two families of compounds were designed, synthesized and biologically evaluated: the symmetrical compounds 7{x,x} (in which m = 2 on both sides) and the non-symmetrical compounds 8{x,y} (in which m = 1, on one side, and m = 2, on the other side) (Fig. 3). The corresponding synthetic itineraries (Scheme 1) use 1,4-benzenediacetic acid (9) and 2-(4-(bromomethyl)phenyl)acetic (14) acid, respectively, and a library of amines of the general structure 12{x} as starting materials.
The results obtained indicate that 12{3}, 12{5} and 12{1} gave the most active compounds in the three families of compounds 4{x,y}, 7{x,x} and 8{x,y} when the same amine is present on both ends of the compound.
Furthermore, the EC50 exactly follows the order 7{x,x} < 8{x,x} < 4{x,x}. This observation let us conclude that for the kind of tetramines studied by our group as potential anti-HIV compounds, the presence of two methylene units on each side of the central phenyl ring increases the biological activity contrary to what has been observed in the case of AMD3100.
In order to understand the results obtained, a computational study was carried out in which the interactions of CXCR4 with 4{3,3}, 7{3,3} and 8{3,3} were analyzed. Such a study revealed that although the three compounds could interact with CXCR4 in the same pocket region, the docked poses of compounds 4{3,3} and 7{3,3} show a very similar binding mode (Fig. 8a and b) whilst compound 8{3,3} shows a different one (Fig. 8c), establishing accordingly different interactions with CXCR4.
Experimental
General
All solvents and chemicals were reagent grade. Unless otherwise mentioned, all solvents and chemicals were purchased from commercial vendors (Fluka, Aldrich, ABCR and ACROS Organics) and used without purification. 1H and 13C NMR spectra were recorded on a Varian 400-MR spectrometer (1H-NMR at 400 MHz and 13C-NMR at 100.6 MHz). Chemical shifts were reported in parts per million (δ) and are referenced to tetramethylsilane (TMS) in 1H-NMR spectra and to the residual signal of the solvents CDCl3 (77.0) and methanol-d4 (49.0), in 13C-NMR spectra. Coupling constants are reported in hertz (Hz). Standard and peak multiplicities are designed as follows: s, singlet; d, doublet; dd, doublet of doublets; dt, doublet of triplets t, triplet; q, quadruplet; quint, quintuplet; br, broad signal. IR spectra were recorded on a Nicolet Magna 560 FTIR spectrophotometer and a Thermo Scientific Nicolet iS10 FTIR spectrophotometer with Smart iTr. Wavenumbers (ν) are expressed in cm−1. MS data (m/z (%), EI, 70 eV) were obtained by using an Agilent Technologies 5975 spectrometer and a Hewlett Packard HP5988A quadrupole mass spectrometer operating in electronic ionization (EI) mode at 70 eV and at 4 kV accelerating potential, on a VG AutoSpec (Micromass Instruments) Trisector EBE spectrometer operating in Fast Atom Bombardment (FAB) mode. HRMS data were obtained by using a VG AutoSpec (Micromass Instruments) Trisector EBE high resolution spectrometer (EI or FAB mode). Elemental microanalyses were obtained on a Carlo-Erba CHNS-O/EA 1180 and a EuroVector Instruments Euro EA elemental analyzer. The melting points were determined with a Büchi-Tottoli 530 capillary apparatus and are uncorrected. Automatic flash chromatography was performed in an Isco Combiflash medium pressure liquid chromatograph with RediSep® silica gel columns (35–70 μm) using a suitable mixture of solvents as the eluent. Microwave irradiation experiments were carried out in an InitiatorTM (Biotage) microwave apparatus, operating at a frequency of 2.45 GHz with a continuous irradiation power from 0 to 400 W. Reactions were carried out in 0.5, 2.5, 5, and 20 mL glass tubes, sealed with aluminum/Teflon crimp tops, which can be exposed to temperatures up to 250 °C and 20 bar internal pressure. Temperature was measured with an IR sensor on the outer surface of the process vial. After the irradiation period, the reaction vessel was cooled rapidly to 50 °C by air jet cooling.
Synthetic procedures
N,N′-Bis(3-(pyrrolidin-1-yl)propyl)-1,4-di(aminocarbonylmethyl)benzene (13{1,1}).
A mixture of 1,4-benzenediacetic acid (9) (2.0 g, 10.30 mmol), hexamethyldisilazane (HMDS) (2.86 mL, 20.60 mmol) and trimethylchlorosilane (12 drops) in dry 1,2-dichloroethane (60 mL) was refluxed overnight under N2. The solution was evaporated at reduced pressure. The residual material was bis(trimethylsilyl) 1,4-benzenediacetate (10). This product was dissolved in anhydrous CH2Cl2 (20 mL) and anhydrous DMF (4 drops) and oxalyl chloride (1.86 mL, 21.86 mmol) was added dropwise to the solution at 0 °C. The resulting solution was stirred for 1 h at 0 °C under N2 and for 1 h at room temperature before the solvent was removed at reduced pressure. The residual material was 1,4-benzenediacetyl dichloride (11). Anhydrous CH2Cl2 (28 mL) was added to the flask and cooled to 0 °C. 1-(3-Amino-propyl)pyrrolidine (12{1}) (2.68 mL, 20.60 mmol) and triethylamine (TEA) (3.20 mL, 22.96 mmol) were added. The solution was stirred overnight at room temperature under N2. A K2CO3 solution (25%) (40 mL) was added and the aqueous layer was separated and extracted with CH2Cl2 (3 × 40 mL). The combined organic extracts were washed with water (2 × 40 mL), brine (40 mL) and dried with MgSO4 and subsequently concentrated. Purification of the residue by flash chromatography (silica, 75
:
25
:
2, CH2Cl2/MeOH/NH3) provided 2.43 g (5.84 mmol, 57%) of N,N′-bis(3-pyrrolidin-1-yl)propyl)-1,4-di(aminocarbonylmethyl)benzene (13{1,1}) as a white solid, mp 160–161 °C. IR (KBr): ν (cm−1): 3264 (N–H), 3080 (Csp2–H), 2962, 2874, 2791 (Csp3–H), 1642 (C
O), 1562 (NH). 1H-NMR (400 MHz, CDCl3): δ (ppm): 7.23 (s, 4H, C1–H), 6.92 (br s, 2H, N–H), 3.49 (s, 4H, C3–H), 3.31 (q, 3J = 5.6 Hz, 4H, C5–H), 2.49 (t, 3J = 6.5 Hz, 4H, C7–H), 2.46–2.40 (m, 8H, C8–H), 1.74–1.68 (m, 8H, C9–H), 1.64 (quint, 3J = 6.5 Hz, 4H, C6–H). 13C-NMR (100.6 MHz, CDCl3): δ (ppm): 170.8 (C4), 134.1 (C2), 129.7 (C1), 54.9 (C7), 54.0 (C8), 43.6 (C3), 39.4 (C5), 27.1 (C6), 23.4 (C9). MS (70 eV, EI): m/z (%) = 414.2 (16) [M]+, 330.1 (32) [M − C5H10N]+, 274.0 (10) [M − C8H16N2]+, 260.0 (43) [M − C9H18N2]+, 97.9 (31) [C6H12N]+, 83.9 (100) [C5H10N]+, 69.9 (29) [C4H8N]+. HRMS (70 eV, EI): m/z calculated for C24H38N4O2: 414.2995, [M]+; Found: 414.2992.
N,N′-Bis(2-(pyrrolidin-1-yl)ethyl)-1,4-di(aminocarbonylmethyl)benzene (13{2,2}).
As stated above for 13{1,1}, but using 1,4-benzenediacetic acid (9) (2.0 g, 10.30 mmol), hexamethyldisilazane (HMDS) (2.86 mL, 20.60 mmol), trimethylchlorosilane (12 drops), DMF (4 drops), oxalyl chloride (1.86 mL, 21.86 mmol), 1-(2-aminoethyl)pyrrolidine (12{2}) (2.65 mL, 20.60 mmol) and triethylamine (TEA) (3.20 mL, 22.96 mmol). The final compound was purified by flash chromatography (silica, 75
:
25
:
2, CH2Cl2/MeOH/NH3) to afford 3.06 g (7.82 mmol, 76%) of N,N′-bis(2-(pyrrolidin-1-yl)ethyl)-1,4-di(aminocarbonylmethyl)benzene (13{2,2}) as a white solid, mp 194–196 °C. IR (KBr): ν (cm−1): 3288 (N–H), 3083 (Csp2–H), 2964, 2939, 2872, 2800 (Csp3–H), 1648 (C
O), 1549 (NH). 1H-NMR (400 MHz, CDCl3): δ (ppm): 7.24 (s, 4H, C1–H), 6.16 (br s, 2H, N–H), 3.53 (s, 4H, C3–H), 3.32 (q, 3J = 6.1 Hz, 4H, C5–H), 2.54 (t, 3J = 6.2 Hz, 4H, C6–H), 2.48–2.40 (m, 8H, C7–H), 1.77–1.67 (m, 8H, C8–H). 13C-NMR (100.6 MHz, CDCl3): δ (ppm): 170.8 (C4), 134.0 (C2), 129.6 (C1), 54.3 (C6), 53.7 (C7), 43.3 (C3), 38.2 (C5), 23.4 (C8). MS (70 eV, EI): m/z (%) = 386.3 (2) [M]+, 246.2 (2) [M − C8H16N2]+, 84.1 (100) [C5H10N]+, 70.1 (2) [C4H8N]+. Anal. calculated for C22H34N4O2: C: 68.36%, H: 8.87%, N: 14.49%, O: 8.28%; Found: C: 68.11%, H: 8.75%, N: 14.69%.
N,N′-Bis(3-(2-pipecolin-1-yl)propyl)-1,4-di(aminocarbonylmethyl)benzene (13{3,3}).
As stated above for 13{1,1}, but using 1,4-benzenediacetic acid (9) (2.0 g, 10.30 mmol), hexamethyldisilazane (HMDS) (2.86 mL, 20.60 mmol), trimethylchlorosilane (12 drops), DMF (4 drops), oxalyl chloride (1.86 mL, 21.86 mmol), 1-(3-aminopropyl)-2-pipecoline (12{3}) (3.77 mL, 20.60 mmol) and triethylamine (TEA) (3.20 mL, 22.96 mmol). The final compound was purified by flash chromatography (silica, 75
:
25
:
2, CH2Cl2/MeOH/NH3) to afford 3.46 g (7.35 mmol, 71%) of N,N′-bis(3-(2-pipecolin-1-yl)propyl)-1,4-di(aminocarbonylmethyl)benzene (13{3,3}) as an off-white solid, mp 119–120 °C. IR (KBr): ν (cm−1): 3292 (N–H), 3074 (Csp2–H), 2926, 2858, 2787, 2729 (Csp3–H), 1648 (C
O), 1546 (NH). 1H-NMR (400 MHz, CDCl3): δ (ppm): 7.24 (s, 4H, C1–H), 7.09 (br s, 2H, N–H), 3.50 (s, 4H, C3–H), 3.41–3.31 (m, 2H, C5–H), 3.26–3.16 (m, 2H, C5–H), 2.87–2.79 (m, 2H, C8–H), 2.79–2.70 (m, 2H, C7–H), 2.29–2.19 (m, 4H, C7–H, C12–H), 2.07–1.97 (m, 2H, C8–H), 1.70–1.52 (m, 10H, C6–H, C9–H, C10–H, C11–H), 1.48–1.36 (m, 2H, C9–H), 1.35–1.16 (m, 4H, C10–H, C11–H), 1.00 (d, 3J = 6.3 Hz, 6H, C13–H). 13C-NMR (100.6 MHz, CDCl3): δ (ppm): 170.5 (C4), 134.1 (C2), 129.7 (C1), 56.3 (C12), 52.6 (C7), 51.7 (C8), 43.6 (C3), 39.7 (C5), 34.5 (C11), 26.0 (C9), 24.9 (C6), 23.5 (C10), 18.6 (C13). MS (70 eV, EI): m/z (%) = 470.4 (6) [M]+, 455.4 (20) [M − CH3]+, 358.3 (8) [M − C7H14N]+, 112.1 (100) [C7H14N]+. HRMS (70 eV, EI): m/z calculated for C28H46N4O2: 470.3621, [M]+; Found: 470.3615.
