Synthesis of two distinct pyrrole moiety-containing arenes from nitroanilines using Paal–Knorr followed by an indium-mediated reaction†
Received
9th October 2015
, Accepted 11th November 2015
First published on 12th November 2015
Abstract
Synthesis of arenes substituted with two differently substituted-pyrrole moieties was investigated. A Paal–Knorr condensation reaction of nitroanilines with 1,4-diketone to nitrophenyl-1H-pyrroles followed by an indium-mediated reduction-triggered coupling reaction with another kind of 1,4-diketone resulted in two distinct pyrrole-containing arenes, variously substituted 1-((1H-pyrrol-1-yl)phenyl)-1H-pyrroles, in reasonable yield.
Introduction
The pyrrole nucleus has received prominent attention as a key structural moiety because it is one of the most important heterocyclic units for medicinal and pharmaceutical chemistry, and pyrrole derivatives exhibit a broad spectrum of biological activity.1 Moreover, pyrrole has also been applied in various areas; polypyrroles have been applied as conducting polymers,2 while pyrrolic macrocycles, such as calix[4]pyrroles, have been used as anion receptors.3 As pyrrole derivatives are used in a wide-range of applications, developing more efficient synthetic methods is still one of the main issues. However, the Paal–Knorr condensation remains the most attractive and easiest method for the synthesis of pyrroles. Therefore, the use of 1,4-dicarbonyls has become one of the most versatile and widely applied methods among the many strategies that have been developed.4 In addition to the Paal–Knorr reaction, various synthetic methods have been developed to synthesize pyrroles, including Hantzsch synthesis,5 tandem reaction,6 rearrangement of o-vinyl oximes,7 [3 + 2] cycloaddition of 1,3-dipolar reagents with alkynes,8 hydroamination of diynes,9 and olefin cross-metathesis,10 to mention a few.11 Recently, we developed a new synthetic strategy of one-pot reductive condensation reactions using nitroarenes and 1,4-dicarbonyls that resulted in the efficient production of pyrroles,12 which was an extension of our various indium-mediated reductive heterocyclizations via the reductive cyclization reaction of nitroarenes to nitrogen-containing heterocycles.13 None of the known methods involve this kind of one-pot reductive condensation reactions starting from nitroarenes and 1,4-dicarbonyls to pyrroles. With the success of the new synthetic strategy, we could go one step further to a more challenging synthesis for new and useful organic materials, which could not be prepared by the Paal–Knorr reaction or any other method.
There have been few reports for the synthesis and applications of bis-pyrrole-arenes.14 Most of the known bis-pyrrole arenes consist of two identical pyrrole rings, undoubtedly because synthetic methods are not well developed other than the Paal–Knorr reaction of diaminobenzenes with 1,4-diketones. In addition to bis-pyrrole arene derivatives, there are structurally similar terphenyl compounds, some of which are used as organic scintillation materials for fast neutron detection in nuclear physics, medical, and other basic sciences.15para-Terphenyl, 1,4-diphenylbenzene is a representative organic scintillator being used for scintillation detectors.16 However, the number of scintillators which have proven practical is small because of the lack of useful, cheap, and large volume synthetic methods.
With successful reductive condensation reactions of nitroarenes and 1,4-dicarbonyls toward pyrroles,12 we became interested in the synthesis of para-terphenyl type heterocyclic compounds, ((1H-pyrrol-1-yl)phenyl)-1H-pyrroles, which could potentially be useful organic materials as biologically active compounds and/or organic scintillation materials. Only a few of ((1H-pyrrol-1-yl)phenyl)-1H-pyrroles are known because there are no production methods available other than the Paal–Knorr reaction starting from diaminoarenes.16 However, if nitroanilines are used as substrates for the Paal–Knorr type condensation reaction, first with 1,4-dicarbonyls followed by our newly developed indium reduction-triggered intermolecular heterocyclization with other 1,4-dicarbonyls that are different from the first 1,4-dicarbonyls structurally, plenty of unsymmetrical bis-pyrrole arene derivatives, which are mostly unknown compounds, could be easily synthesized. In addition, nitroanilines are less expensive than diaminobenzenes or dinitrobenzenes, which will be one of the additional merits to access the reactions in the field of process chemistry.
In this study we report the development of a new synthetic strategy to terphenyl type heterocyclic compounds using the Paal–Knorr condensation reaction followed by indium reduction-triggered intermolecular heterocyclization starting from nitroanilines with 1,4-dicarbonyls that could produce two distinct pyrrole moiety-containing arenes, with an aim to increasing the number of potential organic scintillators.
Results and discussion
Prior to trying to develop the reaction of nitroanilines to produce two distinct pyrrole moiety-containing arenes, we examined the reductive coupling reactions of dinitrobenzenes with 1,4-diketones to produce a bis-pyrrole product using indium reduction-triggered intermolecular heterocyclization that was developed by us for one-pot pyrrole synthesis from nitroarenes;12i.e., dinitrobenzene (1 equiv.)/1,4-diketone (2 equiv.)/indium (8 equiv.)/acetic acid (20 equiv.) in toluene (20 mL). In those reactions, the corresponding bis-pyrrole-arene product was obtained in reasonable yield except for 1,2-dinitrobenzene (Table 1). In the case of 1,2-dinitrobenzene, unexpectedly, 2-(2,5-dimethyl-1H-pyrrol-1-yl)aniline was obtained as a major product after 2 h (68%) at 80 °C without the desired 1,1′-(1,3-phenylene)bis[2,5-dimethyl-1H-pyrrole]. Even with a prolonged reaction time of up to 24 h, the 1,1′-(1,3-phenylene)bis[2,5-dimethyl-1H-pyrrole] (1) was only obtained in 5% yield and 2-(2,5-dimethyl-1H-pyrrol-1-yl)benzenamine (1′) remained as the major product (58%) (entry 1, Table 1). At reflux for 3 h, the yield of 1 was improved to 15%, however, 1′ was the major product yet again (50%). It is believed that too much closely congested bis-pyrrole product formation may prevent the second pyrrole ring formation from the reduced amino intermediate with 1,4-diketone and leave an amino group instead of one more heterocyclization. As proposed in our previous paper for pyrrole ring formation,12 dinitrobenzene would be transformed into a diaminobenzene intermediate, which would couple with 1,4-diketone to make a bis-pyrrole product except 1,2-dinitrobenzene. For the diketone part, phenyl-substituted diketones such as 1-phenyl-1,4-pentanedione (entry 4, Table 1) or 1,4-diphenyl-1,4-butanedione (entry 5, Table 1) was less reactive than 2,5-hexanedione (entries 1–3, Table 1) and required higher reaction temperature. As the use of 1,4-diphenyl-1,4-butanedione showed poor results as 1,2-dinitrobenzene, both may not be advantageous for synthetic application. Overall, except the reaction of sterically congested 1,2-dinitrobenzene or 1,4-diphenyl-1,4-butanedione, our protocol could be used as an alternative method for the preparation of two identical pyrrole-substituted bis-pyrrole-arenes in addition to other well-known methods starting from diaminobenzenes.
Table 1 Indium-mediated reductive heterocyclization of dinitrobenzene (1 mmol) with a 1,4-diketone compound (2 equiv.) in the presence of indium (8 equiv.)/acetic acid (20 equiv.) toward ((1H-pyrrol-1-yl)phenyl)-1H-pyrroles in toluene (20 mL)
In addition to dinitroarene substrates, nitroaniline is a good alternative candidate as a starting substrate for the synthesis of bis-pyrrole-arene derivatives since nitroaniline would be transformed into diaminoarene by an in situ indium-mediated reductive reaction that is an intermediate for the follow-up heterocyclization with 1,4-diketone in the reaction medium. Therefore, we examined several indium-mediated reductive reaction triggered heterocyclizations of 4-nitroaniline with representative 1,4-diketones that were used for the reductive coupling reactions of dinitrobenzenes and summarized in Table 2. Those reactions of 4-nitroaniline with 1,4-diketones in the presence of indium (4 equiv.) and acetic acid (10 equiv.) in toluene presented a similar trend to the experiments that were done with dinitrobenzenes with 1,4-diketones. Again, the reaction using 1,4-diphenyl-1,4-butanedione was not very successful and produced a low yield contaminated with other by-products (entry 3, Table 2) while reactions using 2,5-hexanedione (entry 1) or 1-phenyl-1,4-hexanedione (entry 2) produced the desired bis-pyrrole product even with improved yield compared to reactions of dinitrobenzenes with 1,4-diketones. Those results gave us a useful clue for developing the synthesis of two distinct pyrrole moiety-containing arene derivatives.
Table 2 Indium-acetic acid-mediated reductive heterocyclization of nitroanilines (1 mmol) with a 1,4-diketone (2 equiv.) in the presence of indium (4 equiv.) and acetic acid (10 equiv.) in toluene (5 mL)
Nitroaniline substrates can be used not only for the preparation of two identical pyrrole-substituted symmetrical bis-pyrrole arenes, but also for the preparation of its unsymmetrical analogs, in other words, two distinguishable pyrrole containing terphenyl type heterocyclic compounds with a simple synthetic strategy. Thus, we designed the two step synthesis with a combination of the Paal–Knorr reaction followed by an indium-mediated heterocyclization reaction starting from nitroanilines for the synthesis of more diverse bis-pyrrole substituted arenes. By applying the Paal–Knorr condensation reaction to nitroanilines with 1,4-diketones, the amino group of nitroanilines can be transformed into a pyrrole moiety with an unreacted nitro group. Then indium-mediated heterocyclization reaction of this intermediate in the presence of another 1,4-diketone can introduce an additional pyrrole moiety onto the nitro site of the intermediate, which resulted in an unsymmetrical bis-pyrrole-arene molecule if the 1,4-diketones for each step are not identical. With this two-step synthesis, many new bis-pyrrole-arenes can be synthesized, which were not known because of the lack of applicable synthetic methods.
