DOI:
10.1039/C6NJ00336B
(Paper)
New J. Chem., 2016,
40, 7573-7579
Catalyst-free Biginelli-type synthesis of new functionalized 4,7-dihydropyrazolo[1,5-a]pyrimidines†
Received
(in Montpellier, France)
31st January 2016
, Accepted 6th July 2016
First published on 7th July 2016
Abstract
The Biginelli-type reaction of 5-amino-3-arylpyrazole-4-carbonitriles with aldehydes and 1,3-dicarbonyl compounds was studied in detail. We found that the reaction requires no catalysts and proceeds in boiling DMF to give 2-aryl-6-RC(O)-4,7-dihydropyrazolo[1,5-a]pyrimidin-3-carbonitriles in good yields. The reaction of aminopyrazoles with dicarbonyls leading to 6-unsubstituted pyrazolo[1,5-a]pyrimidines proceeds competitively and becomes the dominant process under acid-promoted conditions. 2-Aryl-8-oxo-4,5,6,7,8,9-hexahydropyrazolo[5,1-b]quinazolin-3-carbonitriles were obtained under catalyst-free conditions in up to 80% yields starting from 5-amino-3-arylpyrazole-4-carbonitriles, aldehydes and 1,3-cyclohexanedione.
1. Introduction
In the last two decades, the classical urea-based multicomponent reaction named after Italian chemist Pietro Biginelli, as well as the reactions of so-called Biginelli compounds (3,4-dihydropyrimidin-2(1H)-ones and -thiones) have attracted great attention (for recent reviews see ref. 1–9). Less attention is paid to the non-classical Biginelli-type reaction employing aminoazoles as N,N-dinucleophilic components.9–13 This approach is well suited for combinatorial chemistry, providing a diversity of di-, tri- and tetrazolopyrimidines. Our interests have been focused on the chemistry of unusual Biginelli-type compounds14 and, particularly, on the chemistry of pyrazolo- and triazolo[1,5-a]pyrimidines.15
Pyrazolo[1,5-a]pyrimidines are important scaffolds which are present in many synthetic drugs such as dorsomorphin16 or other bone morphogenetic protein receptor inhibitors useful for treatment of anemia, muscular dystrophy, atherosclerosis, etc.17 Pyrazolo[1,5-a]pyrimidines also have been recognized as purine analogs with c-AMP phosphodiesterase inhibitory activity,18 and as tyrosine kinase inhibitors.19 4,7-Dihydropyrazolo[1,5-a]pyrimidines (DPPMs) behave as potent mimics of Hantzsch 1,4-dihydropyridine calcium channel blockers20 and related pyrazolo[5,1-b]quinazolines were found to be potent KATP channel openers.21
Two most commonly used approaches to build the 4,7-dihydroazolo[1,5-a]pyrimidine core are known. The first one consists of the reaction of 3(5)-aminoazoles with α,β-unsaturated carbonyls (for reviews see ref. 11–13, 22) and the other is based on the modified Biginelli reaction of 3(5)-aminoazoles with aldehydes and active methylene carbonyl compounds9–13 (Scheme 1).
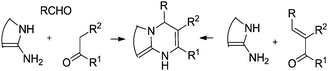 |
| Scheme 1 General approaches to the synthesis of dihydroazolopyrimidines. | |
When attempting to use 3(5)-aminopyrazoles with no substituents at the C-4 position, one may encounter the problem of a dual reactivity of these heterocycles. Thus, 3(5)-aminopyrazoles may react either as straight urea-type N,N-binucleophiles leading to the expected DPPMS,9,12 or as a masked enamine species – C,N-binucleophiles to afford 4,7-dihydropyrazolo[3,4-b]pyridines12,23,24 (Scheme 2).
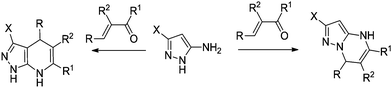 |
| Scheme 2 The reactions of aminopyrazoles with α,β-unsaturated carbonyls. | |
The problem of chemoselectivity in this context could be solved in two different ways – either by using selected reaction conditions (e.g., pH of reaction media, different catalysts and solvents, sonication or microwave irradiation) to modulate the selectivity,23–25 or,– by blocking the C-4 position in aminopyrazoles with a protecting group.24–27 From this point of view, easily available15a 5-aminopyrazole-4-carbonitriles could be considered as prospective reagents bearing the reactive cyano group at C-4, which may react under Biginelli conditions to afford functionalized heterocycles. In the present paper, we have studied the reaction of 5-amino-3-arylpyrazole-4-carbonitriles with active methylene 1,3-dicarbonyls and aldehydes with the aim to develop a synthetic route toward DPPMs.
2. Results and discussion
As a model reaction, we examined the reaction of 5-amino-3-phenylpyrazole-4-carbonitriles 1a with benzaldehyde 2A and N-(4-chlorophenyl)-3-oxobutanamide 3a (Scheme 3) in boiling DMF with no catalysts. As expected, the reaction proceeds smoothly to give DPPM 4a. In order to optimize the reaction conditions, we studied the effects of solvent amount, reaction time, reagents ratio and different work-up procedures on the yield of the target product 4A.
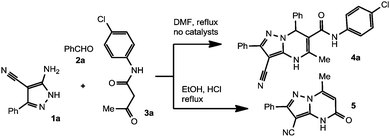 |
| Scheme 3 Synthesis of pyrazolopyrimidines 4 and 5. | |
The best yield of pure 4a (60% after recrystallization) was obtained when the reagents were taken in equimolar amounts and the reaction was conducted in a three-component mode. The best results were obtained when minimum amounts of DMF (0.5 mL per ∼2 mmol of each reagent) were used, under short term heating (∼20 min) and with a final precipitation of the product by addition of MeOH. Meanwhile, the reaction 1a + 2a + 3a in boiling EtOH under acid catalyzed conditions (HCl) proceeds in a quite different way to give pyrazolo[1,5-a]pyrimidine-5(4H)-one-3-carbonitrile 5 in 35% yield. According to the NMR data for crude pyrazolopyrimidine 4a, product 5 is also the main by-product of the uncatalyzed process.