N,N′-Bis(3-(morpholin-4-yl)propyl)-1,4-di(aminocarbonylmethyl)benzene (13{4,4}).
As stated above for 13{1,1}, but using 1,4-benzenediacetic acid (9) (2.0 g, 10.30 mmol), hexamethyldisilazane (HMDS) (2.86 mL, 20.60 mmol), trimethylchlorosilane (12 drops), DMF (4 drops), oxalyl chloride (1.86 mL, 21.86 mmol), 3-morpholinopropylamine (12{4}) (3.01 mL, 20.60 mmol) and triethylamine (TEA) (3.20 mL, 22.96 mmol). The final compound was purified by flash chromatography (silica, 75
:
25
:
2, CH2Cl2/MeOH/NH3) to afford 3.65 g (8.18 mmol, 79%) of N,N′-bis(3-(morpholin-4-yl)propyl)-1,4-di(aminocarbonylmethyl)benzene (13{4,4}) as a yellow solid, mp 172–173 °C. IR (KBr): ν (cm−1): 3295 (N–H), 3080 (Csp2–H), 2930, 2867, 2804, 2757 (Csp3–H), 1643 (C
O), 1547 (NH), 1117, 864. 1H-NMR (400 MHz, CDCl3): δ (ppm): 7.24 (s, 4H, C1–H), 6.51 (br s, 2H, N–H), 3.62 (t, 3J = 4.4 Hz, 8H, C9–H), 3.51 (s, 4H, C3–H), 3.31 (q, 3J = 6.1 Hz, 4H, C5–H), 2.42–2.34 (m, 12H, C7–H, C8–H), 1.64 (quint, 3J = 6.1 Hz, 4H, C6–H). 13C-NMR (100.6 MHz, CDCl3): δ (ppm): 170.5 (C4), 134.1 (C2), 129.7 (C1), 66.9 (C9), 57.3 (C7), 53.6 (C8), 43.5 (C3), 39.1 (C5), 25.1 (C6). MS (70 eV, EI): m/z (%) = 446.3 (15) [M]+, 416.3 (12) [M − CH2O]+, 346.1 (4) [M − C5H10NO]+, 171.1 (10) [M − C8H15N2O2]+, 100.1 (100) [C5H10NO]+. Anal. calculated for C24H38N4O4: C: 64.55%, H: 8.58%, N: 12.55%, O: 14.33%; Found: C: 64.48%, H: 8.39%, N: 12.66%.
N,N′-Bis(3-(piperidin-1-yl)propyl)-1,4-di(aminocarbonylmethyl)benzene (13{5,5}).
As stated above for 13{1,1}, but using 1,4-benzenediacetic acid (9) (2.0 g, 10.30 mmol), hexamethyldisilazane (HMDS) (2.86 mL, 20.60 mmol), trimethylchlorosilane (12 drops), DMF (4 drops), oxalyl chloride (1.86 mL, 21.86 mmol), 1-(3-aminopropyl)piperidine (12{5}) (3.38 mL, 20.60 mmol) and triethylamine (TEA) (3.20 mL, 22.96 mmol). The final compound was purified by flash chromatography (silica, 75
:
25
:
2, CH2Cl2/MeOH/NH3) to afford 3.45 g (7.79 mmol, 76%) of N,N′-bis(3-(piperidin-1-yl)propyl)-1,4-di(aminocarbonylmethyl)benzene (13{5,5}) as a white solid, mp 151–153 °C. IR (KBr): ν (cm−1): 3289 (N–H), 3089 (Csp2–H), 2930, 2850, 2809, 2771 (Csp3–H), 1640 (C
O), 1559 (NH). 1H-NMR (400 MHz, CDCl3): δ (ppm): 7.24 (s, 4H, C1–H), 7.18 (br s, 2H, N–H), 3.49 (s, 4H, C3–H), 3.30 (q, 3J = 6.0 Hz, 4H, C5–H), 2.43–2.28 (m, 12H, C7–H, C8–H), 1.64 (quint, 3J = 6.4 Hz, 4H, C6–H), 1.54 (quint, 3J = 5.6 Hz, 8H, C9–H), 1.48–1.39 (m, 4H, C10–H). 13C-NMR (100.6 MHz, CDCl3): δ (ppm): 170.7 (C4), 134.1 (C2), 129.6 (C1), 57.8 (C7), 54.5 (C8), 43.5 (C3), 39.5 (C5), 25.9 (C9), 24.9 (C6), 24.2 (C10). MS (70 eV, EI): m/z (%) = 442.3 (9) [M]+, 344.2 (18) [M − C6H12N]+, 274.1 (12) [M − C10H20N2]+, 98.1 (100) [C6H12N]+, 84.0 (5) [C5H10N]+. Anal. calculated for C26H42N4O2: C: 70.55%, H: 9.56%, N: 12.66%, O: 7.23%; Found: C: 70.40%, H: 9.42%, N: 12.50%.
N,N′-Bis(3-(imidazol-1-yl)propyl)-1,4-di(aminocarbonylmethyl)benzene (13{6,6}).
As stated above for 13{1,1}, but using 1,4-benzenediacetic acid (9) (2.0 g, 10.30 mmol), hexamethyldisilazane (HMDS) (2.86 mL, 20.60 mmol), trimethylchlorosilane (12 drops), DMF (4 drops), oxalyl chloride (1.86 mL, 21.86 mmol), 1-(3-aminopropyl)imidazole (12{6}) (2.51 mL, 20.60 mmol) and triethylamine (TEA) (3.20 mL, 22.96 mmol). The final compound was purified by flash chromatography (silica, 80
:
20
:
1, CH2Cl2/MeOH/NH3) to afford 1.75 g (4.29 mmol, 42%) of N,N′-bis(3-(imidazol-1-yl)propyl)-1,4-di(aminocarbonylmethyl)benzene (13{6,6}) as a yellow crystalline solid, mp 132–133 °C. IR (KBr): ν (cm−1): 3292 (N–H), 3104 (Csp2–H), 2930 (Csp3–H), 1639 (C
O), 1560 (NH), 1511. 1H-NMR (400 MHz, methanol-d4): δ (ppm): 7.60 (s, 2H, C8–H), 7.26 (s, 4H, C1–H), 7.08 (s, 2H, C10–H), 6.95 (s, 2H, C9–H), 3.98 (t, 3J = 7.0 Hz, 4H, C7–H), 3.47 (s, 4H, C3–H), 3.15 (t, 3J = 6.7 Hz, 4H, C5–H), 1.94 (quint, 3J = 6.8 Hz, 4H, C6–H). 13C-NMR (100.6 MHz, methanol-d4): δ (ppm): 174.2 (C4), 138.4 (C8), 135.7 (C2), 130.4 (C1), 129.0 (C9), 120.6 (C10), 45.5 (C7), 43.5 (C3), 37.6 (C5), 31.9 (C6). MS (FAB): m/z (%) = 409.1 (100) [M + H]+, 341.0 (3) [M − C3H3N2]+. HRMS (FAB): m/z calculated for C22H29N6O2: 409.2352, [M + H]+; Found: 409.2356.
N,N′-Bis(2-(piperidin-1-yl)ethyl)-1,4-di(aminocarbonylmethyl)benzene (13{7,7}).
As stated above for 13{1,1}, but was carried out by using 1,4-benzenediacetic acid (9) (2.0 g, 10.30 mmol), hexamethyldisilazane (HMDS) (2.86 mL, 20.60 mmol), trimethylchlorosilane (12 drops), DMF (4 drops), oxalyl chloride (1.86 mL, 21.86 mmol), 1-(2-aminoethyl)piperidine (12{7}) (2.99 mL, 20.60 mmol) and triethylamine (TEA) (3.20 mL, 22.96 mmol). Upon removal of the solvent, 4.27 g (10.3 mmol, 100%) of N,N′-bis(2-(piperidin-1-yl)ethyl)-1,4-di(aminocarbonylmethyl)benzene (13{7,7}) were obtained without further purification as a pale orange solid, mp 174–176 °C. IR (KBr): ν (cm−1): 3298 (N–H), 3091 (Csp2–H), 2924, 2849, 2779, 2751 (Csp3–H), 1642 (C
O), 1556 (NH). 1H-NMR (400 MHz, CDCl3): δ (ppm): 7.26 (s, 4H, C1–H), 6.16 (br s, 2H, N–H), 3.55 (s, 4H, C3–H), 3.27 (q, 3J = 6.0 Hz, 4H, C5–H), 2.34 (t, 3J = 6.1 Hz, 4H, C6–H), 2.31–2.21 (m, 8H, C7–H), 1.47–1.33 (m, 12H, C8–H, C9–H). 13C-NMR (100.6 MHz, CDCl3): δ (ppm): 170.7 (C4), 134.1 (C2), 129.8 (C1), 56.7 (C6), 54.0 (C7), 43.3 (C3), 36.1 (C5), 25.9 (C8), 24.3 (C9). MS (70 eV, EI): m/z (%) = 414.3 (4) [M]+, 98.1 (100) [C6H12N]+. HRMS (70 eV, EI): m/z calculated for C24H38N4O2: 414.2995, [M]+; Found: 414.2987.
N,N′-Bis(2-(morpholin-4-yl)ethyl)-1,4-di(aminocarbonylmethyl)benzene (13{8,8}).
As stated above for 13{1,1}, but using 1,4-benzenediacetic acid (9) (2.0 g, 10.30 mmol), hexamethyldisilazane (HMDS) (2.86 mL, 20.60 mmol), trimethylchlorosilane (12 drops), DMF (4 drops), oxalyl chloride (1.86 mL, 21.86 mmol), 2-morpholinoethylamine (12{8}) (2.73 mL, 20.60 mmol) and triethylamine (TEA) (3.20 mL, 22.96 mmol). Upon removal of the solvent, 4.33 g (10.3 mmol, 100%) of N,N′-bis(2-(morpholin-4-yl)ethyl)-1,4-di(aminocarbonylmethyl)benzene (13{8,8}) were obtained without further purification as a white solid, mp 173–175 °C. IR (KBr): ν (cm−1): 3287 (N–H), 3061 (Csp2–H), 2967, 2932, 2864, 2787 (Csp3–H), 1645 (C
O), 1540 (NH), 1117, 868. 1H-NMR (400 MHz, CDCl3): δ (ppm): 7.27 (s, 4H, C1–H), 6.02 (br s, 2H, N–H), 3.58 (t, 3J = 4.6 Hz, 8H, C8–H), 3.56 (s, 4H, C3–H), 3.31 (q, 3J = 5.9 Hz, 4H, C5–H), 2.42 (t, 3J = 6.1 Hz, 4H, C6–H), 2.36 (t, 3J = 4.4 Hz, 8H, C7–H). 13C-NMR (100.6 MHz, CDCl3): δ (ppm): 170.6 (C4), 134.1 (C2), 129.8 (C1), 66.8 (C8), 56.5 (C6), 53.1 (C7), 43.3 (C3), 35.7 (C5). MS (70 eV, EI): m/z (%) = 418.3 (5) [M]+, 100.1 (100) [C5H10NO]+. Anal. calculated for C22H34N4O4: C: 63.13%, H: 8.19%, N: 13.39%, O: 15.29%; Found: C: 63.30%, H: 8.17%, N: 13.23%.
N,N′-Bis(3-(4-methylpiperazin-1-yl)propyl)-1,4-di(aminocarbonylmethyl)benzene (13{9,9}).