First, we tried a one-batch reaction with stepwise adoption of two different reaction conditions, i.e., the Paal–Knorr and indium-mediated reactions, without isolation of the intermediate after the Paal–Knorr reaction since both the Paal–Knorr and indium-mediated reactions can be performed in the same solvent and under comparable acidic conditions. Thus, the Paal–Knorr reaction of nitroaniline (1 mmol) with 2,5-hexanedione (1 equiv.) in the presence of a catalytic amount of p-TsOH·H2O (0.02 equiv.) in toluene at reflux was performed for 1.5 h. For the dehydration of the reaction medium, the reaction was performed with an excess amount of MgSO4 (2–3 mmol) in the beginning. After cooling down the reaction mixture, indium (4 equiv.), acetic acid (10 equiv.) and 1-phenyl-1,4-pentanedione were added to the reaction mixture directly and refluxed again for 4 h. The reaction went well with a good yield (74%, NMR yield with an internal standard, 1,3,5-trimethoxybenzene), however, flash column chromatography purification from the small amount of undesired symmetrically substituted bis-pyrrole-benzene derivatives such as 2,5-dimethyl-1-(3-(2,5-dimethyl-1H-pyrrol-1-yl)phenyl)-1H-pyrrole (3, ∼3%) and 1,1′-p-phenylenebis[2-methyl-5-phenylpyrrole] (4, ∼3%) was a problem because of Rf value similarity to the desired product, 2,5-dimethyl-1-(4-(2-methyl-5-phenyl-1H-pyrrol-1-yl)phenyl)-1H-pyrrole. Accordingly, we were obliged to change our strategy to the two step synthesis. Consequently, after completion of the Paal–Knorr reaction of nitroaniline under the above-mentioned conditions, the reaction mixture was worked up and purified with conventional methods. The isolated intermediate, 2,5-dimethyl-1-(3-nitrophenyl)-1H-pyrrole (6) (97% yield, Table 3, entry 1) was then reacted with 1-phenyl-1,4-pentanedione (1 equiv.) in the presence of indium (4 equiv.) and acetic acid (10 equiv.) in toluene at reflux for 4 h. The desired product, 2,5-dimethyl-1-(4-(2-methyl-5-phenyl-1H-pyrrol-1-yl)phenyl)-1H-pyrrole, was obtained in 92% yield without any problems. Technically the stepwise two step synthesis is better for purification than the one-batch reaction so the two step reaction was chosen for the two different pyrrole-substituted bis-pyrrole-arene derivative syntheses.
Table 3 Paal–Knorr reaction of nitroanilines (1 mmol) with 1,4-diketones (1 mmol) toward variously substituted (1H-pyrrol-1-yl)nitroarenes under toluene (2.5 mL) reflux reaction conditionsa
Thus, the Paal–Knorr reaction of variously substituted nitroanilines (1 equiv.) with 1,4-diketones (1 equiv.) in the presence of a catalytic amount of p-TsOH·H2O (0.02 equiv.) with a dehydrating reagent (MgSO4 (2–3 equiv.)) was carried out under toluene reflux to obtain diverse (1H-pyrrol-1-yl)nitroarenes. By applying this Paal–Knorr reaction, fourteen pyrrole-ring substituted nitrobenzene derivatives were prepared in high yields (Table 3). As the nitro group can be transformed into a pyrrole ring by our indium-mediated reductive cyclization reaction, these derivatives can be useful intermediates for the synthesis of two different interesting pyrrole-substituted bis-pyrrolearenes.
With the (1H-pyrrol-1-yl)nitroarene derivatives prepared, indium-mediated heterocyclizations for another pyrrole ring construction with 1,4-diketones, which are different from the first 1,4-diketones of the Paal–Knorr reaction, were completed in the presence of indium (4 equiv.) and acetic acid (10 equiv.) in toluene at reflux (1-phenyl-1,4-pentanedione) or at 80 °C (2,5-hexanedione) (Table 4). In most cases, heterocyclization for the second pyrrole ring formation was successful with reasonably good yield (70–92%). While the Paal–Knorr reactions of variously substituted nitroanilines with 1,4-diketones were not affected by the electronic effect of the substitution, reductive heterocyclization reactions of the substituted (1H-pyrrol-1-yl)nitroarene derivatives seemed to be affected by the substitution. The strong inductive effect of fluoro-substitution of the substrate slowed the reaction time and/or product yield (Table 4, entries 6, 7, 13, 14), which indicates that the inductive effect may decrease the reactivity of the indium-mediated reductive reaction of the nitro group to the amino intermediate prior to heterocyclization reactions with 1,4-diketones.
Table 4 Indium-mediated heterocyclization of variously substituted (1H-pyrrol-1-yl)nitroarenes (1 mmol) with 1,4-diketone(1 equiv.) in the presence of indium (4 equiv.) and acetic acid (10 equiv.) in toluene (5 mL) at reflux (1-phenyl-1,4-pentanedione) or at 80 °C (2,5-hexanedione)
Since the shape of terphenyl type bis-pyrrole-arene compounds is not well-documented and would be an important clue for its application, the exact structure of a representative compound, 2,5-dimethyl-1-[4-(2-methyl-5-phenyl-1H-pyrrol-1-yl)phenyl]-1H-pyrrole (20), was elucidated by X-ray crystallography.17 The molecular structure with an atom-labeling scheme is shown in Fig. 1. The ORTEP plot of molecule 20 displays its structure, in which the 2-methyl-5-phenyl-substituted pyrrolyl ring (A) is twisted out of the plane of the central aryl ring (B) by a dihedral angle of 72.11° and the 2,5-dimethyl-substituted pyrrolyl ring (C) is twisted out of the plane of the central aryl ring (B) by a dihedral angle of −68.55° on its substituent. The dihedral angles of the two pyrrole rings may change to some extent if there is an additional substituent on the aryl ring or the two pyrrole rings are at different positions, like the para- or meta-position, and will result in slight physical property changes, which will be of interest to material chemists for application.
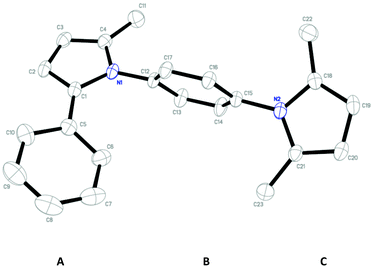 |
| Fig. 1 Molecular structure of 2,5-dimethyl-1-[4-(2-methyl-5-phenyl-1H-pyrrol-1-yl)phenyl]-1H-pyrrole (20) with an atom-labeling scheme. | |
Conclusions
In conclusion, we developed a simple method for the synthesis of terphenyl type heterocyclic compounds, arene derivatives substituted with two different pyrrole moieties in a molecule, using the Paal–Knorr condensation reaction of nitroanilines with 1,4-dicarbonyls toward (1H-pyrrol-1-yl)nitroarenes followed by indium reduction-triggered intermolecular heterocyclization of the nitro group with another kind of 1,4-dicarbonyls that could produce two distinct pyrrole moiety-containing arenes. With this unique method many ((1H-pyrrol-1-yl)phenyl)-1H-pyrrole derivatives, which up until now were mostly unknown compounds because of the lack of synthetic methods or strategies for production, could be synthesized with ease.
Experimental
General considerations
Most chemical reagents were purchased from Sigma-Aldrich Co. (St. Louis, Missouri, USA) and were used without further purification. Solvents were purchased and dried using standard methods. 1H and 13C NMR spectra were recorded at 400 and 100 MHz, respectively (JEOL, Tokyo, Japan). Chemical shifts are reported in parts per million relative to the residual solvent as an internal standard or a TMS. GC-MS spectra were recorded on an Agilent 6890N GC connected to an Agilent 5975 mass selective detector (Hewlett-Packard Co., Palo Alto, California, USA). Infrared (IR) spectra were recorded using an MB104 FTIR (ABB Bomem, Inc., Zurich, Switzerland). The elemental analysis data were obtained by using a Thermo Scientific Flash 2000 (Thermo Fisher Scientific, USA). Melting points were determined on an electrothermal apparatus and were uncorrected. All the major products were isolated by flash column chromatography on silica gel (230–400 mesh ATSM, Merck & Co., Inc., Whitehouse Station, New Jersey, USA) using a mixed solvent eluent (ethyl acetate/hexane). Analytical thin-layer chromatography (TLC) was performed using a 0.25 mm silica gel 60F254 precoated-glass plate. For single-crystal X-ray structure determinations, data were collected at 50 kV, 30 mA using a Bruker SMART Apex II X-ray Diffractometer (Bruker Nano Analytics, Berlin, Germany) equipped with a Mo tube, a graphite-monochromator, and a CCD area-detector. Bruker SHELXTL software was used for structure analysis.
