DOI:
10.1039/C5FO00892A
(Paper)
Food Funct., 2016,
7, 483-490
Acutely administered grape-seed proanthocyanidin extract acts as a satiating agent†
Received
21st July 2015
, Accepted 14th October 2015
First published on 30th October 2015
Abstract
Grape-seed proanthocyanidins’ role as stimulators of active GLP-1 in rats suggests that they could be effective as satiating agents. Wistar rats were used to study the effects of proanthocyanidins on food intake with different doses, administration times and proanthocyanidin extract compositions. A dose of 423 mg of phenolics per kg body weight (BW) of grape-seed proanthocyanidin extract (GSPE) was necessary to decrease the 12-hour cumulative food intake by 18.7 ± 3.4%. Proanthocyanidins were effective when delivered directly into the gastrointestinal tract one hour before, or simultaneously at the start of the feeding period. Proanthocyanidins without galloyl forms, such as those from cocoa extract, were not as effective as grape-seed derived forms. GSPE increased the portal levels of active GLP-1 and total ghrelin and decreased the CCK levels, simultaneously with a decrease in gastric emptying. In conclusion, grape-seed proanthocyanidins could be useful as a satiating agent under the conditions defined in this study.
Introduction
As a result of increased food consumption, decreased physical activity and changes in the nutritional value of foods, overweight and obesity are today major problems in developed societies.1 The relationship between overweight and various related diseases, such as high blood pressure, coronary artery disease, stroke, type 2 diabetes mellitus, hyperlipidemia, arthritis/degenerative joint disease, obstructive sleep apnea, infertility, gallstones and depression, among others, is well known. Many strategies have been proposed to solve these problems, and one of these is the administration of functional ingredients.2,3 Functional ingredients could act against excessive body weight gain, by means of several mechanisms, such as modifying the activity of the digestive enzymes,4 hampering nutrient absorption and/or inducing thermogenic effects.3,5 Another strategy for functional ingredients is to reduce food intake by several mechanisms, such as reducing the desire for food, decreasing the feeling of hunger, inducing fullness and early meal termination and maintaining the sensation of fullness between meals.6
Proanthocyanidins are a group of polyphenols widely distributed in nature (in fruits, vegetables and their beverage products such as red wine and tea). They have been shown to have protective effects against several diseases7–10 but their effects on food intake have been scarcely studied.11 Most studies on proanthocyanidins have focused on their effects on body weight, but not specifically on food intake control. Some animal studies mention a role of grape flavanols in impairing weight gain,12,13–16 although food intake was neither measured nor modified in many of them. A short-term study of healthy humans consuming grape-seed proanthocyanidin extract (GSPE) showed a reduced 24-hour energy intake in subjects with an energy requirement ≥ the median of 7.5 MJ per day.17 As regards the mechanisms that may be involved in this reduction of food intake, a recent review shows that some polyphenols appear to have the potential to modulate food intake due to their action on the neuropeptides involved in food intake and satiety, such as NPY, AgRP, POMC and CART, although how the polyphenols affect the function of these peptides in the brain is not fully understood.18 A direct site of action of proanthocyanidins could be the enteroendocrine system, where enteroendocrine cells secrete peptidic hormones that control digestive processes and food intake, such as the orexigenic hormone ghrelin and the anorexigenic hormones cholecystokinin (CCK), glucagon-like-peptide-1 (GLP-1) and peptide YY (PYY)19 that could, in turn, affect the neuropeptide system. Proanthocyanidins were recently shown to modulate GLP-1 levels through their capacity to inhibit the GLP-1-degrading protease dipeptidyl-peptidase 4 (DPP4).20 Proanthocyanidins also increase GLP-1 plasma levels in rats after an oral glucose load,21 although direct GLP-1 stimulatory effects were not found in the STC-1 cell line.22 Finally proanthocyanidins might also act through alteration of the number of enteroendocrine cells in the intestine.23 Similarly, a single administration of berry purée (800 mg polyphenols including proanthocyanidins and other flavonoids) together with sucrose in healthy humans tended to increase GLP-1,24 and the proanthocyanidin cinnamtannin A2 increases GLP-1 in mice.25 Ghrelin, which is produced mainly in the stomach and which has a main role in the regulation of food intake, is modulated by isoflavones, but there is less information regarding other types of flavonoids. There is even less information on the effects of flavonoids on other enterohormones such as PYY or CCK and, indeed, none at all about proanthocyanidins.11
The aim of this study is to analyze whether proanthocyanidins modify food intake and the food intake-controlling enterohormones. Two proanthocyanidin extracts with different compositions from cocoa and grape seeds were compared under standard feeding conditions to test their effects on food intake. GSPE was also tested in two different models of disrupted metabolism very common in human societies under overfeeding situations: a hyperphagic model caused by a tasty diet and an impaired glucose tolerant aged rat model.