As stated above for 13{1,1}, but using 1,4-benzenediacetic acid (9) (2.0 g, 10.30 mmol), hexamethyldisilazane (HMDS) (2.86 mL, 20.60 mmol), trimethylchlorosilane (12 drops), DMF (4 drops), oxalyl chloride (1.86 mL, 21.86 mmol), 1-(3-aminopropyl)-4-methylpiperazine (12{9}) (3.58 mL, 20.60 mmol) and triethylamine (TEA) (3.20 mL, 22.96 mmol). The final compound was purified by flash chromatography (silica, 75
:
25
:
2, CH2Cl2/MeOH/NH3) to afford 2.63 g (5.56 mmol, 54%) of N,N′-bis(3-(4-methylpiperazin-1-yl)propyl)-1,4-di(aminocarbonylmethyl)-benzene (13{9,9}) as a yellow solid, mp 161–163 °C. IR (KBr): ν (cm−1): 3240 (N–H), 3066 (Csp2–H), 2943, 2876, 2830, 2811 (Csp3–H), 1644 (C
O), 1576 (NH). 1H-NMR (400 MHz, methanol-d4): δ (ppm): 7.25 (s, 4H, C1–H), 3.47 (s, 4H, C3–H), 3.55 (s, 4H, C3–H), 3.22 (t, 3J = 6.8 Hz, 4H, C5–H), 2.81 (s, 8H, C9–H), 2.63 (s, 8H, C8–H), 2.52 (s, 6H, C10–H), 2.46 (t, 3J = 7.2 Hz 4H, C7–H), 1.70 (quint, 3J = 6.8 Hz, 4H, C6–H). 13C-NMR (100.6 MHz, methanol-d4): δ (ppm): 174.1 (C4), 135.8 (C2), 130.3 (C1), 56.2 (C7), 55.0 (C9), 52.5 (C8), 44.9 (C10), 43.5 (C3), 38.4 (C5), 27.1 (C6). MS (FAB): m/z (%) = 473.1 (100) [M + H]+, 373.0 (4) [M − C5H11N2]+. HRMS (FAB): m/z calculated for C26H44N6O2: 473.3604, [M + H]+; Found: 473.3604.
N,N′-Bis(3-(pyrrolidin-1-yl)propyl)-1,4-bis(2-aminoeth-1-yl)-benzene (7{1,1}).
The borane dimethylsulfide complex (1.19 mL, 11.89 mmol) was added at 0 °C to a solution of N,N′-bis(3-(pyrrolidin-1-yl)propyl)-1,4-di(aminocarbonylmethyl)benzene (13{1,1}) (414.58 mg, 1.0 mmol) in anhydrous THF (27 mL). The solution was refluxed for 6 h under N2, cooled down to room temperature, diluted with 7.5 mL of 1.25 M HCl/MeOH solution and refluxed for 1 h. The solvent was removed in vacuo and the crude material was diluted with 1 M NaOH solution (pH 14). The aqueous solution was extracted twice with CH2Cl2, dried with MgSO4 and concentrated. Purification of the residue by flash chromatography (basic alumina, CH2Cl2/MeOH; 100
:
0 to 90
:
10 in 18 min) afforded 296 mg (0.77 mmol, 77%) of N,N′-bis(3-(pyrrolidin-1-yl)propyl)-1,4-bis(2-aminoeth-1-yl)benzene (7{1,1}) as a white solid, mp 60–62 °C. IR (KBr): ν (cm−1): 3263 (N–H), 2948, 2801 (Csp3–H), 1697 (NH), 1458 (Csp3–H), 887 (NH). 1H-NMR (400 MHz, CDCl3): δ (ppm): 7.13 (s, 4H, C1–H), 2.90–2.82 (m, 4H, C4–H), 2.81–2.74 (m, 4H, C3–H), 2.68 (t, 3J = 7.1 Hz, 4H, C5–H), 2.50–2.42 (m, 12H, C7–H, C8–H), 1.91 (br s, 2H, N–H), 1.78–1.72 (m, 8H, C9–H), 1.69 (quint, 3J = 7.1 Hz, 4H, C6–H). 13C-NMR (100.6 MHz, CDCl3): δ (ppm): 137.9 (C2), 128.9 (C1), 54.9 (C7), 54.4 (C8), 51.3 (C4), 48.6 (C5), 36.0 (C3), 29.4 (C6), 23.5 (C9). MS (70 eV, EI): m/z (%) = 386.4 (2) [M]+, 302.2 (4) [M − C5H10N]+, 274.2 (8) [M − C7H14N]+, 141.1 (47) [C8H17N2]+, 112.1 (9) [C7H14N]+, 98.1 (11) [C6H12N]+, 84.1 (100) [C5H10N]+, 70.1 (8) [C4H8N]+. HRMS (70 eV, EI): m/z calculated for C24H42N4: 386.3409, [M]+; Found: 386.3405.
N,N′-Bis(2-(pyrrolidin-1-yl)ethyl)-1,4-bis(2-aminoeth-1-yl)-benzene (7{2,2}).
As stated above for 7{1,1}, but using the borane dimethylsulfide complex (0.58 mL, 6.15 mmol), N,N′-bis(2-(pyrrolidin-1-yl)ethyl)-1,4-di(aminocarbonylmethyl)benzene (13{2,2}) (466.2 mg, 1.21 mmol), anhydrous THF (4 mL), 1.25 M HCl/MeOH (9 mL). The final compound was purified by flash chromatography (basic alumina, CH2Cl2/MeOH; 100
:
0 to 90
:
10 in 18 min) to afford 267.0 mg (74.5 mmol, 75%) of N,N′-bis(2-(pyrrolidin-1-yl)ethyl)-1,4-bis(2-aminoeth-1-yl)benzene (7{2,2}) as a yellow oil. IR (KBr): ν (cm−1): 3305 (N–H), 2929, 2875, 2796 (Csp3–H), 1673 (NH), 1458. 1H-NMR (400 MHz, CDCl3): δ (ppm): 7.13 (s, 4H, C1–H), 2.91–2.84 (m, 4H, C4–H), 2.81–2.73 (m, 8H, C3–H, C5–H), 2.59 (t, 3J = 6.5 Hz, 4H, C6–H), 2.52–2.45 (m, 8H, C7–H), 2.17 (br s, 2H, N–H), 1.78–1.71 (m, 8H, C8–H). 13C-NMR (100.6 MHz, CDCl3): δ (ppm): 137.7 (C2), 128.7 (C1), 55.9 (C6), 54.2 (C7), 51.4 (C4), 48.4 (C5), 35.9 (C3), 23.4 (C8). MS (FAB): m/z (%) = 359.3 (100) [M + H]+, 358.3 (14) [M]+, 288.3 (4) [M − C4H8N]+, 274.3 (12) [M − C5H10N]+, 231.1 (37) [M − C7H15N2]+, 127.1 (15) [C7H15N2]+. HRMS (FAB): m/z calculated for C22H39N4: 359.3175, [M + H]+; Found: 359.3159.
N,N′-Bis(3-(2-pipecolin-1-yl)propyl)-1,4-bis(2-aminoeth-1-yl)benzene (7{3,3}).
As stated above for 7{1,1}, but using the borane dimethylsulfide complex (1.19 mL, 11.89 mmol), N,N′-bis(3-(2-pipecolin-1-yl)propyl)-1,4-di(aminocarbonylmethyl)benzene (13{3,3}) (470.7 mg, 1.0 mmol), anhydrous THF (27 mL), 1.25 M HCl/MeOH (7.5 mL). The final compound was purified by flash chromatography (basic alumina, CH2Cl2/MeOH; 100
:
0 to 90
:
10 in 18 min) to afford 237.0 mg (0.53 mmol, 53%) of N,N′-bis(3-(2-pipecolin-1-yl)propyl)-1,4-bis(2-aminoeth-1-yl)benzene (7{3,3}) as an orange oil. IR (KBr): ν (cm−1): 3280 (N–H), 2930, 2854, 2794 (Csp3–H), 1655 (NH), 1449, 1372. 1H-NMR (400 MHz, CDCl3): δ (ppm): 7.13 (s, 4H, C1–H), 2.89–2.80 (m, 6H, C4–H, C8–H), 2.80–2.74 (m, 4H, C3–H), 2.73–2.65 (m, 2H, C7–H), 2.62 (t, 3J = 7.0 Hz, 4H, C5–H), 2.36–2.27 (m, 2H, C7–H), 2.26–2.18 (m, 2H, C12–H), 2.12–2.04 (m, 2H, C8–H), 1.92 (br s, 2H, N–H), 1.69–1.61 (m, 6H, C6–H, C10–H), 1.60–1.53 (m, 4H, C9–H, C11–H), 1.52–1.43 (m, 2H, C9–H), 1.31–1.21 (m, 4H, C10–H, C11–H), 1.02 (d, 3J = 6.2 Hz, 6H, C13–H). 13C-NMR (100.6 MHz, CDCl3): δ (ppm): 138.0 (C2), 129.0 (C1), 56.1 (C12), 52.4 (C7), 52.3 (C8), 51.4 (C4), 49.0 (C5), 36.2 (C3), 34.8 (C11), 26.3 (C9), 26.0 (C6), 24.2 (C10), 19.3 (C13). MS (70 eV, EI): m/z (%) = 442.4 (3) [M]+, 330.3 (9) [M − C7H14N]+, 302.3 (18) [M − C9H18N]+, 169.2 (97) [C10H21N2]+, 140.1 (7) [C9H18N]+, 126.1 (13) [C8H16N]+, 112.1 (100) [C7H14N]+, 98.1 (28) [C6H12N]+. HRMS (70 eV, EI): m/z calculated for C28H50N4: 442.4035, [M]+; Found: 442.4027.
N,N′-Bis(3-(morpholin-4-yl)propyl)-1,4-bis(2-aminoeth-1-yl)benzene (7{4,4}).
As stated above for 7{1,1}, but using the borane dimethylsulfide complex (0.48 mL, 5.1 mmol), N,N′-bis(3-(morpholin-4-yl)propyl)-1,4-di(aminocarbonylmethyl)benzene (13{4,4}) (446.6 mg, 1.0 mmol), anhydrous THF (4 mL), 1.25 M HCl/MeOH (7.5 mL). The final compound was purified by flash chromatography (basic alumina, CH2Cl2/MeOH; 100
:
0 to 90
:
10 in 18 min) to afford 339.1 mg (0.81 mmol, 81%) of N,N′-bis(3-(morpholin-4-yl)propyl)-1,4-bis(2-aminoeth-1-yl)benzene (7{4,4}) as a yellow oil. IR (KBr): ν (cm−1): 3292 (N–H), 2928, 2852, 2807 (Csp3–H), 1673 (NH), 1457, 1118, 863. 1H-NMR (400 MHz, CDCl3): δ (ppm): 7.15 (s, 4H, C1–H), 3.65 (t, 3J = 4.6 Hz, 8H, C9–H), 2.92–2.85 (m, 4H, C4–H), 2.83–2.77 (m, 4H, C3–H), 2.70 (t, 3J = 6.9 Hz, 4H, C5–H), 2.46–2.32 (m, 14H, C7–H, C8–H, N–H), 1.68 (quint, 3J = 7.0 Hz, 4H, C6–H). 13C-NMR (100.6 MHz, CDCl3): δ (ppm): 137.6 (C2), 128.8 (C1), 66.8 (C9), 57.3 (C7), 53.7 (C8), 51.0 (C4), 48.4 (C5), 35.6 (C3), 26.3 (C6). MS (70 eV, EI): m/z (%) = 418.3 (6) [M]+, 388.3 (5) [M − CH2O]+, 157.1 (21) [C8H17N2O]+, 128.1 (7) [C7H14NO]+, 114.1 (10) [C6H12NO]+, 100.1 (100) [C5H10NO]+. HRMS (70 eV, EI): m/z calculated for C24H42N4O2: 418.3308, [M]+; Found: 418.3311.
N,N′-Bis(3-(piperidin-1-yl)propyl)-1,4-bis(2-aminoeth-1-yl)benzene (7{5,5}).