General procedures
General procedure for the indium-mediated reaction of dinitrobenzenes with 1,4-diketones to obtain ((1H-pyrrol-1-yl)phenyl)-1H-pyrroles.
The dinitrobenzene derivative (1.0 mmol) and indium powder (920 mg, 8.0 mmol) were placed in toluene (20 mL), and were added acetic acid (1.144 mL, 20 mmol) and 1,4-diketone (2.0 mmol) in toluene (20 mL) to the mixture. The reaction mixture was stirred at 80 °C (2,5-hexanedione) or at reflux (1-phenyl-1,4-pentanedione or 1,4-diphenyl-1,4-butanedione) under nitrogen. After the reaction was completed, the reaction mixture was diluted with ethyl acetate (30 mL) or dichloromethane (30 mL), filtered through Celite, poured into 10% aqueous NaHCO3 solution (30 mL), and then the aqueous layer was extracted with ethyl acetate (30 mL × 3) or dichloromethane (30 mL × 3). The combined organic extracts were dried over MgSO4, filtered, and concentrated. The residue was eluted with ethyl acetate/hexane (v/v = 5/95) through a neutral silica gel column to give the corresponding pyrroles. The structures of the pyrroles were characterized by 1H NMR, 13C NMR, FTIR, and GC-MS and were mostly known compounds. For unknown compounds, HRMS data were additionally reported.
2,5-Dimethyl-1-(2-(2,5-dimethyl-1H-pyrrol-1-yl)phenyl)-1H-pyrrole (1)18,19.
Yield 5%. White solid, mp 148–150 °C (lit.18 mp 132 °C). TLC (30% ethyl acetate/hexane) Rf 0.80; 1H NMR (400 MHz, CDCl3) δ 7.42–7.37 (m, 2H), 7.25–7.18 (m, 2H), 5.75 (s, 4H), 1.81 (s, 12H); 13C NMR (100 MHz, CDCl3) δ 135.6, 131.1, 129.0, 127.8, 106.9, 12.9; IR (NaCl) 3114, 3047, 2927, 2854, 1581, 1450, 1392 cm−1; GC-MS m/z (rel intensity) 264 (M+, 93), 249 (100), 233 (17), 296 (14), 168 (12), 154 (10).
2-(2,5-Dimethyl-1H-pyrrol-1-yl)benzenamine (1′)18.
Yield 58%. Pale yellow solid, mp 63–64 °C (lit.18 mp 75 °C). TLC (30% ethyl acetate/hexane) Rf 0.67; 1H NMR (400 MHz, CDCl3) δ 7.20 (td, 1H, J = 7.8, 1.5 Hz), 7.05 (dd, 1H, J = 7.8, 1.5 Hz), 6.81–6.78 (m, 2H), 5.93 (s, 2H), 3.43 (s, 2H), 1.98 (s, 6H); 13C NMR (100 MHz, CDCl3) δ 144.1, 129.32, 129.28, 128.4, 124.4, 118.2, 115.5, 105.8, 12.3; IR (NaCl) 3476, 3367, 3101, 2920, 1616, 1504, 1461, 1400 cm−1; GC-MS m/z (rel intensity) 186 (M+, 63), 171 (100), 156 (10), 144 (9), 92 (11), 65 (13), 51 (11).
2,5-Dimethyl-1-(3-(2,5-dimethyl-1H-pyrrol-1-yl)phenyl)-1H-pyrrole (2)19.
Yield 70%. White solid, TLC (30% ethyl acetate/hexane) Rf 0.80; mp 102–103 °C (lit.19a mp 99–100 °C); 1H NMR (400 MHz, CDCl3) δ 7.55 (t, 1H, J = 7.9 Hz), 7.26 (dd, 2H, J = 7.9, 1.8 Hz), 7.09 (t, 1H, J = 1.8 Hz), 5.91 (s, 4H), 2.07 (s, 12H); 13C NMR (100 MHz, CDCl3) δ 139.6, 129.4, 128.6, 128.0, 127.4, 106.1, 13.0; IR (NaCl) 3105, 3055, 2923, 1600, 1523, 1492, 1454, 1396, 1311 cm−1; GC-MS m/z (rel intensity) 264 (M+, 100), 249 (20), 233 (9), 168 (10), 154 (12), 131 (16).
2,5-Dimethyl-1-(4-(2,5-dimethyl-1H-pyrrol-1-yl)phenyl)-1H-pyrrole (3)19.
Yield 62%. White solid, TLC (20% ethyl acetate/hexane) Rf 0.60; mp 250–251 °C (lit.19a mp 256–257 °C); 1H NMR (400 MHz, CDCl3) δ 7.29 (s, 4H), 5.93 (s, 4H), 2.08 (s, 12H); 13C NMR (100 MHz, CDCl3) δ 138.2, 128.78, 128.77, 106.0, 13.0; IR (KBr) 3109, 3033, 2987, 2923, 1515, 1406 cm−1; GC-MS m/z (rel intensity) 264 (M+, 100), 249 (10), 233 (5), 168 (10), 154 (15), 131 (20).
1,1′-p-Phenylenebis[2-methyl-5-phenylpyrrole] (4).
Yield 57%. White solid, TLC (20% ethyl acetate/hexane) Rf 0.78; mp 237–238 °C; 1H NMR (400 MHz, CDCl3) δ 7.19–7.05 (m, 14H), 6.36 (d, 2H, J = 3.2 Hz), 6.10 (d, 2H, J = 3.2 Hz), 2.15 (s, 6H); 13C NMR (100 MHz, CDCl3) δ 138.4, 134.1, 133.2, 131.5, 128.9, 127.9, 127.7, 125.8, 108.9, 107.8, 13.2; IR (NaCl) 3051, 2974, 1596, 1512, 1446, 1392, 1216 cm−1; GC-MS m/z (rel intensity) 388 (M+, 100), 373 (5), 230 (5), 194 (11), 115 (5); HRMS m/z calc. for C28H24N2 388.1939, found 388.1932.
1,1′-p-Phenylenebis[2,5-diphenylpyrrole] (5).
Yield 19%. White solid, TLC (30% ethyl acetate/hexane) Rf 0.73; mp 310–311 °C; 1H NMR (400 MHz, DMSO-d6) δ 7.20–7.16 (m, 12H), 7.08–7.04 (m, 8H), 6.99 (bd s, 4H), 6.42 (bd s, 4H); 13C NMR (100 MHz, CDCl3) δ 137.8, 135.8, 133.0, 129.2, 128.7, 127.9, 126.3, 110.2; IR (KBr) 3066, 1600, 1515, 1485, 1388, 1330 cm−1; HRMS m/z calc. for C38H28N2 512.2252, found 512.2247.
General procedure for the indium-mediated reaction of nitroanilines with 2,5-hexanedione or 1-phenyl-1,4-pantanedione to obtain ((1H-pyrrol-1-yl)phenyl)-1H-pyrroles.
The nitroaniline derivative (1.0 mmol) and indium powder (460 mg, 4.0 mmol) in toluene (1 mL) were placed, and were added 1,4-diketone (1.0 mmol) and acetic acid (0.572 mL, 10 mmol) in toluene (4 mL) to the mixture. The reaction mixture was stirred at 80 °C or refluxed under nitrogen. After the reaction was completed, the reaction mixture was diluted with ethyl acetate (30 mL) or dichloromethane (30 mL), filtered through Celite, poured into 10% NaHCO3 (30 mL), and then the aqueous layer was extracted with ethyl acetate (30 mL × 3) or dichloromethane (30 mL × 3). The combined organic extracts were dried over MgSO4, filtered, and concentrated. The residue was eluted with ethyl acetate/hexane (v/v = 5/95) through a silica gel column to give the corresponding pyrroles (3, 87%; 4, 75%; 5, 23%).
General procedure for the Paal–Knorr reaction of nitroanilines with 2,5-hexanedione or 1-phenyl-1,4-pantanedione to obtain (1H-pyrrol-1-yl)nitroarenes.
The nitroaniline derivative (1.0 mmol) was added to a mixture of a catalytic amount of p-TsOH·H2O (0.02 equiv.) and MgSO4 (2–3 equiv.) in toluene (1.5 mL), followed by the addition of 2,5-hexanedione or 1-phenyl-1,4-pentanedione (1.0 mmol) in toluene (1 mL). The reaction mixture was stirred at reflux under a nitrogen atmosphere. After the reaction was completed, the reaction mixture was diluted with ethyl acetate (30 mL) and was poured into 10% aqueous NaHCO3 solution. Then the aqueous layer was extracted with ethyl acetate (30 mL × 3) or dichloromethane (30 mL × 3). The combined organic extracts were dried over MgSO4, filtered, and concentrated. The residue was eluted with hexane for most derivatives or ethyl acetate/hexane (v/v = 5/95) for pyrrole-ring substituted nitrobenzene derivatives through a neutral silica gel column to give the corresponding pyrroles. The structures of the pyrroles were characterized by 1H NMR, 13C NMR, FTIR, and GC-MS. For unknown compounds, HRMS data were additionally reported.
2,5-Dimethyl-1-(4-nitrophenyl)-1H-pyrrole (6)20,21.