With the optimized procedure described above, the analogs of compound 4a were obtained in 32–53% yields (Table 1). However, we failed to obtain DPPMs 4 by ternary condensation of aminopyrazole 1b with aldehydes and 2,4-pentanedione. Instead, the sole isolated product was 5,7-dimethylpyrazolo[1,5-a]pyrimidine-3-carbonitrile 6 as the result of the reaction of 1b with 2,4-pentanedione alone (Scheme 4). It is noteworthy that the reaction proceeds notwithstanding the conditions of temperature or the acidity of the reaction media.
Table 1 Synthesis of DPPMs 4b–g
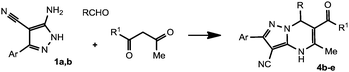
|
Entry |
Product |
Ar |
R |
R1 |
Yielda (%) |
The yields are given for pure products after recrystallization from MeOH.
|
1 |
4b
|
4-MeC6H4 |
Ph |
4-ClC6H4NH |
37 |
2 |
4c
|
Ph |
4-NO2C6H4 |
4-ClC6H4NH |
52 |
3 |
4d
|
4-MeC6H4 |
4-NMe2C6H4 |
4-ClC6H4NH |
32 |
4 |
4e
|
4-MeC6H4 |
Ph |
OEt |
53 |
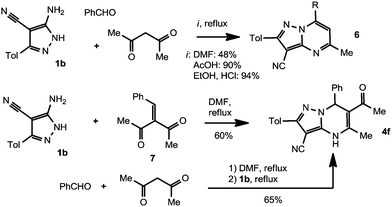 |
| Scheme 4 Synthesis of compounds 4f and 6. | |
In contrast, unsaturated diketone 7 reacts with aminopyrazole 1b to afford the desired DPPM 4f in a good yield. In order to find the optimal conditions, we attempted to combine the preparation of starting endione 7 and Biginelli-type condensation in a one-pot procedure. Thus, when PhCHO and Ac2CH2 were briefly heated in DMF to give condensation product 7 followed by treatment with aminopyrazole 1b, compound 4f was obtained in 65% yield. Using the same approach, ethyl acetoacetate was converted into 4,7-dihydropyrazolo[1,5-a]pyrimidine 4e in 53% yield.
The obtained observations allowed us to draw some tentative conclusions about how this Biginelli-type reaction proceeds in a three-component mode. We suggest that the reaction proceeds first by Knoevenagel reaction of an aldehyde with an active methylene compound, probably catalyzed either by aminopyrazole 1 which is basic enough, or DMF. Next, the uncatalyzed reaction of the formed condensation product with aminopyrazole leads to the formation of target compounds 4 (Scheme 5). The presumable formation of aza-Michael adduct 8 as an intermediate in the suggested mechanism is in agreement with previously reported data.11,22
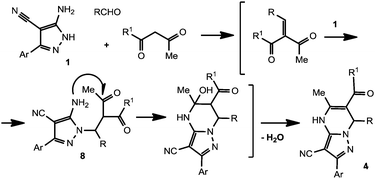 |
| Scheme 5 Probable mechanistic pathway for the synthesis of DPPMs 4. | |
It should be noted that, despite the known benefits of base-catalyzed Biginelli-type reactions (Atwal modification,28 Bakibaev and Filimonov protocol29) or non-catalyzed protocols,7,14b,30 most methods commonly used for the synthesis of Biginelli compounds require an acid as a catalyst.6–9 In our previous paper7 it was proposed as a joke that sodium chloride could be used as the “catalyst” for reactions that proceed quite efficiently without any additives and catalysts (the term “chemical placebo” was introduced by us7 to define a compound that is evidently ineffective as a catalyst but has to be added anyway, probably for non-scientific reasons or tradition). Recently, a detailed paper31 appeared in which conditions for Biginelli reaction were critically discussed. Inter alia, the authors reasonably doubted that NaCl could be an effective catalyst and claimed that “there is no reasonable doubt of the importance of catalysis for the Biginelli reaction, and the role of the catalyst is not only to facilitate the formation of the final product but also to select a preferred reaction pathway”. We have to admit that the above Biginelli-type reaction may proceed without any additional catalysts, at least in certain cases. In the present case, an acid catalyst strongly disfavors formation of the desired Biginelli products. Nevertheless, HCl was found to be a good catalyst for the reaction between aminopyrazoles 1a and 1,3-dicarbonyls.
The obtained results encouraged us to continue our studies with other dicarbonyl compounds. Thus, dihydroresorcinol (1,3-cyclohexanedione) reacts with aminopyrazoles 1a,b and aldehydes to give the expected 2-aryl-8-oxo-4,5,6,7,8,9-hexahydropyrazolo[5,1-b]quinazoline-3-carbonitriles 8a–d (Table 2).
Table 2 Synthesis of compounds 8a–d

|
Entry |
Product |
Ar |
R1 |
Yielda (%) |
The yields are given for pure products after recrystallization from MeOH.
|
1 |
8a
|
4-MeC6H4 |
Ph |
80 |
2 |
8b
|
4-MeC6H4 |
4-Me2NC6H4 |
56 |
3 |
8c
|
4-MeC6H4 |
Et |
62 |
4 |
8d
|
Ph |
4-MeC6H4 |
47 |
In order to examine the reactivity of the synthesized compounds, we unsuccessfully attempted to carry out thiolysis by passing H2S for 24 h through the DMF solution of compound 8a in the presence of Et3N. One may suggest that the deprotonation of N(4)H in a basic medium causes the formation of a highly mesomerically stabilized anion (Scheme 6) so the HS− anion should attack a negatively charged substrate which is unfavorable for thiolysis. The low reactivity of the cyano group toward the HS− anion might well be a consequence of tautomerism which involves the delocalization of the negative charge onto the cyano group. In order to confirm this suggestion, we synthesized the N(4)-alkyl derivative 9 by treatment of 8a with EtBr in KOH–H2O–MeCN system. It was found that compound 9 undergoes thiolysis under the same conditions to yield thioamide 10 (Scheme 7). Given these results, we may conclude that successful modification of the functionality at C(3) leading to compounds like 10 could be performed using protective groups (e.g., MOM14d) at the N(4) position, which could be easily removed later.