Results
Grape-seed proanthocyanidins limit food intake
We hypothesized that the interaction of proanthocyanidins with the enteroendocrine system, previously shown to raise GLP-1 levels,21 could lead to a reduction in food intake. We thus first tested GSPE, at a dose that had previously been shown to be effective at increasing GLP-1 levels, of 846 mg phenolics per kg BW. The GSPE significantly reduced periodic and cumulative food intake 12 hours (h) after feeding initiation in healthy rats (Fig. 1A and B) and in a glucose-resistant model (Fig. 1C and D). A conditional taste aversion (CTA) test and a pica behavior test were performed and no signs of gastrointestinal malaise were found. In the CTA test, the rats developed aversion to a new vanilla flavor when it was paired with a treatment of the nausea-inducing agent LiCl, while the GSPE dose did not produce this effect (Fig. 2A). In the pica test, the rats reduced their daily cumulative intake of chow and increased their intake of non-nutritive kaolin pellets when treated with LiCl (Fig. 2B). The GSPE treatment led to a similar reduction in food intake, but it was not accompanied by an increase in kaolin consumption (Fig. 2C).
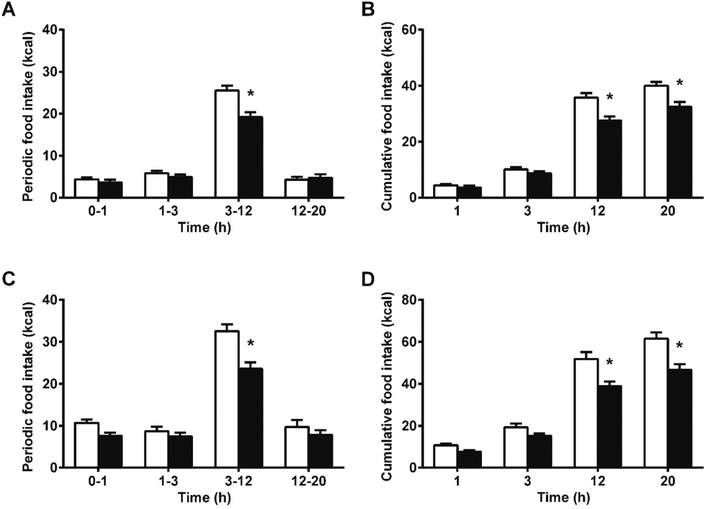 |
| Fig. 1 GSPE effects on food intake in normal and impaired glucose-tolerant rats. Periodic (A) and cumulative (B) food intake in healthy female Wistar rats and in glucose-resistant male rats (C, D). Daily chow intake was measured at each time point after an intragastric dose of GSPE (846 mg per kg BW). Light bars correspond to control (vehicle-administered) animals and dark bars to GSPE-treated animals. A mixed-model two-way ANOVA showed significant time, treatment and interaction terms in both cases (p < 0.05). Asterisks represent significant differences in food intake of treated vs. control group at each time point, assessed by Bonferroni post-hoc tests (p < 0.0001). | |
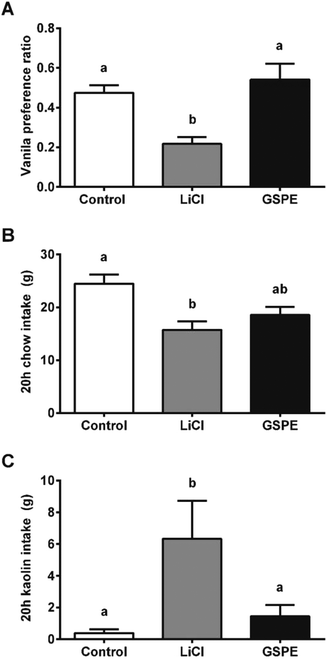 |
| Fig. 2 GSPE effects on the gastrointestinal comfort. Conditional taste aversion test (A) and pica behavior test (B, C) after an intragastric dose of GSPE (846 mg per kg BW). LiCl was used to induce gastrointestinal distress. The different letters represent significant differences between groups assessed by Bonferroni post-hoc tests after a significant one-way ANOVA (p < 0.05). | |