Yield 97%. Yellow solid, mp 131–132 °C (lit.20 mp 145 °C; lit.21 mp 125–129 °C). TLC (30% ethyl acetate/hexane) Rf 0.78; 1H NMR (400 MHz, CDCl3) δ 8.35 (d, 1H, J = 9.0 Hz), 7.39 (d, 1H, J = 9.0 Hz), 5.96 (s, 1H), 2.07 (s, 3H); 13C NMR (100 MHz, CDCl3) δ 146.8, 144.8, 128.8, 128.5, 124.5, 107.4, 13.0; IR (NaCl) 3105, 3074, 2923, 2854, 1593, 1519, 1492, 1396, 1338 cm−1; GC-MS m/z (rel intensity) 216 (M+, 100), 201 (5), 169 (46), 154 (30), 128 (9), 115 (5), 76 (1), 50 (11).
2,5-Dimethyl-1-(3-nitrophenyl)-1H-pyrrole (7)22.
Yield 93%. Yellow solid, mp 84–85 °C (lit.22 mp 84–85 °C). TLC (30% ethyl acetate/hexane) Rf 0.56; 1H NMR (400 MHz, CDCl3) δ 8.22–8.18 (m, 1H), 8.04 (t, 1H, J = 1.8 Hz), 7.60 (t, 1H, J = 8.0 Hz), 7.52–7.48 (m, 1H), 5.87 (s, 2H), 1.98 (s, 6H); 13C NMR (100 MHz, CDCl3) δ 148.6, 140.1, 134.2, 129.9, 128.6, 123.2, 122.4, 106.9, 13.0; IR (NaCl) 3097, 2927, 2854, 1535, 1485, 1350 cm−1; GC-MS m/z (rel intensity) 216 (M+, 100), 201 (5), 169 (30), 154 (33), 128 (8), 77 (8).
2,5-Dimethyl-1-(2-methyl-4-nitrophenyl)-1H-pyrrole (8)21.
Yield 99%. Yellow solid, mp 51–52 °C (lit.21 mp 101–103 °C). TLC (30% ethyl acetate/hexane) Rf 0.78; 1H NMR (400 MHz, CDCl3) δ 8.15 (d, 1H, J = 2.0 Hz). 8.09 (dd, 1H, J = 8.5, 2.0 Hz), 7.27 (d, 1H, J = 8.5 Hz), 5.88 (s, 2H), 1.98 (s, 3H), 1.84 (s, 6H); 13C NMR (100 MHz, CDCl3) δ 147.5, 144.2, 139.3, 130.0, 127.8, 125.7, 121.9, 106.5, 17.2, 12.4; IR (NaCl) 3105, 2923, 1589, 1527, 1492, 1350 cm−1; GC-MS m/z (rel intensity) 230 (M+, 100), 215 (63), 198 (8), 183 (32), 169 (52), 154 (30); HRMS m/z calc. for C13H14N2O2 230.1055, found 230.1035.
2,5-Dimethyl-1-(2-methyl-5-nitrophenyl)-1H-pyrrole (9)22.
Yield 96%. Yellow solid, mp 103–104 °C (lit.22 mp 103–104 °C). TLC (30% ethyl acetate/hexane) Rf 0.85; 1H NMR (400 MHz, CDCl3) δ 8.22 (dd, 1H, J = 8.5, 2.3 Hz), 8.08 (d, 1H, J = 2.3 Hz), 7.51 (d, 1H, J = 8.5 Hz), 5.95 (s, 2H), 2.05 (s, 3H), 1.92 (s, 6H); 7.49 (d, 1H, J = 8.3 Hz), 5.95 (s, 2H), 2.05 (s, 3H), 1.91 (s, 6H); 13C NMR (100 MHz, CDCl3) δ 146.8, 145.5, 139.2, 131.3, 127.9, 124.2, 123.3, 106.5, 17.3, 12.4; IR (NaCl) 3109, 2985, 2912, 1589, 1523, 1492, 1342 cm−1; GC-MS m/z (rel intensity) 230 (M+, 100), 215 (56), 198 (14), 183 (24), 169 (40), 154 (26).
1-(2-Methoxy-4-nitrophenyl)-2,5-dimethyl-1H-pyrrole (10)21.
Yield 94%. Yellow solid, mp 99–100 °C (lit.21 mp 68–70 °C). TLC (30% ethyl acetate/hexane) Rf 0.75; 1H NMR (400 MHz, CDCl3) δ 7.95 (dd, 1H, J = 8.5, 2.2 Hz), 7.91 (d, 1H, J = 2.2 Hz), 7.33 (d, 1H, J = 8.5 Hz), 5.95 (s, 2H), 2.93 (s, 3H), 1.97 (s, 6H); 13C NMR (100 MHz, CDCl3) δ 156.2, 148.2, 133.6, 130.6, 128.9, 115.9, 107.1, 106.3, 56.3, 12.4; IR (NaCl) 3109, 2927, 2854, 1589, 1527, 1458, 1346, 1235 cm−1; GC-MS m/z (rel intensity) 246 (M+, 100), 231 (54), 215 (8), 199 (27), 185 (21), 154 (14); HRMS m/z calc. for C13H14N2O3 246.1004, found 246.1009.
1-(2-Fluoro-4-nitrophenyl)-2,5-dimethyl-1H-pyrrole (11).
Yield 87%. Yellow solid, mp 78–79 °C. TLC (30% ethyl acetate/hexane) Rf 0.79; 1H NMR (400 MHz, CDCl3) δ 8.19–8.13 (m, 2H), 7.48–7.43 (m, 1H), 5.98 (s, 2H), 2.04 (s, 6H); 13C NMR (100 MHz, CDCl3) δ 157.7 (d, J = 255.7 Hz), 147.7 (d, J = 7.4 Hz), 133.0 (d, J = 13.2 Hz), 131.1, 128.9, 119.7 (d, J = 3.3 Hz), 112.7 (d, J = 25.7 Hz), 107.5, 12.4 (d, J = 1.7 Hz); IR (NaCl) 3058, 2989, 1608, 1539, 1500, 1454, 1350, 1218 cm−1; GC-MS m/z (rel intensity) 234 (M+, 93), 204 (100), 187 (65), 172 (22), 148 (31), 109 (18); HRMS m/z calc. for C12H11FN2O2 234.0805, found 234.0788.
1-(4-Fluoro-3-nitrophenyl)-2,5-dimethyl-1H-pyrrole (12).
Yield 94%. Yellow solid, mp 107–108 °C. TLC (30% ethyl acetate/hexane) Rf 0.75; 1H NMR (400 MHz, CDCl3) δ 7.95 (dd, 1H, J = 6.6, 2.6 Hz), 7.53–7.48 (m, 1H), 7.45–7.37 (m, 1H), 5.93 (s, 2H), 2.05 (s, 6H); 13C NMR (100 MHz, CDCl3) δ 154.4 (d, J = 265.7 Hz), 135.4 (d, J = 4.1 Hz), 135.2 (d, J = 8.3 Hz), 128.6, 125.6 (d, J = 2.5 Hz), 119.1 (d, J = 21.5 Hz), 119.0, 107.0, 12.9; IR (NaCl) 3082, 2923, 1593, 1542, 1446, 1353 cm−1; GC-MS m/z (rel intensity) 234 (M+, 100), 188 (55), 172 (28), 94 (16), 51 (12); HRMS m/z calc. for C12H11FN2O2 234.0805, found 234.0789.
2-Methyl-1-(4-nitrophenyl)-5-phenyl-1H-pyrrole (13).
Yield 93%. Yellow solid, mp 102–103 °C. TLC (30% ethyl acetate/hexane) Rf 0.71; 1H NMR (400 MHz, CDCl3) δ 8.22 (d, 2H, J = 8.8 Hz), 7.29 (d, 2H, J = 8.8 Hz), 7.18–7.13 (m, 3H), 7.02 (d, 2H, J = 7.1 Hz), 6.37 (d, 1H J = 3.4 Hz), 6.14 (d, 1H, J = 3.4 Hz), 2.20 (s, 3H); 13C NMR (100 MHz, CDCl3) δ 146.4, 145.1, 134.4, 132.8, 131.3, 128.9, 128.2, 128.0, 126.3, 124.3, 110.2, 109.1, 13.4; IR (NaCl) 3073, 2923, 1596, 1519, 1496, 1346 cm−1; GC-MS m/z (rel intensity) 278 (M+, 100), 232 (84), 217 (29), 204 (14), 189 (13), 115 (17), 76 (11); HRMS m/z calc. for C17H14N2O2 278.1055, found 278.1042.
2-Methyl-1-(3-nitrophenyl)-5-phenyl-1H-pyrrole (14).
Yield 96%. Yellow solid, mp 91–92 °C. TLC (20% ethyl acetate/hexane) Rf 0.70; 1H NMR (400 MHz, CDCl3) δ 8.18 (dt, 1H, J = 8.0, 1.3 Hz), 8.07 (t, 1H, J = 1.3 Hz), 7.51 (t, 1H, J = 8.0 Hz), 7.42 (dt, 1H, J = 8.0, 1.3 Hz), 7.18–7.09 (m, 3H), 7.02 (dd, 2H, J = 8.0, 1.2 Hz), 6.37 (d, 1H, J = 3.4 Hz), 6.14 (d, 1H, J = 3.4 Hz), 2.18 (3H, s); 13C NMR (100 MHz, CDCl3) δ 148.4, 140.5, 134.5, 134.4, 132.7, 131.4, 129.7, 128.2, 128.0, 126.2, 123.1, 122.1, 109.7, 108.6, 13.3; IR (NaCl) 3089, 2923, 1600, 1535, 1481, 1353 cm−1; GC-MS m/z (rel intensity) 278 (M+, 100), 232 (51), 217 (55), 189 (11), 154 (9), 115 (18), 76 (12); HRMS m/z calc. for C17H14N2O2 278.1055, found 278.1038.