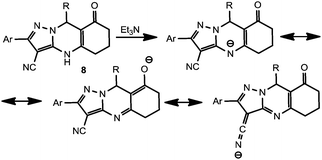 |
| Scheme 6 Possible mesomeric mechanism for stabilization of the negative charge for anions of 8. | |
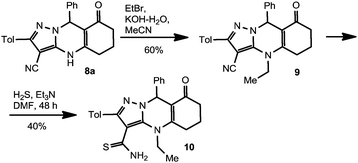 |
| Scheme 7 The synthesis and thiolysis of compound 9. | |
3. Conclusions
In conclusion, we have developed a simple and selective synthetic protocol based on the Biginelli-type multicomponent reaction of 5-amino-3-arylpyrazole-4-carbonitriles with aldehydes and 1,3-dicarbonyl compounds. The reported method provides catalyst-free access to functionalized 4,7-dihydropyrazolo[1,5-a]pyrimidine-3-carbonitriles and 4,5,6,7,8,9-hexahydropyrazolo[5,1-b]quinazolin-3-carbonitriles which could be further transformed by N-alkylation or thiolysis. In certain cases the Biginelli reaction competes with the side process of the formation of pyrazolo[1,5-a]pyrimidines by reaction of aminopyrazole and dicarbonyl components without the participation of an aldehyde. The latter reaction predominates in the presence of an acid catalyst, whereas the Biginelli-type reaction proceeds clearly without any catalysts in pure DMF.
4. Experimental
4.1 Materials and methods
1H NMR spectra were recorded on a Bruker Avance 400 spectrometer (400 MHz) and on a Varian Mercury VX-200 spectrometer (200 MHz) in DMSO-d6 with TMS as the internal standard. Chemical shifts are given in ppm, coupling constants are given in Hz. 13C NMR spectra were recorded on a Bruker Avance 500 spectrometer (125 MHz) and on a Bruker Avance 400 spectrometer (100 MHz) in DMSO-d6 with TMS as the internal standard. Mass spectra (EI, 70 eV) were obtained on a Varian 1200L instrument using a direct probe exposure (DEP) method. IR spectra were recorded in KBr pellets using a Perkin Elmer Spectrum One FT-IR spectrometer. Elemental analyses were obtained using a EuroEA-3000 instrument. Melting points were determined using a Köfler hot-stage apparatus. DMF was distilled under reduced pressure and stored under molecular sieves (4 Å). The purity of the compounds was checked using TLC (Merck ALUGRAM Xtra SIL G/UV 254 plates) with EtOAc/hexane mixtures as eluents. 5-Amino-3-arylpyrazole-4-carbonitriles 1a and b were obtained by a reported procedure.15a H2S gas was produced by water hydrolysis of Al2S3 and dried by passing its flow through the layer of Al2S3. Starting aldehydes, 1,3-dicarbonyl compounds and solvents are commercially available.
4.2 General process for preparation of pyrazolopyrimidines 4a–g and pyrazoloquinazolines 8a–d
A mixture of the appropriate aldehyde (2.2 mmol), 1,3-dicarbonyl compound (2 mmol) and the corresponding aminopyrazole 1 (2 mmol) in DMF (0.5 mL) was heated under reflux for 20 min. The mixture was cooled and MeOH (20 mL) was added. The precipitate was filtered off and washed with MeOH (3 × 3 mL).
N-(4-Chlorophenyl)-3-cyano-5-methyl-2,7-diphenyl-4,7-dihydropyrazolo[1,5-a]pyrimidine-6-carboxamide 4a.
White solid, mp 327–329 °C, 1H NMR (200 MHz, DMSO-d6) δ 10.44 (br. s, 1H, NH), 9.96 (br. s, 1H, NH), 7.67–7.82 (m, 2H, ArH), 7.56 (d, J = 9.0, 2H, ArH), 7.37–7.50 (m, 3H, ArH), 7.15–7.37 (m, 7H, ArH), 6.56 (s, 1H, C(7)H), 2.21 (s, 3H, CH3); 13C NMR (100 MHz, DMSO-d6) δ 165.15, 151.52, 144.49, 141.11, 138.38, 136.09, 131.22, 129.85, 129.40, 129.18, 129.02, 128.72, 127.42, 127.35, 126.51, 121.62, 114.97, 105.13, 70.85, 60.78, 17.49; IR (KBr, cm−1) νmax 1495, 1511, 1590, 1603, 1631, 1667, 2226, 2922, 3060, 3270 (br.); MS (m/z, EI, 70 eV): 467 (M(37Cl)+, 3%), 465 (M(35Cl)+, 9%), 339 (34%), 311 (56%), 235 (63%), 153 (55%), 127 (100%). Anal. calcd for C27H20ClN5O (465.14): C, 69.60; H, 4.33; N, 15.03%. Found: C, 69.79; H, 4.14; N, 14.86%.
N-(4-Chlorophenyl)-3-cyano-5-methyl-2-(4-methylphenyl)-7-phenyl-4,7-dihydropyrazolo[1,5-a]pyrimidine-6-carboxamide 4b.
White solid, mp 332–334 °C (MeOH, decomp.); 1H NMR (200 MHz, DMSO-d6) δ 10.44 (br. s, 1H, NH), 9.96 (br. s, 1H, NH), 7.64 (d, J = 7.8, 2H, ArH), 7.55 (d, J = 8.8, 2H, ArH), 7.10–7.42 (m, 9H, ArH), 6.54 (s, 1H, C(7)H), 2.30 (s, 3H, CH3), 2.21 (s, 3H, CH3); 13C NMR (125 MHz, DMSO-d6) δ 165.17, 151.57, 144.42, 141.15, 139.43, 138.38, 136.08, 129.88, 129.14, 128.99, 128.67, 128.49, 127.50, 127.35, 126.40, 121.64, 115.05, 105.14, 70.69, 60.75, 21.35, 17.48; IR (KBr, cm−1) νmax 1494, 1509, 1590, 1602, 1630, 2224, 2917, 3028, 3130, 3270 (br.) cm−1; MS (m/z, EI, 70 eV): 481 (M(37Cl)+, 8%), 479 (M(35Cl)+, 20%), 353 (100%), 325 (39%), 155 (33%), 127 (90%). Anal. calcd for C28H22ClN5O (479.96): C, 70.07; H, 4.62; N, 14.59%. Found: C, 69.91; H, 4.40; N, 14.40%.
