DOI:
10.1039/C6FO01721E
(Review Article)
Food Funct., 2017,
8, 39-63
Astaxanthin in cardiovascular health and disease: mechanisms of action, therapeutic merits, and knowledge gaps
Received
27th November 2016
, Accepted 1st December 2016
First published on 2nd December 2016
Abstract
Cardiovascular disease is the main contributor to morbidity and mortality worldwide. Based on its unique chemical features, the xanthophyll carotenoid astaxanthin is being proposed as a suitable preventive and therapeutic agent in cardiovascular disease. This review focuses on recent advances in astaxanthin research relevant to cardiovascular health and disease, i.e. its direct antioxidant, indirect antioxidant, anti-inflammatory, anti-hypertensive, anti-diabetic, renoprotective, lipid-lowering and anti-atherosclerotic activities in vitro and in vivo. Disparities in the biological activities and health benefits of astaxanthin observed in vitro (strong evidence), in animals (moderate evidence), and in humans (weak evidence) and the variety of astaxanthin sources hamper efforts to establish areas of astaxanthin application in human health care. A list of knowledge gaps and experimental pitfalls is proposed to overcome some of the short-comings in astaxanthin research.
Introduction
Cardiovascular and other non-communicable diseases (NCDs) have become the primary health concern for most countries around the world. Currently, more than 36 million people globally die from NCDs each year, accounting for 63% of annual global deaths.1 Among NCDs, cardiovascular disease (CVD) is the main contributor to morbidity and mortality in many societies and accounted for an estimated 16.7 million deaths globally in 2010. This number is projected to increase to 23.3 million by 2030 and will have a pronounced impact particularly in low- and middle-income countries where 80% of CVD-associated deaths occur.2,3 The financial burden of CVD is equally staggering. The price of not investing in CVD prevention and treatment has been projected to reach up to $47 trillion worldwide in the next 25 years.3
Most CVDs, such as myocardial infarction (heart attack), localized cerebral ischemia (stroke) and peripheral vascular disease are secondary to atherosclerosis. Briefly, atherosclerosis can be described as an un-physiological, local thickening of the vessel walls, leading to the partial occlusion of the arterial lumen (Fig. 1). Atherosclerosis begins with the damage and subsequent impairment of the functional integrity of the vascular endothelium. Contributing events have been identified, such as high glucose levels (Diabetes mellitus [DM]), mechanical stress (hypertension), infections of the vessel wall (viruses and bacteria) and smoking (circulating toxins). Upon exposure to these stimuli, endothelial cells of the vessel wall become dysfunctional, resulting in increased release of pro-inflammatory mediators and elevated production of free radicals and other reactive oxygen species (ROS). At the same time, the redox balance of endothelial cells becomes further compromised, for example due to a reduction in the expression of eNOS (responsible for the release of the vasodilator nitric oxide) and superoxide dismutase (SOD; responsible for the conversion of superoxide to the less reactive hydrogen peroxide). Whereas healthy arteries are able to egress oxidized low-density lipoprotein (oxLDL) that enters the vessel wall into the lymphatic system, the removal of oxLDL particles becomes compromised once the integrity of the endothelial barrier is damaged. During the early stage of atherosclerosis, macrophages recruited to the site of injury become overloaded with oxLDL and turn into foam cells, a hallmark of fatty streaks. With the infiltration and proliferation of smooth muscle cells, fatty streaks are transformed into fibrous plaques. Atherosclerotic plaques can cause clinical symptoms by limiting the blood supply of an organ. Most dangerous are plaque raptures which often trigger the formation of a thrombus resulting in the complete occlusion of the arterial lumen seen, for instance, in myocardial infarction and stroke.4–9
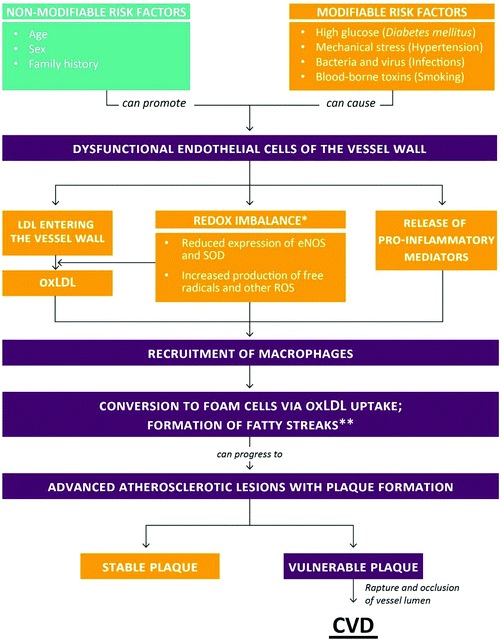 |
| Fig. 1 Risk factors for and stages of atherosclerosis leading to CVD (for details see text). *Some authors suggested oxidative stress as secondary to inflammation. **Fatty streaks usually do not result in clinical complications and can undergo regression. | |
Currently, the general consensus is that chronic, low-grade inflammation and oxidative stress are involved in both the initiation and progression of atherosclerosis, although some authors have suggested that oxidative stress is probably the consequence of many diseases rather than their cause.4,7,10 With respect to atherosclerosis, Stocker and Keaney proposed an “oxidative response to inflammation” model.11 Yet, despite compelling evidence for the occurrence of oxidative damage in atherosclerotic tissue, such as lipid peroxidation, intervention studies with dietary antioxidants (vitamin C, vitamin E, β-carotene) in primary and secondary prevention of CVD have been disappointing.12–14 The use of efficacious drugs, e.g. statins, calcium channel blockers, diuretics15–17 is often limited due to their high costs, the occurrence of unfavorable side-effects or patients’ preference.18–20 Hence, the development of new and/or refined intervention strategies is urgently warranted to provide further treatment options against the onset and progression of CVDs.
Because of its unique chemical features, the xanthophyll carotenoid astaxanthin has been proposed as a suitable preventive and therapeutic agent in CVD21–23 and other diseases.24 The purpose of this review is to critically assess the potential of astaxanthin (from natural and synthetic sources) in modifying CVD onset and progression. Since the first 1991 report by Miki on the antioxidant properties of astaxanthin in animals,25 the number of publications addressing actions of this carotenoid in vitro and in vivo has grown substantially. Thus, this paper will focus on recent developments in astaxanthin research relevant to cardiovascular health and disease, and try to identify gaps in our knowledge pertaining to the biological activities of this unique biomolecule.
Astaxanthin: structure, sources and bioavailability
Chemical structure
Based on the presence of oxygen in their chemical structure, carotenoids are typically classified into carotenes (non-oxygenated compounds, e.g., β-carotene and lycopene) and xanthopylls (oxygenated compounds, e.g., lutein, astaxanthin, canthaxanthin, and zeaxanthin). Astaxanthin (3,3′-dihydroxy-β,β′-carotene-4,4′-dione; CAS no. 472-61-7) is an orange-red, fat-soluble pigment with the molecular formula C40H52O4 and molar mass of 596.84 g mol−1. Astaxanthin consists of two oxygenated β-ionone-type ring systems linked by a chain of conjugated double bonds (polyene chain). Oxygen is present in the ring system as both hydroxyl and keto groups. Due to the presence of two stereogenic carbons (3 and 3′) on the β-ionone moieties, astaxanthin can exist in three stereoisomeric forms, two chiral [(3S,3′S) and (3R,3′R)] and one meso (3R,3′S) (Fig. 2). Whereas the three optical stereoisomers exist in nature in variable ratios, synthetic astaxanthin is an equimolar mixture of the racemate and the meso form. In addition, astaxanthin may exist in trans and cis (E and Z) geometrical isomers, depending on the configuration of the double bonds of the polyene chain. All-trans astaxanthin (all-E-astaxanthin) presents the predominant form, although at least two cis-isomers (Z-isomers) also occur in nature and synthetic preparations.26–28
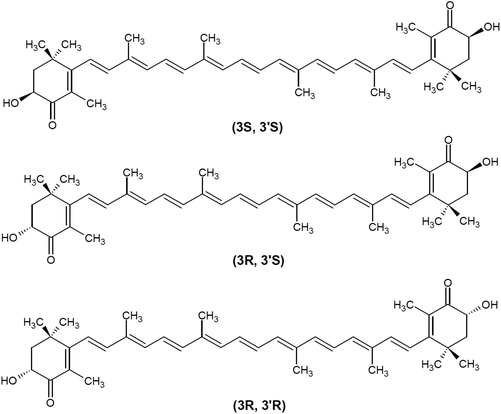 |
| Fig. 2 Chemical structure of main optical astaxanthin isomers. | |
Natural and synthetic sources
Astaxanthin occurs naturally in a wide variety of living organisms and is visually known to most people for the pink color of flamingo feathers and the red flesh of wild salmon. Being unable to synthesize astaxanthin themselves, flamingos and wild salmon depend on their diet to meet their astaxanthin requirements. The presence of carotenoids in tissue reflects the animal's dietary sources along the food chain where astaxanthin can be found in yeast, microalgae, copepods, krill, complex plants, snails and some birds (Table 1).22,28
Table 1 Natural sources of astaxanthin in animals22,27–29,32
Species |
Examples |
Primary stereoisomer |
Fishes |
Salmon |
• 3S,3′S (47.1–90.0%) |
• Atlantic (Salmo salar) |
• Pacific (Onchorhynchus species) |
Marine organisms |
Crustaceans |
• 3R,3′R |
• Shrimp (Pandalus borealis) |
• Crabs |
• Lobster |
• Antarctic krill (Euphausia superba) |
Snails |
• Pomaceae canaliculata eggs |
|
Birds |
• Flamingo feathers |
|
• Quail retina |
As humans are unable to synthesize carotenoids, they, too, need to obtain them from their diet. Main intake of astaxanthin in humans is from seafood with wild sockeye salmon, for example, providing 26–38 mg kg−1 flesh.22,28 Wild salmon predominantly ingest astaxanthin from copepods and krill, which contain mostly the 3R,3′R and 3S,3′S astaxanthin enantiomers and only little of the 3R,3′S form. With respect to the distributions of the optical isomers of all-trans astaxanthin in wild salmon, ranges of 47.1–90.0% for the 3S,3′S enantiomer, 7.7–15.2% for the 3R,3′R enantiomer, and 0–8.6% for the 3R,3′S meso form have been reported for Atlantic and Pacific salmon.29
In 2008, already more than 70% of the world's salmon production was farmed.30 As salmon grown in aquaculture are unable to obtain astaxanthin from the food chain, commercial astaxanthin products are added to the fish feed as source of pigment to obtain the characteristic orange-red color of the flesh. The origin of astaxanthin sources can be easily monitored by HPLC analysis of the isomers present in the flesh. Salmon fed yeast-derived astaxanthin in their diet, for example, show primarily the 3R,3′R isomer, whereas salmon supplemented with synthetic astaxanthin show an abundance of the 3R,3′S isomer. Hence, wild salmon flesh contains only little 3R,3′S, while farmed salmon have either very high levels of synthetic 3R,3′S or 3R,3′R from yeast.31
Commercially, astaxanthin can be produced from microalgae (e.g. Haematococcus pluvialis J. Von Flotow, 1844 harvested during the “red stage” of cultivation, milled (to increase bioavailability) and dried or astaxanthin-rich oleoresin, obtained by different extractions methods such as ethanol- or supercritical CO2-extraction), yeasts (e.g. mutated Xanthophyllomyces dendrorhous), bacteria (e.g. dried cells of a modified strain of Paracoccus carotinifaciens, an aerobic Gram-negative bacterium isolated from the soil, and its fermentation medium) or via chemical synthesis.27,33–35 Astaxanthin sourced from the microalgae H. pluvialis is the only one currently authorized for direct human consumption, while all the sources are authorized for use in salmon and trout feeding under different condition.33
In order to rate the mechanism(s) of action and therapeutic efficacy of astaxanthin, it is important to be aware of the compositional differences between astaxanthin products originating from H. pluvialis, X. dendrorhous (P. rhodozyma), P. carotinifaciens or from chemical synthesis (Table 2). As mentioned above, synthetic astaxanthin is a mixture of the racemic and the meso forms. In terms of geometrical isomers, all-E (trans) astaxanthin is the dominant from in synthetic astaxanthin whereas the Z-(cis) isomers usually occur in minor amounts (Table 3 and Fig. 3).
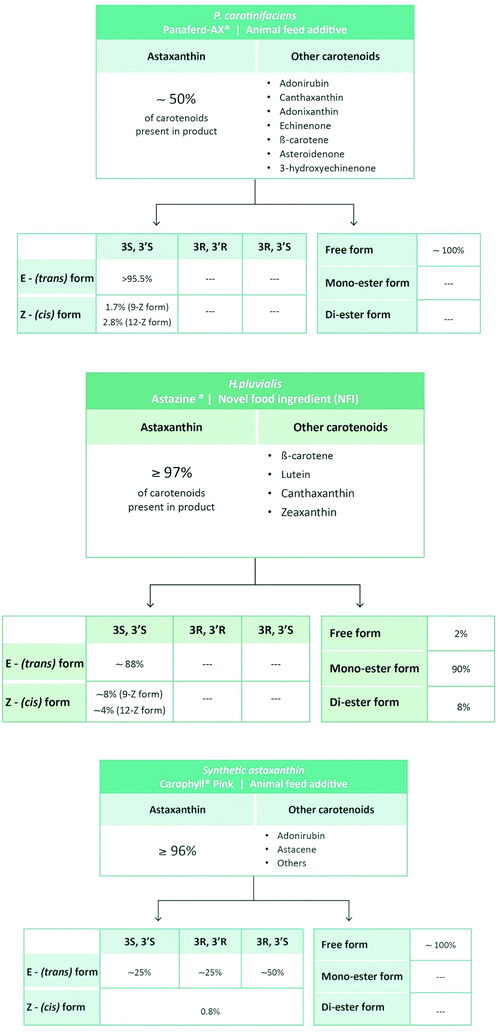 |
| Fig. 3 Carotenoid composition of synthetic and natural astaxanthin products.36,37 Note that we do not describe two products listed in Table 2 because they are derived from the fermentation Saccharomyces cerevisiae (yeast) and Paracoccus carotinifaciens (Phaffia rhodozyma), therefore containing approximately 30% of carotenoids other than astaxanthin (see http://ec.europa.eu/food/fs/sc/scan/out111_en.pdf). | |
Table 2 Natural and synthetic sources of astaxanthin22,27,28,32
Species |
Examples |
Primary Stereoisomer |
Microalgae |
• Haematococcus pluvialis |
• 3S,3′S (esterified form) |
Yeast |
• Xanthophyllomyces dendrorhous (formerly Phaffia rhodozyma) |
• 3R,3′R (free form) |
Bacteria |
• Paracoccus carotinifaciens |
• 3S,3′S (free form) |
|
• Synthetic astaxanthin |
• 3R,3′S (free form) |
Table 3 Studies reporting plasma values and distribution of astaxanthin isomers in humans
Authors |
Year |
Form of astaxanthin |
Dosage |
Plasma levels (μmol l−1) |
Comment |
Values derived from graph.
|
Østerlie et al.53 |
2000 |
• Synthetic |
• 100 mg |
• 2.2 |
• Increased relative proportion of Z-isomers due to selective uptake process |
• Free |
• Single dose |
|
|
• 74% all-E-astaxanthin |
|
|
|
• 9% 9Z-astaxanthin |
|
|
|
• 17% 13Z-astaxanthin |
|
|
|
• 3S,3′S:3R,3′S:3R,3′R = 1 : 2 : 1 |
|
|
|
Coral-Hinostroza et al.48 |
2004 |
• Synthetic |
• 10 mg |
• 0.13 |
• Dose-response non-linear |
• Astaxanthin di-esters |
• 100 mg |
• 0.47 |
• Selective accumulation of 3R,3′R form |
• 95.2% all |
• Single dose |
|
• Selective accumulation of Z-isomers |
• 1.2% 9Z |
|
|
• Astaxanthin ester hydrolysis required for absorption |
• 3.6% 13Z |
|
|
|
• 3S,3′S:3R,3′S:3R,3′R = 19.7 : 49.0 : 31.3 |
|
|
|
Rüfer et al.49 |
2008 |
• Wild salmon (WS): |
• 1.25 mg (from 250 g salmon flesh) |
• Day 3: |
• Astaxanthin isomer pattern in plasma resembles that of salmon |
• 3S,3′S:3R,3′S:3R,3′R = 69.7 : 2.1 : 28.2 |
• 28 days |
• 0.027 (WS) |
• Selective absorption of 3S,3′S isomer |
• Aqua-cultured salmon (AS): |
|
• 0.042 (AS) |
|
• 3S,3′S:3R,3′S:3R,3′R = 31.9 : 47.8 : 20.3 |
|
• Day 10: |
|
|
|
• 0.034 (WS) |
|
|
|
• 0.047 (AS) |
|
|
|
• Day 28: |
|
|
|
• 0.039 (WS) |
|
|
|
• 0.043 (AS) |
|
Okada et al.43 |
2009 |
• Natural (H. pluvialis) |
• 48 mg |
• 0.19 |
— |
• 70% mono-ester |
• Single dose |
|
|
• 28% di-ester |
|
|
|
• 2% free |
|
|
|
Park et al.54 |
2010 |
• Natural (H. pluvialis) |
• 2 mg |
• 2 mg: |
— |
|
• 8 mg |
• 0.095a |
|
|
• 4 and 8 weeks |
(4 weeks) |
|
|
|
• 0.09a (8 weeks) |
|
|
|
• 8 mg: |
|
|
|
• 0.13a (4 weeks) |
|
|
|
• 0.115a (8 weeks) |
|
In contrast to synthetic astaxanthin, natural astaxanthin products also contain other carotenoids. In case of the commercially available product derived from P. carotinifaciens, carotenoids other than astaxanthin can make up to 50% of the total carotenoids present in the product (Fig. 3).36 So far, little is known as to whether the different carotenoids present in natural astaxanthin products act synergistically, in an additive manner or possibly cancel each other out.