To disrupt food intake control, we stimulated the animals’ energy consumption with a palatable food offer. As shown in Fig. 3A, increasing amounts of a palatable hypercaloric solution proportionally increased energy intake. Fig. 3B shows that under these conditions, food intake was significantly reduced since the beginning of the feeding period. Despite the significant effects on food intake appeared earlier in the hyperphagic model, the % inhibition in food intake in the normophagic model was constant from 1 hour to 20 h. A 2-way ANOVA of experiments with different degrees of hyperphagia discarded any effect of time in the reduction of food intake, but showed an enhancement in the GSPE action proportional to the degree of hyperphagia (Table S1†).
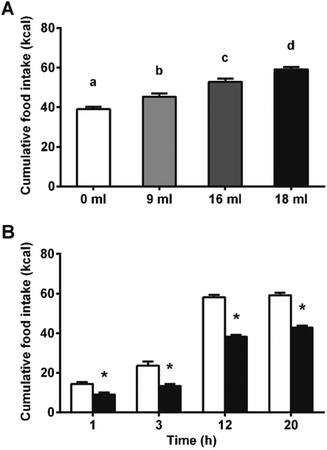 |
| Fig. 3 GSPE effects on food intake under hyperphagic conditions. (A) Effects of the diet supplementation with a palatable hypercaloric solution (HS) on the daily energetic intake. Increased amounts of offered HS proportionally increased the daily energy intake. The different letters represent significant differences between groups assessed by Bonferroni post-hoc test after a significant one-way ANOVA (p < 0.05). (B) GSPE effects on the cumulative food intake in a hyperphagic paradigm. An intragastric dose of GSPE (846 mg per kg BW) was gavaged and food intake was measured at each time point after a diet supplementation with 16 ml HS. Asterisks represents significant differences in food intake of treated (dark bars) vs. control group (light bars) at each time point, assessed by Bonferroni post-hoc tests (p < 0.01) after significant time, treatment and interaction terms in a two-way ANOVA (p < 0.05). | |
Conditions required for a satiating effect of proanthocyanidins
To better define the satiating effects of the GSPE, we compared different dosages, administration times and animal models. The results are shown in Table 1. In first place, we found that the effects of GSPE on food intake were not affected by gender or age, since a GSPE dose of 846 mg phenolics per kg BW produced similar satiating effects in female and aged male Wistar rats. Afterwards, the importance of the time of administration was studied. We found that the satiating effects of 846 mg phenolics per kg BW were similar when the extract is administered 1 h before food and when the extract is administered at the same time as food. Conversely, there was no effect when the GSPE was administered 1 h after the meal initiation.