N-(4-Chlorophenyl)-3-cyano-5-methyl-7-(4-nitrophenyl)-2-phenyl-4,7-dihydropyrazolo[1,5-a]pyrimidine-6-carboxamide 4c.
White solid, mp 317–319 °C (MeOH, decomp.); 1H NMR (400 MHz, DMSO-d6) δ 10.67 (br. s, 1H, NH), 10.00 (br. s, 1H, NH), 8.17 (d, J = 8.8, 2H, ArH), 7.65–7.80 (m, 2H, ArH), 7.37–7.63 (m, 7H, ArH), 7.31 (d, J = 8.8, 2H, ArH), 6.69 (s, 1H, C(7)H), 2.22 (s, 3H, CH3); 13C NMR (125 MHz, DMSO-d6) δ (125 MHz, DMSO-d6) 164.85, 151.94, 147.92, 147.80, 144.51, 138.19, 136.77, 131.02, 129.97, 129.39, 129.04, 128.81, 127.65, 126.56, 124.45, 121.71, 114.79, 104.14, 71.24, 60.14, 17.63; IR (KBr, cm−1) νmax 1348, 1493, 1521, 1629, 1664, 2227, 2853, 2923, 3269 (br.); MS (m/z, EI, 70 eV): 510 (M(35Cl)+, 2%), 384 (9%), 242 (100%), 127 (34%). Anal. calcd for C27H19ClN6O3 (510.93): C, 63.47; H, 3.75; N, 16.45%. Found: C, 63.70; H, 3.77; N, 16.31%.
N-(4-Chlorophenyl)-3-cyano-7-(4-dimethylaminophenyl)-5-methyl-2-(4-methylphenyl)-4,7-dihydropyrazolo[1,5-a]pyrimidine-6-carboxamide 4d.
White solid, mp 311–313 °C (MeOH, decomp.); 1H NMR (500 MHz, DMSO-d6) δ 10.38 (br. s, 1H, NH), 9.91 (br. s, 1H, NH), 7.67 (d, J = 8.0, 2H, ArH), 7.60 (d, J = 9.0, 2H, ArH), 7.33 (d, J = 8.5, 2H, ArH), 7.28 (d, J = 8.0, 2H, ArH), 7.09 (d, J = 8.5, 2H, ArH), 6.62 (d, J = 9.0, 2H, ArH), 6.45 (s, 1H, C(7)H), 2.84 (s, 6H, N(CH3)2), 2.34 (s, 3H, CH3), 2.24 (s, 3H, CH3); 13C NMR (125 MHz, DMSO-d6) δ 165.34, 151.25, 150.66, 144.13, 139.31, 138.53, 135.90, 129.86, 128.97, 128.93, 128.64, 128.47, 128.30, 127.36, 126.35, 126.30, 121.60, 121.54, 115.17, 112.54, 105.41, 70.40, 60.29, 21.36, 17.45; IR (KBr, cm−1) νmax 1494, 1520, 1600, 1633, 1667, 2221, 2799, 2919, 3273 (br.); MS (m/z, EI, 70 eV): 510 (M(37Cl)+, 9%), 508 (M(35Cl)+, 22%), 382 (100%), 354 (41%), 198 (24%), 127 (37%). Anal. calcd for C30H27ClN6O (523.03): C, 68.89; H, 5.20; N, 16.07%. Found: C, 69.01; H, 5.25; N, 15.89%.
Ethyl 3-cyano-5-methyl-2-(4-methylphenyl)-7-phenyl-4,7-dihydropyrazolo[1,5-a]pyrimidine-6-carboxylate 4e.
White solid, mp 239–241 °C (MeOH); 1H NMR (200 MHz, DMSO-d6) δ 10.95 (br. s, 1H, NH), 7.62 (d, J = 8.0, 2H, ArH), 7.10–7.40 (m, 7H, ArH), 6.25 (s, 1H, C(7)H), 3.97 (q, J = 7.0, 2H, CH2), 2.45 (s, 3H, CH3), 2.30 (s, 3H, CH3), 1.06 (t, J = 7.0, 3H, CH2CH3); 13C NMR (125 MHz, DMSO-d6) δ 165.36, 151.76, 145.61, 143.11, 142.36, 139.47, 129.87, 128.97, 128.51, 128.33, 127.49, 126.41, 114.73, 98.96, 71.55, 60.07, 60.06, 21.35, 18.43, 14.40; IR (KBr, cm−1) νmax 1551, 1571, 1594, 1645, 1704, 2224, 2930, 2989, 3107, 3252 (br.); MS (m/z, EI, 70 eV): 398 (M+, 61%), 369 (20%), 321 (100%), 293 (44%), 103 (26%), 91 (31%). Anal. calcd for C24H22N4O2 (398.46): C, 72.34; H, 5.57; N, 14.06%. Found: C, 72.95; H, 5.70; N, 13.83%.
2-(4-Methylphenyl)-8-oxo-9-phenyl-4,5,6,7,8,9-hexahydropyrazolo[5,1-b]quinazoline-3-carbonitrile 8a.
White solid, mp 329–331 °C (MeOH); 1H NMR (200 MHz, DMSO-d6) δ 11.32 (br. s, 1H, NH), 7.63 (d, J = 8.2, 2H, ArH), 6.95–7.20 (m, 7H, ArH), 6.21 (s, 1H, C(9)H), 2.56–2.86 (m, 2H, CH2), 2.12–2.40 (m, 2H, CH2), 2.30 (s, 3H, CH3), 1.70–2.10 (m, 2H, CH2); 13C NMR (125 MHz, DMSO-d6) δ 193.38, 151.23, 150.89, 142.65, 141.43, 139.14, 129.51, 128.49, 127.92, 127.43, 127.09, 126.02, 114.27, 107.50, 71.68, 57.83, 36.35, 26.08, 20.97, 20.65; IR (KBr, cm−1) νmax 1571, 1585, 1643, 1721, 2223, 2918, 3199 (br.), 3249; MS (m/z, EI, 70 eV): 380 (M+, 7%), 312 (34%), 199 (35%), 149 (100%), 115 (30%). Anal. calcd for C24H20N4O (380.44): C, 75.77; H, 5.30; N, 14.73%. Found: C, 75.54; H, 5.12; N, 14.53%.