Obtaining distinct analytical and biological effect profiles of each astaxanthin product is further complicated by the fact that commercially available natural astaxanthin supplements and additives are not only usually stabilized with antioxidants of natural (e.g.D-alfa-tocopherol) or synthetic origin (e.g.DL-alfa-tocopherol) but also often standardized with different natural oils in order to meet the label indications, thus giving each product a specific lipophilic profile. Obviously, the significant differences in the composition of natural astaxanthin products make comparisons with respect to bioequivalence (pharmacokinetics) and efficacy (pharmacodynamics) extremely challenging.
Bioavailability
To date, more than 500 carotenoids have been identified. Of the approximately 50 carotenoids present in food, only 20 are absorbed in the intestine and distributed to organs and body tissues.27,38,39 Owing to their hydrophobic nature, intestinal absorption of carotenoids is similar to the one of dietary lipids. In brief, following their incorporation into mixed lipid micelles in the lumen, carotenoids are taken up into the intestinal mucosa lining by passive diffusion and incorporated into chylomicrons to be released subsequently into the lymphatic system. Once the digestion of chylomicrons by lipoprotein lipase occurred in the liver, carotenoids assimilate with lipoproteins, especially LDL, to be further distributed to other tissues. Carotenoids are mainly accumulated in liver and adipose tissue but have also been detected in comparatively high amounts in the adrenal gland, corpus luteum, retina, skin and testes. The absorption of carotenoids is influenced by their chemical properties and a variety of diet- and non-diet-related parameters (such as fat content, fat type, age and gender).38,40–42 With respect to astaxanthin (but also many other carotenoids), its bioavailability is determined by three levels of chemical complexity: (1) the level of esterification, (2) the optical stereoisomer form and (3) the geometrical isomer form (Fig. 4). In addition, concomitant food intake seems to greatly increase the absorption and smoking to dramatically reduce the half-life of astaxanthin.43
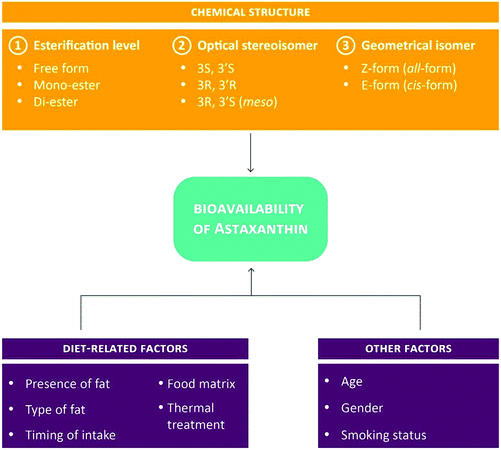 |
| Fig. 4 Parameters affecting the bioavailability of astaxanthin.28,42,43 | |
The absorption of astaxanthin from different sources has been demonstrated in animals, mainly in mice and rats but also other species (e.g. dogs and cats)44–47 as well as in humans, albeit only in 5 rather small intervention studies (with participant numbers ranging from 3 to 14 subjects) using not only different astaxanthin forms (astaxanthin from wild salmon or farmed salmons (1 study), astaxanthin from H. pluvialis (2 studies) and synthetic astaxanthin (2 study)) but also different regiments (single dose or repeated dosages). Furthermore, various authors tested significantly different dosages, with only 3 studies using dietary dosages ranging from 1–4 mg per day and 2 studies (with a total of 6 subjects), assessing synthetic astaxanthin at 100 mg per day. Of note, none of the studies was designed in crossover, making the comparison of intra-treatment effects difficult. Furthermore, the reported statistical significances have to be treated with caution, due to the small number of subjects included in these bioavailability studies. Table 3 summarizes plasma values and distribution of astaxanthin isomers in humans. These data indicate (1) a non-linear dose-response for plasma astaxanthin concentrations in human subjects (possibly due to a saturation mechanism at higher doses), (2) a lower bioavailability of esterified astaxanthin in humans (possibly due to the required hydrolysis before uptake) and (3) the selective accumulation of Z(cis)-isomers. Results regarding the absorption of optical isomers are conflicting, with one study each reporting the selective accumulation of either 3R,3′R astaxanthin (in case of synthetic astaxanthin die-ester administration) or the 3S,3′S form (in case of salmon intake).48,49 Moreover, in the two studies using repeated dosages of astaxanthin concentrations attainable by normal dietary habits, only little variation in plasma concentrations has been observed for the entire study duration, suggesting that steady state levels were reached early. Little is known regarding the metabolism and excretion of astaxanthin in vivo.47 Whereas the C9, C′9 cleavage of astaxanthin and reduction of the polyenic 7,8 double bond are common to humans and rats, their metabolisms have also been shown to react differently (e.g. CYP activation profile) to astaxanthin intake.50,51
It is worth pointing out, that the reported plasma values are not only within the range of the nano molar to low micro molar range used in various cell culture studies (details reported below), but also correspond to those detected in animal tissues.44,52 The consistency between in vitro, plasma and tissue concentrations of astaxanthin provide the promising prospect that astaxanthin may exert cardiovascular-protective effects not only in cell culture but, indeed, also in vivo.
Astaxanthin: CVD-relevant biological functions and mechanisms of action in vitro and in vivo
(Direct) antioxidant activity – protection from lipid peroxidation and LDL oxidation
As an attribute of life in an aerobic atmosphere, humans and other animals are exposed to a variety of reactive species (RS) with potentially damaging effects on important biomolecules, such as carbohydrates, DNA, lipids and proteins.55,56 In 1991, Sies defined oxidative stress “as a disturbance in the prooxidant–antioxidant balance in favor of the former, leading to potential damage”.57 This notion has now evolved into the “Redox Code”.58 Of note, not all damage induced by oxidative stress is oxidative damage. For instance, oxidative stress may cause indirect damage by altering levels of potentially harmful metabolites or signaling molecules.4 The antioxidant activity of astaxanthin has been assessed by several groups in different test-tube systems. Astaxanthin showed superior antioxidant activity compared to other dietary antioxidants in some but not all studies (Table 4). Based on these and other results, it has been suggested that astaxanthin and other carotenoids are probably best suited for the removal of singlet oxygen and peroxyl radicals. Astaxanthin's potential for quenching singlet oxygen largely depends on a direct energy transfer between the two molecules. In contrast, the prevention of lipid peroxidation by astaxanthin involves the scavenging, and thus deactivation, of peroxyl radicals by forming resonance-stabilized, carbon-centered radical adducts.59 Mechanistic details of singlet oxygen quenching, peroxyl radical deactivation and scavenging of other RS by astaxanthin haven been described by several authors.25,59–61 In 2013, Capelli et al. published a direct comparison between the antioxidant capacity of natural vs. synthetic astaxanthin.62 In a first set of experiments, the scavenging potential of astaxanthin against two RS, i.e. superoxide and hydroxyl radical, was tested. Natural astaxanthin showed not only a 14–65× higher antioxidant activity compared with vitamin C, vitamin E, β-carotin and pycnogenol, but was also 20× more efficient than synthetic astaxanthin. However, from the information provided it is unclear whether the presented activities refer to the scavenging of superoxide, hydroxyl radical or both. In another study, the potential of natural astaxanthin to quench or scavenge singlet oxygen, superoxide, and peroxyl radical but not peroxynitrite was higher compared with synthetic astaxanthin.62 Whereas the reported data for singlet oxygen quenching and peroxyl radical scavenging are in general agreement with other reports, results are conflicting with a recent study. When using electron spin resonance and spin trapping to directly measure free radical reactions, Dose et al. did not detect any superoxide anion scavenging activity for synthetic astaxanthin.63 This discrepancy supports the notion that a combination of various assays should be used to assess antioxidant activities in order to adjust for methodological differences (e.g. temperature, solvent, pH, etc.) that might influence experimental outcomes.64
Table 4 Antioxidant activity of astaxanthin in test-tube systems
Authors |
Year |
Method |
Result |
Comment |
Miki25 |
1991 |
Singlet oxygen quenching |
Astaxanthin > α-tocopherol |
Source of astaxanthin not specified |
Suppression of lipid peroxidation by free radical scavenging |
Astaxanthin > zeaxanthin > lutein > β-carotene > α-tocopherol |
Lim et al.65 |
1992 |
Scavenging of peroxyl radical (generated by lipid-soluble radical generator) |
High antioxidant conc.: astaxanthin > zeaxanthin > canthaxanthin > β-carotene |
Synthetic astaxanthin |
Low antioxidant conc.: α-tocopherol > astaxanthin ≈ β-carotene |
Scavenging of peroxyl radical (generated by water-soluble radical generator) |
Astaxanthin > zeaxanthin > β-carotene > cantaxanthin |
Jørgensen and Skibsted60 |
1993 |
Scavenging of peroxyl radicals (in a heterogeneous lipid/water system) |
Astaxanthin ≈ β-carotene ≈ cantaxanthin ≈ zeaxanthin |
Synthetic astaxanthin |
Scavenging of peroxyl radicals (in a homogeneous chloroform system) |
Astaxanthin > cantaxanthin > β-carotene > zeaxanthin (effects of antioxidants are stability dependent) |
Shimidzu et al.66 |
1996 |
Singlet oxygen quenching (in a lipophilic system) |
Astaxanthin = β-carotene > zeaxanthin ≫ α-tocopherol |
Natural astaxanthin |
Singlet oxygen quenching (in a more hydrophilic system) |
Astaxanthin > zeaxanthin ≫ β-carotene |
Iwamoto et al.67 |
2000 |
Oxidation of isolated human LDL |
Astaxanthin > lutein > α-tocopherol |
Natural astaxanthin |
Goto et al.68 |
2001 |
Suppression of lipid peroxidation in liposomal membranes |
Astaxanthin > β-carotene |
Source of astaxanthin not specified |
Nishida et al.69 |
2007 |
Singlet oxygen quenching (in a lipophilic system) |
Lycopene > astaxanthin = β-carotene = cantaxanthin ≫ α-tocopherol |
Astaxanthin obtained from SIGMA (no further specification provided) |
Singlet oxygen quenching (in a hydrophilic system) |
Astaxanthin > lycopene ≈ cantaxanthin > β-carotene ≫ α-tocopherol |
Hama et al.70 |
2012 |
Hydroxyl radical scavenging (in aqueous solutions with liposome-encapsulated antioxidants) |
Astaxanthin > β-carotene |
Astaxanthin obtained from SIGMA (no further specification provided) |
Sueishi et al.71 |
2012 |
Oxygen radical absorbance capacity (of cyclodextrin-solubilized antioxidants) |
Myricetin > epicatechin > resveratrol > astaxanthin |
Source of astaxanthin not specified |
Chang et al.72 |
2013 |
Superoxide scavenging (in a a pyrogallol-luminol system) |
Trolox (α-tocopherol derivative)) > astaxanthin > β-carotene > vitamin C > cantaxanthin |
Astaxanthin obtained from SIGMA (no further specification provided) |
Hydroxyl radical scavenging (in a CuSO4-Phen-Vc-H2O2 system) |
Trolox > astaxanthin > canthaxanthin > β-carotene > vitamin C |
Hydrogen peroxide scavenging (in a luminol-H2O2 system) |
At low concentration: trolox > vitamin C > astaxanthin > canthaxanthin > β-carotene |
At high concentration: trolox > vitamin C > astaxanthin > β-carotene > canthaxanthin |
Régnier et al.73 |
2015 |
TEAC assay |
Astaxanthin-P > astaxantin-CO2 > astaxanthin-S (astaxanthin-P: obtained by solvent extraction; astaxantin-CO2: underwent a CO2 supercritical) |
Natural astaxanthin from H. pluvialis (Astaxanthin-P and –CO2) vs. synthetic form (Astaxanthin-S) |
ORAC assay |
Astaxanthin-P > astaxantin-CO2 > astaxanthin-S |
In addition to test-tube systems (which are of dubious relevance to human physiology), the antioxidant activity of astaxanthin has also been investigated in cell culture models of the cardiovascular system. The spectrum of atherosclerosis-related cells includes endothelial cells, vascular smooth muscle cells, macrophages and T-cells among others.5 Incubation of human umbilical vein endothelial cells (HUVECs) with natural astaxanthin (from Hematococcus algae) resulted in a marked reduction in ROS-induced lipid peroxidation. At the same time, the activities of antioxidant enzymes (catalase, glutathione peroxidase, glutathione reductase and SOD) were partly preserved.74 Also in HUVECs, the incubation for 48 h with two natural astaxanthin extracts obtained from H. pluvialis and synthetic astaxanthin caused a similar dose-dependent decline (conc. range 1–15 μM) in cell viability, with significant effects apparent only at the highest concentration used. Upon exposure of HUVEC to tert-butyl hydroperoxide, a molecule damaging to mitochondrial membranes, intracellular levels of ROS increase. At a concentration of 5 μM, the cellular antioxidant activity (measured with the fluorescent indicator DCFH-DA) was significantly higher in HUVECs treated with one of the two natural astaxanthin extracts compared with synthetic astaxanthin.73 This observation is in agreement with results obtained in other cell culture models.72 Astaxanthin (0.05–0.5 μM) also reduced intracellular ROS levels (visualized with the fluorescent probe CM-H2DCFPA) in a dose-dependent manner in HUVECs stressed with fluctuating glucose levels for 3 days.75 Regrettably, the source of astaxanthin used in this cell culture study is unknown. In the human macrophage-like cell line U397, astaxanthin (10 μM for 24 h) provided partial protection from hydrogen peroxide-induced cytotoxicity (MTT assay). Of note, astaxanthin alone was non-toxic to these cells.76 Subsequent work by the same group showed that pre-treatment with astaxanthin (10 μM) for 1 h also reduces LPS-induced superoxide production while preserving the activities of antioxidant enzymes SOD and catalase in U397 cells.77 For both studies in U397 cells, astaxanthin from the chemical supplier SIGMA has been used but there was no further specification regarding the source (SIGMA offers both natural and synthetic astaxanthin). In addition, it should be kept in mind that the suitability of U397 cells for studying CVD and other disease mechanisms has been questioned due to the fact that these cells show certain metabolic aberrations, especially the lack of apolipoprotein E (APOE) expression under basal and dexamethasone-induction.78
Further insights into the possible application of astaxanthin as an antioxidant arise from several ex vivo and in vivo studies conducted in laboratory animals. Already in 1991, Miki was able to demonstrate that malondialdehyde (MDA) levels (as marker of lipid peroxidation) in ghosts of red blood cells obtained from male rats fed a vitamin E-deficient diet supplemented with astaxanthin (1 mg per 100 g; astaxanthin of unknown source) for 4 weeks were significantly lower compared with those animals fed a vitamin E-deficient diet without the xanthophyll carotenoid.25 Sub-chronic treatment of rats with a synthetic astaxanthin derivative (disodium disuccinate astaxanthin; 0.1% and 0.4% in feed) for 7 days promoted systemic antioxidant effects, evident by a dose-dependent reduction in 9-HETE (a region-isomer oxidation product of arachidonic acid) and in 9-HODE (an oxidative product of linoleic acid origin).79 Levels of the lipid peroxidation marker MDA were also reduced in aortic rings of male diabetic rats subjected to intra-gastric intubation with astaxanthin (purchased from SIGMA with no further specification given) for 42 days. Of note, treatment with astaxanthin alone did not change MDA levels from baseline.80 In contrast, levels of lipid peroxidation in plasma of chicks fed ad libitum for 4 weeks with an experimental diet containing synthetic astaxanthin (0.5 g kg−1) or a basal diet were the same. Interestingly, β-carotene added to the basal diet significantly increased the extent of lipid peroxidation, possibly indicating a pro-oxidant effect of this particular carotenoid.65