Table 1 Satiating effects of proanthocyanidins under different conditions
Sex |
BW (g) |
Diet |
Time of dose |
Extract |
Dose |
12 h inhibition (%) |
Satiating effects of proanthocyanidins observed with different doses, diets, time of administration, animal models, and types of extracts. Time of dose indicates the hour of the extract treatment referred to the food replacement (0 h). Dose is expressed as mg phenolics per kg BW. Food intake data are expressed as 12 h cumulative food intake inhibition (%) of treated vs. control group. Asterisks represent significant differences assessed by an unpaired t-test (* p < 0.05; ** p < 0.01; *** p < 0.001). |
Effects of different GSPE moments of administration in both genders
|
♀ |
207 |
Chow |
−1 h |
GSPE |
846 |
22.2 ± 3.8** |
♂ |
542 |
Chow |
−1 h |
GSPE |
846 |
25.0 ± 4.5** |
♀ |
204 |
Chow |
0 h |
GSPE |
846 |
27.7 ± 9.0* |
♂ |
543 |
Chow |
1 h |
GSPE |
846 |
16.2 ± 7.1 |
Effects of lower GSPE doses under standard and hyperphagic conditions
|
♀ |
223 |
Chow |
−1 h |
GSPE |
423 |
18.7 ± 3.4*** |
♀ |
217 |
Chow + 11 ml HS |
−1 h |
GSPE |
423 |
15.9 ± 3.1* |
♀ |
219 |
Chow + 11 ml HS |
−1 h |
GSPE |
84.6 |
1.4 ± 5.0 |
|
Effects of CCE at doses equivalent to GSPE
|
♀ |
196 |
Chow |
−1 h |
CCE |
423 |
−3.86 ± 4.4 |
♀ |
196 |
Chow |
−1 h |
CCE |
846 |
−3.56 ± 2.0 |
♂ |
542 |
Chow |
−1 h |
CCE |
846 |
1.8 ± 4.8 |
Regarding the dosage, we found that 423 mg phenolics per kg BW significantly reduced food intake in a standard feeding model and a hyperphagic model. A lower dose of 84.6 mg phenolics per kg BW presented no effect in food intake.
Finally, the importance of the extract composition was studied by treating the rats with a different proanthocyanidin-rich extract, derived from cocoa (CCX), at doses with a total phenolic content and antioxidant capacity equivalent to the assayed GSPE doses (423 or 846 mg phenolics per kg BW). The treatment with CCX did not inhibit food intake.
GSPE causes changes in portal enterohormones and delays gastric emptying
Our next goal was to analyze the hormonal changes induced by 846 mg phenolics per kg BW of GSPE. Twenty minutes (min) after GSPE gavaging, there were no changes in active GLP-1 (3.22 ± 0.37 vs. 4.01 ± 0.36 pmol L−1) or in insulin (2.68 ± 0.53 vs. 2.57 ± 0.3 μg L−1). Table 2 shows the lack of changes in insulin, CCK or total ghrelin levels sixty min after the GSPE administration. At this point (t = 60 min), we mimicked food intake by an intragastric meal. Twenty min after the intragastric meal (t = 80 min), the GSPE group showed increased active GLP-1 levels (3.84 ± 0.35 vs. 8.64 ± 1.00 pmol L−1, p < 0.05), which led to an increase in insulinemia observed at the same time point (1.76 ± 0.31 vs. 3.29 ± 0.72 μg L−1, p < 0.05). Sixty min after the intragastric meal (t = 120 min), the treatment also increased total ghrelin (4.78 ± 0.46 vs. 6.63 ± 0.77 μg L−1, p < 0.05) and prevented the postprandial rise in CCK observed in the control group (2.00 ± 0.45 vs. 0.76 ± 0.05 μg L−1, p < 0.05). All these changes were not due to the modulation of mRNA expression (Table 3).
Table 2 Portal hormone levels 60 min after the GSPE gavage
Hormone (μg L−1) |
Control |
GSPE |
No significant differences, assessed by t-test (p < 0.05) were found between control and GSPE. |
Insulin |
0.98 ± 0.33 |
0.80 ± 0.18 |
Ghrelin |
3.87 ± 0.31 |
3.45 ± 0.17 |
CCK |
0.67 ± 0.06 |
0.75 ± 0.06 |
Table 3 Gene expression of the enterohormones measured in plasma (relative units)
|
Control |
GSPE |
Measured in corpus.