9-(4-Dimethylaminophenyl)-2-(4-methylphenyl)-8-oxo-4,5,6,7,8,9-hexahydropyrazolo[5,1-b]quinazoline-3-carbonitrile 8b.
White solid, mp 299–301 °C (MeOH); 1H NMR (200 MHz, DMSO-d6) δ 11.18 (br. s, 1H, NH), 7.64 (d, J = 6.8, 2H, ArH), 7.24 (d, J = 6.8, 2H, ArH), 7.03 (d, J = 7.2, 2H, ArH), 7.57 (d, J = 7.2, 2H, ArH), 6.09 (s, 1H, C(9)H), 2.56–3.00 (m, 2H, CH2), 2.80 (s, 6H, N(CH3)2), 2.18–2.37 (m, 2H, CH2), 2.30 (s, 3H, CH3), 1.75–2.06 (m, 2H, CH2); 13C NMR (125 MHz, DMSO-d6) δ 193.74, 151.39, 150.79, 150.47, 142.90, 139.41, 129.87, 129.53, 128.43, 128.13, 126.38, 114.79, 112.48, 108.32, 71.80, 57.73, 36.85, 26.47, 21.37, 21.14; IR (KBr, cm−1) νmax 1524, 1569, 1615, 1643, 2230, 2798, 2944, 3102, 3165 (br.), 3233, 3502 (br.); MS (m/z, EI, 70 eV): 422 (M+, 100%), 395 (26%), 304 (30%), 211 (11%), 120 (36%), 91 (32%). Anal. calcd for C26H25N5O (423.51): C, 73.74; H, 5.95; N, 16.54%. Found: C, 73.56; H, 6.13; N, 16.35%.
9-Ethyl-2-(4-methylphenyl)-8-oxo-4,5,6,7,8,9-hexahydropyrazolo[5,1-b]quinazoline-3-carbonitrile 8c.
White solid, mp 224–226 °C (MeOH); 1H NMR (200 MHz, DMSO-d6) δ 10.89 (br. s, 1H, NH), 7.71 (d, J = 8.0, 2H, ArH), 7.28 (d, J = 8.0, 2H, ArH), 5.29 (s, 1H, C(9)H), 2.53–2.72 (m, 2H, CH2), 2.18–2.42 (m, 2H, CH2), 2.33 (s, 3H, CH3), 1.58–2.10 (m, 4H, 2CH2), 0.56 (t, J=7.0, 3H, CH2CH3); 13C NMR (125 MHz, DMSO-d6) δ 194.31, 152.46, 151.38, 143.80, 139.42, 129.90, 128.52, 126.43, 114.76, 105.68, 71.57, 55.65, 36.91, 27.04, 26.48, 21.38, 21.22, 7.92; IR (KBr, cm−1) νmax 1443, 1568, 1588, 1640, 2216, 2934, 3105, 3181 (br.), 3245, 5322 (br.); MS (m/z, EI, 70 eV): 332 (M+, 12%), 303 (100%), 116 (13%), 91 (20%). Anal. calcd for C20H20N4O (332.40): C, 72.27; H, 6.06; N, 16.86%. Found: C, 72.49; H, 5.87; N, 16.70%.
9-(4-Methylphenyl)-8-oxo-2-phenyl-4,5,6,7,8,9-hexahydropyrazolo[5,1-b]quinazoline-3-carbonitrile 8d.
White solid, mp 309–311 °C (MeOH); 1H NMR (200 MHz, DMSO-d6) δ 10.77 (br. s, 1H, NH), 7.63–7.82 (m, 2H, ArH), 7.33–7.52 (m, 3H, ArH), 7.12 (d, 2H, J = 8.0, ArH), 7.06 (d, J = 8.0, 2H, ArH), 6.17 (s, 1H, C(9)H), 2.60–2.84 (m, 2H, CH2), 2.20 (s, 3H, CH3), 2.12–2.34 (m, 2H, CH2), 1.74–2.05 (m, 2H, CH2); 13C NMR (100 MHz, DMSO-d6) δ 193.76, 151.51, 151.12, 143.10, 139.00, 137.63, 131.06, 129.88, 129.42, 129.37, 127.41, 126.50, 114.63, 108.03, 72.19, 58.04, 36.78, 26.48, 21.13, 21.08; IR (KBr, cm−1) νmax 1443, 1543, 1574, 1588, 1645, 2217, 2947, 3198, 3247, 3468 (br.); MS (m/z, EI, 70 eV): 380 (M+, 100%), 365 (18%), 352 (31%), 289 (49%), 91 (61%), 77 (92%). Anal. calcd for C24H20N4O (380.44): C, 75.77; H, 5.30; N, 14.73%. Found: C, 75.93; H, 5.41; N, 14.72%.
4.3 Synthesis of 6-acetyl-5-methyl-2-(4-methylphenyl)-7-phenyl-4,7-dihydropyrazolo[1,5-a]pyrimidine-3-carbonitrile 4f
Method A.
A mixture of 3-benzylidene-2,4-pentanedione (0.38 g, 2 mmol) and aminopyrazole 1b (0.40 g, 2 mmol) in DMF (0.5 mL) was heated under reflux for 0.5 h. The mixture was cooled and MeOH (20 mL) was added. The precipitate was filtered off and washed with MeOH (3 × 3 mL) to give compound 4f. Yield 60%, white solid, mp 258–260 °C (MeOH); 1H NMR (200 MHz, DMSO-d6) δ 7.63–7.82 (m, 2H, ArH), 7.33–7.52 (m, 3H, ArH), 7.12 (d, J = 8.0, 2H, ArH), 7.06 (d, J = 8.0, 2H, ArH), 6.17 (s, 1H, C(9)H), 2.60–2.84 (m, 2H, CH2), 2.20 (s, 3H, CH3), 2.12–2.34 (m, 2H, CH2), 1.74–2.05 (m, 2H, CH2); 13C NMR (125 MHz, DMSO-d6) δ 195.05, 151.76, 145.16, 142.76, 141.76, 139.49, 129.90, 129.16, 128.67, 128.36, 127.71, 126.42, 114.72, 108.96, 71.60, 59.86, 30.98, 21.36, 19.51; IR (KBr, cm−1) νmax 1443, 1549, 1569, 1581, 1624, 2225, 2938, 3028, 3178, 3239, 3285, 3502 (br.); MS (m/z, EI, 70 eV): 368 (M+, 67%), 353 (33%), 325 (21%), 291 (55%), 91 (37%), 43 (100%). Anal. calcd for C23H20N4O (368.43): C, 74.98; H, 5.47; N, 15.21%. Found: C, 75.00; H, 5.29; N, 15.07%.