As stated above for 7{1,1}, but using the borane dimethylsulfide complex (1.19 mL, 11.89 mmol), N,N′-bis(3-(piperidin-1-yl)propyl)-1,4-di(aminocarbonylmethyl)benzene (13{5,5}) (442.6 mg, 1.0 mmol), anhydrous THF (27 mL) 1.25 M HCl/MeOH (7.5 mL). The final compound was purified by flash chromatography (basic alumina, CH2Cl2/MeOH; 100
:
0 to 90
:
10 in 18 min) to afford 252.5 mg (0.61 mmol, 61%) of N,N′-bis(3-(piperidin-1-yl)propyl)-1,4-bis(2-aminoeth-1-yl)benzene (7{5,5}) as a yellow oil. IR (KBr): ν (cm−1): 3283 (N–H), 2933, 2852, 2801 (Csp3–H), 1676 (NH), 1443. 1H-NMR (400 MHz, CDCl3): δ (ppm): 7.13 (s, 4H, C1–H), 2.89–2.82 (m, 4H, C4–H), 2.81–2.73 (m, 4H, C3–H), 2.65 (t, 3J = 7.0 Hz, 4H, C7–H), 2.41–2.26 (m, 12H, C5–H, C8–H), 2.20 (br s, 2H, N–H), 1.67 (quint, 3J = 7.0 Hz, 4H, C6–H), 1.58–1.49 (m, 8H, C9–H), 1.47–1.36 (m, 4H, C10–H). 13C-NMR (100.6 MHz, CDCl3): δ (ppm): 137.9 (C2), 129.0 (C1), 57.9 (C5), 54.8 (C8), 51.3 (C4), 48.8 (C7), 36.0 (C3), 27.1 (C6), 26.1 (C9), 24.6 (C10). MS (70 eV, EI): m/z (%) = 414.4 (1) [M]+, 316.3 (3) [M − C6H12N]+, 288.2 (4) [M − C8H16N]+, 155.2 (60) [C9H19N2]+, 126.1 (6) [C8H16N]+, 112.1 (9) [C7H14N]+, 98.1 (100) [C6H12N]+, 84.1 (5) [C5H10N]+. HRMS (70 eV, EI): m/z calculated for C26H46N4: 414.3722, [M]+; Found: 414.3710.
N,N′-Bis(3-(imidazol-1-yl)propyl)-1,4-bis(2-aminoeth-1-yl)benzene (7{6,6}).
As stated above for 7{1,1}, but using the borane dimethylsulfide complex (1.19 mL, 11.89 mmol), N,N′-bis(3-(imidazol-1-yl)propyl)-1,4-di(aminocarbonylmethyl)benzene (13{6,6}) (408.5 mg, 1.0 mmol), anhydrous THF (27 mL), 1.25 M HCl/MeOH (7.5 mL). The final compound was purified by flash chromatography (basic alumina, CH2Cl2/MeOH; 100
:
0 to 90
:
10 in 18 min) to afford 340.5 mg (0.89 mmol, 89%) of N,N′-bis(3-(imidazol-1-yl)propyl)-1,4-bis(2-aminoeth-1-yl)benzene (7{6,6}) as a yellow oil. IR (KBr): ν (cm−1): 3283 (N–H), 3106 (Csp2–H), 2932, 2822 (Csp3–H), 1666 (NH), 1509, 1443. 1H-NMR (400 MHz, CDCl3): δ (ppm): 7.40 (s, 2H, C8–H), 7.13 (s, 4H, C1–H), 7.03 (t, 3J = 1.1 Hz, 2H, C10–H), 6.86 (t, 3J = 1.3 Hz, 2H, C9–H), 3.98 (t, 3J = 6.9 Hz, 4H, C7–H), 2.86–2.80 (m, 4H, C4–H), 2.79–2.72 (m, 4H, C3–H), 2.57 (t, 3J = 6.8 Hz, 4H, C5–H), 1.89 (m, 6H, C6–H, N–H). 13C-NMR (100.6 MHz, CDCl3): δ (ppm): 138.0 (C2), 137.4 (C8), 129.5 (C10), 129.0 (C1), 119.0 (C9), 51.1 (C4), 46.3 (C5), 44.8 (C7), 36.1 (C3), 31.4 (C6). MS (FAB): m/z (%) = 381.3 (43) [M + H]+, 380.3 (3) [M]+, 341.0 (3) [M − C3H3N2]+, 137.0 (100) [C7H11N3]+. HRMS (FAB): m/z calculated for C22H33N6: 381.2767, [M + H]+; Found: 381.2771.
N,N′-Bis(2-(piperidin-1-yl)ethyl)-1,4-bis(2-aminoeth-1-yl)benzene (7{7,7}).
As stated above for 7{1,1}, but using the borane dimethylsulfide complex (1.19 mL, 11.89 mmol), N,N′-bis(2-(piperidin-1-yl)ethyl)-1,4-di(aminocarbonylmethyl)benzene (13{7,7}) (414.6 mg, mmol), anhydrous THF (27 mL), 1.25 M HCl/MeOH (7.5 mL). The final compound was purified by flash chromatography (basic alumina, CH2Cl2/MeOH; 100
:
0 to 90
:
10 in 18 min) to afford 320.4 mg (82.8 mmol, 83%) of N,N′-bis(2-(piperidin-1-yl)ethyl)-1,4-bis(2-aminoeth-1-yl)benzene (7{7,7}) as a yellow oil. IR (KBr): ν (cm−1): 3300 (N–H), 2933, 2851, 2801 (Csp3–H), 1678 (NH), 1442. 1H-NMR (400 MHz, CDCl3): δ (ppm): 7.14 (s, 4H, C1–H), 2.89–2.84 (m, 4H, C4–H), 2.82–2.75 (m, 4H, C3–H), 2.72 (t, 4H, 3J = 6.4 Hz, C5–H), 2.42 (t, 4H, 3J = 6.4 Hz, C6–H), 2.38–2.29 (m, 8H, C7–H), 1.91 (br s, 2H, N–H), 1.55–1.47 (m, 8H, C8–H), 1.44–1.36 (m, 4H, C9–H). 13C-NMR (100.6 MHz, CDCl3): δ (ppm): 137.8 (C2), 128.7 (C1), 58.5 (C6), 54.7 (C7), 51.4 (C4), 46.6 (C5), 35.9 (C3), 26.0 (C8), 24.4 (C9). MS (FAB): m/z (%) = 387.4 (100) [M + H]+, 386.3 (11) [M]+, 288.3 (11) [M − C6H12N]+, 141.1 (9) [C8H17N2]+, 112.1 (89) [C7H14N]+. HRMS (FAB): m/z calculated for C24H38N4O2: 387.3488, [M + H]+; Found: 387.3496.
N,N′-Bis(2-(morpholin-4-yl)ethyl)-1,4-bis(2-aminoeth-1-yl)benzene (7{8,8}).
As stated above for 7{1,1}, but using the borane dimethylsulfide complex (0.48 mL, 5.1 mmol), N,N′-bis(2-(morpholin-4-yl)ethyl)-1,4-di(aminocarbonylmethyl)benzene (13{8,8}) (418.5 mg, 1.0 mmol), anhydrous THF (4 mL) 1.25 M HCl/MeOH (7.5 mL). The final compound was purified by flash chromatography (basic alumina, CH2Cl2/MeOH; 100
:
0 to 90
:
10 in 18 min) to afford 375.2 mg (0.96 mmol, 96%) of N,N′-bis(2-(morpholin-4-yl)ethyl)-1,4-bis(2-aminoeth-1-yl)benzene (7{8,8}) as a yellow oil. IR (KBr): ν (cm−1): 3305 (N–H), 2932, 2852, 2810 (Csp3–H), 1672 (NH), 1455, 1117, 867. 1H-NMR (400 MHz, CDCl3): δ (ppm): 7.14 (s, 4H, C1–H), 3.63 (t, 3J = 4.6 Hz, 8H, C8–H), 2.91–2.84 (m, 4H, C4–H), 2.83–2.75 (m, 4H, C3–H), 2.72 (t, 3J = 6.2 Hz, 4H, C5–H), 2.47 (t, 3J = 6.1 Hz, 4H, C6–H), 2.42–2.35 (m, 8H, C7–H), 1.78 (br s, 2H, N–H). 13C-NMR (100.6 MHz, CDCl3): δ (ppm): 137.8 (C2), 128.7 (C1), 66.9 (C8), 58.1 (C6), 53.6 (C7), 51.2 (C4), 45.9 (C5), 35.9 (C3). MS (70 eV, EI): m/z (%) = 390.3 (4) [M]+, 360.3 (2) [M − CH2O]+, 304.2 (3) [M − C4H8NO]+, 290.2 (27) [M − C5H10NO]+, 143.1 (14) [C7H15N2O]+, 114.1 (23) [C6H12NO]+, 100.1 (100) [C5H10NO]+. HRMS (EI): m/z calculated for C22H38N4O2: 390.2995, [M]+; Found: 390.2992.
N,N′-Bis(3-(4-methylpiperazin-1-yl)propyl)-1,4-bis(2-amino-eth-1-yl)benzene (7{9,9}).
As stated above for 7{1,1}, but using the borane dimethylsulfide complex (1.19 mL, 11.89 mmol), N,N′-bis(3-(4-methylpiperazin-1-yl)propyl)-1,4-di(aminocarbonylmethyl)benzene (13{9,9}) (472.6 mg, 1.0 mmol), anhydrous THF (27 mL) 1.25 M HCl/MeOH (7.5 mL). The final compound was purified by flash chromatography (basic alumina, CH2Cl2/MeOH; 100
:
0 to 90
:
10 in 18 min) to afford 379.8 mg (0.85 mmol, 85%) N,N′-bis(3-(4-methylpiperazin-1-yl)propyl)-1,4-bis(2-aminoeth-1-yl)benzene (7{9,9}) as a yellow crystalline solid, mp 56–58 °C. IR (KBr): ν (cm−1): 3286 (N–H), 2934, 2876, 2794 (Csp3–H), 1671 (NH), 1458. 1H-NMR (400 MHz, CDCl3): δ (ppm): 7.13 (s, 4H, C1–H), 2.89–2.81 (m, 4H, C4–H), 2.81–2.73 (m, 4H, C3–H), 2.65 (t, 3J = 7.0 Hz, 4H, C5–H), 2.59–2.28 (m, 18H, C8–H, C9–H, N–H), 2.36 (t, 3J = 7.1 Hz, 4H, C7–H), 2.27 (s, 6H, C10–H), 1.66 (quint, 3J = 7.0 Hz, 4H, C6–H). 13C-NMR (100.6 MHz, CDCl3): δ (ppm): 137.9 (C2), 128.9 (C1), 56.9 (C7), 55.1 (C9), 53.3 (C8), 51.2 (C4), 48.5 (C5), 46.1 (C10), 36.0 (C3), 27.0 (C6). MS (70 eV, EI): m/z (%) = 444.4 (19) [M]+, 429.4 (3) [M − CH3]+, 414.4 (3) [M − C2H6]+, 401.4 (11) [M − C2H5N]+, 170.2 (16) [C9H20N3]+, 141.1 (11) [C8H17N2]+, 127.1 (19) [C7H15N2]+, 113.1 (100) [C6H13N2]+, 99.1 (13) [C5H11N2]+. HRMS (70 eV, EI): m/z calculated for C26H48N6: 444.3940, [M]+; Found: 444.3943.
2-(4-(Bromomethyl)phenyl)acetyl chloride (15).
To a solution of 0.235 g (1 mmol) of 2-(4-(bromomethyl)phenyl)acetic acid (14) in 10 mL of toluene was added 0.385 g (3.2 mmol) of thionyl chloride. The mixture was heated to 90 °C for 6 hours to obtain a yellow solution. The mixture was cooled to room temperature and the solvent was removed under reduced pressure to give 0.237 g (0.96 mmol, 96%) of 2-(4-(bromomethyl)phenyl)acetyl chloride (15) as a yellow solid which was used without further purification. 1H-NMR (400 MHz, CDCl3) δ (ppm): 7.44–7.38 (m, 2H, C3–H), 7.28–7.24 (m, 2H, C4–H), 4.48 (s, 2H, C1–H), 4.14 (s, 2H, C6–H). 13C-NMR (100.6 MHz, CDCl3) δ (ppm): 171.6 (C7), 137.8 (C2), 131.3 (C5), 129.9 (C3), 129.6 (C4), 52.6 (C6), 32.6 (C1).
2-(4-(Bromomethyl)phenyl)-N-(3-morpholinopropyl)acetamide (16{4}).
