DOI:
10.1039/C6GC90086K
(Editorial)
Green Chem., 2016,
18, 5082-5085
Safety first
The principle that – “Substances and the forms of a substance used in a chemical process should be chosen so as to minimize the potential for chemical accidents, including releases, explosions, and fires” – has been important over the last 25 years, and remains so today. Breaking this, the 12th principle of Green Chemistry (Fig. 1), down into its constituent parts, one can see how it is already embedded both in a strong safety culture and in individual risk assessments in many industrial and academic laboratories, even if it is not always acknowledged as part of Green Chemistry. However, there remain areas where it may yet be further embraced, and, echoing a call that has been made many times before, there are elements of this principle and other aspects of Green Chemistry that still need to be seamlessly integrated into a default way in which all chemistry is taught and practised.
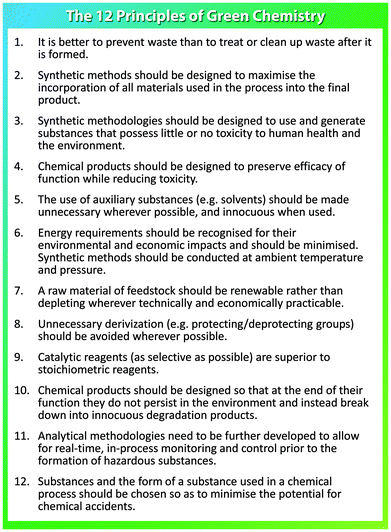 |
| Fig. 1 The 12 Principles of Green Chemistry. | |
Substances
When considering the substances used in a chemical process, there are many encouraging case studies, including the move away from the regular use of benzene,1 as a consequence of its health concerns, and away from carbon tetrachloride, as a consequence of its harm to the ozone layer, and from a legislation perspective, as a direct result of the Montreal protocol.2 Focus has since fallen on other chlorinated solvents, such as dichloroethane (DCE), which is listed as a Substance of Very High Concern and is included in the candidate list for authorisation under EU Registration, Evaluation, Authorisation & Restriction of Chemicals (REACH) legislation;3 and increasingly on chloroform and dichloromethane (DCM), solvents that are suspected of causing cancer.
Recent years have also seen a plethora of guides advising on the overall choice of solvents in the pharmaceutical industry,4 solvents for chromatography,5 and solvents for specific reaction transformations such as amide formation6 or reductive amination.7
When considering reagents, here too guides are becoming more widespread.8 For a range of different transformations, chemists are encouraged to think of the sustainability impact of different substance choices. However, this is far from a solved problem. Further exemplification of the scope and limitations of greener solvents and reagents (as well as a consistent assessment of their merits, failings and emphasis of their unknown qualities from a sustainability perspective) is required to enable further advances.
Forms of a substance
When considering the form of the substance used in a chemical process, many chemists will be familiar with the well documented advantages and increasing use of supercritical fluids, either as a reaction medium9 or for purification.10
However, choices about the forms of substances used may also manifest themselves in more mundane aspects of the day to day running of a laboratory. Such choices may be as simple as choosing 60% NaH in mineral oil by default, rather than the more flammable 95% dispersion; using pre-packed silica gel columns for chromatography rather than exposing chemists to the risks of particulate silica when packing their own columns; or ordering reagents in solution in preferred solvents, for example ordering Grignard reagents in 2-methyl tetrahydrofuran (2-MeTHF) rather than tetrahydrofuran (THF),11 or propane phosphonic acid anhydride (T3P) in ethyl acetate rather than dimethyl formamide.
If the form of a substance is presumed to include its pack size, then this principle may even be at play in prosaic decisions about the quantities of materials bought. For example, chemists should consider minimising the environmental and financial costs of storage and disposal by minimising quantities bought, and there are some arrangements where chemical suppliers supply only the exact amounts to be needed for a given reaction. It may even extend to choices such as purchasing easily transferred pellets of solid carbon dioxide rather than large blocks which may cause ergonomic issues on transfer and use.