Method B.
A mixture of PhCHO (0.23 g, 2 mmol) and 2,4-pentanedione (0.2 g, 2 mmol) in DMF (0.5 mL) was heated under reflux for 20 min. Then aminopyrazole 1b (0.4 g, 2 mmol) was added and the mixture was heated under reflux for an additional 20 min. The mixture was cooled and MeOH (20 mL) was added. The precipitate was filtered off and washed with MeOH (3 × 3 mL) to give pyrazolopyrimidine 4f (yield 65%). The melting point, EA, IR and NMR data are identical to those of the above sample. When ethyl acetoacetate (2 mmol) was used instead of 2,4-pentanedione, pyrazolopyrimidine 4e was obtained in 53% yield.
4.4 Synthesis of 7-methyl-5-oxo-2-phenyl-4,5-dihydropyrazolo[1,5-a]pyrimidine-3-carbonitrile 5
A mixture of N-(4-chlorophenyl)-3-oxobutanamide (0.42 g, 2 mmol), 3-aminopyrazole 1a (0.37 g, 2 mmol), PhCHO (0.23 g, 2 mmol) and 3 drops of conc. HCl in EtOH (3 mL) was heated under reflux for 30 min. The mixture was cooled, the precipitate was filtered off and washed with MeOH (3 × 3 mL) to give pyrazolopyrimidine 5 as a white solid. Yield 35%, mp > 320 °C (subl.) [Lit.:32 mp > 330 °C]; 1H NMR (200 MHz, DMSO-d6) δ 13.35 (br. s, 1H, NH), 7.85–8.05 (m, 2H, ArH), 7.45–7.65 (m, 3H, C(7)H), 5.88 (s, 1H, C(6)H), 2.33 (s, 3H, CH3); 13C NMR (125 MHz, DMSO-d6) δ 154.96, 153.16, 151.74, 146.78, 130.35, 130.29, 129.18, 126.97, 113.61, 98.90, 72.31, 18.67; IR (KBr, cm−1) νmax 1446, 1574, 1626, 1661, 2227, 2844, 2953, 2988, 3063 (br.), 3141, 3292; MS (m/z, EI, 70 eV): 250 (M+, 100%), 221 (51%), 193 (11%), 127 (27%), 62 (20%). Anal. calcd for C14H10N4O (250.26): C, 67.19; H, 4.03; N, 22.39%. Found: C, 67.34; H, 3.99; N, 22.40%.
4.5 Synthesis of 5,7-dimethyl-2-(4-methylphenyl)pyrazolo-[1,5-a]pyrimidine-3-carbonitrile 6
A mixture of 2,4-pentanedione (0.38 g, 2 mmol), PhCHO (0.23 g, 2 mmol) and aminopyrazole 1b (0.40 g, 2 mmol) in DMF (0.5 mL) was heated under reflux for 30 min. The mixture was cooled and MeOH (20 mL) was added. The precipitate of the product was filtered off and washed with MeOH (3 × 3 mL) to give product 6 as a yellow solid. Yield 48%, mp 229–231 °C (MeOH); 1H NMR (200 MHz, DMSO-d6) δ 7.91 (d, J = 8.0, 2H, ArH), 7.33 (d, J = 8.0, 2H, ArH), 7.09 (s, 1H, C(6)H), 2.72 (s, 3H, CH3), 2.56 (s, 3H, CH3), 2.37 (s, 3H, CH3); 13C NMR (125 MHz, DMSO-d6) δ 163.65, 155.70, 151.83, 147.58, 140.72, 130.13, 128.05, 127.24, 115.10, 112.32, 112.30, 24.76, 21.48, 16.88; IR (KBr, cm−1) νmax 1440, 1556, 1626, 2212, 2923, 3445; MS (m/z, EI, 70 eV): 262 (M+, 100%), 246 (9%), 234 (7%), 128 (9%), 90 (37%), 65 (43%). Anal. calcd for C16H14N4 (262.31): C, 73.26; H, 5.38; N, 21.36%. Found: C, 73.25; H, 5.49; N, 21.19%. When the same reaction was conducted in AcOH or EtOH–HCl, compound 6 was obtained in 90 and 94% yield.
4.6 Preparation of 4-methyl-2-(4-methylphenyl)-8-oxo-9-phenyl-4,5,6,7,8,9-hexahydropyrazolo[5,1-b]quinazoline-3-carbonitrile 9
A mixture of compound 8a (4.07 g, 10.7 mmol), EtBr (7.0 g, 4.75 mL, 64 mmol) and saturated aq. KOH solution (5.3 mL) in MeCN (30 mL) was heated under reflux for 1 h. The mixture was cooled and poured into 400 mL of brine. The resulting mixture was extracted with EtOAc (3 × 70 mL), dried with Na2SO4 and then evaporated to give a yellow precipitate, which was recrystallized from EtOH to give pure compound 9 as a pale-yellow solid, yield 60%, mp 207–209 °C (EtOH); 1H NMR (400 MHz, DMSO-d6) δ 7.61 (d, J = 8.0, 2H, ArH), 7.15–7.29 (m, 7H, ArH), 6.25 (s, 1H, C(9)H), 4.01–4.25 (m, 2H, CH2CH3), 2.92–3.02 (m, 1H, CH2), 2.64–2.74 (m, 1H, CH2), 2.29 (s, 3H, CH3), 2.18–2.36 (m, 2H, CH2), 1.96–2.06 (m, 1H, CH2), 1.77–1.92 (m, 1H, CH2); 1.36 (t, J = 6.8, 3H, CH2CH3); 13C NMR (100 MHz, DMSO-d6) δ 193.99, 152.63, 152.24, 143.32, 141.61, 139.63, 129.88, 128.97, 128.42, 128.14, 127.32, 126.81, 115.66, 109.87, 73.40, 57.70, 42.05, 36.04, 24.69, 21.38, 20.90, 15.23; IR (KBr, cm−1) νmax 1399, 1538, 1614, 1645, 2210, 2951, 3022; MS (m/z, EI, 70 eV): 408 (M+, 100%), 379 (65%), 331 (62%), 303 (40%), 91 (35%). Anal. calcd for C26H24N4O (408.49): C, 76.45; H, 5.92; N, 13.72%. Found: C, 76.50; H, 6.12; N, 13.67%.