The oxidation of LDL is believed to be of particular importance for the onset and progression of atherosclerosis and CVD, even though this hypothesis still needs full human confirmation.81 In the above mentioned study of male diabetic rats, astaxanthin treatment attenuated the oxidation of LDL by about 30 percent.80 However, no beneficial effect was observed in Watanabe heritable hyper-lipidemic rabbits fed with a diet containing astaxanthin (100 mg kg−1). After 24 weeks, the LDL oxidation lag time was 53.7 min and 56.4 min (P = 0.47) in the control and astaxanthin groups, respectively. In the same study, vitamin E (in the form of α-tocopherol at a concentration of 500 mg kg−1) increased the oxidation lag time to 109 (P < 0.001), suggesting a reduced susceptibility of LDL to oxidation.82
Taken together, test-tube and cell culture studies have provided interesting discoveries regarding the antioxidant potential of astaxanthin. The extrapolation of these results to pre-clinical models and human subjects, however, requires great care due to numerous (often underappreciated) methodological pitfalls (such as non-physiologic oxygen tension, cell culture contaminations, cell plating efficacy, test agent degradation, etc.) afflicting in vitro experiments.83–85 Likewise, peculiarities of pre-clinical models, such as the ability of rodents to synthesize the antioxidant vitamin C, need to be considered for the interpretation of animal research results.86
Astaxanthin's potential as an antioxidant has also been investigated in humans. A review published by Fassett and Coombes in 2012 provides an excellent overview of studies (published between 2000 and 2011) assessing orally administered astaxanthin (in a dose ranging between 1.8 to 100 mg per day, given once for durations of up to two years) in healthy subjects.22 In summary, there is some evidence for the reduction in fatty acid and LDL oxidation in humans without underlying disease. Recently, Baralic et al. treated young, healthy soccer players with astaxanthin (4 mg per day; derived from H. pluvialis) for 90 days. At the end of the intervention, total antioxidant status was unchanged from baseline, whereas the pro-oxidant/antioxidant balance declined.87 With regard to human subjects prone to the development of atherosclerosis and CVD, intervention studies indicate that certain population sub-groups treated with astaxanthin might benefit from lower levels of oxidative stress. In a randomized, double-blinded trial in overweight and obese males and females, astaxanthin (5 or 20 mg per day) intake for three weeks resulted in significantly reduced levels of lipid peroxidation biomarkers (MDA and isoprostane) in the plasma. At the same time, plasma levels of SOD and total antioxidant capacity were significantly increased.88 Recently, Coombes et al. published the outcome of the XANTHIN study, a randomized, placebo-controlled, double-blind trial conducted in 58 renal transplant recipients treated with astaxanthin (12 mg per day; obtained from H. pluvialis) for 1 year. Plasma values clearly indicate the absorption of astaxanthin into the blood; yet, the carotenoid had no effect on oxidative stress (assessed as total plasma F2-isoprostane) as a primary outcome.89
(Direct) antioxidant activity – protection from singlet oxygen toxicity
Several review articles highlight carotenoids, and here in particular astaxanthin, as superior quenchers of singlet oxygen, an electrochemically excited form of oxygen.24,38,42 Singlet oxygen belongs to the class of non-radial RS. Biological sources of singlet oxygen relevant for atherosclerosis and CVD include its generation by neutrophils and macrophages as well as via the decomposition of two reacting peroxyl radicals (Russel mechanism).4,90,91 Detailed information on the endogenous generation of singlet oxygen has been published elsewhere.91,92 Mechanistic studies suggest that energy is transferred from singlet oxygen to the astaxanthin molecule during singlet oxygen quenching. Astaxanthin in the energy-rich triplet state can return to the ground state by dissipating the energy as heat or by physical quenching, leaving the astaxanthin molecule not only intact but also ready for further quenching events. As a highly reactive species, singlet oxygen can oxidize lipids and many other cellular biomolecules.4,92 In addition, there is growing interest in singlet oxygen as an agent with cardiovascular cytotoxic effects, especially in experimental research on ischemia-reperfusion injury.93 However, the detection of singlet oxygen in vivo is very difficult, thus requiring researchers to instead measure biomarkers to quantify singlet oxygen toxicity indirectly.93 Zhai and Ashraf, for example, measured 5,8-endoperoxide, a specific product of β-carotene oxidation by singlet oxygen, during ischemia-reperfusion in rat hearts. There is some evidence from cell culture studies that carotenoids might protect cells from singlet oxygen toxicity.94,95 Recently, Bosio et al. reported new results which question the singlet oxygen-quenching activity of the carotenoid β-carotene. Using a microscope-based time-resolved spectroscopic method to directly examine singlet oxygen in mammalian cells, the authors concluded that β-carotene does not quench singlet oxygen.96 In light of this observation and the absence for experimental evidence from CVD-relevant pre-clinical models or human studies showing singlet oxygen quenching of astaxanthin, the actual contribution of singlet oxygen quenching by astaxanthin (and other carotenoids) might need re-evaluation.
At this point, it is important to keep in mind that singlet oxygen (like many other RS) also acts as an important cell signal and messenger, possibly with anti-atherogenic properties.90,97,98 Consequently, reducing RS levels via antioxidant interventions may obviously be a double edged sword.
In conclusion, experimental evidence for a direct and biologically meaningful (in terms of protection from atherosclerosis and CVD) antioxidant activity of astaxanthin is equivocal. Accordingly, it is rather difficult to explain all biological effects of astaxanthin merely by its antioxidant activity. Below we will focus on indirect antioxidant and non-antioxidant effects of astaxanthin, which might be more suitable for decoding the true potential of astaxanthin as a modulator of human health and disease.
Indirect antioxidant activity
Many enzymes contribute to the main line of defense against potentially harmful RS. Recently, the antioxidant activity of paraoxonase (PON1) and its implication on CVD has gained increasing interest.99,100 PON1 has been proposed to protect lipoproteins from oxidative modifications.101 Current cell culture data show that synthetic astaxanthin, albeit at very high concentrations of 20 μM boosts cellular antioxidant defense mechanism by inducing PON1.63 In contrast, natural astaxanthin (3–100 μg ml−1) added to serum obtained from normocholesterolemic rabbits did not alter the activity of PON1. However, when added to high-cholesterol feed (50–500 mg per 100 g diet), astaxanthin prevented the reduction in PON1 activity seen in untreated, hypercholesterolemic rabbits, suggesting that the effect of natural astaxanthin on PON1 was not related to a direct effect on the enzyme.102 Results showing a higher PON1 activity in healthy subjects treated with natural astaxanthin further supports the notion that astaxanthin might exert its health-beneficial effects by regulating the activity of this enzyme.103 This observation might be of clinical relevance as humans with low PON1 activity may be at increased risk of developing CVD.
Another important regulator of the cellular redox balance is Nuclear factor-E2-related factor (Nrf2), a transcription factor with high sensitivity to oxidative stress. Nrf2 is mainly maintained in the cytoplasm bound to Keap 1 (Kelch-like ECH-associated protein 1). Modification of Keap 1 by oxidative stress causes the release of Nrf2 from Keap 1 and the subsequent translocation of Nrf2 into the nucleus. Here, Nrf2 binds to the antioxidant response element (ARE) in DNA to initiate the expression of (1) phase II detoxification enzymes, (2) molecular chaperones and proteases, (3) DNA repair enzymes, and (4) anti-inflammatory response proteins and others.104,105 Recent reviews indicate that the Nrf2-ARE signaling pathway might not only be important for CVD but also numerous other human health and disease states.106–111 Of note, this hypothesis is yet to be confirmed in humans.112,113 Reports with regard to the activation of the Nrf2-ARE signaling pathway in vitro by astaxanthin are conflicting, possibly due to differences in cell type and other experimental parameters (Table 5). Unlike other bioactive plant metabolites, such as curcumin and the carotenoid lycopene,114,115 the ability of astaxanthin to activate the Nrf2-ARE signaling pathway appears rather low (if at all), despite evidence of the beneficial effect of astaxanthin on other oxidative stress-related parameters (e.g. reduced ROS and increase glutathione [GSH] levels) in some cell lines.63,116 As GSH synthesis and production of ROS are partly controlled by the Nrf2-ARE signaling pathway, their modulation by astaxanthin in the absence of its effect on Nrf2 translocation/ARE activation suggests a complex regulatory network that requires further investigation.
Table 5 Effects of astaxanthin on Nrf2-ARE signaling pathway and oxidative stress parameters in un-stressed cell cultures
Authors |
Year |
Cell type |
Astaxanthin concentration & source |
ARE trans-activation |
Effect on expression of Nrf2 target genes (mRNA/protein) |
Other oxidative stress-relevant effects |
(—) = not reported |
Ben-Dor et al.114 |
2005 |
HepG2 |
7–10 μM |
Low |
None/none |
• GSH unchanged |
Sigma |
• ROS reduced |
Ye et al.117 |
2012 |
PC12 |
10 μM |
(—) |
Low/low to moderate |
(—) |
Wako (Catalog No. 013–23051) |
Saw et al.116 |
2013 |
HepG2 |
3.13–100 μM |
moderate to high |
Low/(—) |
• GSH increased |
Santa Cruz (Catalog No. sc-202473) |
Li et al.118 |
2013 |
ARPE-19 |
5–20 μM |
(—) |
Moderate/moderate |
• ROS reduced |
Sigma |
Franceschelli et al.77 |
2014 |
U937 |
10 μM |
(—) |
(—)/none |
• ROS unchanged |
Sigma |
Dose et al.63 |
2016 |
HepG2 |
1–5 μM |
(—) |
(—) |
• GSH increased |
Synthetic |
Huh7 |
1–20 μM |
(—) |
None/— |
(—) |
Synthetic |
To the best of our knowledge, the potential of astaxanthin to modulate the Nrf2-ARE signaling pathway has not been studied in humans, and only rarely in animals. Rats treated orally with astaxanthin (25 mg per kg BW; astaxanthin (CAS no. 472-61-7, purity ≥92%), SIGMA), responded with increased expression of Nrf2 and its downstream target, HO-1. At the same time, the damaging effects of cyclophosphamide, an alkylating agent used in cancer research that disturbs the oxidant and antioxidant balance, have been attenuated.119 However, the mechanism(s) responsible for the activation of the Nrf2-ARE signaling pathway by astaxanthin reported in some studies have not been completely elucidated.120 Researchers working in this field should also be open to the possibility that astaxanthin exerts its effects without Nrf2-ARE activation, as recently shown for curcumin in patients with nondiabetic or diabetic proteinuric chronic kidney disease.121
Anti-inflammatory activity
In 1986, Ross recognized chronic inflammation as a hallmark of atherosclerosis.122 Since then, several immune mediators (e.g. chemokines and lipids) and at least four inflammatory signaling pathways (MAPK, PI3K, JAK/STAT and NFκB) have emerged as targets in CVD.123,124 Furthermore, inflammation has not only been suggested to play a causative role in CVD, but has also implicated in the development of other CVD-related illnesses, especially DM, hypertension, chronic kidney disease, hypercholesterolemia and obesity.125–128 In clinical practice, high-sensitivity C-reactive protein (hs-CRP) serves as a commonly used inflammatory biomarker to determine CVD risk, although recent evidence suggest that hs-CRP might not always be suitable as a biological marker to this effect.129,130 Considering the close link between inflammation and oxidative stress, it is unsurprising that the number of studies investigating astaxanthin as an agent to lower inflammation is only second to those addressing its antioxidant effects. In vitro, astaxanthin has been shown to reduce markers of inflammation in several stimulated cell lines, for instance, rat alveolar macrophages,131 U937 cells,77 THP-1 cells,132 RAW 264.7 cells,133 proximal tubular epithelial cells,134 HUVECs75 and human lymphocytes.135 Of note, in all cases, the anti-inflammatory activity has been predominantly ascribed to the RS-lowering effects of astaxanthin. Additional evidence to support the notion that astaxanthin is able to reduce the inflammatory load can be derived from experiments in different animal models (rats, mice, dogs and chicken) related to CVD. Most (though not all)136,137 animal studies showed a concomitant reduction in oxidative stress and inflammation and are thus in general agreement with results reported in vitro.138–145 In 2015, Ni et al. published probably the most detailed report to date investigating astaxanthin's potential to attenuate inflammation in animals. When NASH (nonalcoholic steatohepatitis) was induced (by diet), administration of natural astaxanthin (0.02% in diet [≈20 mg per kg BW] from H. pluvialis, Fuji Chemicals) reduced both hepatic inflammation and insulin resistance in these animals. Whether the observed biochemical (e.g. reduced hepatic lipid accumulation and attenuated JNK signaling) and cellular changes (e.g. reduced T cell recruitment in liver) were independent effects of astaxanthin or a result, at least partly, of its antioxidant activity (evident by reduced lipid peroxidation) requires further clarification. In direct comparison to vitamin E, astaxanthin was more effective at both preventing and treating NASH in this rodent model. Importantly, astaxanthin also alleviated NASH progression in humans.146 Free-living, young healthy females supplemented with natural astaxanthin (2 and 8 mg per day; from H. pluvialis, La Haye Laboratories) for 8 weeks showed lower CRP levels and indications of an improved immune cell response and cytokine profile. Interestingly, astaxanthin reduced DNA damage but not lipid peroxidation in plasma. In terms of efficacy, 2 mg astaxanthin appeared to be superior compared to the higher dosage (8 mg) in most test parameters.54 However, natural astaxanthin had no effect on plasma CRP levels in renal transplant patients supplemented with 12 mg per day.89
Blood pressure activity
Raised blood pressure (BP) has been pointed out as “the biggest single contributor to the global burden of disease and to global mortality”.147 By 2025, the number of hypertensive individuals is expected to reach 1.56 billion globally.148 Considering that hypertension affects all regions and populations in the world, the implementation of cost-effective treatments and management protocols is warranted.149 Although the exact pathogenesis of hypertension remains unknown, it is now generally accepted that circadian signaling pathways and metabolic feedback loops in several organs (especially brain, kidney and heart) contribute to the onset and progression of elevated BP. Additionally, environmental and behavioral factors as well as sex differences need to be considered to treat hypertensive patients effectively.150–152
The effectiveness of astaxanthin to reduce the BP in commonly used rat models is summarized in Table 6. Despite different test conditions, synthetic and natural astaxanthin showed a reproducible (and mostly significant) blood pressure-lowering activity in these studies. Of note, the anti-hypertensive effects were of similar magnitude as those reported for the antioxidant vitamin E and captopril, an angiotensin-converting enzyme inhibitor used for the treatment of hypertension.153,154 Mostly nitric oxide- and oxidative stress-related mechanisms have been suggested to explain astaxanthin's anti-hypertensive effects in vivo.154–156 In humans, neither healthy subjects (treated with 2 mg natural astaxanthin per day)157 nor patients prone to CVD (Xanthin trial conducted for renal transplant recipients supplemented with 12 mg natural astaxanthin for 12 months) benefited from astaxanthin intake in terms of improved blood pressure values.89 However, test subjects in both trials were normotensive. Hence, additional well-controlled, large-scale intervention studies are needed to elucidate the efficacy of astaxanthin as an anti-hypertensive agent in humans.