2-Methyl-1-(2-methyl-4-nitrophenyl)-5-phenyl-1H-pyrrole (15).
Yield 95%. Yellow solid, mp 94–95 °C. TLC (30% ethyl acetate/hexane) Rf 0.73; 1H NMR (400 MHz, CDCl3) δ 8.13 (dd, 1H, J = 8.4, 2.4 Hz), 8.09 (d, 1H, J = 2.4 Hz), 7.45 (d, 1H, J = 8.5 Hz), 7.14–7.09 (m, 3H), 7.03 (d, 2H, J = 7.6 Hz), 6.40 (d, 1H, J = 3.4 Hz), 6.15 (d, 1H, J = 3.4 Hz), 2.01 (s, 3H), 1.94 (s, 3H); 13C NMR (100 MHz, CDCl3) δ 147.2, 144.7, 139.0, 134.3, 132.9, 130.7, 130.3, 128.2, 127.0, 126.2, 125.8, 121.7, 109.0, 108.4, 17.5, 12.7; IR (NaCl) 3074, 2923, 1600, 1527, 1446, 1350 cm−1; GC-MS m/z (rel intensity) 292 (M+, 100), 277 (16), 246 (15), 230 (22), 115 (9), 89 (7); HRMS m/z calc. for C18H16N2O2 292.1212, found 292.1248.
2-Methyl-1-(6-methyl-3-nitrophenyl)-5-phenyl-1H-pyrrole (16).
Yield 91%. Yellow solid, mp 134–135 °C. TLC (30% ethyl acetate/hexane) Rf 0.75; 1H NMR (400 MHz, CDCl3) δ 8.21 (d, 1H, J = 2.2 Hz), 8.15 (dd, 1H, J = 8.3, 2.2 Hz), 7.36 (d, 1H, J = 8.3 Hz), 7.16–7.08 (m, 3H), 7.06–7.02 (m, 2H) 6.39 (s, 1H, J = 3.4 Hz), 6.15 (s, 1H, J = 3.4 Hz), 2.02 (s, 3H), 1.92 (s, 3H); 13C NMR (100 MHz, CDCl3) δ 146.5, 145.2, 139.6, 134.4, 132.9, 131.5, 130.8, 128.2, 127.1, 126.2, 124.4, 123.1, 108.9, 108.3, 17.7, 12.8; IR (NaCl) 3070, 2927, 2854, 1600, 1523, 1442, 1350 cm−1; GC-MS m/z (rel intensity) 292 (M+, 100), 277 (17), 246 (11), 230 (25), 115 (11), 89 (6); HRMS m/z calc. for C18H16N2O2 292.1212 found 292.1215.
1-(2-Methoxy-4-nitrophenyl)-2-methyl-5-phenyl-1H-pyrrole (17).
Yield 88%. Yellow solid, mp 119–120 °C. TLC (30% ethyl acetate/hexane) Rf 0.70; 1H NMR (400 MHz, CDCl3) δ 7.85–7.81 (m, 2H), 7.24–7.06 (m, 4H), 7.02 (d, 2H, J = 6.8 Hz), 6.37 (d, 1H, J = 3.2 Hz), 6.14 (d, 1H, J = 3.2 Hz), 3.76 (s, 1H), 2.08 (s, 1H); 13C NMR (100 MHz, CDCl3) δ 155.8, 147.9, 134.7, 134.3, 133.1, 131.6, 130.6, 128.0, 127.2, 126.1, 115.9, 108.9, 108.1, 107.1, 56.1, 12.5; IR (NaCl) 3093, 2939, 1600, 1527, 1450, 1346, 1257 cm−1; GC-MS m/z (rel intensity) 308 (M+, 100), 293 (12), 262 (16), 231 (11), 115 (7); HRMS m/z calc. for C18H16N2O3 308.1161 found 308.1147.
1-(2-Fluoro-4-nitrophenyl)-2-methyl-5-phenyl-1H-pyrrole (18).
Yield 50%. Orange solid, mp 101–102 °C. TLC (30% ethyl acetate/hexane) Rf 0.71; 1H NMR (400 MHz, CDCl3) 1H NMR (400 MHz, CDCl3) δ 8.07 (dd, 1H, J = 9.1, 2.3 Hz), 8.02 (dd, 1H, J = 8.5, 2.3 Hz), 7.31–7.25 (m, 1H), 7.19–7.12 (m, 3H), 7.06–7.03 (m, 2H), 6.40 (d, 1H J = 3.4 Hz), 6.18 (d, 1H J = 3.4 Hz), 2.15 (s, 3H); δ 7.99 (dd, 1H, J = 9.1, 2.3 Hz), 7.94 (dd, 1H, J = 8.7, 2.3 Hz), 7.21 (t, 1H, J = 8.0 Hz), 7.12–6.95 (m, 5H), 6.32 (d, 1H J = 3.4 Hz), 6.09 (d, 1H J = 3.4 Hz), 2.06 (s, 3H); 13C NMR (100 MHz, CDCl3) δ 157.4 (d, 1H, J = 255.7 Hz), 147.4 (d, 1H, J = 9.1 Hz), 134.9, 133.6 (d, 1H, J = 13.2 Hz), 132.5, 131.4, 128.3, 127.5, 126.6, 119.6 (d, 1H, J = 3.3 Hz), 112.6 (d, 1H J = 24.8 Hz), 110.0, 109.0, 12.5; IR (NaCl) 3090, 2923, 1604, 1531, 1504, 1350 cm−1 GC-MS m/z (rel intensity) 296(M+, 100), 250 (46), 235 (7), 115 (6), 94 (6); HRMS m/z calc. for C17H13FN2O2 296.0961 found 296.0961.
1-(4-Fluoro-3-nitrophenyl)-2-methyl-5-phenyl-1H-pyrrole (19).
Yield 93%. Yellow Solid, mp 108–109 °C. TLC (30% ethyl acetate/hexane) Rf 0.63; 1H NMR (400 MHz, CDCl3) δ 7.92 (dd, 1H, J = 6.3, 2.0 Hz), 7.36–7.34 (m, 1H), 7.26–7.11 (m, 6H), 7.03 (d, 2H, J = 7.3 Hz), 6.35 (d, 1H, J = 2.9 Hz), 6.13 (d, 1H, J = 2.9 Hz), 2.18 (s, 3H); 13C NMR (100 MHz, CDCl3) δ 154.1 (d, J = 266.5 Hz), 135.8 (d, J = 4.1 Hz), 135.3 (d, J = 9.1 Hz), 134.4, 132.5, 131.3, 128.3, 128.1, 126.4, 125.4 (d, J = 2.5 Hz), 119.0, 118.8, 109.8, 108.7, 13.3; IR (NaCl) 3093, 2943, 1600, 1531, 1450, 1346 cm−1; GC-MS m/z (rel intensity) 296 (M+, 100), 250 (48), 235 (45), 207 (7), 115 (11), 94 (11), 63 (5); HRMS m/z calc. for C17H13FN2O2 296.0961 found 296.0949.
General procedure for the indium-mediated reaction of (1H-pyrrol-1-yl)nitroarenes with 2,5-hexanedione or 1-phenyl-1,4-pentanedione to obtain unsymmetrical ((1H-pyrrol-1-yl)phenyl)-1H-pyrroles.
The nitrobenzene derivative (1.0 mmol) and indium powder (460 mg, 4.0 mmol) were placed in toluene (2 mL), and were added acetic acid (0.572 mL, 10 mmol) and 2,5-hexanedione (or 1-phenyl-1,4-pentanedione, 1.0 mmol) in toluene (3 mL) to the mixture. The reaction mixture was stirred at 80 °C for 2,5-hexanedione (or reflux for 1-phenyl-1,4-pentanedione) under nitrogen. After the reaction was completed, the reaction mixture was diluted with ethyl acetate (30 mL), filtered through Celite, poured into 10% aqueous NaHCO3 solution (30 mL), and then the aqueous layer was extracted with ethyl acetate (30 mL × 3). The combined organic extracts were dried over MgSO4, filtered, and concentrated. The residue was eluted with hexane for most derivatives or ethyl acetate/hexane (v/v = 5/95) for benzonitrile derivatives through a neutral silica gel column to give the corresponding pyrroles. As all of the final products were unknown compounds, the structures of the pyrroles were fully characterized by 1H NMR, 13C NMR, FTIR, GC-MS and HRMS data.
2,5-Dimethyl-1-[4-(2-methyl-5-phenyl-1H-pyrrol-1-yl)phenyl]-1H-pyrrole (20).