4.7 Synthesis of 4-methyl-2-(4-methylphenyl)-8-oxo-9-phenyl-4,5,6,7,8,9-hexahydropyrazolo[5,1-b]quinazoline-3-carbothioamide 10
A slow stream of dry H2S was passed through a solution of compound 9 (0.5 g, 1.22 mmol) in a mixture of Et3N (10 mL) and DMF (10 mL) for 48 h (controlled by TLC) at 50 °C (bath temperature). The mixture turned green-yellow. After cooling, the mixture was poured into cold water (300 mL) and the pH was adjusted to 7.0 with diluted (3%) HCl. The precipitate formed was filtered off and washed with plenty of water. Compound 10 was obtained in 40% yield as a pale-yellow solid, mp 235–237 °C; 1H NMR (200 MHz, DMSO-d6) δ 10.17 (br. s, 1H, NH2), 9.84 (br. s, 1H, NH2), 7.56 (d, J = 8.0, 2H, ArH), 7.13–7.35 (m, 5H, ArH), 7.13 (d, J = 8.0, 2H, ArH), 6.23 (s, 1H, C(9)H), 4.00–4.35 (m, 2H, CH2CH3), 2.85–3.05 (m, 1H, CH2), 2.56–2.75 (m, 1H, CH2), 2.27 (s, 3H, CH3), 2.13–2.40 (m, 2H, CH2), 1.70–2.10 (m, 2H, CH2), 1.25 (t, J = 7.0, 3H, CH2CH3); 13C NMR (100 MHz, DMSO-d6) δ 197.77, 193.70, 153.56, 146.08, 142.44, 137.66, 134.56, 130.37, 129.23, 128.80, 128.05, 127.29, 127.16, 109.38, 108.16, 57.23, 41.81, 36.16, 25.24, 21.31, 21.14, 15.76; IR (KBr, cm−1) νmax 1360, 1423, 1539, 1593, 1622, 2928, 3059, 3223 (br.); MS (m/z, EI, 70 eV): 442 (M+, 100%), 413 (80%), 409 (48%), 379 (60%), 320 (37%), 256 (25%), 105 (23%), 73 (29%). Anal. calcd for C26H26N4OS (442.58): C, 70.56; H, 5.92; N, 12.66%. Found: C, 70.55; H, 6.10; N, 12.48%.
Acknowledgements
We thank V. V. Vashchenko, E. V. Vashchenko, V. I. Musatov (STC “Institute for Single Crystals” NAS of Ukraine, Kharkov) and Enamine Ltd for spectroscopic measurements. V. V. D. is grateful for financial support by the Russian Ministry of Education and Science (State Assignment to Higher Education Institutions, project no. 547).
Notes and references
- D. Dallinger, A. Stadler and C. O. Kappe, Pure Appl. Chem., 2004, 76, 1017 CrossRef CAS.
- C. O. Kappe, Acc. Chem. Res., 2000, 33, 879 CrossRef CAS PubMed.
- C. O. Kappe, Eur. J. Med. Chem., 2000, 35, 1043 CrossRef CAS PubMed.
- C. O. Kappe, QSAR Comb. Sci., 2003, 22, 630 CAS.
- M. Matache, C. Dobrota, N. D. Bogdan and D. P. Funeriu, Curr. Org. Synth., 2011, 8, 356 CrossRef CAS.
-
Suresh, J. S. Sandhu, ARKIVOC, 2012, (i), 66.
- M. A. Kolosov, V. D. Orlov, D. A. Beloborodov and V. V. Dotsenko, Mol. Diversity, 2009, 13, 5 CrossRef CAS PubMed.
- S. V. Vdovina and V. A. Mamedov, Russ. Chem. Rev., 2008, 77, 1017 CrossRef CAS.
- J.-P. Wan and Y. Liu, Synthesis, 2010, 3943 CrossRef CAS.
- Y. V. Sedash, N. Yu. Gorobets, V. A. Chebanov, I. S. Konovalova, O. V. Shishkin and S. M. Desenko, RSC Adv., 2012, 2, 6719 RSC.
-
V. A. Chebanov, S. M. Desenko and T. W. Gurley, Azaheterocycles Based on a,b-Unsaturated Carbonyls, Springer, 2008 Search PubMed.
- V. A. Chebanov, K. A. Gura and S. M. Desenko, Top. Heterocycl. Chem., 2010, 23, 41 CrossRef CAS.
- V. A. Chebanov and S. M. Desenko, Curr. Org. Chem., 2006, 10, 297 CrossRef CAS.