0.3884 g (1.57 mmol) of 2-(4-(bromomethyl)phenyl)acetyl chloride (15) was dissolved in 10 mL of acetonitrile and cooled at 0 °C and it was added dropwise to a solution of 0.226 g (1.57 mmol) of N-aminopropylmorpholine (12{4}) and 0.651 g (4.71 mmol) of potassium carbonate in 10 mL of acetonitrile. The solution was heated under microwave irradiation at 100 °C for 25 minutes. The mixture was filtered and the solvent was removed under reduced pressure. The orange solid was dissolved in 10 mL of chloroform and the solution was filtered again. The solvent was removed under reduced pressure to obtain 0.379 g (1.07 mmol, 68%) of 2-(4-(bromomethyl)phenyl)-N-(3-morpholinopropyl)acetamide (16{4}) as an orange foamy solid. 1H-NMR (400 MHz, CDCl3) δ (ppm): 7.50–7.30 (m, 4H, C3–H, C4–H), 4.57 (s, 2H, C1–H), 4.25–3.91 (m, 4H, C12–H, C13–H), 3.58 (s, 2H, C6–H), 3.37 (q, J = 6.1 Hz, 2H, C9–H), 2.92 (t, J = 7.0 Hz, 2H, C11–H), 2.14–2.04 (q, J = 6.8 Hz, 2H, C10–H). 13C-NMR (100.6 MHz, CDCl3) δ (ppm): 171.9 (C7), 136.3 (C2), 135.7 (C5), 129.7 (C3), 129.0 (C4), 63.4 (C13), 55.1 (C11), 52.0 (C12), 46.0 (C1), 43.2 (C6), 36.1 (C9), 23.1 (C10).
2-(4-(Bromomethyl)phenyl)-N-(3-(pyrrolidin-1-yl)propyl)acetamide (16{1}).
As stated above for 16{4}, but using 0.224 g (0.905 mmol) of 2-(4-(bromomethyl)phenyl)acetyl chloride (15), 6 mL of acetonitrile, 0.116 g (0.905 mmol) of N-aminopropylpyrrolidine (12{1}), and 0.375 g (2.715 mmol) of potassium carbonate in 4 mL of acetonitrile. The crude product was purified using chromatographic filtration (basic alumina, cyclohexane) to afford 0.215 g (0.634 mmol, 70%) of 2-(4-(bromomethyl)phenyl)-N-(3-(pyrrolidin-1-yl)propyl)acetamide (16{1}) as a pale orange foamy solid. 1H-NMR (400 MHz, CDCl3) δ (ppm): 7.45 (br m, 1H, N8–H), 7.41–7.33 (m, 4H, C3-H, C4–H), 4.56 (s, 2H, C1–H), 3.58 (s, 2H, C6–H), 3.37 (q, J = 6.1 Hz, 2H, C9–H), 2.98 (t, J = 6.9 Hz, 2H, C11–H), 3.25–2.50 (s, 4H, C12–H), 2.07–2.17 (m, 4H, C13–H), 2.06–2.01 (m, 2H, C10–H). 13C NMR (100.6 MHz, CDCl3) δ (ppm): 171.9 (C7), 136.2 (C2), 135.7 (C5), 129.7 (C3), 129.0 (C4), 53.7 (C12), 52.7 (C11), 46.0 (C1), 43.3 (C6), 36.1 (C9), 25.2 (C14), 23.2 (C13).
2-(4-(Bromomethyl)phenyl)-N-(3-(piperidin-1-yl)propyl)acetamide (16{5}).
As stated above for 16{4}, but using 0.529 g (2.14 mmol) of 2-(4-(bromomethyl)phenyl)acetyl chloride (15), 20 mL of acetonitrile, 0.304 g (2.14 mmol) of 3-(piperidin-1-yl)propan-1-amine (12{5}) and 0.887 g (6.42 mmol) of potassium carbonate. 0.2268 g (0.64 mmol, 30%) of 2-(4-(bromomethyl)phenyl)-N-(3-(piperidin-1-yl)propyl)acetamide (16{5}) were obtained as a dark orange foamy solid. 1H-NMR (400 MHz, methanol-d4) δ (ppm): 7.47–7.25 (m, 5H, C3–H, C4–H, N8–H), 4.62 (s, 2H, C1–H), 3.54 (s, 2H, C6–H), 3.16–3.04 (m, 2H, C9–H), 2.15 – 1.65 (m, 14H, C10–H, C11–H, C12–H, C13–H, C14–H). 13C NMR (100.6 MHz, methanol-d4) δ (ppm): 170.4 (C7), 132.8 (C2), 129.9 (C5), 129.0 (C3), 128.6 (C4), 54.1 (C11), 52.8 (C12), 45.1 (C6), 42.0 (C9), 35.7 (C1), 24.0 (C10), 22.8 (C13), 21.2 (C14).
2-(4-(Bromomethyl)phenyl)-N-(3-(2-methylpiperidin-1-yl)propyl)acetamide (16{3}).
As stated above for 16{4}, but using 0.4905 g (1.98 mmol) of 2-(4-(bromomethyl)phenyl)acetyl chloride (15), 20 mL of acetonitrile, 0.310 g (1.98 mmol) of 3-(piperidin-1-yl)propan-1-amine (12{3}) and 0.821 g (5.94 mmol) of potassium carbonate. 0.1455 g (0.40 mmol, 20%) of 2-(4-(bromomethyl)phenyl)-N-(3-(2-methylpiperidin-1-yl)propyl)acetamide (16{3}) were obtained as a dark orange foamy solid. 1H-NMR (400 MHz, CDCl3) δ (ppm): 7.37–7.33 (m, 2H, C3–H), 7.30–7.25 (m, 2H, C4–H) 7.15 (br s, 1H, N8–H), 4.58 (s, 2H, C1–H), 3.53 (s, 2H, C6–H), 3.43–3.35 (m, 1H, C9–H), 3.27–3.18 (m, 1H, C9–H), 2.84–2.78 (m, 1H, C11–H), 2.78–2.71 (m,1H, C12–H), 2.25–2.19 (m, 2H, C12–H, C16–H), 2.03–1.97 (m, 1H, C11–H), 1.66–1.51 (m, 5H, C13–H, C10–H, C14–H, C15–H), 1.43–1.37 (m, 1H, C10–H), 1.30 (m, 1H, C14–H), 1.21–1.15 (m, 1H, C15–H), 0.98 (d, J = 6.3 Hz, 3H, C17–H). 13C-NMR (100.6 MHz, CDCl3) δ (ppm): 170.3 (C7), 136.2 (C2), 135.7 (C5), 129.6 (C3), 129.0 (C4), 56.3 (C16), 52.7 (C12), 51.7 (C11), 45.9 (C1), 43.6 (C6), 39.9 (C9), 34.5 (C15), 25.9 (C10), 24.9 (C13), 23.5 (C14), 18.6 (C17).
2-(4-((3-Morpholinopropylamino)methyl)phenyl)-N-(3-morpholinopropyl)acetamide (17{4,4}).
0.1475 g (0.42 mmol) of 2-(4-(bromomethyl)phenyl)-N-(3-morpholinpropyl)acetamide (16{4}) were dissolved in 6 mL of acetonitrile and a few drops of methanol and cooled at 0 °C and it was added dropwise to a solution of 0.0599 g (0.42 mmol) of 3-aminopropyl-N-morpholine (12{4}) and 0.1741 g (1.26 mmol) of potassium carbonate in 4 mL of acetonitrile. The solution was heated under microwave irradiation at 150 °C for 25 minutes. The mixture was filtered and the solvent was removed under reduced pressure. The residue was separated by automatic flash chromatography (basic alumina; 95
:
5
:
0.5; CH2Cl2/MeOH/NH3) to afford 0.0598 g (0.143 mmol, 34%) of 2-(4-((3-morpholinopropylamino)methyl)phenyl)-N-(3-morpholinopropyl)acetamide (17{4,4}) as an orange oil. IR (KBr) ν (cm−1): 3292 (N–H), 2927, 2854, 2813 (Csp3–H), 1649 (C
O), 1552 (N–H), 1116 (C–O–C), 862 (C–O–C). 1H-NMR (400 MHz, CDCl3) δ (ppm): 7.32–7.28 (m, 2H, C2–H), 7.25–7.20 (m, 2H, C3–H), 6.37 (br s,1H, N8–H), 3.77 (s, 2H, C1–H), 3.72–3.56 (m, 8H, C13–H), 3.53 (s, 2H, C6–H), 3.31 (q, J = 6.4 Hz, 2H, C9–H), 2.70 (t, J = 6.9, 2H, C15–H), 2.45–2.32 (m, 13H, N14–H, C11–H, C12–H), 1.72 (q, J = 6.9 Hz, 2H, C10–H), 1.66–1.58 (q, J = 6.6 Hz, 2H, C16–H). 13C-NMR (100.6 MHz, CDCl3) δ (ppm): 170.8 (C7), 139.3 (C2), 133.7 (C5), 129.3 (C3), 128.6 (C4), 66.9 (C13), 57.2 (C1), 53.8 (C11, C12), 48.0 (C15), 43.6 (C6), 38.9 (C9), 26.5 (C10), 25.1 (C16). MS (70 eV, EI) m/z (%) = 418.4 (17) [M]+, 388.3 (12) [M − CH2O]+, 276.2 (29) [M − C7H15N2O]+, 100.1 (100) [C5H10NO]+. HRMS (EI): m/z calculated for C23H38N4O3 [M]+: 418.2944; Found: 418.2940.
2-(4-((3-(Pyrrolidin-1-yl)propylamino)methyl)phenyl)-N-(3-morpholinopropyl)acetamide (17{4,1}).
As stated above for 17{4,4}, but using 0.8193 g (2.31 mmol) of 2-(4-(bromomethyl)phenyl)-N-(3-morpholinpropyl)acetamide (16{4}), 20 mL of acetonitrile, 0.2967 g (2.31 mmol) of 3-aminopropyl-N-pyrrolidine (12{1}) and 0.959 g (6.94 mmol) of potassium carbonate. Upon removal of the solvent, the residue was separated by automatic flash chromatography (basic alumina; 98
:
2
:
0.5; CH2Cl2/MeOH/NH3) to afford 0.2790 g (0.693 mmol, 30%) of 2-(4-((3-(pyrrolidin-1-yl)propyl-amino)methyl)phenyl)-N-(3-morpholinopropyl)acetamide (17{4,1}) as an orange oil. IR (KBr) ν (cm−1): 3284 (N–H), 2951, 2854, 2806 (Csp3–H), 1647 (C
O), 1550 (N–H), 1116 (C–O–C), 862 (C–O–C). 1H-NMR (400 MHz, CDCl3) δ (ppm): 7.35–7.27 (m, 2H, C3–H), 7.25–7.17 (m, 2H, C4–H), 6.50 (br m, 1H, N8–H), 3.78 (s, 2H, C7–H), 3.63–3.55 (m, 4H, C13–H), 3.52 (s, 2H, C6–H), 3.29 (q, J = 6.5 Hz, 2H, C9–H), 2.71 (t, J = 7.0 Hz, 2H, C15–H), 2.60–2.39 (m, 7H, N14–H, C17–H, C18–H), 2.39–2.30 (m, 6H, C11–H, C12–H), 1.85–1.68 (m, 6H, C16–H, C19–H), 1.62 (q, J = 6.6 Hz, 2H, C10–H). 13C-NMR (100.6 MHz, CDCl3) δ (ppm): 170.9 (C7), 139.2 (C2), 133.7 (C5), 129.3 (C3), 128.6 (C4), 66.8 (C13), 57.1 (C11), 54.7 (C17), 54.2 (C18), 53.6 (C12), 53.4 (C1), 48.1 (C15), 43.5 (C6), 38.8 (C9), 29.0 (C16), 25.2 (C10), 23.4 (C19). MS (70 eV, EI): m/z (%) = 402.4 (1) [M]+, 372.3 (2) [M − CH2O]+, 276.2 (14) [M − C7H14N2]+, 189.1 (10) [M − C11H23N3O]+, 171.1 (11) [C8H15N2O2]+, 127.1 (38) [C7H15N2]+, 100.1 (100) [C5H10NO]+, 84.1 (100) [C5H10N]+, 70.1 (30) [C4H8N]+. HRMS (EI): m/z calculated for C23H37N4O2 [M − H]+: 401.2917; Found: 401.2915.