Yield 92% (Table 4, entry 1), 79% (entry 8). White solid, mp 161–162 °C. TLC (20% ethyl acetate/hexane) Rf 0.66; 1H NMR (400 MHz, CDCl3) δ 7.25–7.03 (m, 9H), 6.39 (d, 1H, J = 3.4 Hz), 6.14 (d, 1H, J = 3.4 Hz), 5.92 (s, 2H), 2.23 (s, 3H), 2.04 (s, 6H); 13C NMR (100 MHz, CDCl3) δ 138.7, 137.9, 134.2, 133.2, 131.4, 129.1, 128.7, 128.6, 127.8, 127.7, 125.8, 108.8, 107.8, 105.9, 13.3, 12.8; IR (NaCl) 3105, 3058, 2977, 2920, 1600, 1512, 1442, 1392 cm−1; GC-MS m/z (rel intensity) 326 (M+, 100), 311 (24), 230 (12), 162 (36), 115 (10); HRMS m/z calc. for C23H22N2 326.1783, found 326.1770.
2,5-Dimethyl-1-[3-(2-methyl-5-phenyl-1H-pyrrol-1-yl)phenyl]-1H-pyrrole (21).
Yield 92% (Table 4, entry 2), 90% (entry 9). White solid, mp 87–88 °C. TLC (30% ethyl acetate/hexane) Rf 0.83; 1H NMR (400 MHz, CDCl3) δ 7.48 (t, 1H, J = 7.9 Hz), 7.28 (dt, 1H, J = 7.9, 2.0 Hz), 7.16–7.14 (m, 3H), 7.07 (t, 3H, J = 2.0 Hz), 6.94 (t, 1H, J = 2.0 Hz), 6.34 (d, 1H, J = 3.4 Hz), 6.10 (d, 1H, J = 3.4 Hz), 5.83 (s, 2H), 2.19 (s, 3H), 1.86 (s, 6H); 13C NMR (100 MHz, CDCl3) δ 140.1, 139.5, 134.3, 133.3, 131.4, 129.4, 128.68, 128.61, 128.0, 127.9, 127.5, 127.2, 125.8, 108.9, 107.8, 105.8, 13.3, 12.7; IR (NaCl) 3101, 3062, 2974, 2920, 1604, 1492, 1392 cm−1; GC-MS m/z (rel intensity) 326 (M+, 100), 311 (11), 295 (5), 230 (5), 162 (11); HRMS m/z calc. for C23H22N2 326.1783, found 326.1759.
2,5-Dimethyl-1-[2-methyl-4-(2-methyl-5-phenyl-1H-pyrrol-1-yl)phenyl]-1H-pyrrole (22).
Yield 85%. White solid, mp 127–129 °C. TLC (30% ethyl acetate/hexane) Rf 0.84; 1H NMR (400 MHz, CDCl3) δ 7.13–7.07 (m, 8H), 6.38 (d, 1H, J = 3.4 Hz), 6.11 (d, 1H, J = 3.4 Hz), 5.92 (s, 2H), 2.22 (s, 3H), 1.93 (s, 6H), 1.87 (s, 3H); 13C NMR (100 MHz, CDCl3) δ 139.4, 138.2, 137.3, 134.2, 133.4, 131.4, 130.6, 129.4, 128.0, 127.8, 127.7, 126.8, 125.7, 108.8, 107.7, 105.7, 17.0, 13.2, 12.3; IR (NaCl) 3101, 3058, 2977, 2920, 1604, 1513, 1442, 1419, 1388 cm−1; GC-MS m/z (rel intensity) 340 (M+, 100), 325 (86), 310 (14), 230 (13), 169 (30), 154 (13), 115 (11); HRMS m/z calc. for C24H24N2 340.1939, found 340.1918.
2,5-Dimethyl-1-[2-methyl-5-(2-methyl-5-phenyl-1H-pyrrol-1-yl)phenyl]-1H-pyrrole (23).
Yield 84%. Yellow oily liquid. TLC (30% ethyl acetate/hexane) Rf 0.83; 1H NMR (400 MHz, CDCl3) δ 7.33 (d, 1H, J = 8.0 Hz), 7.21 (dd, 1H, J = 8.0, 2.0 Hz), 7.15–7.05 (m, 5H), 6.89 (d, 1H, J = 2.0 Hz), 6.32 (d, 1H, J = 3.4 Hz), 6.09 (d, 1H, J = 3.4 Hz), 5.84 (s, 2H), 2.18 (s, 3H), 1.93 (s, 3H), 1.77 (s, 6H); 13C NMR (100 MHz, CDCl3) δ 138.5, 137.8, 136.4, 134.2, 133.4, 131.4, 130.9, 129.0, 128.2, 128.1, 128.0, 127.9, 125.7, 108.7, 107.7, 105.4, 16.7, 13.3, 12.3; IR (NaCl) 3055, 2923, 1612, 1508, 1442, 1392 cm−1; GC-MS m/z (rel intensity) 340 (M+, 100), 325 (96), 309 (22), 230 (14), 169 (27), 154 (15), 115 (11); HRMS m/z calc. for C24H24N2 340.1939, found 340.1939.
2,5-Dimethyl-1-[2-methoxy-4-(2-methyl-5-phenyl-1H-pyrrol-1-yl)phenyl]-1H-pyrrole (24).
Yield 70%. Colorless oily liquid. TLC (30% ethyl acetate/hexane) Rf 0.68; 1H NMR (400 MHz, CDCl3) δ 7.17–7.06 (m, 6H), 6.90 (dd, 1H, J = 8.0, 2.2 Hz), 6.70 (d, 1H, J = 2.2 Hz), 6.39 (d, 1H, J = 3.4 Hz), 6.13 (d, 1H, J = 3.4 Hz), 3.54 (s, 3H), 2.27 (s, 3H), 1.97 (s, 6H); 13C NMR (100 MHz, CDCl3) δ 156.0, 140.1, 134.2, 133.3, 131.3, 130.1, 128.9, 127.8, 127.7, 126.5, 125.8, 120.4, 112.8, 108.7, 107.7, 105.3, 55.8, 13.3, 12.3; IR (NaCl) 3070, 2923, 1604, 1519, 1454, 1388 cm−1; GC-MS m/z (rel intensity) 356 (M+, 100), 341 (34), 325 (8), 178 (10), 158 (6), 115 (5), 77 (4); HRMS m/z calc. for C24H24N2O 356.1889, found 340.1884.
2,5-Dimethyl-1-[2-fluoro-4-(2-methyl-5-phenyl-1H-pyrrol-1-yl)phenyl]-1H-pyrrole (25).
Yield 69%. White solid, mp 158–159 °C. TLC (30% ethyl acetate/hexane) Rf 0.79; 1H NMR (400 MHz, CDCl3) δ 7.25–7.10 (m, 4H), 7.06–7.03 (m, 4H), 6.38 (d, 1H, J = 3.2 Hz), 6.13 (d, 1H, J = 3.2 Hz), 5.94 (s, 2H), 2.24 (s, 3H), 2.02 (s, 6H); 13C NMR (100 MHz, CDCl3) δ 158.0 (d, J = 252.4 Hz), 140.4 (d, J = 9.1 Hz), 134.3, 132.9, 131.3, 130.6 (J = 1.7 Hz), 128.9, 128.0, 127.7, 126.1, 125.7 (d, J = 13.2 Hz), 124.7 (d, J = 3.3 Hz), 116.9 (d, J = 22.3 Hz), 109.2, 108.2, 106.3, 13.3, 12.3; IR (NaCl) 3051, 2920, 1600, 1519, 1442, 1388 cm−1; GC-MS m/z (rel intensity) 344 (M+, 100), 329 (7), 248 (4), 172 (14), 15 (4); HRMS m/z calc. for C23H21FN2 344.1689, found 344.1687.
2,5-Dimethyl-1-[4-fluoro-3-(2-methyl-5-phenyl-1H-pyrrol-1-yl)phenyl]-1H-pyrrole (26).
Yield 71%. White solid, mp 138–139 °C. TLC (20% ethyl acetate/hexane) Rf 0.70; 1H NMR (400 MHz, CDCl3) δ 7.27 (t, 1H, J = 8.8 Hz), 7.19–7.06 (m, 6H), 6.91 (dd, 1H, J = 6.8, 2.4 Hz), 6.35 (d, 1H, J = 3.4 Hz), 6.12 (d, 1H, J = 3.4 Hz), 5.81 (s, 2H), 2.17 (s, 3H), 1.80 (s, 6H); 13C NMR (100 MHz, CDCl3) δ 157.4 (d, J = 251.6 Hz), 135.1 (d, J = 4.1 Hz), 134.7, 132.9, 132.1, 130.9, 129.2 (d, J = 8.3 Hz), 128.6, 128.1, 127.78 (d, J = 14.1 Hz), 127.74, 126.2, 116.8 (d, J = 22.3 Hz), 109.2, 108.0, 105.8, 12.6, 12.4 (d, J = 2.5 Hz); IR (NaCl) 3063, 2923, 1608, 1442, 1400, 1380 cm−1; GC-MS m/z (rel intensity) 344 (M+, 100), 329 (5), 187 (4), 172 (13) 115 (4); HRMS m/z calc. for C23H21FN2 344.1689, found 344.1693.
2,5-Dimethyl-1-[3-methyl-4-(2-methyl-5-phenyl-1H-pyrrol-1-yl)phenyl]-1H-pyrrole (27).