Table 6 Anti-hypertensive activity of astaxanthin in animal models
Authors |
Year |
Animal model |
Astaxanthin concentration & Source |
Treatment duration |
Blood pressure differencea (mmHg) |
Blood pressure difference has been calculated from: BPControl − BPAstaxanthin at the end of the treatment period. Negative numbers indicate a blood-pressure lowering effect of astaxanthin. SBP = systolic BP, DBP = diastolic BP, MBP = mean BP. *Significantly different; avalues were estimated from published line graphs. |
Hussein et al.155 |
2005 |
Wistar Kyoto rats (WKY, normotensive); |
50 mgper kg BW (from Fuji Chemicals) |
2 weeks |
WKY: |
Spontaneously hyptertensive rat (SHR); male |
|
|
MBP: −18 |
|
|
|
SHR: |
|
|
|
MBP: −17* |
SHR-stroke prone rats; male |
5 and 50 mg per kg BW (from Fuji Chemicals) |
5 weeks |
5 mg per kg BW: |
|
|
|
MBP: −21*,a |
|
|
|
50 mg per kg BW: |
|
|
|
MBP: −19 |
Hussein et al.156 |
2006 |
SHR rats; male |
5 mg per kg BW (from Fuji Chemicals) |
7 weeks |
SBP: −24* |
MBP: −26* |
Hussein et al.158 |
2007 |
SHR/NDmcr-cp (metabolic syndrome model); male |
50 mg per kg BW (from Fuji Chemicals) |
18 weeks |
SBP: −19*,a |
DBP: −23*,a |
Preuss et al.153 |
2011 |
Sprague-Dawley rats; male |
25, 50 and 100 mg per kg diet (from H. pluvialis) |
8 months |
25 mg per kg diet: |
SBP: −2 |
DBP: +0.1 |
|
50 mg kg−1 diet: |
SBP: −6.2 |
DBP: −6.9 |
|
100 mg kg−1 diet: |
SBP: −7.5* |
DBP: −11.3* |
Sasaki et al.154 |
2011 |
SHR-stroke prone rats; male |
300 and 600 mg per kg diet (from Sigma) |
3 weeks |
300 mg kg−1 diet: |
SBP: −25*,a |
600 mg kg−1 diet |
SBP: −27*,a |
Monroy-Ruiz et al.159 |
2011 |
SHR rats; male |
75 and 200 mg per kg BW (from BASF) |
8 weeks |
75 mg per kg BW: |
SBP: −24*,a |
200 mg per kg BW: |
SBP: −28*,a |
Anti-diabetic activity
The incidence of DM has increased dramatically in the past decades and is expected to rise to 592 million globally by 2035, with type 2 diabetes accounting for more than >85% of total DM cases. DM is a chronic metabolic disease that follows from defects in insulin secretion, insulin signaling, or both.160 There is now general consensus that lifestyle changes and especially an adequate diet rich in bioactive metabolites of animal and plant origin are important interventions for reducing the global health burden of DM.161,162
Hyperglycemia is an important factor contributing to diabetic complications.163 The blood glucose-lowering effects of astaxanthin have been studied in different animal models with variable outcome (Fig. 5). A recent meta-analysis of randomized controlled trials indicates a minor glucose-lowering effect of astaxanthin (concentration range of 4–20 mg per day) in humans.164
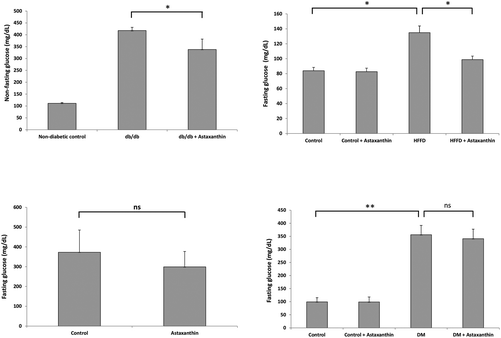 |
| Fig. 5 Anti-diabetic effect of astaxanthin in mice. Left upper graph: Female db/db mice (n = 12 per group) treated orally with 1 mg of astaxanthin (of unknown origin) per mouse and day for 12 weeks showed lower non-fasting blood glucose levels (in mg dL−1) compared to untreated animals. *P < 0.01 versus db/db mice after 6 weeks of treatment. **P < 0.01 versus db/db mice after 12 weeks of treatment. Data were taken from Uchiyama et al.168 Right upper graph: Male mice (n = 6 per group) were fed a control or high fat-high fructose (HFFD) diet for 45 days. Fasting glucose levels were significantly lower in HFFD-fed animals treated orally with 6 mg per kg BW of astaxanthin (obtained from Sigma). *P < 0.05. Data were taken from Arunkumar et al.145 Left lower graph: Male KK-Ay mice (obese/diabetic model; n = 7 per group) fed an astaxanthin-containing diet (1 g kg−1 diet; astaxanthin oil from AstaReal®, Japan) for four weeks showed lower, though not significantly different (ns), fasting glucose levels. Data were taken from Ravi Kumar et al.136 Right lower panel: In male, streptozotocin-induced diabetic rats (n = 6 per group) treated orally with 10 mg per kg BW of astaxanthin (Sigma) for 42 days, fasting glucose levels were not significantly different (ns) from control animals. Data presented here in mg dL−1 were converted from μM values of fasting blood glucose shown in the original publication by Zhao et al.80 | |
Several mechanisms have been proposed to explain the putative anti-diabetic effects of astaxanthin. In rats (n = 8 per group) fed a diet supplemented with natural astaxanthin from H. pluvialis (25, 50 and 100 mg kg−1 diet) for 2 months, HOMA units were significantly lower compared to controls, suggesting improved insulin sensitivity in astaxanthin-treated animals.153 Compared to control animals maintained on a high fat-high fructose diet (HFFD) for 45 days, HOMA units were also lower (P < 0.05) in HFFD-fed mice (n = 6 per group) that were orally treated with astaxanthin (6 mg per kg BW; Sigma).165 Additional data by the same authors suggest that astaxanthin might have improved insulin sensitivity by enhancing GLUT-4 translocation in skeletal muscle and IRS-PI3K-Akt signaling pathway.145,165,166 In L6 rat myoblasts, incubation with astaxanthin (5 μM; Fuji Chemical Industry Co Ltd, Toyama, Japan) also enhanced insulin-stimulated GLUT4 translocation and glucose uptake. In agreement with the results obtained in HFFD-fed mice, the astaxanthin effects in L6 cells were associated with an increase in insulin receptor substrate-1 tyrosine and Akt phosphorylation, as well as a decrease in c-Jun N-terminal kinase and insulin receptor substrate-1 serine 307 phosphorylation.167 Abdelzaher et al. observed a similar response in HUVECs exposed to glucose fluctuations. Here, astaxanthin (0.5 μM; Fuji Chemical Industries) significantly inhibited the glucose-induced increase in c-Jun N-terminal kinase and p38 phosphorylation.75 Moreover, astaxanthin may attenuate some negative effects of DM by (1) improving the ACh-induced endothelium-dependent relaxation in aortic rings (demonstrated in diabetic rats),80 (2) attenuating endoplasmic reticulum stress (shown in HFFD-fed mice),140 (3) lowering levels of oxidative stress (discussed above), and (4) reducing inflammation (discussed above).
Renoprotective activity
Chronic diseases of the kidney are among the main causes of CVD and vice versa.169,170 The kidney contributes to human health by controlling several important functions, including electrolyte balance, vitamin D metabolism, waste excretion, erythropoietin production and blood pressure regulation. The identification of renoprotective agents is urgently needed as the number of therapeutic drug options for patients with chronic kidney disease (CKD), especially those of unknown etiology, is limited.171,172 Using a mouse model of adriamycin-induced focal and segmental glomerulosclerosis, daily administration of astaxanthin (obtained from Alexis® Biochemicals, Enzo Life Sciences, Inc., New York, N.Y., USA) at a concentration of 50 mg per kg body weight by oral gavage significantly improved parameters of kidney malfunction, including creatinine clearance, proteinuria, serum albumin, lipid peroxidation, antioxidant enzyme activity and Nrf2 expression.142 Similarly, astaxanthin (free type ≥97%, Sigma) given orally via gavage at a dose of 5 mg per kg BW partly protected mice from ischemia/reperfusion-induced renal injury.139
Furthermore, it is now well established that CKD, as a co-morbidity of DM, contribute to the onset and progression of CVD in many diabetic patients.173 Fruit and vegetable-based diets rich in bioactive plant metabolites have been associated with reduced risks of developing CVD and DM.174–177 In alloxan-induced diabetic rats, treatment with astaxanthin (20 mg per kg BW) extracted from shrimp shell waste ameliorated nephropathy, possibly due to the observed increase in antioxidant enzyme activity (catalase and SOD) and prevention of oxidative damage (lipid peroxidation and protein carbonyl).178 Astaxanthin treatment (0.02% in diet; Fuji Chem. Industry Co., Ltd, Toyama, Japan) for 12 weeks also prevented the progression of diabetic nephropathy induced by oxidative stress in mice. Astaxanthin concentration in the kidney reached 29.4 μg g−1 tissue compared to 46.7 and 270.3 μg g−1 tissue in heart and liver, respectively, thus confirming previous results showing the accumulation of astaxanthin following oral intake.179 Using mesangial cells as an in vitro model, the same authors not only detected a substantial accumulation of astaxanthin in the mitochondria of these cells but also reduced levels of mitochondria-associated lipid peroxidation and ROS production. Mesangial cells surround the vascular endothelium in the glomerulus, release cytokines and produce the extra cellular matrix to support neighboring cells. As the overproduction of mesangial matrix is an important event in the pathogenesis of diabetic nephropathy, protecting mesangial cells (possibly by astaxanthin) is vital.180
Recently, however, hope for using astaxanthin as a viable treatment option in CKD patients suffered a setback when Fassett and Coombes published data from the abovementioned XANTHIN trial, showing no effect of natural astaxanthin treatment on arterial stiffness, oxidative stress, or inflammation in renal transplant recipients.89
Lipid-lowering activity
Testing for anomalies of the lipid profile is among the most commonly ordered clinical blood analyses. There is now strong evidence that elevated total cholesterol (TC), low-density lipoprotein cholesterol (LDL-C) and triglyceride levels (TG) as well as low high-density lipoprotein cholesterol (HDL-C) levels contribute to dyslipidemia and increase the risk of CVD.181 When supplemented to laboratory animals, astaxanthin showed promising lipid-lowering activity. Mice supplemented with 0.03% (but not lower concentrations) of natural astaxanthin in the diet (H. pluvialis from Fuji Chemicals) for 12 weeks exhibited significantly lower plasma TG concentrations compared with the control animals. At the same time, there were no significant differences in plasma TC and HDL-C concentrations between the control and treatment groups.182 In a rat model of metabolic syndrome, oral administration of astaxanthin (50 mg per kg per day) for 22 weeks induced a significant increase in HDL-C, and significantly lower levels of plasma TG and non-esterified fatty acids. Additionally, astaxanthin affected white adipose tissue by decreasing the size of the fat cells. The effects might be due, at least partly, to the elevated adiponectin level measured in astaxanthin-treated animals and humans.158,183 A decrease in serum adiponectin has been observed in obese, insulin-resistant, type 2 diabetic rodents and humans, indicating a possible causative role of this reduced adiponectin concentrations in insulin resistance and fat accumulation of obesity.184 Adiponectin may also improve dyslipidemia by regulating HDL metabolism.185,186 A recent study in mice fed a high fat diet further showed that oral astaxanthin (6 and 30 mg per kg BW; in olive oil; no further specification) treatment for 8 weeks lowers hepatic lipid accumulation via activation of peroxisome proliferator-activated receptor (PPAR) alpha and inhibition of PPAR gamma and Akt-mTORC1 signaling.187 Hypocholesterolemic effects of astaxanthin (from H. pluvialis; provided by Algaen) were also observed in APOE-knockout mice fed a high-fat and high-cholesterol-diet. After 4 weeks, astaxanthin (0.03% in the diet) increased the expression of genes involved in the hepatic cholesterol metabolism (i.e. LDL receptor, 3-hydroxy-3-methylglutaryl CoA reductase and sterol regulatory element binding protein 2) and of enzymes important for β-oxidation (e.g. carnitine palmitoyltransferase-1 and acyl-CoA oxidase). In contrast, there was no difference in the expression of lipogenic genes between astaxanthin-treated and control animals.188
Astaxanthin's lipid-lowering effect, however, has not been observed in all in vivo studies. In hypercholesterolemic rabbits, for instance, natural astaxanthin (from Fuji Chemicals) treatment with concentrations of 50, 100 and 500 mg kg−1 diet for 60 days neither changed the profile of serum fatty acids nor prevented the diet-induced increase in blood lipids (TC, TG, HDL-C and non-HDL cholesterol). Likewise, in humans, the number of randomized controlled trials showing no effect of astaxanthin on dyslipidemia outweighs those showing a positive trend. Based on their meta-analysis, Ursoniu et al. concluded that astaxanthin supplementation does not exert any significant effect on the plasma lipid profile in humans. Nonetheless, it is probably too early to dismiss astaxanthin's potential as a lipid-lowering agent due to the considerable heterogeneity of study designs and data collection methods, making high quality randomized controlled studies one of the most pressing future research activities.
Astaxanthin in the antioxidant network
There is now good evidence that antioxidant compounds usually do not work in isolation but exert cooperative effects to maintain the redox balance in an organism. Hydrophilic vitamin C, for example, has been shown to regenerate lipophilic vitamin E at the cytosol/membrane interface. Similar interactions have been described for certain polyphenols (e.g. quercetin and catechins) and the carotenoids β-carotene and lycopene.189–193 Less is known about the synergistic interactions of astaxanthin within the antioxidant network. Du et al. reported that astaxanthin contributes to the protection of membrane integrity against photosensitized oxidation in synergy with other carotenoids.194 Within membranes, the polar astaxanthin is oriented perpendicular to the membrane surface whereas highly lipophilic carotenoids (e.g. lycopene) are located within the cell membrane. A thermodynamic effect has been suggested to explain the synergistic activity between astaxanthin and lycopene as antioxidants.42,195 In diabetic rats, a combination of natural astaxanthin (from H. pluvialis) and α-tocopherol (but not vitamin C) reduced the level of oxidative stress to a greater extent than either of the antioxidants alone. Of note, astaxanthin treatment increased the concentrations of α-tocopherol in serum and liver, and vice versa.196 In cells, the combination of astaxanthin (from Santa Cruz) with long-chain omega fatty acids (which have been suggested to act as antioxidants as well)197 showed a moderately higher activation of the Nrf2-ARE signaling pathway compared to individual treatments.116 From these observations it becomes clear that looking for a combination of antioxidants (and other nutrients and non-nutrients for that matter) presents probably a more promising approach for attenuating oxidative stress- and inflammation-driven diseases in humans.
Does oral astaxanthin intake reduce atherosclerosis and CVD events in vivo?
With respect to the circulatory system, the ultimate goal of an intervention with astaxanthin (or any other compound claiming preventive or therapeutic activity) is the reduction in the incidence of atherosclerosis and CVD events. To the best of our knowledge, the ability of astaxanthin to reduce CVD endpoints such as stroke severity and myocardial infarct size has only been studied in animal models but, so far, never in humans. Based on the animal experiments summarized in Table 7, it appears that astaxanthin has some potential to reduce the occurrence of atherosclerosis and CVD events in vivo. Future studies will need to show whether the same holds true for human patients.
Table 7 Animal studies of astaxanthin effects related to the reduction of atherosclerosis and CVD events
Authors |
Year |
Animal model |
Astaxanthin concentration & Source |
Treatment duration |
Outcome |
CDX-085 is a water dispersible astaxanthin prodrug. Upon oral ingestion, it is hydrolyzed in the intestine, yielding free astaxanthin for absorption and systemic distribution.
Studies reporting effects of the formerly available astaxanthin derivate DDA (disodium disuccinate astaxanthin) have been reviewed elsewhere.22
|
Studies using natural or synthetic astaxanthin
|
Jacobsson et al.82 |
2004 |
Watanabe heritable hyperlipidemic rabbits |
100 mg kg−1 diet; from AstaCarotene, Sweden |
24 weeks |
No effect on atherosclerotic area in the thoracic aorta |
Li et al.198 |
2004 |
Watanabe heritable hyperlipidemic rabbits |
100 mg kg−1 diet; from AstaCarotene, Sweden |
24 weeks |
Improved plaque stability in thoracic aorta |
Augusti et al.199 |
2009 |
Atherosclerotic New Zealand white rabbits; male |
500, 1000 and 5000 mg kg−1 diet; from H. pluvialis (Fuji Chemicals) |
60 days |
No effect on atherosclerotic lesion formation in the aorta |
Shen et al.200 |
2009 |
Sprague-Dawley rats; male |
20 μL of 0.1 M astaxanthin (from Sigma) in DMSO; injected intra-cerebroventricularly prior middle cerebral artery occlusion |
Once |
Reduced infarction size 2 days after middle cerebral artery occlusion |
Lu et al.201 |
2010 |
Sprague-Dawley rats; male |
Oral administration of astaxanthin (20, 50, 80 mg kg−1; from Sigma) twice at 5 h and 1 h prior to ischemia |
Twice |
Reduced infarct volume |
Sasaki et al.154 |
2011 |
Stroke-prone spontaneously hypertensive rats; male |
300 and 600 mg kg−1 diet (from Sigma) |
3 weeks |
Anti-thrombogenic effect in cerebral microvessels |
Studies using the astaxanthin prodrug CDX-085
,
|
Khan et al.202 |
2010 |
C57BL/6 J mice; male |
0.4% CDX-085 in feed (Cardax Pharmaceuticals, Hawaii) |
14 days |
Anti-thrombogenic effecting the common carotid artery |
Ryu et al.203 |
2012 |
LDL receptor knockout and control mice |
0.08 and 0.4% CDX-085 |
12 weeks |
Lower extent of atherosclerosis in the aortic arch |
APOE-knockout and control mice |
9 weeks |
No anti-atherosclerotic effects |
Safety aspects of astaxanthin
The safety of astaxanthin from different sources has been evaluated by different authorities, including EFSA and FDA for its possible use as a food ingredient, feed additive and as a supplement ingredient.