2-(4-((3-(2-Methylpiperidin-1-yl)propylamino)methyl)phenyl)-N-(3-morpholinopropyl)acetamide (17{4,3}).
As stated above for 17{4,4}, but using 0.7520 g (2.12 mmol) of 2-(4-(bromomethyl)phenyl)-N-(3-morpholinpropyl)acetamide (16{4}), 20 mL of acetonitrile, 0.331 g (2.12 mmol) of 3-aminopropyl-N-pipecoline (12{3}) and 0.879 g (6.36 mmol) of potassium carbonate. Upon removal of the solvent, the residue was separated by automatic flash chromatography (basic alumina; 98
:
2
:
0.5; CH2Cl2/MeOH/NH3) to afford 0.1826 g (0.424 mmol, 20%) of 2-(4-((3-(2-methylpiperidin-1-yl)propylamino)methyl)phenyl)-N-(3-morpholinopropyl)acetamide (17{4,4}) as a dark orange oil. IR (KBr) ν (cm−1): 3289 (N–H), 3054 (Csp2–H), 2930, 2853, 2807 (Csp3–H), 1647 (C
O), 1551 (N–H), 1445 (CH2), 1118 (C–O–C), 863 (C–O–C). 1H-NMR (400 MHz, CDCl3) δ (ppm): 7.31 (m, 2H, C3–H), 7.21 (m, 2H, C4–H), 6.39 (br m, 1H, N8–H), 3.77 (s, 2H, C1–H), 3.58 (m, 4H, C13–H), 3.53 (s, 2H, C6–H), 3.30 (m, 2H, C9–H), 2.91–2.80 (m, 1H, C17–H), 2.78–2.68 (m, 1H, C1–H8), 2.65 (t, J = 6.9 Hz, 2H, C15–H), 2.46–2.19 (m, 9H, C11–H, C12–H, C18–H, C22–H, N14–H), 2.08–2.14 (m, 1H, C17–H), 1.77–1.21 (m, 10H, C10–H, C16–H, C19–H, C20–H, C21–H), 1.04 (d, J = 6.2 Hz, 3H, C23–H). 13C-NMR (100.6 MHz, CDCl3) δ (ppm): 170.9 (C7), 139.6 (C2), 133.6 (C5), 129.3 (C3), 128.6 (C4), 66.8 (C13), 57.2 (C11), 55.9 (C22), 53.6 (C12), 53.6 (C1), 52.2 (C17), 52.0 (C18), 48.4 (C15), 43.6 (C6), 38.9 (C9), 34.6 (C21), 26.1 (C10), 25.8 (C16), 25.2 (C19), 23.9 (C20), 19.0 (C23). MS (70 eV, EI): m/z (%) = 430.1 (15) [M]+, 429.1 (31) [M − H]+, 400.1 (2) [M − CH2O]+, 331.0 (3) [M − C6H11N]+, 303.9 (4) [M − C8H16N]+, 275.9 (12) [M − C9H18N2]+, 170.8 (13) [C8H15N2O2]+, 154.8 (19) [C9H19N2]+, 125.9 (28) [C8H16N]+, 111.9 (100) [C7H14N]+, 99.9 (40) [C5H10NO]+. HRMS (EI): m/z calculated for C25H42N4O2 [M]+: 430.3308; Found: 430.3303.
2-(4-((3-(Piperidin-1-yl)propylamino)methyl)phenyl)-N-(3-(piperidin-1-yl)propyl)acetamide (17{5,5}).
As stated above for 17{4,4}, but using 0.7192 g (2.03 mmol) of 2-(4-(bromomethyl)phenyl)-N-(3-piperidin-1-ylpropyl)acetamide (16{5}), 20 mL of acetonitrile, 0.2895 g (2.03 mmol) of 3-aminopropyl-N-piperidine (12{5}) and 0.8417 g (6.09 mmol) of potassium carbonate. Upon removal of the solvent, the residue was separated by automatic flash chromatography (basic alumina; 98
:
2
:
0.5; CH2Cl2/MeOH/NH3) to afford 0.1515 g (0.365 mmol, 18%) of 2-(4-((3-(piperidin-1-yl)propylamino)methyl)phenyl)-N-(3-(piperidin-1-yl)propyl) acetamide (17{5,5}) as an orange oil. IR (KBr) ν (cm−1): 3293 (N–H), 3055 (Csp2–H), 2931, 2852, 2768 (Csp3–H), 1644 (C
O), 1548 (N–H), 1441. 1H-NMR (400 MHz, CDCl3) δ (ppm): 7.35–7.28 (m, 2H, C3–H), 7.22 (m, 2H, C4–H), 7.00 (br m, 1H, N8–H), 3.76 (s, 2H, C1–H), 3.50 (s, 2H, C6–H), 3.30 (m, 2H, C9–H), 2.69 (t, J = 6.8 Hz, 2H, C16–H), 2.48–2.22 (m, 13H, N15–H, C11–H, C12–H, C18–H, C19–H), 1.78–1.38 (m, 16H, C10–H, C17–H, C13–H, C14–H, C20–H, C21–H). 13C-NMR (100.6 MHz, CDCl3) δ (ppm): 170.8 (C7), 138.5 (C2), 133.8 (C5), 129.2 (C3), 129.0 (C4), 57.9 (C11), 57.7 (C18), 54.6 (C12), 54.5 (C19), 53.4 (C1), 48.2 (C16), 43.6 (C6), 39.5 (C9), 26.0 (C17), 26.0 (C10), 25.0 (C14, C21), 24.3 (C20), 24.2 (C13). MS (70 eV, EI): m/z (%) = 414.4 (5) [M]+, 288.3 (22) [M − C8H16]+, 274.3 (91) [M − C8H16N]+, 169.2 (27) [C9H17N2O]+, 141.1 (46) [C8H17N2]+, 126.1 (11) [C8H16N]+, 112.1 (43) [C7H14N]+, 98.0 (100) [C6H12N]+, 84.0 (48) [C5H10N]+. HRMS (EI): m/z calculated for C25H42N4O [M]+: 414.3359; Found: 414.3359.
2-(4-((3-(2-Methylpiperidin-1-yl)propylamino)methyl)phenyl)-N-(3-(piperidin-1-yl)propyl)acetamide (17{5,3}).
As stated above for 17{4,4}, but using 1.0457 g (2.96 mmol) of 2-(4-(bromomethyl)phenyl)-N-(3-(piperidin-1-yl)propyl)acetamide (16{5}), 20 mL of acetonitrile, 0.4625 g (2.96 mmol) of 3-aminopropyl-N-pipecoline (12{3}) and 1.2273 g (8.88 mmol) of potassium carbonate. Upon removal of the solvent, the residue was separated by automatic flash chromatography (basic alumina; 98
:
2
:
0.5; CH2Cl2/MeOH/NH3) to afford 0.3426 g (0.799 mmol, 27%) of 2-(4-((3-(2-methylpiperidin-1-yl)propylamino)methyl)phenyl)-N-(3-(piperidin-1-yl)propyl)acetamide (17{5,3}) as a dark orange oil. IR (KBr) ν (cm−1): 3286 (N–H), 3054 (Csp2–H), 2931, 2852, 2800 (Csp3–H), 1647 (C
O), 1552 (N–H). 1H NMR (400 MHz, CDCl3) δ (ppm): 7.33–7.27 (m, 2H, C3–H), 7.22 (m, 2H, C4–H), 7.02 (br s, 1H, N8–H), 3.72 (s, 2H, C1–H), 3.50 (s, 2H, C6–H), 3.30 (q, J = 5.8 Hz, 2H, C9–H), 2.92–2.75 (m, 2H, C18–H, C19–H), 2.72–2.62 (m, 2H, C16–H), 2.52–2.22 (m, 9H, C11–H, C12–H, N15–H, C23–H, C19–H), 2.21–2.09 (m, 1H, C18–H), 1.80–1.22 (m, 16H, C10–H, C13–H, C14–H, C17–H, C20–H, C21–H, C22–H), 1.06 (d, J = 6.2 Hz, 3H, C24–H). 13C NMR (100.6 MHz, CDCl3) δ (ppm): 170.7 (C7), 138.7 (C2), 134.1 (C5), 129.3 (C3), 128.6 (C4), 57.9 (C19), 56.1 (C22), 54.5 (C12), 53.5 (C1), 52.2 (C11), 51.9 (C18), 48.3 (C16), 43.7 (C6), 39.6 (C9), 34.3 (C22), 26.0 (C13, C14), 25.9 (C10), 25.4 (C17), 25.1 (C20), 24.3 (C21), 18.8 (C24). MS (70 eV, EI): m/z (%) = 428.5 (5) [M]+, 288.3 (21) [M − C9H18N]+, 274.3 (60) [M − C9H18N2]+, 169.2 (26) [C9H17NO]+, 155.2 (59) [C9H19N2]+, 126.1 (38) [C8H16N]+, 112.0 (75) [C7H14N]+, 98.2 (100) [C6H12N]+, 84.1 (34) [C5H10N]+. HRMS (EI): m/z calculated for C26H44N4O [M]+: 428.3515; Found: 428.3495.
2-(4-((3-(2-Methylpiperidin-1-yl)propylamino)methyl)phenyl)-N-(3-(2-methylpiperidin-1-yl)propyl)acetamide (17{3,3}).
As stated above for 17{4,4}, but using 0.6312 g (1.72 mmol) of 2-(4-(bromomethyl)phenyl)-N-(3-(2-methylpiperidin-1-yl)propyl)acetamide (16{3}), 20 mL of acetonitrile, 0.2688 g (1.72 mmol) of 3-aminopropyl-N-pipecoline (12{3}) and 0.7132 g (5.16 mmol) of potassium carbonate. Upon removal of the solvent, the residue was separated by automatic flash chromatography (basic alumina; 99
:
1
:
0.5; CH2Cl2/MeOH/NH3) to afford 0.2437 g (0.55 mmol, 32%) of 2-(4-((3-(2-methylpiperidin-1-yl)propylamino)methyl)phenyl)-N-(3-(2-methylpiperidin-1-yl)propyl)acetamide (17{3,3}) as a dark orange oil. IR (KBr) ν (cm−1): 3286 (N–H), 3054 (Csp2–H), 2929, 2854, 2793 (Csp3–H), 1647 (C
O), 1552 (N–H). 1H NMR (400 MHz, CDCl3) δ (ppm): 7.33–7.27 (m, 2H, C3–H), 7.24–7.18 (m, 2H, C4–H), 6.94 (br m, 1H, N8–H), 3.76 (s, 2H, C1–H), 3.51 (s, 2H, C6–H), 3.32–3.40 (m, 1H, C9–H), 3.17–3.24 (m, 1H, C9–H), 2.89–2.62 (m, 4H, C11–H, C12–H, C21–H, C22–H), 2.47–1.94 (m, 7H, C11–H, C12–H, C16–H, C21–H, C22–H, C26–H, N8–H), 1.71–1.14 (m, 16H, C10–H, C13–H, C14–H, C15–H, C20–H, C23–H, C24–H, C25–H), 1.08–0.94 (m, 6H, C17–H, C27–H). 13C NMR (100.6 MHz, CDCl3) δ (ppm): 170.7 (C7), 138.9 (C2), 133.8 (C5), 129.3 (C3), 128.7 (C4), 56.3 (C16), 55.9 (C26), 53.7 (C1), 52.6 (C11), 52.3 (C12), 51.8 (C21), 48.4 (C22), 43.7 (C6), 39.6 (C9), 34.6 (C15, C25), 26.1 (C10), 25.8 (C20), 25.0 (C13), 23.8 (C14), 23.3 (C23), 22.5 (C24), 19.2 (C17), 18.9 (C27). MS (70 eV, EI): m/z (%) = 442.8 (2) [M]+, 302.3 (10) [M − C9H18N]+, 288.1 (29) [M − C9H18N2]+, 183.2 (9) [C10H19N2O]+, 155.2 (23) [C9H19N]+, 126.1 (19) [C8H16N]+, 112.1 (100) [C7H14N]+, 98.1 (66) [C6H12N]+. HRMS (EI): m/z calculated for C27H46N4O [M]+: 442.3672; found: 442.3673.