Yield 81%. Pale yellow solid, mp 88–89 °C. TLC (30% ethyl acetate/hexane) Rf 0.82; 1H NMR (400 MHz, CDCl3) δ 7.28 (d, 1H, J = 8.0 Hz), 7.03–6.99 (m, 7H), 6.35 (d, 1H, J = 3.4 Hz), 6.07 (d, 1H, J = 3.4 Hz), 5.83 (s, 2H), 2.01 (s, 3H), 1.96 (s, 6H). 1.79 (s, 3H); 13C NMR (100 MHz, CDCl3) δ 138.9, 138.1, 134.1, 133.5, 130.9, 130.5, 130.0, 128.5, 127.9, 126.9, 126.3, 125.8, 108.3, 107.6, 105.9, 17.3, 12.8, 12.7; IR (NaCl) 3101, 3055, 2977, 2920, 1604, 1512, 1442, 1415, 1384 cm−1; GC-MS m/z (rel intensity) 340 (M+, 100), 325 (50), 309 (11), 263 (10), 230 (13), 170 (24), 154 (10), 115 (10); HRMS m/z calc. for C24H24N2 340.1939, found 340.1926.
2,5-Dimethyl-1-[4-methyl-3-(2-methyl-5-phenyl-1H-pyrrol-1-yl)phenyl]-1H-pyrrole (28).
Yield 78%. White solid, mp 148–149 °C. TLC (30% ethyl acetate/hexane) Rf 0.88; 1H NMR (400 MHz, CDCl3) δ 7.29 (d, 1H, J = 8.0 Hz), 7.14–7.06 (m, 7H), 6.38 (d, 1H, J = 3.4 Hz), 6.11 (d, 1H, J = 3.4 Hz), 5.87 (s, 2H), 2.05 (s, 3H), 1.97 (s, 6H), 1.93 (s, 3H); 1H NMR (400 MHz, CDCl3) δ 7.22 (d, 1H, J = 7.8 Hz), 7.06–6.99 (m, 7H), 6.30 (d, 1H, J = 3.2 Hz), 6.03 (d, 1H, J = 3.2 Hz), 5.79 (s, 2H), 1.97 (s, 3H), 1.89 (s, 6H), 1.86 (s, 3H); 13C NMR (100 MHz, CDCl3) δ 139.2, 137.3, 136.6, 134.2, 133.5, 131.2, 130.9, 129.3, 128.6, 128.1, 128.0, 127.3, 125.8, 108.3, 107.6, 105.9, 16.9, 12.7, 12.6; IR (NaCl) 3062, 2923, 1611, 1508, 1446, 1400 cm−1; GC-MS m/z (rel intensity) 340 (M+, 100), 325 (60), 309 (12), 230 (16), 170 (2), 154 (10), 115 (10); HRMS m/z calc. for C24H24N2 340.1939, found 340.1929.
2,5-Dimethyl-1-[3-methoxy-4-(2-methyl-5-phenyl-1H-pyrrol-1-yl)phenyl]-1H-pyrrole (29).
Yield 77%. Colorless oily liquid. TLC (30% ethyl acetate/hexane) Rf 0.67; 1H NMR (400 MHz, CDCl3) δ 7.16–7.04 (m, 6H), 6.80–6.77 (m, 2H), 6.79–6.78 (m, 2H), 6.39 (d, 1H, J = 3.4 Hz), 6.13 (d, 1H, J = 3.4 Hz), 5.92 (s, 2H), 3.62 (s, 3H), 2.15 (s, 6H), 2.06 (s, 6H); 13C NMR (100 MHz, CDCl3) δ 156.0, 139.6, 134.5, 133.6, 131.7, 130.5, 128.6, 127.7, 127.6, 127.2, 125.7, 120.4, 112.2, 108.0, 107.2, 105.8, 55.8, 12.8, 12.6; IR (NaCl) 3062, 2922, 1604, 1519, 1454, 1388 cm−1; GC-MS m/z (rel intensity) 356 (M+, 100), 341 (12), 325 (5), 178 (8), 154(4), 115 (5), 77 (4); HRMS m/z calc. for C24H24N2O 356.1889, found 340.1874.
2,5-Dimethyl-1-[3-fluoro-4-(2-methyl-5-phenyl-1H-pyrrol-1-yl)phenyl]-1H-pyrrole (30).
Yield 40%. Orange oily liquid. TLC (30% ethyl acetate/hexane) Rf 0.81; 1H NMR (400 MHz, CDCl3) δ 7.22–7.02 (m, 8H), 6.97 (dd, 1H, J = 8.4, 2.2 Hz), 6.40 (d, 1H, J = 3.4 Hz), 6.15 (d, 1H, J = 3.4 Hz), 5.91 (s, 1H), 2.19 (s, 3H), 2.04 (s, 6H); 13C NMR (100 MHz, CDCl3) δ 158.0 (d, J = 252.4 Hz), 139.9 (d, J = 9.1 Hz), 134.7, 133.0, 131.9, 131.0 (d, J = 1.7 Hz), 128.5, 128.0, 127.4, 126.8 (d, J = 12.4 Hz), 126.2, 124.4 (d, J = 3.3 Hz), 116.6 (d, J = 20.7 Hz), 109.0, 108.0, 106.4, 12.8, 12.5 (d, J = 2.5 Hz); IR (NaCl) 3058, 2923, 1604, 1515, 1438, 1384 cm−1; GC-MS m/z (rel intensity) 344 (M+, 100), 329 (6), 302 (4), 171 (10), 115 (4); HRMS m/z calc. for C23H21FN2 344.1689, found 344.1694.
2,5-Dimethyl-1-[2-fluoro-5-(2-methyl-5-phenyl-1H-pyrrol-1-yl)phenyl]-1H-pyrrole (31).
Yield 55%. Pale yellow solid, mp 142–143 °C. TLC (20% ethyl acetate/hexane) Rf 0.65; 1H NMR (400 MHz, CDCl3) δ 7.29–7.22 (m, 2H), 7.18–7.05 (m, 5H), 6.97 (dd, 1H, J = 6.8, 2.0 Hz), 6.33 (d, 1H, J = 3.2 Hz), 6.10 (d, 1H, J = 3.2 Hz), 5.86 (s, 2H), 2.19 (s, 2H), 1.85 (s, 6H); 13C NMR (100 MHz, CDCl3) δ 157.3 (d, J = 253.2 Hz), 135.7 (d, J = 3.3 Hz), 134.4, 133.1, 131.4, 130.8, 129.4 (d, J = 7.4 Hz), 128.9, 128.1, 128.0, 127.0 (d, J = 14.1 Hz), 126.0, 116.9, 116.7, 109.0, 107.9, 106.2, 13.2, 12.2; IR (NaCl) 3066, 2920, 1608, 1515, 1446, 1388, 1261 cm−1; GC-MS m/z (rel intensity) 344 (M+, 100), 329 (7), 309 (5), 248(4), 172 (1), 115 (4); HRMS m/z calc. for C23H21FN2 344.1689, found 344.1697.
Acknowledgements
B. H. Kim acknowledges Kwangwoon University for his sabbatical leave to the Department of Chemistry and Biochemistry at the University of California, Santa Barbara, USA in 2015. The authors thank Ms Yoonmi Choi of the Korea Research Institute of Chemical Technology for the X-ray analysis.
Notes and references
-
(a) P. A. Jacobi, L. D. Coults, J. S. Guo and S. I. Leung, J. Org. Chem., 2000, 65, 205–213 CrossRef CAS PubMed;
(b) A. Fürstner, Angew. Chem., Int. Ed., 2000, 39, 3012–3043 CrossRef;
(c) A. Fürstner, H. Szillat, B. Gobor and R. Mynott, J. Am. Chem. Soc., 1998, 120, 8305–8314 CrossRef;
(d) D. L. Boger, C. W. Boyce, M. A. Labrili, C. A. Sehon and Q. Jin, J. Am. Chem. Soc., 1999, 121, 54–62 CrossRef CAS.
-
(a) A. F. Diaz, J. I. Castillo, J. A. Logan and W. Y. Lee, J. Electroanal. Chem., 1981, 129, 115–132 CrossRef CAS;
(b) K. K. Kanazawa, A. F. Diaz, R. H. Geiss, W. D. Gill, J. F. Kwak, J. A. Logan, J. F. Rabolt and G. B. Street, J. Chem. Soc., Chem. Commun., 1979, 854–855 RSC.
-
(a)
J. L. Seller, P. A. Gale and W. S. Cho, in Anion Receptor Chemistry; Monographs in Supramolecular Chemistry, ed. J. F. Stoddart, RSC publishing, Cambridge, U. K., 2006 Search PubMed;
(b) P. D. Beer and P. A. Gale, Angew. Chem., Int. Ed., 2001, 40, 486–516 CrossRef CAS;
(c) P. A. Gale, Coord. Chem. Rev., 2003, 240, 191–221 CrossRef CAS;
(d) P. A. Gale and R. Quesada, Coord. Chem. Rev., 2006, 250, 3219–3244 CrossRef CAS;
(e) P. A. Gale, S. E. Garcia-Garrido and J. Garric, Chem. Soc. Rev., 2008, 37, 151–190 RSC;
(f) V. Estévez, M. Villacampa and J. C. Menéndez, Chem. Soc. Rev., 2010, 39, 4402–4421 RSC;
(g) S. K. Kim, D. E. Gross, D. Cho, V. Lynch and J. L. Sessler, J. Org. Chem., 2011, 76, 1005–1012 CrossRef CAS PubMed.