The first evaluation of astaxanthin goes back to the 1980s, when synthetic astaxanthin won approval from FDA for use as a pigment in salmon feed; in the meantime, other sources of astaxanthin (e.g. from X. dendrorhous) have also been positively evaluated by the EFSA Panel on Additives and Products or Substances used in Animal Feed (FEEDAP) for the same use. At the same time, it is worth mentioning that until now, only astaxanthin from H. pluvialis (either in dried form or extracted with ethanol or supercritical CO2) has been authorized for direct human consumption (in form of dietary supplements) both by the FDA (notifications filed at dosages up to 12 mg per die and up to 24 mg per die for no more than 30 days) as well as by EFSA, with FDA having received also a notification for a new dietary ingredient derived from Paracoccus carotinifaciens at a dose of 6 mg per die.204
Regarding the possible use of astaxanthin in conventional foods, FDA had no questions for different GRAS (Generally Recognized as Safe) notifications made with astaxanthin extracted from H. pluvialis at the proposed level of use (up to a maximum level of 0.15 milligrams (mg) astaxanthin per serving). In contrast, the EFSA has recently rejected the market authorization at the requested use levels for one product intended to be incorporated into yogurts.47 In refusing the market authorization at the requested use level (maximum recommended intake of 4 mg astaxanthin per day (0.06 mg per kg BW) and the estimated mean intake based on the use levels in the proposed food categories (0.106 mg per kg BW per day), respectively), the EFSA has pointed out a concern raised by the EFSA FEEDAP Panel regarding a two-year carcinogenicity study conducted with a preparations containing about 8% of synthetic astaxanthin.37,205 On the other hand, Edwards et al. recently concluded that the reported “hepatocellular adenoma in female rats is most probably a species-specific phenomenon of doubtful human relevance”.37,206
Conclusion and future direction
After several decades of astaxanthin research, where do we stand? Evidence backing-up the notion of astaxanthin not only as an excellent, but often also superior, antioxidant (compared to ‘classical’ antioxidants such as vitamin C and E) in vitro is clear-cut, with some indication that natural astaxanthin shows a higher quenching and scavenging efficacy than synthetic astaxanthin. With respect to astaxanthin's antioxidant activity in vivo, the level of support provided by animal experiments is still moderate to good. For humans, especially healthy subjects, the evidence is equivocal, whereas no conclusive statement can be made regarding the potential of astaxanthin to effectively treat elevated levels of oxidative stress in humans with pre-existing atherosclerosis and CVD.207 This review and those published by other authors reveal a similar picture for illnesses closely related to CVD (DM, hypertension, CKD, dyslipidemia, chronic inflammation): a steep decline in the number of positive (i.e. health-claim supporting) results from in vitro experiments to animal studies to human trials. At the same time, it is worth pointing out that not only absorption and tissue distribution but also toxicological aspects pertaining to animal and human health have been well-characterized for astaxanthin (unlike many other plant- or animal-derived food ingredients), resulting in astaxanthin as a compound that is both, bioavailable and safe for human consumption and merits further investigations. For these future works, it might be important to identify pitfalls and knowledge gaps in astaxanthin research (Table 8). From this (non-comprehensive) list, it is evident that experimental shortcomings and outstanding questions both contribute to the still rather poor positioning of astaxanthin as a viable option for the prevention and treatment of CVD and other acute and chronic diseases.
Table 8 List of knowledge gaps and potential pitfalls in astaxanthin research
Knowledge gaps in astaxanthin research
|
• The impact of the astaxanthin product composition (particularly the difference of the optical and geometrical astaxanthin isomers, the minor compounds present in natural astaxanthin, or the influence of the different commercial formulations) needs to be studied in more detail. |
• The optimal preventive/therapeutic dosages of astaxanthin to be used in humans (and animals) require refinement and experimental support (evidence-based approach). |
• A guideline of how to extrapolate results obtained in cell culture experiments to the in vivo situation is lacking; in particular, more work is needed to better understand how (1) different solvents affect the solubility of astaxanthin in cell culture media and (2) astaxanthin concentrations used in vitro relate to, for example, actual plasma levels in animals and humans. |
• Strong (but not definite) experimental evidence indicate that RS are probably not responsible for aging. Considering that aging, however, is the most important risk factor for CVD, the question arises whether astaxanthin, touted as a superior antioxidant in vivo, can have a significant impact on cardiovascular health and disease. |
• Astaxanthin – drug interactions (e.g. in the gastro-intestinal tract and blood), like for most natural compounds, have been poorly investigated. |
• Little is known about the long-term accumulation and tissue detectability of astaxanthin following the termination of intervention; the same applies to astaxanthin excretion. |
• Different sources of astaxanthin yield different products and this is seldom acknowledged by researchers. |
• Many authors only poorly define the origin and composition of astaxanthin being investigated, making data comparisons difficult, if not impossible. |
Potential pitfalls and cofounders in astaxanthin research
|
(A) Cell culture studies
|
• Increased MTT reduction (a marker of cell viability) in the presence of astaxanthin suggests that this carotenoid may (1) influence cell growth and/or proliferation by currently unknown mechanisms, or (2) interact with the MTT chemicals. As previously shown for other antioxidants, significant effects of astaxanthin on the MTT signal need to be considered when interpreting MTT data as a measure of astaxanthin's antioxidant potential in vitro.131,215,216 |
• Cause and consequences of natural astaxanthin precipitates in cell culture medium need to be explored.73 |
• Problem of cell culture artefacts (caused, e.g., by the use of fluorescent dyes, different oxygen tensions or solvents, non-authenticated cell lines, etc.) are often underappreciated.85,217 |
(B) Animal experiments
|
• Rodents have been shown to synthesize vitamin C; what does this mean for studying antioxidants in rats/mice and how do we best extrapolate results to humans?86 |
• Predictive power in conditions such as stroke has been described as poor.212 |
(C) Human trials
|
• Antioxidant intervention studies in humans have been suggested to require several years to see effects on the vascular system and require the inclusion of sufficient number of well-defined study subjects.218,219 |
• Selected biomarkers and their analytical method are often sub-optimal220–222 |
Moreover, well-designed studies are urgently required to discern the activity profile of natural and synthetic astaxanthin and their constituents (here especially the relative contribution of the different optical and geometrical isomers but also of non-astaxanthin nature) in order to establish areas of application in consumer health care.
Finally, aging – as the main risk factor for CVD – should move more into the center of attention. There is now good experimental in vivo evidence that oxidative stress – although intimately linked to several aging-related pathologies – is probably not a key driver of the aging process.208–211 Consequently, antioxidant treatments (especially when un-targeted) may not show the desired effects in terms of disease prevention, deceleration and reversal.212–214 Although one might argue that astaxanthin does show many effects beyond antioxidant activity, it is still unclear whether its indirect and non-antioxidant effects are cause or consequence of the concomitantly observed reduction in oxidative stress.
In conclusion, the pitfalls afflicting many experimental studies and gaps in our understanding regarding the effects of astaxanthin on cardiovascular health and disease leave plenty of room for discovery with regards to this promising bioactive molecule, which is both bioavailable and safe for human consumption.
Acknowledgements
The authors wish to thank ACADELION Scientific and Medical Communications for developing the conceptual outline of this article, and for critically reading and carefully editing the manuscript, and the The Visual Agency S.r.l. Milan for the graphical support.
Supported in part by European FEDER Funds and Programa de actividades en tecnologías ALIBIRD-CM S2013/ABU-2728 de la Comunidad de Madrid.
References
- L. Chaker, A. Falla, S. J. van der Lee, T. Muka, D. Imo and L. Jaspers,
et al., The global impact of non-communicable diseases on macro-economic productivity: a systematic review, Eur. J. Epidemiol., 2015, 30(5), 357–395 CrossRef PubMed.
- S. Bansilal, J. M. Castellano and V. Fuster, Global burden of CVD: focus on secondary prevention of cardiovascular disease, Int. J. Cardiol., 2015, 201(Suppl), S1–S7 CrossRef PubMed.
- L. J. Laslett, P. Alagona, B. A. Clark, J. P. Drozda, F. Saldivar and S. R. Wilson,
et al., The worldwide environment of cardiovascular disease: prevalence, diagnosis, therapy, and policy issues: a report from the American College of Cardiology, J. Am. Coll. Cardiol., 2012, 60(25 Suppl), S1–49 CrossRef PubMed.
-
B. Halliwell and J. M. C. Gutteridge, Reactive species and disease: facts, fiction or filibuster? Free Radicals in Biology and Medicine, Oxford University Press, 2007, pp. 488–613 Search PubMed.
-
M. Garelnabi, S. Kakumanu and D. Litvinov, Role of Oxidized Lipids in Atherosclerosis, Oxidative Stress and Diseases, InTech, 2012, pp. 119–2040 Search PubMed.
- P. Srinivasa Rao and V. Kiranmayi, Biochemical mechanisms underlying atherogenesis, J. Clin. Sci. Res., 2012, 1, 24–34 Search PubMed.
- J. Rudolf and K. B. Lewandrowski, Cholesterol, lipoproteins, high-sensitivity c-reactive protein, and other risk factors for atherosclerosis, Clin. Lab. Med., 2014, 34(1), 113–127 CrossRef PubMed.
-
M. Linton, P. Yancey, S. Davies, W. Jerome, E. Linton and K. Vickers, The Role of Lipids and Lipoproteins in Atherosclerosis, South Dartmouth (MA), Endotext [Internet], MDText.com, Inc., 2015 Search PubMed.
- N. Siti Hawa, K. Yusof and J. Kamsiah, The Role of Oxidative Stress, Antioxidants and Vascular Inflammaton in Cardiovascular Disease (A Review), Vasc. Pharmacol., 2015, 71, 40–56 CrossRef CAS PubMed.
- R. Lee, M. Margaritis, K. M. Channon and C. Antoniades, Evaluating oxidative stress in human cardiovascular disease: methodological aspects and considerations, Curr. Med. Chem., 2012, 19(16), 2504–2520 CrossRef CAS PubMed.
- R. Stocker and J. F. Keaney, Role of oxidative modifications in atherosclerosis, Physiol. Rev., 2004, 84(4), 1381–1478 CrossRef CAS PubMed.
- D. P. Vivekananthan, M. S. Penn, S. K. Sapp, A. Hsu and E. J. Topol, Use of antioxidant vitamins for the prevention of cardiovascular disease: meta-analysis of randomised trials, Lancet, 2003, 361(9374), 2017–2023 CrossRef CAS.
- Y. Wang, O. K. Chun and W. O. Song, Plasma and dietary antioxidant status as cardiovascular disease risk factors: a review of human studies, Nutrients, 2013, 5(8), 2969–3004 CrossRef CAS PubMed.
- S.-K. Myung, W. Ju, B. Cho, S.-W. Oh, S. M. Park and B.-K. Koo,
et al., Efficacy of vitamin and antioxidant supplements in prevention of cardiovascular disease: systematic review and meta-analysis of randomised controlled trials, Br. Med. J., 2013, 346, f10 CrossRef PubMed.
- C. Werner and U. Laufs, Moving beyond the “LDL hypothesis”, VASA Zeitschrift für Gefässkrankheiten, 2015, 44(5), 333–340 CrossRef PubMed.
- A. D. Hendrani, T. Adesiyun, R. Quispe, S. R. Jones, N. J. Stone and R. S. Blumenthal,
et al., Dyslipidemia management in primary prevention of cardiovascular disease: Current guidelines and strategies, World J. Cardiol., 2016, 8(2), 201–210 CrossRef PubMed.
- D. Ettehad, C. A. Emdin, A. Kiran, S. G. Anderson, T. Callender and J. Emberson,
et al., Blood pressure lowering for prevention of cardiovascular disease and death: a systematic review and meta-analysis, Lancet, 2015, 387(10022), 957–967 CrossRef.
- C. G. Harmsen, H. Støvring, D. E. Jarbøl, J. Nexøe, D. Gyrd-Hansen and J. B. Nielsen,
et al., Medication effectiveness may not be the major reason for accepting
cardiovascular preventive medication: a population-based survey, BMC Med. Inf. Decis. Making, 2012, 12, 89 CrossRef PubMed.
- G. J. Njie, R. K. C. Finnie, S. D. Acharya, V. Jacob, K. K. Proia and D. P. Hopkins,
et al., Reducing Medication Costs to Prevent Cardiovascular Disease: A Community Guide Systematic Review, Prev. Chronic Dis., 2015, 12, E208 Search PubMed.
- J. Gatwood and J. E. Bailey, Improving medication adherence in hypercholesterolemia: challenges and solutions, Vasc. Health Risk Manag., 2014, 10, 615–625 CrossRef PubMed.
- H. Yanai, K. Ito, H. Yoshida and N. Tada, Antihypertensive effects of astaxanthin, Integr. Blood Pressure Control, 2008, 1, 1–3 CrossRef CAS.
- R. G. Fassett and J. S. Coombes, Astaxanthin in cardiovascular health and disease, Molecules, 2012, 17(2), 2030–2048 CrossRef CAS PubMed.
- Y. Kishimoto, H. Yoshida and K. Kondo, Potential Anti-Atherosclerotic Properties of Astaxanthin, Mar. Drugs, 2016, 14(2), 35 CrossRef PubMed.
- E. Yamashita, Let astaxanthin be thy medicine, PharmaNutrition, 2015, 3(4), 115–122 CrossRef CAS.
- W. Miki, Biological functions and activities of animal carotenoids, Pure Appl. Chem., 1991, 63(1), 141–146 CrossRef CAS.
- V. M. Moretti, T. Mentasti, F. Bellagamba, U. Luzzana, F. Caprino and G. M. Turchini,
et al., Determination of astaxanthin stereoisomers and colour attributes in flesh of rainbow trout (Oncorhynchus mykiss) as a tool to distinguish the dietary pigmentation source, Food Addit. Contam., 2006, 23(11), 1056–1063 CrossRef CAS PubMed.
- I. Higuera-Ciapara, L. Félix-Valenzuela and F. M. Goycoolea, Astaxanthin: a review of its chemistry and applications, Crit. Rev. Food Sci. Nutr., 2006, 46(2), 185–196 CrossRef CAS PubMed.
- R. R. Ambati, S. M. Phang, S. Ravi and R. G. Aswathanarayana, Astaxanthin: sources, extraction, stability, biological activities and its commercial applications–a review, Mar. Drugs, 2014, 12(1), 128–152 CrossRef PubMed.
- S. A. Turujman, W. G. Wamer, R. R. Wei and R. H. Albert, Rapid liquid chromatographic method to distinguish wild salmon from aquacultured salmon fed synthetic astaxanthin, J. AOAC Int., 1997, 80(3), 622–632 CAS.
-
S. Hall, A. Delaporte, M. Phillips, M. Beveridge and M. O'Keefe, Blue Frontiers: Managing the Environmental Costs of Aquaculture. Penang, The WorldFish Center, Malaysia, 2011.
- P. A. Megdal, N. A. Craft and G. J. Handelman, A simplified method to distinguish farmed (Salmo salar) from wild salmon: fatty acid ratios versus astaxanthin chiral isomers, Lipids, 2009, 44(6), 569–576 CrossRef CAS PubMed.
- Y.-Y. Chen, P.-C. Lee, Y.-L. Wu and L.-Y. Liu, In Vivo Effects of Free Form Astaxanthin Powder on Anti-Oxidation and Lipid Metabolism with High-Cholesterol Diet, PLoS One, 2015, 10(8), e0134733 Search PubMed.
- M. M. R. Shah, Y. Liang, J. J. Cheng and M. Daroch, Astaxanthin-Producing Green Microalga Haematococcus pluvialis: From Single Cell to High Value Commercial Products, Front. Plant Sci., 2016, 7, 531 Search PubMed.
- M. Rodríguez-Sáiz, J. L. de la Fuente and J. L. Barredo, Xanthophyllomyces dendrorhous for the industrial production of astaxanthin, Appl. Microbiol. Biotechnol., 2010, 88(3), 645–658 CrossRef PubMed.
- A. Tsubokura, H. Yoneda and H. Mizuta, Paracoccus carotinifaciens sp. nov., a new aerobic gram-negative astaxanthin-producing bacterium, Int. J. Syst. Bacteriol., 1999, 49(Pt 1), 277–282 CrossRef CAS PubMed.
- EFSA FEEDAP Panel, Scientific Opinion of the Panel on Additives and Products or Substances used in Animal Feed (FEEDAP) on a request from the European Commission on safety and efficacy of Panaferd-AX (red carotenoid-rich bacterium Paracoccus carotinifaciens) as feed additiv, EFSA J., 2007, 546, 1–30 Search PubMed.