N-(4-(2-(3-Morpholinopropylamino)ethyl)benzyl)-3-morpholinopropan-1-amine (8{4,4}).
The borane dimethylsulfide complex (0.450 mL, 5.93 mmol) was added dropwise at 0 °C to a solution of 2-(4-((3-morpholinopropylamino)methyl)phenyl)-N-(3-morpholinopropyl)acetamide (17{4,4}) (03
315 g, 0.792 mmol) in anhydrous THF (15 mL). The solution was refluxed for 6 h under N2, cooled down to room temperature, diluted with 8 mL of 1.25 M HCl/MeOH solution and refluxed for 1 h. The solvent was removed in vacuo and the crude material was diluted with 1 M NaOH solution until pH = 14. The aqueous solution was extracted five times with CH2Cl2, dried with MgSO4, and concentrated. Purification of the residue by automatic flash chromatography (basic alumina, CH2Cl2/MeOH/NH3, 98
:
2
:
0.5) afforded 0.1487 g (0.368 mmol, 46%) of N-(4-(2-(3-morpholinopropylamino)ethyl)benzyl)-3-morpholinopropan-1-amine (8{4,4}) as a yellow oil. IR (KBr) ν (cm−1): 3297 (N–H), 2936, 2852, 2807 (Csp3–H), 1669 (N–H), 1456 (CH2), 1117 (C–O–C), 862 (C–O–C). 1H NMR (400 MHz, CDCl3) δ (ppm): 7.26 (m, 2H, C3–H), 7.18–7.13 (m, 2H, C4–H), 3.81–3.72 (m, 2H, C1–H), 3.72–3.62 (m, 8H, C13–H), 2.89–2.84 (m, 2H, C7–H), 2.79 (m, 2H, C8–H), 2.74–2.61 (m, 4H, C9–H, C15–H), 2.46–2.28 (m, 12H, C11–H, C12–H), 2.08 (br s, 2H, N8–H, N14–H), 1.75–1.61 (m, 4H, C10–H). 13C NMR (100.6 MHz, CDCl3) δ (ppm): 138.3 (C2), 137.6 (C5), 128.8 (C3), 128.4 (C4), 66.8 (C13), 57.3 (C11), 53.7 (C12), 53.7 (C1), 51.1 (C7), 48.3 (C9), 48.0 (C15) 35.8 (C6), 26.5 (C10). MS (70 eV, EI): m/z (%) = 404.3 (6) [M]+, 374.3 (6) [M − CH2O]+, 157.1 (13) [C8H17N2O]+, 114.1 (15) [C6H12NO]+, 100.1 (100) [C5H10NO]+, 86.1 (5) [C4H8NO]+. HRMS (EI): m/z calculated for C23H40N4O2 [M]+: 404.3151; Found: 404.3158.
N-(4-(2-(3-Morpholinopropylamino)ethyl)benzyl)-3-(pyrroli-din-1-yl)propan-1-amine (8{4,1}).
As stated above for 8{4,4}, but using 0.2374 g (0.59 mmol) of 2-(4-((3-(pyrrolidin-1-yl)propylamino)methyl)phenyl)-N-(3-morpholinopropyl)acetamide (17{4,1}), 0.335 mL (0.2689 g, 3.54 mmol) of the borane dimethylsulfide complex, 15 mL of THF and 7 mL of 1.25 M HCl/MeOH. Upon removal of the solvent, the residue was separated by automatic flash chromatography (basic alumina; 98
:
2
:
0.5; CH2Cl2/MeOH/NH3) to afford 0.1100 g (0.28 mmol, 48%) of N-(4-(2-(3-morpholinopropylamino)ethyl)benzyl)-3-(pyrrolidin-1-yl)propan-1-amine (8{4,1}). IR (KBr) ν (cm−1): 3288 (N–H), 2930, 2852, 2804 (Csp3–H), 1663 (N–H), 1457 (CH2), 1118 (C–O–C), 862 (C–O–C). 1H NMR (400 MHz, CDCl3) δ (ppm): 7.28–7.21 (m, 2H, C3–H), 7.13–7.19 (m, 2H, C4–H), 3.79–3.72 (m, 2H, C1–H), 3.64–3.67 (m, 4H, C13–H), 2.90–2.84 (m, 2H, C7–H), 2.76–2.81 (m, 2H, C6–H), 2.66–2.72 (m, 4H, C9–H, C15–H), 2.53–2.33 (m, 12H, C11–H, C12–H, C17–H, C18–H), 2.01 (s, 2H, N8–H, N14–H), 1.82–1.57 (m, 8H, C10–H, C16–H, C19–H). 13C NMR (100.6 MHz, CDCl3) δ (ppm): 138.5 (C2), 137.6 (C5), 128.6 (C3), 128.2 (C4), 66.9 (C13), 57.3 (C11, C17), 54.2 (C18), 53.7 (C12), 53.6 (C1), 51.1 (C7), 48.3 (C9), 48.0 (C15), 35.8 (C6), 26.5 (C10, C16), 23.3 (C19). MS (70 eV, EI): m/z (%) = 388.2 (32) [M]+, 387.2 (79) [M − H]+, 358.2 (3) [M − CH2O]+, 290.0 (9) [M − C6H12N]+, 262.0 (17) [M − C7H15N2]+, 156.9 (36) [C8H17N2O]+, 99.9 (100) [C5H10NO]+, 84.0 (100) [C5H10N]+, 70.1 (24) [C4H8N]+. HRMS (EI): m/z calculated for C23H39N4O [M − H]+: 387.3124; Found: 387.3123.
N-(4-(2-(3-Morpholinopropylamino)ethyl)benzyl)-3-(2-methylpiperidin-1-yl)propan-1-amine (8{4,3}).
As stated above for 8{4,4}, but using 0.1678 g (0.39 mmol) of 2-(4-((3-(2-methylpiperidin-1-yl)propylamino)methyl)phenyl)-N-(3-morpholinopropyl)acetamide (17{4,3}), 0.222 mL (0.1778 g, 2.34 mmol) of the borane dimethylsulfide complex, 10 mL of THF and 5 mL of 1.25 M HCl/MeOH. Upon removal of the solvent, the residue was separated by automatic flash chromatography (basic alumina; 98
:
2
:
0.5; CH2Cl2/MeOH/NH3) to afford 0.0764 g (0.18 mmol, 47%) of N-(4-(2-(3-morpholinopropylamino)ethyl)benzyl)-3-(2-methylpiperidin-1-yl)propan-1-amine (8{4,3}). IR (KBr) ν (cm−1): 3288 (N–H), 2929, 2852, 2805 (Csp3–H), 1671 (N–H), 1455 (CH2), 1118 (C–O–C), 862 (C–O–C). 1H NMR (400 MHz, CDCl3) δ (ppm): 7.29–7.10 (m, 4H, C3–H, C4–H), 3.82–3.72 (m, 2H, C1–H), 3.71–3.61 (m, 4H, C13–H), 2.89–2.84 (m, 2H, C7–H), 2.78–2.81 (m, 2H, C6–H), 2.75–2.63 (m, 6H, C9–H, C15–H, C17–H, C18–H), 2.50–1.95 (m, 11H, C11–H, C12–H, C17–H, C18–H, C22–H, N8–H, N14–H), 1.71–1.25 (m, 10H, C10–H, C16–H, C19–H, C20–H, C21–H), 1.10–0.97 (m, 3H, C23–H). 13C NMR (100.6 MHz, CDCl3) δ (ppm): 138.6 (C2), 137.9 (C5), 128.6 (C3), 128.2 (C4), 66.9 (C13), 57.3 (C11), 55.9 (C22), 53.7 (C12), 53.6 (C1), 52.2 (C17, C18), 51.1 (C7), 48.4 (C9), 48.3 (C15), 35.8 (C6), 34.5 (C21), 26.6 (C10), 26.0 (C16), 25.5 (C19), 23.8 (C20), 18.9 (C23). MS (70 eV, EI): m/z (%) = 416.1 (20) [M]+, 415.1 (38) [M − H]+, 386.1 (16) [M − CH2O]+, 259.9 (11) [M − C8H17N2O]+, 156.8 (24) [C8H17N2O]+, 125.9 (32) [C8H16N]+, 111.9 (100) [C7H14N]+, 99.9 (61) [C6H12N]+. HRMS (EI): m/z calculated for C25H43N4O [M − H]+: 415.3437; Found: 415.3438.
N-(4-(2-(3-(Piperidin-1-yl)propylamino)ethyl)benzyl)-3-(piperidin-1-yl)propan-1-amine (8{5,5}).
As stated above for 8{4,4}, but using 0.1134 g (0.27 mmol) of 2-(4-((3-(piperidin-1-yl)propylamino)methyl)phenyl)-N-(3-(piperidin-1-yl)propyl)acetamide (17{5,5}), 0.156 mL (0.1247 g, 1.64 mmol) of the borane dimethylsulfide complex, 10 mL of THF and 5 mL of 1.25 M HCl/MeOH. Upon removal of the solvent, the residue was separated by automatic flash chromatography (basic alumina; 98
:
2
:
0.5; CH2Cl2/MeOH/NH3) to afford 0.0403 g (0.101 mmol, 37%) of N-(4-(2-(3-morpholinopropylamino)ethyl)benzyl)-3-(2-methylpiperidin-1-yl)propan-1-amine (8{5,5}). IR (KBr) ν (cm−1): 3285 (N–H), 2928, 2852, 2800 (Csp3–H), 1673 (N–H), 1455 (CH2). 1H NMR (400 MHz, CDCl3) δ (ppm): 7.27–7.22 (m, 2H, C3–H), 7.19–7.12 (m, 2H, C4–H), 3.76 (s, 2H, C1–H), 2.90–2.84 (m, 2H, C7–H), 2.77–2.82 (m, 2H, C6–H), 2.67 (t, J = 6.9 Hz, 4H, C9–H), 2.47–2.21 (m, 12H, C11–H, C12–H), 2.05 (br s, 2H, N8–H), 1.70 (m, 4H, C10–H), 1.54 (m, 8H, C13–H), 1.41 (m, 4H, C14–H). 13C NMR (100.6 MHz, CDCl3) δ (ppm): 138.5 (C2), 137.7 (C5), 128.7 (C3), 128.2 (C4), 57.7 (C11), 54.6 (C12), 53.5 (C1), 51.0 (C7), 48.7 (C9), 35.7 (C6), 26.7 (C10), 25.9 (C13), 24.3 (C14). MS (70 eV, EI): m/z (%) = 400.4 (1) [M]+, 260.2 (3) [M − C8H16N2]+, 155.1 (7) [C9H19N2]+, 112.1 (8) [C7H14N]+, 98.1 (100) [C6H12N]+, 84.0 (8) [C5H10N]+. HRMS (EI): m/z calculated for C25H44N4 [M]+: 400.3566; Found: 400.3577.
N-(4-(2-(3-(Piperidin-1-yl)propylamino)ethyl)benzyl)-3-(2-methylpiperidin-1-yl)propan-1-amine (8{5,3}).