Measured in duodenum.
Measured in colon. No significant differences, assessed by t-test (p < 0.05) were found between control and GSPE. |
Ghrelina |
1.19 ± 0.31 |
1.35 ± 0.18 |
CCKb |
1.00 ± 0.01 |
1.04 ± 0.15 |
Proglucagonc |
1.12 ± 0.22 |
1.07 ± 0.21 |
Concomitantly, we found that 1 h after the intragastric meal, the GSPE group retained a larger amount of food in the stomach than the control group (Fig. 4).
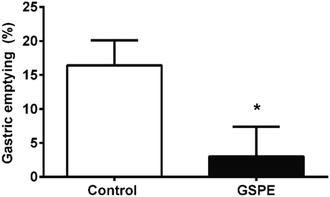 |
| Fig. 4 GSPE effects on gastric emptying. Percentage of food emptied from the stomach 1 h after the gavaging of 1.5 g mashed chow in control rats (light bars) and in rats treated with 846 mg per kg BW GSPE (dark bars). The results are expressed as a % of dry matter relative to a paired oven-dried mashed chow. The asterisk represents significant differences assessed by an unpaired t-test (p < 0.05). | |
Discussion
As recently reviewed,11 few studies have evaluated the effect of flavonoids on food intake, and most of them evaluated it as a secondary issue in studies designed to analyze the effects on body weight and energy balance. In the present study we describe the clear effects of a grape-seed extract on inhibiting food intake, and we also define the effective dose and administration mode.
Our results show that GSPE is effective at reducing food intake when administered under specific conditions that we will define. We found that the minimum amount of proanthocyanidins to be effective is higher than 86.2 mg phenolics per kg BW. From the doses that we tested, the minimal effective dose was 423 mg phenolics per kg BW. Other studies, focusing mainly on the effects on body weight, report no effects of grape-seed extracts on food intake. This discrepancy might be due to the fact that most of these studies used lower doses.26,27 Another reason could be the different methods of administration (proanthocyanidins alone or with other food). In most of the studies where no effect on food intake was observed, the extract had been administered with different vehicles, many of which included other nutrients such as standard chow,16,28 high-fat chow and condensed milk.15 Our data demonstrate that the satiating effect of proanthocyanidin extract was found when it was administered one h before or just before the beginning of the feeding period, while there was no effect on food intake if the extract was administered 1 h after food initiation. This highlights the need for an almost empty gastrointestinal tract when the GSPE is administered for it to be effective. Some authors have proved that the phenolic compounds interact with food components, i.e. proteins.29 Furthermore, some studies showing an inhibitory effect on food intake attribute it to the discomfort caused in the oral cavity because of its tannic effect (astringency).30 Proanthocyanidins were mixed with drinking water or food in these studies.27 In this paper, we avoided this problem by administering the extract intragastrically. The proanthocyanidins thereby reach the stomach and duodenal mucosa directly. We also discarded any alteration in both tissues using histological analysis (results not shown), as well as we made sure that the treatment does not cause gastrointestinal malaise by pica and conditional taste aversion tests.
Another important point is related to the quality of food intake measurements. Measures taken using inadequate methodology could produce controversial results, which create doubts regarding the effectiveness of food intake treatments.31 This could also bias the food intake results obtained in the previous studies focused on the effects of proanthocyanidins on body weight and metabolic parameters. For this reason, in this study animals and people were specifically trained in order to obtain reliable data, as explained in the Experimental section.