- EFSA FEEDAP Panel, Scientific Opinion on the safety and efficacy of astaxanthin (CAROPHYLL® Pink 10% CWS) for salmonids and ornamental fish, EFSA J., 2014, 12(6), 3725 CrossRef.
- H. Tapiero, D. M. Townsend and K. D. Tew, The role of carotenoids in the prevention of human pathologies, Biomed. Pharmacother., 2004, 58(2), 100–110 CrossRef CAS PubMed.
- M. A. Gammone, G. Riccioni and N. D'Orazio, Carotenoids: potential allies of cardiovascular health?, Food Nutr. Res., 2015, 59, 26762 Search PubMed.
- R. S. Parker, Absorption, metabolism, and transport of carotenoids, FASEB J., 1996, 10(5), 542–551 CAS.
- R. M. Clark, L. Yao, L. She and H. C. Furr, A comparison of lycopene and astaxanthin absorption from corn oil and olive oil emulsions, Lipids, 2000, 35(7), 803–806 CrossRef CAS PubMed.
- J. Fiedor and K. Burda, Potential role of carotenoids as antioxidants in human health and disease, Nutrients, 2014, 6(2), 466–488 CrossRef PubMed.
- Y. Okada, M. Ishikura and T. Maoka, Bioavailability of astaxanthin in Haematococcus algal extract: the effects of timing of diet and smoking habits, Biosci., Biotechnol., Biochem., 2009, 73(9), 1928–1932 CrossRef CAS PubMed.
- L. A. Showalter, S. A. Weinman, M. Østerlie and S. F. Lockwood, Plasma appearance and tissue accumulation of non-esterified, free astaxanthin in C57BL/6 mice after oral dosing of a disodium disuccinate diester of astaxanthin (Heptax), Comp. Biochem. Physiol., Part C: Toxicol. Pharmacol., 2004, 137(3), 227–236 CrossRef PubMed.
- A. Ranga-Rao, R. L. Raghunath-Reddy, V. Baskaran, R. Sarada and G. A. Ravishankar, Characterization of microalgal carotenoids by mass spectrometry and their bioavailability and antioxidant properties elucidated in rat model, J. Agric. Food Chem., 2010, 58(15), 8553–8559 CrossRef CAS PubMed.
- J. S. Park, H. W. Kim, B. D. Mathison, M. G. Hayek, S. Massimino and G. A. Reinhart,
et al., Astaxanthin uptake in domestic dogs and cats, Nutr. Metab., 2010, 7, 52 Search PubMed.
- EFSA NDA Panel, Scientific Opinion on the safety of astaxanthin-rich ingredients (AstaREAL A1010 and AstaREAL L10) as novel food ingredients, EFSA J., 2014, 12(7), 3757 CrossRef.
- G. N. Coral-Hinostroza, T. Ytrestøyl, B. Ruyter and B. Bjerkeng, Plasma appearance of unesterified astaxanthin geometrical E/Z and optical R/S isomers in men given single doses of a mixture of optical 3 and 3′R/S isomers of astaxanthin fatty acyl diesters, Comp. Biochem. Physiol., Part C: Toxicol. Pharmacol., 2004, 139(1–3), 99–110 CrossRef PubMed.
- C. E. Rüfer, J. Moeseneder, K. Briviba, G. Rechkemmer and A. Bub, Bioavailability of astaxanthin stereoisomers from wild (Oncorhynchus spp.) and aquacultured (Salmo salar) salmon in healthy men: a randomised, double-blind study, Br. J. Nutr., 2008, 99(5), 1048–1054 CrossRef PubMed.
- E. Wolz, H. Liechti, B. Notter, G. Oesterhelt and A. Kistler, Characterization of metabolites of astaxanthin in primary cultures of rat hepatocytes, Drug Metab. Dispos., 1999, 27(4), 456–462 CAS.
- A. Kistler, H. Liechti, L. Pichard, E. Wolz, G. Oesterhelt and A. Hayes,
et al., Metabolism and CYP-inducer properties of astaxanthin in man and primary human hepatocytes, Arch. Toxicol., 2002, 75(11–12), 665–675 CrossRef CAS PubMed.
- H. Kurihara, H. Kodo, S. Asami, Y. Kiso and T. Tanaka, Contribution of the antioxidative property of astaxanthin to its protective effect on the promotion of cancer metastasis in mice treated with restraint stress, Life Sci., 2002, 70, 2509–2520 CrossRef CAS PubMed.
- M. Østerlie, B. Bjerkeng and S. Liaaen-Jensen, Plasma appearance and distribution of astaxanthin E/Z and R/S isomers in plasma lipoproteins of men after single dose administration of astaxanthin, J. Nutr. Biochem., 2000, 11(10), 482–490 CrossRef.
- J. S. Park, J. H. Chyun, Y. K. Kim, L. L. Line and B. P. Chew, Astaxanthin decreased oxidative stress and inflammation and enhanced immune response in humans, Nutr. Metab., 2010, 7, 18 Search PubMed.
- H. Sies, Biochemistry of oxidative stress, Angew. Chem., Int. Ed. Engl., 1986, 25, 1058–1071 CrossRef.
- B. Halliwell, Antioxidants in human health and disease, Annu. Rev. Nutr., 1996, 16, 33–50 CrossRef CAS PubMed.
-
H. Sies, Oxidative Stress II. Oxidants and Antioxidants, Academic Press, London, 1991 Search PubMed.
- D. Jones and H. Sies, The redox code, Antioxid. Redox Signaling, 2015, 23(9), 734–746 CrossRef CAS PubMed.
- W. Stahl and H. Sies, Antioxidant activity of carotenoids, Mol. Aspects Med., 2003, 24, 345–351 CrossRef CAS PubMed.
- K. Jørgensen and L. H. Skibsted, Carotenoid scavenging of radicals. Effect of carotenoid structure and oxygen partial pressure on antioxidative activity, Zeitschrift für Leb und -forsch, 1993, 196(5), 423–429 CrossRef.
- A. Mortensen, L. H. Skibsted and T. G. Truscott, The interaction of dietary carotenoids with radical species, Arch. Biochem. Biophys., 2001, 385(1), 13–19 CrossRef CAS PubMed.
- B. Capelli, D. Bagchi and G. Cysewski, Synthetic astaxanthin is significantly inferior to algal-based astaxanthin as an antioxidant and may not be suitable as a human nutritional supplement, NutraFoods, 2013, 12, 145–152 CrossRef CAS.
- J. Dose, S. Matsugo, H. Yokokawa, Y. Koshida, S. Okazaki and U. Seidel,
et al., Free Radical Scavenging and Cellular Antioxidant Properties of Astaxanthin, Int. J. Mol. Sci., 2016, 17(1) DOI:10.3390/ijms17010103.
- O. I. Aruoma, Methodological considerations for characterizing potential antioxidant actions of bioactive components in plant foods, Mutat. Res., 2003, 523–524, 9–20 CrossRef CAS PubMed.
- B. P. Lim, A. Nagao, J. Terao, K. Tanaka, T. Suzuki and K. Takama, Antioxidant activity of xanthophylls on peroxyl radical-mediated phospholipid peroxidation, Biochim. Biophys. Acta, 1992, 1126(2), 178–184 CAS.
- N. Shimidzu, M. Goto and W. Miki, Carotenoids as singlet oxygen quenchers in marine organisms, Fish. Sci., 1996, 62(1), 134–137 CAS.
- T. Iwamoto, K. Hosoda, R. Hirano, H. Kurata, A. Matsumoto and W. Miki,
et al., Inhibition of low-density lipoprotein oxidation by astaxanthin, J. Atheroscler. Thromb., 2000, 7(4), 216–222 CrossRef CAS PubMed.
- S. Goto, K. Kogure, K. Abe, Y. Kimata, K. Kitahama and E. Yamashita,
et al., Efficient radical trapping at the surface and inside the phospholipid membrane is responsible for highly potent antiperoxidative activity of the carotenoid astaxanthin, Biochim. Biophys. Acta, 2001, 1512(2), 251–258 CrossRef CAS.
- Y. Nishida, E. Yamashita and W. Miki, Quenching activities of common hydrophilic and lipophilic antioxidants against singlet oxygen using chemiluminescence detection system, Carotenoid Sci., 2007, 11, 16–20 Search PubMed.
- S. Hama, S. Uenishi, A. Yamada, T. Ohgita, H. Tsuchiya and E. Yamashita,
et al., Scavenging of hydroxyl radicals in aqueous solution by astaxanthin encapsulated in liposomes, Biol. Pharm. Bull., 2012, 35(12), 2238–2242 CAS.
- Y. Sueishi, M. Ishikawa, D. Yoshioka, N. Endoh, S. Oowada and M. Shimmei,
et al., Oxygen radical absorbance capacity (ORAC) of cyclodextrin-solubilized flavonoids, resveratrol and astaxanthin as measured with the ORAC-EPR method, J. Clin. Biochem. Nutr., 2012, 50(2), 127–132 CrossRef CAS PubMed.
- C.-S. Chang, C.-L. Chang and G.-H. Lai, Reactive oxygen species scavenging activities in a chemiluminescence model and neuroprotection in rat pheochromocytoma cells by astaxanthin, beta-carotene, and canthaxanthin, Kaohsiung J. Med. Sci., 2013, 29(8), 412–421 CrossRef PubMed.
- P. Régnier, J. Bastias, V. Rodriguez-Ruiz, N. Caballero-Casero, C. Caballo and D. Sicilia,
et al., Astaxanthin from Haematococcus pluvialis Prevents Oxidative Stress on Human Endothelial Cells without Toxicity, Mar. Drugs, 2015, 13(5), 2857–2874 CrossRef PubMed.
- I. Nishigaki, P. Rajendran, R. Venugopal, G. Ekambaram, D. Sakthisekaran and Y. Nishigaki, Cytoprotective role of astaxanthin
against glycated protein/iron chelate-induced toxicity in human umbilical vein endothelial cells, Phytother. Res., 2010, 24(1), 54–59 CrossRef CAS PubMed.
- L. A. Abdelzaher, T. Imaizumi, T. Suzuki, K. Tomita, M. Takashina and Y. Hattori, Astaxanthin alleviates oxidative stress insults-related derangements in human vascular endothelial cells exposed to glucose fluctuations, Life Sci., 2016, 150, 24–31 CrossRef CAS PubMed.
- L. Speranza, M. Pesce, A. Patruno, S. Franceschelli, M. A. de Lutiis and A. Grilli,
et al., Astaxanthin treatment reduced oxidative induced pro-inflammatory cytokines secretion in U937: SHP-1 as a novel biological target, Mar. Drugs, 2012, 10(4), 890–899 CrossRef CAS PubMed.
- S. Franceschelli, M. Pesce, A. Ferrone, M. De Lutiis, A. Patruno and A. Grilli,
et al., Astaxanthin treatment confers protection against oxidative stress in U937 cells stimulated with lipopolysaccharide reducing O2- production, PLoS One, 2014, 9(2), e88359 Search PubMed.
- V. I. Zannis, F. S. Cole, C. L. Jackson, D. M. Kurnit and S. K. Karathanasis, Distribution of apolipoprotein A-I, C-II, C-III, and E mRNA in fetal human tissues. Time-dependent induction of apolipoprotein E mRNA by cultures of human monocyte-macrophages, Biochemistry, 1985, 24(16), 4450–4455 CrossRef CAS PubMed.
- G. J. Gross, S. L. Hazen and S. F. Lockwood, Seven day oral supplementation with Cardax (disodium disuccinate astaxanthin) provides significant cardioprotection and reduces oxidative stress in rats, Mol. Cell. Biochem., 2006, 283(1–2), 23–30 CrossRef CAS PubMed.
- Z.-W. Zhao, W. Cai, Y.-L. Lin, Q.-F. Lin, Q. Jiang and Z. Lin,
et al., Ameliorative effect of astaxanthin on endothelial dysfunction in streptozotocin-induced diabetes in male rats, Arzneimittelforschung, 2011, 61(4), 239–246 CrossRef CAS PubMed.
- F. Visioli, Rusting the pipes: ingestion of oxidized lipids and vascular disease, Vascul. Pharmacol., 2014, 62(1), 47–48 CrossRef CAS PubMed.
- L. S. Jacobsson, X. M. Yuan, B. Ziedén and A. G. Olsson, Effects of alpha-tocopherol and astaxanthin on LDL oxidation and atherosclerosis in WHHL rabbits, Atherosclerosis, 2004, 173(2), 231–237 CAS.
- J. L. Vierck and M. V. Dodson, Interpretation of cell culture phenomena, Methods Cell Sci., 2000, 22(1), 79–81 CrossRef CAS PubMed.
- S. Schaffer, V. Y. M. Lam, I. M. A. Ernst, P. Huebbe, G. Rimbach and B. Halliwell, Variability in APOE genotype status in human-derived cell lines: a cause for concern in cell culture studies?, Genes Nutr., 2014, 9(1), 364 CrossRef PubMed.
- B. Halliwell, Cell culture, oxidative stress, and antioxidants: avoiding pitfalls, Biomed. J., 2014, 37(3), 99–105 Search PubMed.
- A. J. Michels and B. Frei, Myths, artifacts, and fatal flaws: identifying limitations and opportunities in vitamin C research, Nutrients, 2013, 5(12), 5161–5192 CrossRef CAS PubMed.
- I. Baralic, M. Andjelkovic, B. Djordjevic, N. Dikic, N. Radivojevic and V. Suzin-Zivkovic,
et al., Effect of Astaxanthin Supplementation on Salivary IgA, Oxidative Stress, and Inflammation in Young Soccer Players, Evidence-Based Complementary Altern. Med., 2015, 2015, 783761 Search PubMed.
- H. D. Choi, J. H. Kim, M. J. Chang, Y. Kyu-Youn and W. G. Shin, Effects of astaxanthin on oxidative stress in overweight and obese adults, Phytother. Res., 2011, 25(12), 1813–1818 CrossRef CAS PubMed.
- J. S. Coombes, J. E. Sharman and R. G. Fassett, Astaxanthin has no effect on arterial stiffness, oxidative stress, or inflammation in renal transplant recipients: a randomized controlled trial (the XANTHIN trial), Am. J. Clin. Nutr., 2016, 103(1), 283–289 CrossRef CAS PubMed.
- T. W. Stief, The physiology and pharmacology of singlet oxygen, Med. Hypotheses, 2003, 60(4), 567–572 CrossRef CAS PubMed.
- A. N. Onyango, Endogenous Generation of Singlet Oxygen and Ozone in Human and Animal Tissues: Mechanisms, Biological Significance, and Influence of Dietary Components, Oxid. Med. Cell. Longevity, 2016, 2016, 2398573 Search PubMed.
- F. Böhm, R. Edge and T. G. Truscott, Interactions of dietary carotenoids with singlet oxygen (1O2) and free radicals: potential effects for human health, Acta Biochim. Pol., 2012, 59(1), 27–30 Search PubMed.
- M. C. Toufektsian, F. R. Boucher, S. Tanguy, S. Morel and J. G. de Leiris, Cardiac toxicity of singlet oxygen: implication in reperfusion injury, Antioxid. Redox Signaling, 2001, 3(1), 63–69 CrossRef CAS PubMed.
- J. Chen, M. Jarvi, P.-C. Lo, K. Stefflova, B. C. Wilson and G. Zheng, Using the singlet oxygen scavenging property of carotenoid in photodynamic molecular beacons to minimize photodamage to non-targeted cells, Photochem. Photobiol. Sci., 2007, 6(12), 1311–1317 CAS.
- A. V. Rao and L. G. Rao, Carotenoids and human health, Pharmacol. Res., 2007, 55(3), 207–216 CrossRef CAS PubMed.
- G. N. Bosio, T. Breitenbach, J. Parisi, M. Reigosa, F. H. Blaikie and B. W. Pedersen,
et al., Antioxidant β-carotene does not quench singlet oxygen in mammalian cells, J. Am. Chem. Soc., 2013, 135(1), 272–279 CrossRef CAS PubMed.
- T. W. Stief, Is singlet oxygen antiatherosclerotic?, Am. J. Pathol., 2001, 158(2), 781–782 CrossRef CAS PubMed.
- D. Vara and G. Pula, Reactive oxygen species: physiological roles in the regulation of vascular cells, Curr. Mol. Med., 2014, 14(9), 1103–1125 CrossRef CAS PubMed.
- T. M. van Himbergen, Y. T. van der Schouw, H. A. M. Voorbij, L. J. H. van Tits, A. F. H. Stalenhoef and P. H. M. Peeters,
et al., Paraoxonase (PON1) and the risk for coronary heart disease and myocardial infarction in a general population of Dutch women, Atherosclerosis, 2008, 199(2), 408–414 CrossRef CAS PubMed.