-
(a) G. Balme, Angew. Chem., Int. Ed., 2004, 43, 6238–6241 CrossRef CAS PubMed;
(b) B. C. Philippa, O. Matthew, L. B. Duncan, K. Peter, P. Anastasios, P. Miguel and V. L. Steven, Org. Biomol. Chem., 2012, 10, 5774–5779 RSC;
(c) K. Aghapoor, L. Ebadi-Nia, F. Mohsenzadeh, M. M. Morad, Y. Balavar and H. R. Darabi, J. Organomet. Chem., 2012, 708, 25–30 CrossRef;
(d) H. R. Darabi, M. R. Poorheravi, K. Aghapoor, A. Mirzaee, F. Mohsenzadeh, N. Asadollahnejad, H. Taherzadeh and Y. Balavar, Environ. Chem. Lett., 2012, 10, 5–12 CrossRef CAS;
(e) B. Benjamin, M. Thompson and M. John, Org. Lett., 2011, 13, 3289–3291 CrossRef PubMed;
(f) B. K. Banik, I. Banik, M. Renteria and S. K. Dasgupta, Tetrahedron Lett., 2005, 46, 2643–2645 CrossRef CAS;
(g) O. Galangau, C. Dumas-Verdes, E. Y. Schmidt, B. A. Trofimov and G. Clavier, Organometallics, 2011, 30, 6476–6481 CrossRef CAS;
(h) S. H. Kim, J. W. Lim, C. H. Lim and J. N. Kim, Bull. Korean Chem. Soc., 2012, 33, 620–624 CrossRef CAS;
(i) A. Rahmatpour, Appl. Organomet. Chem., 2011, 25, 585–590 CrossRef CAS;
(j) J. Chen, X. Yang, M. Liu, H. Wu, J. Ding and W. Su, Synth. Commun., 2009, 39, 4180–4198 CrossRef CAS.
- V. Estévez, M. Villacampa and J. C. Menéndez, Chem. Commun., 2013, 49, 591–593 RSC.
- X. Qi, X. Xu and C. Park, Chem. Commun., 2012, 48, 3996–3998 RSC.
- H. Wang, D. S. Mueller, R. M. Sachwani, R. Kapadia, H. N. Londino and L. L. Anderson, J. Org. Chem., 2011, 76, 3203–3221 CrossRef CAS PubMed.
- S. T. M. Marie, J. S. Daniel and A. A. Bruce, Org. Lett., 2010, 12, 4916–4919 CrossRef PubMed.
- B. Ramanathan, A. J. Keith, D. Armstrong and A. L. Odom, Org. Lett., 2004, 6, 2957–2960 CrossRef CAS PubMed.
- J. D. Timothy, F. B. John and K. M. C. Louis, Org. Biomol. Chem., 2012, 10, 1322–1328 Search PubMed.
-
(a) F. Chen, T. Shen, Y. Cui and N. Jiao, Org. Lett., 2012, 14, 4926–4929 CrossRef CAS PubMed;
(b) J. M. Kelly and F. J. Leeper, Tetrahedron Lett., 2012, 53, 819–821 CrossRef CAS;
(c) E. Ghabraie, S. Balalaie, M. Bararjanian, H. R. Bijanzadeh and F. Rominger, Tetrahedron, 2011, 67, 5415–5420 CrossRef CAS;
(d) M. Rubin and P. G. Ryabchuk, Chem. Heterocycl. Compd., 2012, 48, 126–138 CrossRef CAS;
(e) S. J. Pridmore, P. A. Slatford, J. E. Taylor, M. K. Whittlesey and J. M. Williams, Tetrahedron, 2009, 65, 8981–8986 CrossRef CAS.
- H. Lee and B. H. Kim, Tetrahedron, 2013, 69, 6698–6708 CrossRef CAS.
-
(a) B. H. Kim, Y. Jin, Y. M. Jun, R. Han, W. Baik and B. M. Lee, Tetrahedron Lett., 2000, 41, 2137–2140 CrossRef CAS;
(b) B. H. Kim, J. W. Cheong, R. Han, Y. M. Jun, W. Baik and B. M. Lee, Synth. Commun., 2001, 31, 3577–3586 CrossRef CAS;
(c) B. H. Kim, R. Han, F. Piao, Y. M. Jun, W. Baik and B. M. Lee, Tetrahedron Lett., 2003, 47, 77–79 CrossRef;
(d) R. Han, S. H. Choi, K. I. Son, Y. M. Jun and B. M. Lee, Synth. Commun., 2005, 35, 1725–1733 CrossRef CAS;
(e) B. H. Kim, R. Han, J. S. Kim, Y. M. Jun, W. Baik and B. M. Lee, Heterocycles, 2004, 62, 41–54 CrossRef;
(f) R. Han, S. Chen, S. J. Lee, F. Qi, X. Wu and B. H. Kim, Heterocycles, 2006, 68, 1675–1684 CrossRef CAS;
(g) R. Han, K. I. Son, G. H. Ahn, Y. M. Jun, B. M. Lee, Y. Park and B. H. Kim, Tetrahedron Lett., 2006, 47, 7295–7299 CrossRef CAS;
(h) G. H. Ahn, J. J. Lee, Y. M. Jun, B. M. Lee and B. H. Kim, Org. Biomol. Chem., 2007, 5, 2472–2485 RSC;
(i) J. S. Kim, J. H. Han, J. J. Lee, Y. M. Jun, B. M. Lee and B. H. Kim, Tetrahedron Lett., 2008, 49, 3733–3738 CrossRef CAS;
(j) R. Han, S. Chen, S. J. Lee, F. Qi, X. Wu and B. H. Kim, Heterocycles, 2013, 87, 155–162 CrossRef;
(k) J. J. Lee, J. Kim, Y. M. Jun, B. M. Lee and B. H. Kim, Tetrahedron Lett., 2009, 50, 8821–8831 CrossRef;
(l) A. Go, G. Lee, J. Kim, S. Bae, B. M. Lee and B. H. Kim, Tetrahedron, 2009, 50, 1215–1226 CrossRef.
-
(a) G. C. Porretta, F. Cerreto, F. Fioravanti, M. Biava, M. Scalzo, M. Fischetti and F. Riccardi, Farmaco, 1989, 44, 51–63 CAS;
(b) S. Kiralp, P. Camurlu, G. Gunbas, C. Tanyeli, I. Akhmedov and L. Toppare, J. Appl. Polym. Sci., 2009, 112, 1082–1108 CrossRef CAS;
(c) A.-Z. A. Elassar, J. Chem. Res., 2012, 36, 328–139 CrossRef CAS.
-
(a)
J. B. Birks, The Theory and Practice of Scintillation Counting, Pergamon, London, U.K., 1967, p. 664 Search PubMed;
(b) Z. Chen, S. Klyatskaya, J. I. Urgel, D. Écija, O. Fuhr, W. Auwärter, J. V. Barth and M. Ruben, Beilstein J. Nanotechnol., 2015, 6, 327–335 CrossRef CAS PubMed.
-
(a) S. V. Budakovsky, N. Z. Galunov, N. L. Karavaeva, J. K. Kim, Y. K. Kim, O. A. Tarasenko and E. V. Martynenko, IEEE Trans. Nucl. Sci., 2007, 546, 2734–2740 CrossRef;
(b) C. Matei, F.-J. Hambsch and S. Oberstedt, Nucl. Instrum. Methods Phys. Res., Sect. A, 2012, 18, 135–139 CrossRef.
- CCDC 1415102 contains the supplementary crystallographic data for this compound, 2,5-dimethyl-1-[4-(2-methyl-5-phenyl-1H-pyrrol-1-yl)phenyl]-1H-pyrrole (20).
- E. Senses, S. Karabocek, N. Karabocek and P. Ekmekcioglu, Asian J. Chem., 2012, 24, 3797–3801 CAS.
-
(a) R. Ghorbani-Vaghei and H. S. Veisi, Afr. J. Chem., 2009, 62, 33–38 CAS;
(b) H. Veisi, Tetrahedron Lett., 2010, 51, 2109–2114 CrossRef CAS.
- J. Chen, Synth. Commun., 2009, 39, 4180–4198 CrossRef CAS.
- M. Kobeissi, O. Yazbeck and Y. Chreim, Tetrahedron Lett., 2014, 55, 2523–2526 CrossRef CAS.
- S. J. Hazlewood, G. K. Hughes, F. Lions, K. J. Baldic, J. W. Cornforth, J. N. Graves, J. J. Maunsell, T. Wilkinson, A. J. Birch and R. H. Harradence, J. Proc. R. Soc. N. S. W., 1937, 71, 92–102 CAS.
Footnote |
† Electronic supplementary information (ESI) available: Copies of NMR spectra of ((1H-pyrrol-1-yl)phenyl)-1H-pyrrole compounds. CCDC 1415102. For ESI and crystallographic data in CIF or other electronic format see DOI: 10.1039/c5ob02101d |
|
This journal is © The Royal Society of Chemistry 2016 |
Click here to see how this site uses Cookies. View our privacy policy here.