The composition of the extract must also be considered. Our results support the theory that the effectiveness of a grape-seed derived extract is higher than a cocoa derived extract. The GSPE extract used here contains monomeric (epi)-catechins, their gallate forms, dimers and trimers of all these monomeric forms32 and some higher structures.33 We do not rule out the presence of some prodelphinidins, as defined by Gu et al.34 We have confirmed that some concordant grape-seed proanthocyanidin extracts also produce an inhibition of food intake (results not shown). Conversely, cocoa extract, which contains proanthocyanidins without galloyl forms,35 did not cause a clear satiating effect. This suggests that the bioactivity of GSPE in inhibiting food intake might be attributed to the galloyl forms of proanthocyanidins. In this regard, different flavonol structures have different abilities to stimulate the taste receptors located at the enteroendocrine cells, with their galloyl forms being most effective at binding to these taste receptors.36 The precise link between the differential stimulation of taste receptors by different flavonoid structures and the enteroendocrine signals generated from the gastrointestinal tract in response has not yet been determined. We have previously proved that GLP-1 levels increase after the administration of GSPE at the same doses as those used in this study.21 Moreover, a revision of this topic11 shows that flavonoids modulate GLP-1 levels and ghrelin, but there is very little information on their effects on other enterohormones. Importantly, whether such modulation involves effects on food intake was not assessed in any of these studies.
A common mechanism of the anorexigenic action of GLP-1 and CCK consists of the reduction of the gastrointestinal transit, contributing to both meal termination and feeling of fullness.37 Our results show that GSPE leads to a reduction in gastric emptying in unconscious rats, concomitantly to an increase in active GLP-1. This inhibition of gastric emptying could help explain the inhibition of food intake. A different effect is found for CCK, which only increased after the meal in the control group. Although we are unable to formally rule out an inhibition of CCK release, baseline CCK levels are maintained in the GSPE group, suggesting that CCK levels may not rise in the treated group due to the slower gastric emptying to the duodenum.
Meanwhile, ghrelin, in its acylated form, is the only enterohormone with orexigenic properties.38 Unexpectedly, we found an increase in total ghrelin after the GSPE treatment. Despite this increase, the food intake data clearly show that the reduction in food intake is sustained over time after the treatment. A possible explanation could be related to the results of Toshinai et al. which show that the orexigenic action of ghrelin is abolished with a prior rise of GLP-1 acting on vagal afferents.39 Our data suggest that this ghrelin desensitization could also occur in the group treated with GSPE, since the stimulation of the GLP-1 release occurred prior to the increase in total ghrelin.
Both effects on portal enterohormone levels occur after the intragastric meal. Although our study was not designed to evaluate the effects of nutrients on the GSPE action, this result suggests that other molecules must be present in the gastrointestinal tract, after GSPE administration, to produce changes in the release of enterohormones. We have confirmed earlier findings that GSPE influences the secretion of active GLP-1 after a nutrient load21 and extended them to changes in the secretion of CCK and ghrelin.
Finally, we have proved the effectiveness of GSPE administration in healthy animals with an adjusted food intake control, and an even greater effectiveness with a disrupted food intake control, as occurs with a highly palatable diet.40 This greater effectiveness with the high-sucrose palatable diet could point to a role of GLP-1 through an increased glucose-stimulated GLP-1 release. We have also proved the extract's bioactivity in a model of impaired glucose tolerance (aged rats), highlighting glucose-intolerant subjects as a potential target. Both results point to the utility of this treatment in individuals with difficulties controlling food intake who usually have concomitant defective glucose tolerance.
Experimental
Proanthocyanidin extracts
The grape-seed extract enriched in proanthocyanidins (GSPE) was obtained from Les Dérivés Résiniques et Terpéniques (Dax, France). According to the manufacturer, the extract contains monomeric (21.3%), dimeric (17.4%), trimeric (16.3%), tetrameric (13.3%) and oligomeric (5–13 units; 31.7%) proanthocyanidins. The small molecules (up to trimers) present in GSPE were characterized in more detail by reverse-phase chromatographic analyses by our research group.32 Cocoa derived extract (CCX) was kindly provided by Dr Julian Castillo of Nutrafur, S.A. (Murcia, Spain). According to the manufacturers, the extract contains epicatechin (6.84%), catechin (0.86%), B2 (4.15%) and B1 (0.73%). It was possible to obtain a complementary quantification from Quinones et al.35 The phenolic content of both extracts was quantified with the Folin method41 as 845.5 ± 10.5 mg g−1 GSPE and 452 ± 9.94 mg g−1 CCE.