Further consideration of what is actually required of a given process may yet lead to the increased uptake of supercritical fluids or solventless technologies like ball-milling12 which in some circumstances may bring sustainability advances.
Releases
The potential impact of solvents or reagents on the air or aqueous environment in the event of accidental release are considered in the Globally Harmonised System (GHS) phrases associated with each chemical. These GHS phrases are factored into many of the guides previously mentioned when the overall sustainability credentials of these substances are assessed. It should be remembered that the full life cycle of any substance should be considered when assessing its risk of release. This includes the circumstances under which is it prepared, and/or disposed of, where certain substances (such as precious metals which may leach) or iodine containing compounds (which can degrade to give a purple plume if incinerated) may require special handling.
Explosions
Chemists are used to choosing reagents to minimise the risk of explosions. For example, trimethylsilyldiazomethane has replaced diazomethane in many methylations.13 However there are other potentially explosive reagents that are still widely used when safer alternatives exist. One example from the pharmaceutical industry is the continuing use of triazole coupling reagents: HATU, HBTU, HOAt, etc. These are widely regarded as innocuous, yet not only does their high nitrogen content render them potentially explosive, but in many cases they also give rise to toxic byproducts. These reagents remain popular because amide bond formation, despite being one of the first organic reactions most chemists learn, is far from a solved problem,14 and it has long been acknowledged within the industry that greener, more general amide coupling reactions are required.15 However, despite a number of encouraging developments in the field of amide synthesis over the last decade,16 there is still a need for robust reagents that work on a wide range of substrates to effect what is still one of the most common transformations.
In one sense, the continuing use of triazole coupling reagents in early stage drug discovery highlights a wider pharmaceutical industry issue. Drug discovery chemists need to have a better understanding of the challenges their process development colleagues face, so that the practical implications of process considerations such as heat of reaction and potential for “runaway” reactions can be addressed at an early stage when appropriate to do so, allowing for greater resource to be placed on trickier problems during scale up.
Fires
A common cause of laboratory fires is the mishandling of pyrophoric precious metal catalysts. The last few years have seen considerable efforts to minimise organic synthesis's reliance on precious metals. In some cases transition metal catalysts can be avoided altogether and chemists can revert to older, SNAr type chemistry to form heteroaryl C–N17 and C–O18 bonds. In other cases, while both nickel and palladium catalysts can be a fire risk, there are several drivers for an increasing move from Pd to Ni catalysis. One consideration is the relative carbon footprint of different metals. For example Ni has a carbon footprint of 7.1 CO2 equivalents, whereas the lower abundance of Pd means that it has a carbon footprint of 6649 CO2 equivalents.19
Future considerations minimising the risk of releases, explosions, fires and other accidents will likely focus on how the substance will be used, with particular emphasis on the interplay of chemistry and engineering. One example is recent work on fluorination in flow chemistry.20 On initial assessment, elemental fluorine may not seem the “greenest” of reagents. However, when one considers that nearly all other fluorinating reagents will at some stage have required the use of elemental fluorine in their manufacture, then with appropriate flow engineering controls the atom efficiency of such an approach may yet recommend this as a technology.
Conclusion
The aspects of the 12th principle of Green Chemistry discussed above can also be considered within the framework of the hierarchy of controls (Fig. 2). As I believe we should be moving towards Green Chemistry being as natural a part of day to day laboratory practice as safety is, it is within this widely accepted framework that I would lay out the next steps that need to be taken.
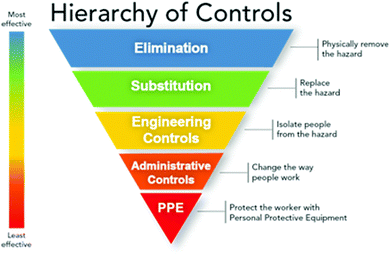 |
| Fig. 2 Hierarchy of controls – most effective at the top (Image courtesy of NIOSH).21 | |
Some solvents and reagents need to be eliminated, and to the already generally accepted blacklist of benzene and carbon tetrachloride, should be added the chlorinated solvents dichloroethane, chloroform, and, for the majority of purposes, dichloromethane. More aspirationally, the dipolar aprotic solvents dimethyl formamide, dimethyl acetamide and N-methyl pyrrolidone should also be included.