-
(a) M. A. Kolosov, O. G. Kulyk, M. J. K. Al-Ogaili and V. D. Orlov, Tetrahedron Lett., 2015, 56, 4666 CrossRef CAS;
(b) M. A. Kolosov, O. G. Kulyk, D. A. Beloborodov and V. D. Orlov, J. Chem. Res., 2013, 115 CrossRef CAS;
(c) M. A. Kolosov, V. D. Orlov, V. V. Vashchenko, S. V. Shishkina and O. V. Shishkin, Collect. Czech. Chem. Commun., 2007, 72, 1219 CrossRef CAS;
(d) M. A. Kolosov, O. G. Kulyk, E. G. Shvets and V. D. Orlov, Synth. Commun., 2014, 44, 1649 CrossRef CAS;
(e) M. A. Kolosov, O. G. Kulyk, M. J. K. Al-Ogaili and V. D. Orlov, Z. Naturforsch., B: J. Chem. Sci., 2012, 67b, 921 Search PubMed;
(f) M. A. Kolosov, M. J. K. Al-Ogaili, V. S. Parkhomenko and V. D. Orlov, Chem. Heterocycl. Compd., 2013, 49, 1484 CrossRef;
(g) M. A. Kolosov and V. D. Orlov, Chem. Heterocycl. Compd., 2008, 44, 1418 CrossRef CAS;
(h) M. A. Kolosov and V. D. Orlov, Chem. Heterocycl. Compd., 2007, 43, 1349 CrossRef CAS;
(i) M. A. Kolosov, O. G. Kulyk, O. I. Starchenko and V. D. Orlov, Chem. Heterocycl. Compd., 2013, 49, 1166 CrossRef CAS.
-
(a) M. A. Kolosov, D. A. Beloborodov, O. G. Kulyk and V. D. Orlov, J. Heterocycl. Chem., 2014, 51(S1), E89 CrossRef CAS;
(b) M. A. Kolosov, E. G. Shvets, O. G. Kulyk and V. D. Orlov, Chem. Heterocycl. Compd., 2015, 51, 1052 CrossRef CAS;
(c) M. A. Kolosov, M. J. K. Al-Ogaili, O. G. Kulyk and V. D. Orlov, Chem. Heterocycl. Compd., 2015, 51, 691 CrossRef CAS.
- P. B. Yu, C. C. Hong, C. Sachidanandan, J. L. Babitt, D. Y. Deng, S. A. Hoyng, H. Y. Lin, K. D. Bloch and R. T. Peterson, Nat. Chem. Biol., 2008, 4, 33 CrossRef CAS PubMed.
- D. W. Engers, A. Y. Frist, C. W. Lindsley, C. C. Hong and C. R. Hopkins, Bioorg. Med. Chem. Lett., 2013, 23, 3248 CrossRef CAS PubMed.
- M. H. Elnagdi, M. R. H. Elmoghayar and G. H. Elgemeie, Adv. Heterocycl. Chem., 1987, 41, 320 CrossRef.
- R. R. Frey, M. L. Curtin, D. H. Albert, K. B. Glaser, L. J. Pease, N. B. Soni, J. J. Bouska, D. Reuter, K. D. Stewart, P. Marcotte, G. Bukofzer, J. Li, S. K. Davidsen and M. R. Michaelides, J. Med. Chem., 2008, 51, 3777 CrossRef CAS PubMed.
- K. S. Atwal and S. Moreland, Bioorg. Med. Chem. Lett., 1991, 1, 291 CrossRef CAS.
- I. Drizin, M. W. Holladay, L. Yi, H. Q. Zhang, S. Gopalakrishnan, M. Gopalakrishnan, K. L. Whiteaker, S. A. Buckner, J. P. Sullivan and W. A. Carroll, Bioorg. Med. Chem. Lett., 2002, 12, 1481 CrossRef CAS PubMed.
-
S. M. Desenko and V. D. Orlov, Azageterotsikly na osnove aromaticheskih nepredelnykh ketonov (Azaheterocycles based on aromatic unsaturated ketones), Folio, Kharkov, Ukraine, ISBN: 966-03-0518-4, 1998 (in Russian) Search PubMed.
- V. A. Chebanov, V. E. Saraev, S. M. Desenko, V. N. Chernenko, I. V. Knyazeva, U. Groth, T. N. Glasnov and C. O. Kappe, J. Org. Chem., 2008, 73, 5110 CrossRef CAS PubMed.
- V. A. Chebanov, Y. I. Sakhno, S. M. Desenko, V. N. Chernenko, V. I. Musatov, S. V. Shishkina, O. V. Shishkin and C. O. Kappe, Tetrahedron, 2007, 63, 1229 CrossRef CAS.
- E. A. Muravyova, S. M. Desenko, R. V. Rudenko, S. V. Shishkina, O. V. Shishkin, Y. V. Sen’ko, E. V. Vashchenko and V. A. Chebanov, Tetrahedron, 2011, 67, 9389 CrossRef CAS.
- Y. I. Sakhno, S. V. Shishkina, O. V. Shishkin, V. I. Musatov, E. V. Vashchenko, S. M. Desenko and V. A. Chebanov, Mol. Diversity, 2010, 14, 523 CrossRef CAS PubMed.
- V. A. Chebanov, V. E. Saraev, S. V. Shishkina, O. V. Shishkin, V. I. Musatov and S. M. Desenko, Eur. J. Org. Chem., 2012, 5515 CrossRef CAS.
-
(a) B. C. O’Reilly and K. S. Atwal, Heterocycles, 1987, 26, 1185 CrossRef;
(b) K. S. Atwal, B. C. O’Reilly, J. Z. Gougoutas and M. F. Malley, Heterocycles, 1987, 26, 1189 CrossRef CAS;
(c) K. S. Atwal, G. C. Rovnyak, B. C. O’Reilly and J. Schwartz, J. Org. Chem., 1989, 54, 5898 CrossRef CAS.
- A. A. Bakibaev and V. D. Filimonov, J. Org. Chem. USSR, 1991, 27, 736 Search PubMed.
- M. A. Kolosov, V. D. Orlov and Yu. M. Vasil’eva, Kharkov Univ. Bull. Chem. Ser., 2006, 731, 69 CAS (in Russian).
- H. G. O. Alvim, T. B. Lima, A. L. de Oliveira, H. C. B. de Oliveira, F. M. Silva, F. C. Gozzo, R. Y. Souza, W. A. da Silva and B. A. D. Neto, J. Org. Chem., 2014, 79, 3383 CrossRef CAS PubMed.
- E. M. Zayed, S. A. S. Ghozlan and A.-A. H. Ibrahim, Monatsh. Chem., 1984, 115, 431 CrossRef CAS.
Footnote |
† Electronic supplementary information (ESI) available: Spectral data. See DOI: 10.1039/c6nj00336b |
|
This journal is © The Royal Society of Chemistry and the Centre National de la Recherche Scientifique 2016 |
Click here to see how this site uses Cookies. View our privacy policy here.