Animals
Two sets of female Wistar rats (Harlan, Barcelona, Spain), weighing 180–200 g upon arrival, were used for the conditional taste aversion test (n = 21) and for the food intake studies and blood sampling (n = 21). Two sets of adult male Wistar rats (Harlan, Barcelona), weighing 450–500 g, were used for the pica test (n = 18) and the food intake studies (n = 15), as a model for glucose-impaired tolerance.42 The studies in male groups took place at the facilities of the Technological Center of Nutrition and Health (http://www.ctns.cat). All of the procedures were approved by the Experimental Animal Ethics Committee of the Universitat Rovira i Virgili.
On arrival, the subjects were single housed at 22 °C under a 12 h light/dark cycle (lights on at 8 am) with access to standard chow pellets (Teklad Global Diets #2014, Harlan, Barcelona) and tap water ad libitum during a 1 week adaptation period. Following this adaptation period, the animal sets for the food intake studies were introduced to a daily 4 h food deprivation before the light offset (from 16:00 h until 20:00 h), and blank chow intake measurements were taken on 4 days prior to each experiment, to habituate the subjects to the experimental schedule. One experiment per week was performed in a cross-over design for all the food intake studies. The animal sets for the gastrointestinal malaise tests underwent special adaptation conditions, as described below.
Food intake experiments
Standard diet.
To assess the effects of an acute dose of proanthocyanidins on food intake, trained animals were treated with GSPE or CCX doses from 423 to 846 mg phenolics per kg of BW intragastrically (i.g.) 1 h before the dark onset (unless otherwise stated), using tap water as a vehicle, and the chow intake was measured 1, 3, 12 and 20 h after the chow replacement with an accuracy of 0.01 g. Parallel controls were performed by administering the vehicle intragastrically.
Palatable hypercaloric meal.
The effect of proanthocyanidins under a hypercaloric meal was tested only in female rats, due to their sensitivity to diet challenges43 using a palatable high-sucrose emulsion (HS) presented in an independent bottle, containing (by weight) 10% powdered skimmed milk, 40% sucrose, 4% lard and 0.35% xanthan gum as a stabilizer (Sigma-Aldrich, St Louis, MO), with a caloric density of 2.10 kcal g−1.44 A GSPE dose of 846 mg phenolics per kg of BW (unless otherwise stated) or the vehicle was administered i.g. 1 h before the dark onset, and chow and HS intake were measured 1, 3, 12 and 20 h after the meal presentation. The effect on different hypercaloric situations was assayed by administering different HS volumes (0, 9, 16, 18 ml) in separate experiments.
Gastrointestinal malaise tests
Conditional taste aversion (CTA).
Seven days prior to the conditioning day, animals were introduced to a water-restriction schedule, so they were accustomed to drinking the whole daily fluid intake in single 30 min sessions (starting at 12:00).45 In order to habituate rats to a 2-bottle choice, water was presented in two sipper bottles simultaneously. On the conditioning day, the rats were given access to two bottles of 0.5% vanilla flavored solution (Dr Oetker, Barcelona) and after the 30 min drinking period, the bottles were removed and the subjects were administered i.g. water, i.g. GSPE (846 mg phenolics per kg of BW) or intraperitoneally (i.p.) with the gastrointestinal distress-inducing agent LiCl (0.15 M, 2% body weight). On the first day after conditioning, the rats had 30 min access to water, and on the subsequent day, a two bottle preference test between water and 0.5% vanilla was conducted. The position of the two bottles was counter-balanced between the subjects and fluid consumption was measured with an accuracy of 0.01 g after the 30 min session. The results were expressed as a preference ratio of the drug-paired flavor by dividing the intake of flavored solution to total fluid intake.