Elimination of unwanted substances will often necessitate their substitution. “Drop-in” like for like replacements are not always possible, but published guides can go some way to facilitating many safer swaps. A wealth of published precedent as to substrate scope has been built up around some greener protocols, such as T3P for simple amide couplings, picoline borane for reductive aminations, and ethanol/water or IPA/water for Suzuki reactions, some of the most common reactions in drug discovery. Accordingly, in future these should be “substituted” as the default choice for these reactions, with less sustainable alternatives only being resorted to where there is a clear reason to believe they will not work, or if a first attempt has failed. For any common transformations, where a lack of sustainable options are found, that should drive future research to find alternatives.
Engineering controls have their place in Green Chemistry. Flow chemistry permits the safe handling of reagents or reactive intermediates that may otherwise not be acceptable – reagents or intermediates that may permit more efficient routes to desired substrates, or even, in the case of flow photochemistry for example, substrates that could not be accessed any other way. In future the potential of flow chemistry should be fully exploited from the earliest stages of drug discovery through to commercial manufacture.
Finally, from an administrative perspective, I would like to see more aspects of green chemistry afforded the respect that is given to safety. To the vast majority of chemists it would be inconceivable not to choose the safest precedented option for a reaction…in future that consideration should be expanded to be the most sustainable precedented option.
Notes and references
- M. T. Smith, Annu. Rev. Public Health, 2010, 31, 133–148 CrossRef PubMed.
-
http://ozone.unep.org/en/handbook-montreal-protocol-substances-deplete-ozone-layer/5
.
- ECHA Registered Substances Database (http://echa.europa.eu/web/guest/information-on-chemicals/registered-substances).
-
(a) K. Alfonsi, J. Colberg, P. J. Dunn, T. Fevig, S. Jennings, T. A. Johnson, H. P. Kleine, C. Knight, M. A. Nagy, D. A. Perry and M. Stefaniak, Green Chem., 2008, 10, 31–36 RSC;
(b) R. K. Henderson, C. Jimenez-Gonzalez, D. J. C. Constable, S. R. Alston, G. G. A. Inglis, G. Fisher, J. Sherwood, S. P. Binks and A. D. Curzons, Green Chem., 2011, 13, 854–862 RSC;
(c)
http://www.acs.org/gcipharmaroundtable, accessed 13 Jan 2016;
(d) D. Prat, O. Pardigon, H. W. Flemming, S. Letestu, V. Ducandas, P. Isnard, E. Guntrum, T. Senac, S. Ruisseau, P. Cruciani and P. Hosek, Org. Process Res. Dev., 2013, 17, 1517–1525 CrossRef CAS;
(e) D. Prat, A. Wells, J. Hayler, H. Sneddon, C. R. McElroy, S. Abou-Shehada and P. J. Dunn, Green Chem., 2016, 18, 288–296 RSC and D. Prat, A. Wells, J. Hayler, H. Sneddon, C. R. McElroy, S. Abou-Shehada and P. J. Dunn, Green Chem., 2015, 17, 4848–4848 Search PubMed;
(f) L. J. Diorazio, D. R. J. Hose and N. K. Adlington, Org. Process Res. Dev., 2016, 20, 760–773 CrossRef CAS;
(g) C. M. Alder, J. D. Hayler, R. K. Henderson, A. M. Redman, L. Shukla, L. E. Shuster and H. F. Sneddon, Green Chem., 2016, 18, 3879–3890 RSC.