As stated above for 8{4,4}, but using 0.1848 g (0.43 mmol) of 2-(4-((3-(2-methylpiperidin-1-yl)propylamino)methyl)phenyl)-N-(3-(piperidin-1-yl)propyl)acetamide (17{5,3}), 0.245 mL (0.1965 g, 2.59 mmol) of the borane dimethylsulfide complex, 12 mL of THF and 6 mL of 1.25 M HCl/MeOH. Upon removal of the solvent, the residue was separated by automatic flash chromatography (basic alumina; 98
:
2
:
0.5; CH2Cl2/MeOH/NH3) to afford 0.0642 g (0.16 mmol, 36%) of N-(4-(2-(3-(piperidin-1-yl)propylamino)ethyl)benzyl)-3-(2-methylpiperidin-1-yl)propan-1-amine (8{5,3}). IR (KBr) ν (cm−1): 3283 (N–H), 2931, 2852, 2800 (Csp3–H), 1671 (N–H), 1443 (CH2). 1H NMR (400 MHz, CDCl3) δ (ppm): 7.27–7.20 (m, 2H, C3–H), 7.13–7.18 (m, 2H, C4–H), 3.74 (s, 2H, C1–H), 2.88–2.84 (m, 2H, C7–H), 2.82–2.77 (m, 2H, C6–H), 2.76–2.63 (m, 4H, C9–H, C16–H), 2.48–1.99 (m, 13H, C11–H, C12–H, C18–H, C19–H, C23–H, N8–H, N15–H), 1.71–1.63 (m, 4H, C10–H, C17–H), 1.62–1.24 (m, 12H, C13–H, C14–H, C20–H, C21–H, C22–H), 1.04 (d, J = 6.3 Hz, 3H, C24–H). 13C NMR (100.6 MHz, CDCl3) δ (ppm): 138.6 (C2), 137.6 (C5), 128.6 (C3), 128.2 (C4), 57.7 (C11, C18), 55.9 (C23), 54.6 (C12), 53.7 (C1), 52.3 (C19), 51.1 (C7), 48.7 (C9), 48.3 (C16), 35.9 (C6), 34.6 (C22), 26.9 (C10), 26.1 (C14), 25.9 (C13), 25.7 (C17), 24.4 (C21), 19.0 (C24). MS (70 eV, EI): m/z (%) = 414.4 (8) [M]+, 260.2 (18) [M − C9H18N2]+, 155.1 (33) [C9H19N2]+, 126.1 (19) [C8H16N]+, 112.1 (87) [C7H14N]+, 98.2 (100) [C6H12N]+, 84.1 (16) [C5H10N]+. HRMS (EI): m/z calculated for C26H46N4 [M]+: 414.3722; Found: 414.33732.
N-(4-(2-(3-(2-Methylpiperidin-1-yl)propylamino)ethyl)benzyl)-3-(2-methylpiperidin-1-yl)propan-1-amine (8{3,3}).
As stated above for 8{4,4}, but using 0.2015 g (0.46 mmol) of 2-(4-((3-(2-methylpiperidin-1-yl)propylamino)methyl)phenyl)-N-(3-(2-methylpiperidin-1-yl)propyl)acetamide (17{3,3}), 0.259 mL (0.2075 g, 2.73 mmol) of the borane dimethylsulfide complex, 13 mL of THF and 6 mL of 1.25 M HCl/MeOH. Upon removal of the solvent, the residue was separated by automatic flash chromatography (basic alumina; 98
:
2
:
0.5; CH2Cl2/MeOH/NH3) to afford 0.088 g (0.205 mmol, 45%) of N-(4-(2-(3-(2-methylpiperidin-1-yl)propylamino)ethyl)benzyl)-3-(2-methylpiperidin-1-yl)propan-1-amine (8{3,3}). IR (KBr) ν (cm−1): 3280 (N–H), 2929, 2853, 2793 (Csp3–H), 1673 (N–H), 1449 (CH2). 1H NMR (400 MHz, CDCl3) δ (ppm): 7.27–7.22 (m, 2H, C3–H), 7.19–7.10 (m, 2H, C4–H), 3.76 (s, 2H, C1–H), 2.95–2.59 (m, 12H, C6–H, C7–H, C9–H, C19–H, C11–H, C12–H), 2.44–2.06 (m, 10H, C11–H, C12–H, C16–H, N8–H, N18–H), 1.73–1.22 (m, 16H, C10–H, C13–H, C14–H, C15–H, C20–H), 1.10–0.99 (m, 6H, C17–H). 13C NMR (100.6 MHz, CDCl3) δ (ppm) (major isomer): 138.5 (C2), 137.7 (C5), 128.7 (C3), 128.3 (C4), 55.9 (C16), 53.6 (C1), 52.2 (C11, C12), 51.1 (C7), 48.8 (C9), 48.3 (C19), 35.8 (C6), 34.5 (C15), 25.9 (C13), 25.5 (C10, C20), 23.8 (C14), 18.9 (C17); (minor isomer): 138.2 (C2), 138.0 (C5), 128.83 (C3), 128.48 (C4). MS (70 eV, EI): m/z (%) = 428.6 (12) [M]+, 288.1 (10) [M − C9H18N]+, 274.1 (22) [M − C9H18N2]+, 169.1 (36) [C10H21N2]+, 126.1 (25) [C8H16N]+, 112.1 (100) [C7H14N]+, 98.1 (69) [C6H12N]+. HRMS (EI): m/z calculated for C27H48N4 [M]+: 428.3879; Found: 428.3870.
Antiviral activity evaluation
HIV-1 strains were titered in MT-4 cells after acute infection, and infectivity was measured by evaluating the cytopathic effect induced after 5-day cultures as described.25,26 Anti-HIV activity (EC50) and cytotoxicity (CC50) measurements of MT-4 cells were based on the viability of cells that had been infected or not infected with HIV-1, all exposed to various concentrations of the test compound. After the MT-4 cells were allowed to proliferate for 5 days, the number of viable cells was quantified by a tetrazolium-based colorimetric method (MTT method).
Acknowledgements
Financial support by the Spanish Ministerio de Economia y Competividad project SAF2010-C21617-C02, BFU2012-31569 and FIS PI13/01083 is greatly acknowledged. R. Puig de la Bellacasa wants to thank Generalitat de Catalunya (AGAUR) for a FI scholarship. A. Gibert wants to thank IQS for a scholarship. R. Badía is a Sara Borrell fellow from FIS.
References
- G. A. Donzella, D. Schols, S. W. Lin, J. A. Esté, K. A. Nagashima, P. J. Maddon, G. P. Allaway, T. P. Sakmar, G. Henson, E. De Clercq and J. P. Moore, Nat. Med., 1998, 4, 72 CrossRef CAS PubMed.
-
(a) G. M. Keating, Drugs, 2011, 71, 1623 CrossRef CAS PubMed;
(b) G. Calandra, G. Bridger and S. Fricker, Curr. Top. Microbiol. Immunol., 2010, 341, 173 CAS;
(c)
http://pubchem.ncbi.nlm.nih.gov/compound/Plerixafor
.
- S. Hatse, K. Princen, E. De Clercq, M. M. Rosenkilde, T. W. Schwartz, P. E. Hernandez-Abad, R. T. Skerlj, G. J. Bridger and D. Schols, Biochem. Pharmacol., 2005, 70, 752 CrossRef CAS PubMed.
- K. Princen, S. Hatse, K. Vermeire, S. Aquaro, E. De Clercq, L. O. Gerlach, M. Rosenkilde, T. W. Schwartz, R. Skerlj, G. Bridger and D. Schols, J. Virol., 2004, 78, 12996 CrossRef CAS PubMed.
- N. D. Stone, S. B. Dunaway, C. Flexner, C. Tierney, G. B. Calandra, S. Becker, Y. J. Cao, I. P. Wiggins, J. Conley, R. T. MacFarland, J. G. Park, C. Lalama, S. Snyder, B. Kallungal, K. L. Klingman and C. W. Hendrix, Antimicrob. Agents Chemother., 2007, 51, 2351 CrossRef CAS PubMed.
-
(a) Y = CH: W. Zhan, Z. Liang, A. Zhu, S. Kurtkaya, H. Shim, J. P. Snyder and D. C. Liotta, J. Med. Chem., 2007, 50(23), 5655 CrossRef CAS PubMed;
(b) Y = N: Z. Liang, W. Zhan, A. Zhu, Y. Yoon, S. Lin, M. Sasaki, J. M. Klapproth, H. Yang, H. E. Grossniklaus, J. Xu, M. Rojas, R. J. Voll, M. M. Goodman, R. F. Arrendale, J. Liu, C. C. Yun, J. P. Snyder, D. C. Liotta and H. Shim, PLoS One, 2012, 7, e34038 CAS.
- C. H. Wu, C. P. Chang, J. S. Song, J. J. Jan, M. C. Chou, S. H. Wu, K. C. Yeh, Y. C. Wong, C. J. Hsieh, C. T. Chen, T. T. Kao, S. Y. Wu, C. F. Yeh, C. T. Tseng, Y. S. Chao and K. S. Shia, ChemMedChem, 2012, 7, 209 CrossRef CAS PubMed.
- J. A. Esté and A. Telenti, Lancet, 2007, 370, 81 CrossRef.
- B. A. Teicher and S. P. Fricker, Clin. Cancer Res., 2010, 16, 2927 CrossRef CAS PubMed.
- M. Terasaki, Y. Sugita, F. Arakawa, Y. Okada, K. Ohshima and M. Shigemori, Brain Tumor Pathol., 2011, 28, 89–97 CrossRef CAS PubMed.
-
(a) S. Pettersson, V. I. Pérez-Nueno, L. Ros-Blanco, R. Puig de La Bellacasa, M. O. Rabal, X. Batllori, B. Clotet, I. Clotet-Codina, M. Armand-Ugón, J. Esté, J. I. Borrell and J. Teixidó, ChemMedChem, 2008, 3, 1549 CrossRef CAS PubMed;
(b)
S. Pettersson, PhD Dissertation, Universitat Ramon Llull, Barcelona, 2009, pp. 250–251 (http://hdl.handle.net/10803/9298) Search PubMed;
(c)
L. Ros, PhD Dissertation, Universitat Ramon Llull, Barcelona, 2011, pp. 170–171 (http://hdl.handle.net/10803/31981) Search PubMed.
- S. Pettersson, V. I. Pérez-Nueno, M. P. Mena, B. Clotet, J. A. Esté, J. I. Borrell and J. Teixidó, ChemMedChem, 2010, 5, 1272 CrossRef CAS PubMed.
- V. I. Pérez-Nueno, D. W. Ritchie, O. Rabal, R. Pascual, J. I. Borrell and J. Teixidó, J. Chem. Inf. Model., 2008, 48, 509 CrossRef PubMed.
- L. Ros-Blanco, J. Anido, R. Bosser, J. Esté, B. Clotet, A. Kosoy, L. Ruíz-Ávila, J. Teixidó, J. Seoane and J. I. Borrell, J. Med. Chem., 2012, 55, 7560 CrossRef CAS PubMed.
- G. J. Bridger, R. T. Skerlj, D. Thornton, S. Padmanabhan, S. A. Martellucci, G. W. Henson, M. J. Abrams, N. Yamamoto, K. De Vreese, R. Pauwel and E. De Clercq, J. Med. Chem., 1995, 38, 366 CrossRef CAS PubMed.
-
W. Kuhn, Method for stabilising phenylacetaldehyde, PCT Int. Appl. WO, 2002046135, 2001 Search PubMed.
- C. Campà, F. Sanchez-Ferrando and M. Tristan-Polo, Nouv. J. Chim., 1985, 9, 493 Search PubMed.
- M. L. Falck-Pedersen and K. Undheim, Tetrahedron, 1996, 52, 7761 CrossRef CAS.
- J. M. Planesas, V. I. Pérez-Nueno, J. I. Borrell and J. Teixidó, J. Mol. Graphics Modell., 2012, 38, 123 CrossRef CAS PubMed.
- C. M. Venkatachalam, X. Jiang, T. Oldfield and M. Waldman, J. Mol. Graphics Modell., 2003, 21, 289 CrossRef CAS PubMed.
- M. Montes, M. A. Miteva and B. O. Villoutreix, Proteins: Struct., Funct., Bioinf., 2007, 68, 712 CrossRef CAS PubMed.
-
LigPrep, Version 2.4, Schrödinger, LLC, New York, NY, 2010 Search PubMed.
- A. S. Karaboga, J. M. Planesas, F. Petronin, J. Teixidó, M. Souchet and V. I. Pérez-Nueno, J. Chem. Inf. Model., 2013, 53, 1043 CrossRef CAS PubMed.
- P. R. Clapham and A. McKnight, Br. Med. Bull., 2001, 58, 43 CrossRef CAS PubMed.
- G. Moncunill, M. Armand-Ugón, E. Pauls, B. Clotet and J. A. Esté, AIDS, 2008, 22, 23 CrossRef CAS PubMed.
- M. Armand-Ugón, I. Clotet-Codina, C. Tintori, F. Manetti, B. Clotet, M. Botta and J. A. Esté, Virology, 2005, 343, 141 CrossRef PubMed.
Footnote |
† Electronic supplementary information (ESI) available. See DOI: 10.1039/c5ob02419f |
|
This journal is © The Royal Society of Chemistry 2016 |