- P. Kresanov, T. Vasankari, M. Ahotupa, J. Kaikkonen, N. Hutri-Kähönen and M. Juonala,
et al., Paraoxonase-1 and oxidized lipoprotein lipids. The Cardiovascular Risk in Young Finns Study, Atherosclerosis, 2015, 241(2), 502–506 CrossRef CAS PubMed.
- M. Aviram and J. Vaya, Paraoxonase 1 activities, regulation, and interactions with atherosclerotic lesion, Curr. Opin. Lipidol., 2013, 24(4), 339–344 CrossRef CAS PubMed.
- P. R. Augusti, A. Quatrin, S. Somacal, G. M. Conterato, R. Sobieski and A. R. Ruviaro,
et al., Astaxanthin prevents changes in the activities of thioredoxin reductase and paraoxonase in hypercholesterolemic rabbits, J. Clin. Biochem. Nutr., 2012, 51(1), 42–49 CrossRef CAS PubMed.
- I. Baralic, B. Djordjevic, N. Dikic, J. Kotur-Stevuljevic, S. Spasic and Z. Jelic-Ivanovic,
et al., Effect of astaxanthin supplementation on paraoxonase 1 activities and oxidative stress status in young soccer players, Phytother. Res., 2013, 27(10), 1536–1542 CAS.
- A. Kaulmann and T. Bohn, Carotenoids, inflammation, and oxidative stress–implications of cellular signaling pathways and relation to chronic disease prevention, Nutr. Res., 2014, 34(11), 907–929 CrossRef CAS PubMed.
- B. Chen, Y. Lu, Y. Chen and J. Cheng, The role of Nrf2 in oxidative stress-induced endothelial injuries, J. Endocrinol., 2015, 225(3), R83–R99 CrossRef CAS PubMed.
- H. Yao, X. Han and X. Han, The cardioprotection of the insulin-mediated PI3K/Akt/mTOR signaling pathway, Am. J. Cardiovasc. Drugs, 2014, 14(6), 433–442 CrossRef CAS PubMed.
- M. A. O'Connell and J. D. Hayes, The Keap1/Nrf2 pathway in health and disease: from the bench to the clinic, Biochem. Soc. Trans., 2015, 43(4), 687–689 CrossRef PubMed.
- B. Choi, K.-S. Kang and M.-K. Kwak, Effect of redox modulating NRF2 activators on chronic kidney disease, Molecules, 2014, 19(8), 12727–12759 CrossRef PubMed.
- G. Joshi and J. A. Johnson, The Nrf2-ARE pathway: a valuable therapeutic target for the treatment of neurodegenerative diseases, Recent Pat. CNS Drug Discovery, 2012, 7(3), 218–229 CrossRef CAS PubMed.
- N. Kweider, B. Huppertz, M. Kadyrov, W. Rath, T. Pufe and C. J. Wruck, A possible protective role of Nrf2 in preeclampsia, Ann. Anat., 2014, 196(5), 268–277 CrossRef PubMed.
- P. Arnold, D. Mojumder, J. Detoledo, R. Lucius and H. Wilms, Pathophysiological processes in multiple sclerosis: focus on nuclear factor erythroid-2-related factor 2 and emerging pathways, Clin. Pharmacol., 2014, 6, 35–42 Search PubMed.
- F. Visioli, Xenobiotics and human health: A new view of their pharma-nutritional role, PharmaNutrition, 2015, 3(2), 60–64 CrossRef CAS.
- M. C. Crespo, J. Tomé-Carneiro, E. Burgos-Ramos, V. Loria Kohen, M. I. Espinosa and J. Herranz,
et al., One-week administration of hydroxytyrosol to humans does not activate Phase II enzymes, Pharmacol. Res., 2015, 95–96, 132–137 CrossRef CAS PubMed.
- A. Ben-Dor, M. Steiner, L. Gheber, M. Danilenko, N. Dubi and K. Linnewiel,
et al., Carotenoids activate the antioxidant response element transcription system, Mol. Cancer Ther., 2005, 4(1), 177–186 CAS.
- T. Esatbeyoglu, K. Ulbrich, C. Rehberg, S. Rohn and G. Rimbach, Thermal stability, antioxidant, and anti-inflammatory activity of curcumin and its degradation product 4-vinyl guaiacol, Food Funct., 2015, 6(3), 887–893 CAS.
- C. L. L. Saw, A. Y. Yang, Y. Guo and A.-N. T. Kong, Astaxanthin and omega-3 fatty acids individually and in combination protect against oxidative stress via the Nrf2-ARE pathway, Food Chem. Toxicol., 2013, 62, 869–875 CrossRef CAS PubMed.
- Q. Ye, B. Huang, X. Zhang, Y. Zhu and X. Chen, Astaxanthin protects against MPP(+)-induced oxidative stress in PC12 cells via the HO-1/NOX2 axis, BMC Neurosci., 2012, 13, 156 CrossRef CAS PubMed.
- Z. Li, X. Dong, H. Liu, X. Chen, H. Shi and Y. Fan,
et al., Astaxanthin protects ARPE-19 cells from oxidative stress via upregulation of Nrf2-regulated phase II enzymes through activation of PI3K/Akt, Mol. Vision, 2013, 19, 1656–1666 CAS.
- D. N. Tripathi and G. B. Jena, Astaxanthin intervention ameliorates cyclophosphamide-induced oxidative stress, DNA damage and early hepatocarcinogenesis in rat: role of Nrf2, p53, p38 and phase-II enzymes, Mutat. Res., 2010, 696(1), 69–80 CAS.
- Q. Wu, X.-S. Zhang, H.-D. Wang, X. Zhang, Q. Yu and W. Li,
et al., Astaxanthin activates nuclear factor erythroid-related factor 2 and the antioxidant responsive element (Nrf2-ARE) pathway in the brain after subarachnoid hemorrhage in rats and attenuates early brain injury, Mar. Drugs, 2014, 12(12), 6125–6141 CrossRef PubMed.
- A. S. Jiménez-Osorio, W. R. García-Niño, S. González-Reyes, A. E. Álvarez-Mejía, S. Guerra-León and J. Salazar-Segovia,
et al., The Effect of Dietary Supplementation With Curcumin on Redox Status and Nrf2 Activation in Patients With Nondiabetic or Diabetic Proteinuric Chronic Kidney Disease: A Pilot Study, J. Renal. Nutr., 2016, 26(4), 237–244 CrossRef PubMed.
- R. Ross, The pathogenesis of atherosclerosis–an update, N. Engl. J. Med., 1986, 314(8), 488–500 CrossRef CAS PubMed.
- C. Gómez-Guerrero, B. Mallavia and J. Egido, Targeting inflammation in cardiovascular diseases. still a neglected field?, Cardiovasc. Ther., 2012, 30(4), e189–e197 CrossRef PubMed.
- M. Bäck, C. Weber and E. Lutgens, Regulation of atherosclerotic plaque inflammation, J. Intern. Med., 2015, 278(5), 462–482 CrossRef PubMed.
- M. Y. Donath, Targeting inflammation in the treatment of type 2 diabetes: time to start, Nat. Rev. Drug Discovery, 2014, 13(6), 465–476 CrossRef CAS PubMed.
- Y. Solak, B. Afsar, N. D. Vaziri, G. Aslan, C. E. Yalcin and A. Covic,
et al., Hypertension as an autoimmune and inflammatory disease, Hypertens. Res., 2016, 39(8), 567–573 CrossRef CAS PubMed.
- V. Guarner and M. E. Rubio-Ruiz, Low-grade systemic inflammation connects aging, metabolic syndrome and cardiovascular disease, Interdiscip. Top. Gerontol., 2015, 40, 99–106 Search PubMed.
- A. R. Tall and L. Yvan-Charvet, Cholesterol, inflammation and innate immunity, Nat. Rev. Immunol., 2015, 15(2), 104–116 CrossRef CAS PubMed.
- F. Strang and H. Schunkert, C-reactive protein and coronary heart disease: all said–is not it?, Mediators Inflammation, 2014, 2014, 757123 Search PubMed.
- O. Zimmermann, K. Li, M. Zaczkiewicz, M. Graf, Z. Liu and J. Torzewski, C-reactive protein in human atherogenesis: facts and fiction, Mediators Inflammation, 2014, 2014, 561428 Search PubMed.
- S. D. Santos, T. B. Cahú, G. O. Firmino, C. C. M. M. B. de Castro, L. B. Carvalho and R. S. Bezerra,
et al., Shrimp waste extract and astaxanthin: rat alveolar macrophage, oxidative stress and inflammation, J. Food Sci., 2012, 77(7), H141–H146 CrossRef CAS PubMed.
- Y. Kishimoto, M. Tani, H. Uto-Kondo, M. Iizuka, E. Saita and H. Sone,
et al., Astaxanthin suppresses scavenger receptor expression and matrix metalloproteinase activity in macrophages, Eur. J. Nutr., 2010, 49(2), 119–126 CrossRef CAS PubMed.
- S.-J. Lee, S.-K. Bai, K.-S. Lee, S. Namkoong, H.-J. Na and K.-S. Ha,
et al., Astaxanthin inhibits nitric oxide production and inflammatory gene expression by suppressing I(kappa)B kinase-dependent NF-kappaB activation, Mol. Cells, 2003, 16(1), 97–105 CAS.
- Y. J. Kim, Y. A. Kim and T. Yokozawa, Protection against oxidative stress, inflammation, and apoptosis of high-glucose-exposed proximal tubular epithelial cells by astaxanthin, J. Agric. Food Chem., 2009, 57(19), 8793–8797 CrossRef CAS PubMed.
- T. R. Campoio, F. A. Oliveira and R. Otton, Oxidative stress in human lymphocytes treated with fatty acid mixture: role of carotenoid astaxanthin, Toxicol. in Vitro, 2011, 25(7), 1448–1456 CrossRef CAS PubMed.
- S. Ravi Kumar, B. Narayan, Y. Sawada, M. Hosokawa and K. Miyashita, Combined effect of astaxanthin and squalene on oxidative stress in vivo, Mol. Cell. Biochem., 2016, 417(1–2), 57–65 CrossRef CAS PubMed.
- K. Takahashi, T. Takimoto, K. Sato and Y. Akiba, Effect of dietary supplementation of astaxanthin from Phaffia rhodozyma on lipopolysaccharide-induced early inflammatory responses in male broiler chickens (Gallus gallus) fed a corn-enriched diet, Anim. Sci. J., 2011, 82(6), 753–758 CrossRef CAS PubMed.
- J. Li, F. Wang, Y. Xia, W. Dai, K. Chen and S. Li,
et al., Astaxanthin Pretreatment Attenuates Hepatic Ischemia Reperfusion-Induced Apoptosis and Autophagy via the ROS/MAPK Pathway in Mice, Mar. Drugs, 2015, 13(6), 3368–3387 CrossRef CAS PubMed.
- X. Qiu, K. Fu, X. Zhao, Y. Zhang, Y. Yuan and S. Zhang,
et al., Protective effects of astaxanthin against ischemia/reperfusion induced renal injury in mice, J. Transl. Med., 2015, 13, 28 CrossRef PubMed.
- S. Bhuvaneswari, B. Yogalakshmi, S. Sreeja and C. V. Anuradha, Astaxanthin reduces hepatic endoplasmic reticulum stress and nuclear factor-κB-mediated inflammation in high fructose and high fat diet-fed mice, Cell Stress Chaperones, 2014, 19(2), 183–191 CrossRef CAS PubMed.
- R. Nakao, O. L. Nelson, J. S. Park, B. D. Mathison, P. A. Thompson and B. P. Chew, Effect of astaxanthin supplementation on inflammation and cardiac function in BALB/c mice, Anticancer Res., 2010, 30(7), 2721–2725 CAS.
- G. Liu, Y. Shi, X. Peng, H. Liu, Y. Peng and L. He, Astaxanthin attenuates adriamycin-induced focal segmental glomerulosclerosis, Pharmacology, 2015, 95(3–4), 193–200 CrossRef CAS PubMed.
- K.-C. Chan, P.-J. Pen and M.-C. Yin, Anticoagulatory and antiinflammatory effects of astaxanthin in diabetic rats, J. Food Sci., 2012, 77(2), H76–H80 CrossRef CAS PubMed.
- B. P. Chew, B. D. Mathison, M. G. Hayek, S. Massimino, G. A. Reinhart and J. S. Park, Dietary astaxanthin enhances immune response in dogs, Vet. Immunol. Immunopathol., 2011, 140(3–4), 199–206 CrossRef CAS PubMed.
- E. Arunkumar, S. Bhuvaneswari and C. V. Anuradha, An intervention study in obese mice with astaxanthin, a marine carotenoid–effects on insulin signaling and pro-inflammatory cytokines, Food Funct., 2012, 3(2), 120–126 CAS.
- Y. Ni, M. Nagashimada, F. Zhuge, L. Zhan, N. Nagata and A. Tsutsui,
et al., Astaxanthin prevents and reverses diet-induced insulin resistance and steatohepatitis in mice: A comparison with vitamin E, Sci. Rep., 2015, 5, 17192 CrossRef CAS PubMed.
- N. R. Poulter, D. Prabhakaran and M. Caulfield, Hypertension, Lancet, 2015, 386(9995), 801–812 CrossRef.
- P. M. Kearney, M. Whelton, K. Reynolds, P. Muntner, P. K. Whelton and J. He, Global burden of hypertension: analysis of worldwide data, Lancet, 2005, 365(9455), 217–223 CrossRef.
- D. T. Lackland and M. A. Weber, Global burden of cardiovascular disease and stroke: hypertension at the core, Can. J. Cardiol., 2015, 31(5), 569–571 CrossRef PubMed.
- M. Singh, G. A. Mensah and G. Bakris, Pathogenesis and clinical physiology of hypertension, Cardiol. Clin., 2010, 28(4), 545–559 CrossRef PubMed.
- G. R. Crislip and J. C. Sullivan, T-cell involvement in sex differences in blood pressure control, Clin. Sci., 2016, 130(10), 773–783 CrossRef CAS PubMed.
- M. H. Smolensky, R. C. Hermida and F. Portaluppi, Circadian mechanisms of 24-hour blood pressure regulation and patterning, Sleep Med. Rev., 2016 DOI:10.1016/j.smrv.2016.02.003.
- H. G. Preuss, B. Echard, E. Yamashita and N. V. Perricone, High dose astaxanthin lowers blood pressure and increases insulin sensitivity in rats: are these effects interdependent?, Int. J. Med. Sci., 2011, 8(2), 126–138 CrossRef CAS PubMed.
- Y. Sasaki, N. Kobara, S. Higashino, J. C. Giddings and J. Yamamoto, Astaxanthin inhibits thrombosis in cerebral vessels of stroke-prone spontaneously hypertensive rats, Nutr. Res., 2011, 31(10), 784–789 CrossRef CAS PubMed.
- G. Hussein, H. Goto, S. Oda, T. Iguchi, U. Sankawa and K. Matsumoto,
et al., Antihypertensive potential and mechanism of action of astaxanthin: II. Vascular reactivity and hemorheology in spontaneously hypertensive rats, Biol. Pharm. Bull., 2005, 28(6), 967–971 CAS.
- G. Hussein, H. Goto, S. Oda, U. Sankawa, K. Matsumoto and H. Watanabe, Antihypertensive potential and mechanism of action of astaxanthin: III. Antioxidant and histopathological effects in spontaneously hypertensive rats, Biol. Pharm. Bull., 2006, 29(4), 684–688 CAS.
- G. A. Spiller and A. Dewell, Safety of an astaxanthin-rich Haematococcus pluvialis algal extract: a randomized clinical trial, J. Med. Food, 2003, 6(1), 51–56 CrossRef CAS PubMed.
- G. Hussein, T. Nakagawa, H. Goto, Y. Shimada, K. Matsumoto and U. Sankawa,
et al., Astaxanthin ameliorates features of metabolic syndrome in SHR/NDmcr-cp, Life Sci., 2007, 80(6), 522–529 CrossRef CAS PubMed.
- J. Monroy-Ruiz, M.-Á. Sevilla, R. Carrón and M.-J. Montero, Astaxanthin-enriched-diet reduces blood pressure and improves cardiovascular parameters in spontaneously hypertensive rats, Pharmacol. Res., 2011, 63(1), 44–50 CrossRef CAS PubMed.
- A. C. Maritim, R. A. Sanders and J. B. Watkins, Diabetes, oxidative stress, and antioxidants: a review, J. Biochem. Mol. Toxicol., 2003, 17(1), 24–38 CrossRef CAS PubMed.
- N. G. Forouhi and N. J. Wareham, Epidemiology of diabetes, Medicine, 2014, 42(12), 698–702 CrossRef PubMed.
- B. M. Leon and T. M. Maddox, Diabetes and cardiovascular disease: Epidemiology, biological mechanisms, treatment recommendations and future research, World J. Diabetes, 2015, 6(13), 1246–1258 CrossRef PubMed.