-
(a) D. S. MacMillan, J. Murray, H. F. Sneddon, C. Jamieson and A. J. B. Watson, Green Chem., 2012, 14, 3016–3019 RSC;
(b) J. P. Taygerly, L. M. Miller, A. Yee and E. A. Peterson, Green Chem., 2012, 14, 3020–3025 RSC;
(c) D. G. Drueckhammer, S. Q. Gao, X. Liang and J. Liao, ACS Sustainable Chem. Eng., 2012, 87–90 Search PubMed;
(d) A. N. Hobbs and R. J. Young, Drug Discovery Today, 2013, 18(3–4), 148–154 CrossRef CAS PubMed;
(e) F. M. Chardon, N. Blaquiere, G. M. Castanedo and S. G. Koenig, Green Chem., 2014, 16, 4102–4105 RSC.
- D. S. MacMillan, J. Murray, H. F. Sneddon, C. Jamieson and A. J. B. Watson, Green Chem., 2013, 15, 596–600 RSC.
- F. I. McGonagle, D. S. MacMillan, J. Murray, H. F. Sneddon, C. Jamieson and A. J. B. Watson, Green Chem., 2013, 15, 1159–1165 RSC.
-
(a) J. P. Adams, C. M. Alder, I. Andrews, A. M. Bullion, M. Campbell-Crawford, M. G. Darcy, J. D. Hayler, R. K. Henderson, C. A. Oare, I. Pendrak, A. M. Redman, L. E. Shuster, H. F. Sneddon and M. D. Walker, Green Chem., 2013, 15, 1542–1549 RSC;
(b)
http://www.reagentguides.com/
.
- C. A. Eckert, Nature, 1996, 383(6598), 313–318 CrossRef CAS.
- D. R. Gere, Science, 1983, 222(4621), 253–259 CAS.
- A. Kadam, M. Nguyen, M. Kopach, P. Richardson, F. Gallou, Z.-K. Wan and W. Zhang, Green Chem., 2013, 15, 1880–1888 RSC.
- A. Stolle, T. Szuppa, S. E. S. Leonhardt and B. Ondruschka, Chem. Soc. Rev., 2011, 40, 2317–2329 RSC.
- D. Seyferth, A. W. Dow, H. Menzel and T. C. Flood, J. Am. Chem. Soc., 1968, 90, 1080–1082 CrossRef CAS.
- V. R. Pattabiraman and J. W. Bode, Nature, 2011, 480, 471–479 CrossRef CAS PubMed.
- D. J. C. Constable, P. J. Dunn, J. D. Hayler, G. R. Humphrey, J. L. Leazer Jr., R. J. Linderman, K. Lorenz, J. Manley, B. A. Pearlman, A. Wells, A. Zaks and T. Y. Zhang, Green Chem., 2007, 9, 411–420 RSC.
- R. Bandichhor, A. Bhattacharya, L. Diorazio, P. Dunn, K. Fraunhoffer, F. Gallou, J. Hayler, M. Hickey, B. Hinkley, D. Hughes, L. Humphreys, B. Kaptein, S. Mathew, L. Oh, P. Richardson, T. White and S. Wuyts, Org. Process Res. Dev., 2014, 18(7), 863–874 CrossRef CAS.
- K. Walsh, H. F. Sneddon and C. J. Moody, ChemSusChem, 2013, 6(8), 1455–1460 CrossRef CAS PubMed.
- K. Walsh, H. F. Sneddon and C. J. Moody, RSC Adv., 2014, 4, 28072–28077 RSC.
- Outsmarting the Shortage: The Emergence of Base Metal Catalysis in Pharma, J. Chris McWilliams, http://www.acs.org/content/acs/en/acs-webinars/technology-innovation/metal-catalysis/video.html (last accessed 1st June, 2016).
- N. J. Willis, C. A. Fisher, C. M. Alder, A. Harsanyi, L. Shukla, J. P. Adams and G. Sandford, Green Chem., 2016, 18, 1313–1318 RSC.
-
http://www.cdc.gov/niosh/topics/hierarchy/
.
|
This journal is © The Royal Society of Chemistry 2016 |