- T. V. Fiorentino, A. Prioletta, P. Zuo and F. Folli, Hyperglycemia-induced oxidative stress and its role in diabetes mellitus related cardiovascular diseases, Curr. Pharm. Des., 2013, 19(32), 5695–5703 CrossRef CAS PubMed.
- S. Ursoniu, A. Sahebkar, M.-C. Serban and M. Banach, Systematic review/Meta-analysis Lipid profile and glucose changes after supplementation with astaxanthin: a systematic review and meta-analysis of randomized controlled trials, Arch. Med. Sci., 2015, 2(2), 253–266 CrossRef PubMed.
- S. Bhuvaneswari, E. Arunkumar, P. Viswanathan and C. V. Anuradha, Astaxanthin restricts weight gain, promotes insulin sensitivity and curtails fatty liver disease in mice fed a obesity-promoting diet, Process Biochem., 2010, 45, 1406–1414 CrossRef CAS.
- S. Bhuvaneswari and C. V. Anuradha, Astaxanthin prevents loss of insulin signaling and improves glucose metabolism in liver of insulin resistant mice, Can. J. Physiol. Pharmacol., 2012, 90(11), 1544–1552 CrossRef CAS PubMed.
- M. Ishiki, Y. Nishida, H. Ishibashi, T. Wada, S. Fujisaka and A. Takikawa,
et al., Impact of divergent effects of astaxanthin on insulin signaling in L6 cells, Endocrinology, 2013, 154(8), 2600–2612 CrossRef CAS PubMed.
- K. Uchiyama, Y. Naito, G. Hasegawa, N. Nakamura, J. Takahashi and T. Yoshikawa, Astaxanthin protects beta-cells against glucose toxicity in diabetic db/db mice, Redox Rep., 2002, 7(5), 290–293 CrossRef CAS PubMed.
- T. Shoji, T. Abe, H. Matsuo, G. Egusa, Y. Yamasaki and N. Kashihara,
et al., Chronic kidney disease, dyslipidemia, and atherosclerosis, J. Atheroscler. Thromb., 2012, 19(4), 299–315 CrossRef CAS PubMed.
- G. Ruiz-Hurtado, P. Sarafidis, M. S. Fernández-Alfonso, B. Waeber and L. M. Ruilope, Global cardiovascular protection in chronic kidney disease, Nat. Rev. Cardiol., 2016, 13(10), 603–608 CrossRef CAS PubMed.
- D. Noone and C. Licht, Chronic kidney disease: a new look at pathogenetic mechanisms and treatment options, Pediatr. Nephrol., 2014, 29(5), 779–792 CrossRef PubMed.
- R. G. Fassett, Current and emerging treatment options for the elderly patient with chronic kidney disease, Clin. Interventions Aging, 2014, 9, 191–199 CrossRef PubMed.
- P. R. Gajjala, M. Sanati and J. Jankowski, Cellular and Molecular Mechanisms of Chronic Kidney Disease with Diabetes Mellitus and Cardiovascular Diseases as Its Comorbidities, Front. Immunol., 2015, 6, 340 Search PubMed.
- J. L. Slavin and B. Lloyd, Health Benefits of Fruits and Vegetables, Adv. Nutr. An. Int. Rev. J., 2012, 3(4), 506–516 CrossRef CAS PubMed.
- A. J. Cooper, N. G. Forouhi, Z. Ye, B. Buijsse, L. Arriola and B. Balkau,
et al., Fruit and vegetable intake and type 2 diabetes: EPIC-InterAct prospective study and meta-analysis, Eur. J. Clin. Nutr., 2012, 66(10), 1082–1092 CrossRef CAS PubMed.
- H. Boeing, A. Bechthold, A. Bub, S. Ellinger, D. Haller and A. Kroke,
et al., Critical review: vegetables and fruit in the prevention of chronic diseases, Eur. J. Nutr., 2012, 51(6), 637–663 CrossRef CAS PubMed.
- D. Hariharan, K. Vellanki and H. Kramer, The Western Diet and Chronic Kidney Disease, Curr. Hypertens. Rep., 2015, 17(3), 16 CrossRef PubMed.
- A. Sila, Z. Ghlissi, Z. Kamoun, M. Makni, M. Nasri and A. Bougatef,
et al., Astaxanthin from shrimp by-products ameliorates nephropathy in diabetic rats, Eur. J. Nutr., 2015, 54(2), 301–307 CrossRef CAS PubMed.
- Y. Naito, K. Uchiyama, W. Aoi, G. Hasegawa, N. Nakamura and N. Yoshida,
et al., Prevention of diabetic nephropathy by treatment with astaxanthin in diabetic db/db mice, Biofactors, 2004, 20(1), 49–59 CrossRef CAS PubMed.
- E. Manabe, O. Handa, Y. Naito, K. Mizushima, S. Akagiri and S. Adachi,
et al., Astaxanthin protects mesangial cells from hyperglycemia-induced oxidative signaling, J. Cell. Biochem., 2008, 103(6), 1925–1937 CrossRef CAS PubMed.
- J. M. H. Galema-Boers and J. E. R. van Lennep, Dyslipidemia testing: Why, for whom and when, Maturitas, 2015, 81(4), 442–445 CrossRef CAS PubMed.
- Y. Yang, T. X. Pham, C. J. Wegner, B. Kim, C. S. Ku and Y.-K. Park,
et al., Astaxanthin lowers plasma TAG concentrations and increases hepatic antioxidant gene expression in diet-induced obesity mice, Br. J. Nutr., 2014, 112(11), 1797–1804 CrossRef CAS PubMed.
- H. Yoshida, H. Yanai, K. Ito, Y. Tomono, T. Koikeda and H. Tsukahara,
et al., Administration of natural astaxanthin increases serum HDL-cholesterol and adiponectin in subjects with mild hyperlipidemia, Atherosclerosis, 2010, 209(2), 520–523 CrossRef CAS PubMed.
- J. H. Stern, J. M. Rutkowski and P. E. Scherer, Adiponectin, Leptin, and Fatty Acids in the Maintenance of Metabolic Homeostasis through Adipose Tissue Crosstalk, Cell Metab., 2016, 23(5), 770–784 CrossRef CAS PubMed.
- L. M. Belalcazar, W. Lang, S. M. Haffner, R. C. Hoogeveen, F. X. Pi-Sunyer and D. C. Schwenke,
et al., Adiponectin and the mediation of HDL-cholesterol change with improved lifestyle: the Look AHEAD Study, J. Lipid Res., 2012, 53(12), 2726–2733 CrossRef CAS PubMed.
- R. B. Goldberg, M. Temprosa, L. Mele, T. Orchard, K. Mather and G. Bray,
et al., Change in adiponectin explains most of the change in HDL particles induced by lifestyle intervention but not metformin treatment in the Diabetes Prevention Program, Metabolism, 2016, 65(5), 764–775 CrossRef CAS PubMed.
- Y. Jia, C. Wu, J. Kim, B. Kim and S.-J. Lee, Astaxanthin reduces hepatic lipid accumulations in high-fat-fed C57BL/6J mice via activation of peroxisome proliferator-activated receptor (PPAR) alpha and inhibition of PPAR gamma and Akt, J. Nutr. Biochem., 2016, 28, 9–18 CrossRef CAS PubMed.
- Y. Yang, J. M. Seo, A. Nguyen, T. X. Pham, H. J. Park and Y. Park,
et al., Astaxanthin-rich extract from the green alga Haematococcus pluvialis lowers plasma lipid concentrations and enhances antioxidant defense in apolipoprotein E knockout mice, J. Nutr., 2011, 141(9), 1611–1617 CrossRef CAS PubMed.
- K. Mukai, S. Mitani, K. Ohara and S.-I. Nagaoka, Structure-activity relationship of the tocopherol-regeneration reaction by catechins, Free Radicals Biol. Med., 2005, 38(9), 1243–1256 CrossRef CAS PubMed.
- Y. Kadoma, M. Ishihara, N. Okada and S. Fujisawa, Free radical interaction between vitamin E (alpha-, beta-, gamma- and delta-tocopherol), ascorbate and flavonoids, In Vivo, 2006, 20(6B), 823–827 CAS.
- M. T. Schroeder, E. M. Becker and L. H. Skibsted, Molecular mechanism of antioxidant synergism of tocotrienols and carotenoids in palm oil, J. Agric. Food Chem., 2006, 54(9), 3445–3453 CrossRef CAS PubMed.
- F. Dai, W.-F. Chen and B. Zhou, Antioxidant synergism of green tea polyphenols with alpha-tocopherol and L-ascorbic acid in SDS micelles, Biochimie, 2008, 90(10), 1499–1505 CrossRef CAS PubMed.
- G. Fabre, I. Bayach, K. Berka, M. Paloncýová, M. Starok and C. Rossi,
et al., Synergism of antioxidant action of vitamins E, C and quercetin is related to formation of molecular associations in biomembranes, Chem. Commun., 2015, 51(36), 7713–7716 RSC.
- H.-H. Du, R. Liang, R.-M. Han, J.-P. Zhang and L. H. Skibsted, Astaxanthin Protecting Membrane Integrity against Photosensitized Oxidation through Synergism with Other Carotenoids, J. Agric. Food Chem., 2015, 63(41), 9124–9130 CrossRef CAS PubMed.
- L. H. Skibsted, Vitamin
an non-vitamin antioxidants and their interaction in food, J. Food Drug Anal., 2012, 20(Suppl. 1), 355–358 CAS.
- M. Nakano, A. Onodera, E. Saito, M. Tanabe, K. Yajima and J. Takahashi,
et al., Effect of astaxanthin in combination with alpha-tocopherol or ascorbic acid against oxidative damage in diabetic ODS rats, J. Nutr. Sci. Vitaminol., 2008, 54(4), 329–334 CrossRef CAS PubMed.
- E. Giordano and F. Visioli, Long-chain omega 3 fatty acids: molecular bases of potential antioxidant actions, Prostaglandins, Leukotrienes Essent. Fatty Acids, 2014, 90(1), 1–4 CrossRef CAS PubMed.
- W. Li, A. Hellsten, L. S. Jacobsson, H. M. Blomqvist, A. G. Olsson and X.-M. Yuan, Alpha-tocopherol and astaxanthin decrease macrophage infiltration, apoptosis and vulnerability in atheroma of hyperlipidaemic rabbits, J. Mol. Cell Cardiol., 2004, 37(5), 969–978 CrossRef CAS PubMed.
- P. R. Augusti, G. M. M. Conterato, S. Somacal, R. Sobieski, A. Quatrin and L. Maurer,
et al., Astaxanthin reduces oxidative stress, but not aortic damage in atherosclerotic rabbits, J. Cardiovasc. Pharmacol. Ther., 2009, 14(4), 314–322 CrossRef CAS PubMed.
- H. Shen, C.-C. Kuo, J. Chou, A. Delvolve, S. N. Jackson and J. Post,
et al., Astaxanthin reduces ischemic brain injury in adult rats, FASEB J., 2009, 23(6), 1958–1968 CrossRef CAS PubMed.
- Y.-P. Lu, S.-Y. Liu, H. Sun, X.-M. Wu, J.-J. Li and L. Zhu, Neuroprotective effect of astaxanthin on H(2)O(2)-induced neurotoxicity in vitro and on focal cerebral ischemia in vivo, Brain Res., 2010, 1360, 40–48 CrossRef CAS PubMed.
- S. K. Khan, T. Malinski, R. P. Mason, R. Kubant, R. F. Jacob and K. Fujioka,
et al., Novel astaxanthin prodrug (CDX-085) attenuates thrombosis in a mouse model, Thromb. Res., 2010, 126(4), 299–305 CrossRef CAS PubMed.
- S. K. Ryu, T. J. King, K. Fujioka, J. Pattison, F. J. Pashkow and S. Tsimikas, Effect of an oral astaxanthin prodrug (CDX-085) on lipoprotein levels and progression of atherosclerosis in LDLR(-/-) and ApoE(-/-) mice, Atherosclerosis, 2012, 222(1), 99–105 CrossRef CAS PubMed.
-
FDA, Natural Astaxanthin - Rich Extract (ARE) - Astaxanthin-rich carotenoid extract [ARE] derived from Paracoccus Carotinifaciens. FDA-2014-S-0023, 2015.
- EFSA FEEDAP Panel, Scientific Opinion on the safety and efficacy of synthetic astaxanthin as feed additive for salmon and trout, other fish, ornamental fish, crustaceans and ornamental birds, EFSA J., 2014, 12(6), 3724 CrossRef.
- J. A. Edwards, P. Bellion, P. Beilstein, R. Rümbeli and J. Schierle, Review of genotoxicity and rat carcinogenicity investigations with astaxanthin, Regul. Toxicol. Pharmacol., 2016, 75, 5–19 CrossRef CAS PubMed.
- E.FS.A N.DA. Panel, Scientific Opinion on the substantiation of health claims related to astaxanthin and maintenance of joints, tendons, and connective tissue (ID 1918, 1978, 3142), protection of DNA, proteins and lipids from oxidative damage (ID 1449, 3141), maintenance of, EFSA J., 2009, 7(9), 1253 Search PubMed.
- J. Gruber, S. Schaffer and B. Halliwell, The mitochondrial free radical theory of ageing–where do we stand?, Front. Biosci., 2008, 13, 6554–6579 CrossRef CAS.
- J. Lapointe and S. Hekimi, When a theory of aging ages badly, Cell. Mol. Life Sci., 2010, 67(1), 1–8 CrossRef CAS PubMed.
- M. Ristow and S. Schmeisser, Extending life span by increasing oxidative stress, Free Radicals Biol. Med., 2011, 51(2), 327–336 CrossRef CAS PubMed.
- A. Sanz, Mitochondrial reactive oxygen species: Do they extend or shorten animal lifespan?, Biochim. Biophys. Acta, 2016, 1857(8), 1116–1126 CrossRef CAS PubMed.
- B. Halliwell, Free radicals and antioxidants: updating a personal view, Nutr. Rev., 2012, 70(5), 257–265 CrossRef PubMed.
- S. I. Liochev, Which Is the Most Significant Cause of Aging?, Antioxidants, 2015, 4(4), 793–810 CrossRef CAS PubMed.
- V. Conti, V. Izzo, G. Corbi, G. Russomanno, V. Manzo and F. De Lise,
et al., Antioxidant Supplementation in the Treatment of Aging-Associated Diseases, Front. Pharmacol., 2016, 7, 24 Search PubMed.
- R. Bruggisser, K. von Daeniken, G. Jundt, W. Schaffner and H. Tullberg-Reinert, Interference of plant extracts, phytoestrogens and antioxidants with the MTT tetrazolium assay, Planta Med., 2002, 68(5), 445–448 CrossRef CAS PubMed.
- H. Y. Jang, S. J. Ji, Y. H. Kim, H. Y. Lee, J. S. Shin and H. T. Cheong,
et al., Antioxidative effects of astaxanthin against nitric oxide-induced oxidative stress on cell viability and gene expression in bovine oviduct epithelial cell and the developmental competence of bovine IVM/IVF embryos, Reprod. Domest. Anim., 2010, 45(6), 967–974 CrossRef CAS PubMed.
- B. Halliwell and M. Whiteman, Measuring reactive species and oxidative damage in vivo and in cell culture: how should you do it and what do the results mean?, Br. J. Pharmacol., 2004, 142(2), 231–255 CrossRef CAS PubMed.
- D. Steinberg, Clinical trials of antioxidants in atherosclerosis: are we doing the right thing?, Lancet, 1995, 346(8966), 36–38 CrossRef CAS.
- R. C. S. Seet, A. M. L. Quek, E. C. H. Lim and B. Halliwell, Biomarkers of oxidative damage are elevated among individuals with high cardiovascular risk: refining subject selection strategies for antioxidant trials, Free Radical. Res., 2013, 47(4), 283–290 CrossRef CAS PubMed.
- B. Halliwell and C. Y. J. Lee, Using isoprostanes as biomarkers of oxidative stress: some rarely considered issues, Antioxid. Redox Signaling, 2010, 13(2), 145–156 CrossRef CAS PubMed.
- F. Visioli, C. A. De La Lastra, C. Andres-Lacueva, M. Aviram, C. Calhau and A. Cassano,
et al., Polyphenols and human health: a prospectus, Crit. Rev. Food Sci. Nutr., 2011, 51(6), 524–546 CrossRef CAS PubMed.
- K. Karimi-Galougahi, C. Antoniades, S. J. Nicholls, K. M. Channon and G. A. Figtree, Redox biomarkers in cardiovascular medicine, Eur. Heart J., 2015, 36(25), 1576–1582 CrossRef PubMed.
|
This journal is © The Royal Society of Chemistry 2017 |