Pica behavior test.
Human grade kaolin powder (A. Vogel, Barcelona) was mixed with 1% arabic gum (Sigma-Aldrich, St Louis) and tap water, squeezed through a pastry decorator and allowed to dry at 35 °C.46 The resulting kaolin pellets, similar in shape and size to chow pellets, were introduced to subjects seven days prior to the pica test, placed with chow in the food hoppers. On the test day, the animals were administered i.g. water, i.g. GSPE (846 mg phenolics per kg of BW) or i.p. the gastrointestinal distress-inducing agent LiCl (0.15 M, 2% body weight) just before the dark onset, and the chow and kaolin intake were measured 20 h later.
Portal vein catheterization and intragastric feeding
The female rats from the food intake set were randomly divided into two groups: a control group (n = 10) treated with tap water, and a proanthocyanidin treated group (n = 11) gavaged with GSPE at 846 mg phenolics per kg BW. The rats were fasted from 15.00 h until 18.00 h before the treatment, and were anesthetized 5 min later with 70 mg per kg BW i.p. of sodium pentobarbital. The abdominal cavity was incised through the midline, and the portal vein was catheterized with a PE tube (I.D. 0.58 mm, O.D. 0.965 mm; Becton Dickinson, Sparks, MD, USA) using a standard procedure. The catheter was fixed with methacrylate and the abdominal cavity was closed with surgical clamps. The body temperature was monitored by an abdominal probe and kept constant at 37.5 ± 0.5 °C by a heated surgical table and overhead lamps. At 60 min after the treatment, 5 ml of mash, containing 1.5 g of standard chow and 25 mg of xanthan gum as a stabilizer, was punctured in the forestomach with an Abbocath-T 18G catheter (Hospira Inc., IL, USA) at a constant rate of 1 ml min−1.
Tissue sampling
Portal blood samples (400 μl) were taken 20, 40, 60, 80 and 120 min after the treatment, and the catheter was refilled with heparinized 0.9% NaCl (600 IU ml−1). The blood was transferred to heparinized tubes and a 1
:
100 volume of a 1
:
1 mix of protease inhibitors was spiked immediately (Pefabloc, Sigma-Aldrich, MO; DPP-IV inhibitor, Millipore, MI). Plasma was collected by centrifugation at 1500g over 15 min at 4 °C and frozen immediately at −80 °C. After the 120 min procedure, the rats were sacrificed by exsanguination of the aortal vein; tissue samples were immediately frozen in liquid nitrogen, and then stored at −80 °C. The stomachs were excised and the stomach content of each individual was collected and dried overnight at 50 °C.
Plasma and tissue quantification
The enterohormones were assayed in duplicate using commercial ELISA kits for insulin (Mercodia, Uppsala, Sweden), GLP-1 3-37 amide (Millipore, Billerica, MA, USA), total CCK (Peninsula Laboratories, San Carlos, CA, USA) and total ghrelin (Phoenix Pharmaceuticals, Burlingame, CA, USA).
Total RNA was extracted using Trizol (Ambion, USA) and trichloromethane–ethanol (Panreac, Barcelona, Spain), and purified using a RNA extraction kit (Qiagen, Hilden, Germany). Complementary DNA was obtained using the High Capacity cDNA Reverse Transcription Kit (Applied Biosystems, Madrid, Spain) and the quantitative reverse transcriptase-polymerase chain reaction (qRT-PCR) amplification was performed using TaqMan Universal PCR Master Mix and the respective specific TaqMan probes (Applied Biosystems, Madrid, Spain). The relative expression of each mRNA was calculated against the control group using the 2−ΔΔCt method, with cyclophilin as the reference gene.
Statistical analysis
The data are represented as mean ± SEM. Periodic and cumulative food intake was analyzed using a mixed-model two-way ANOVA, with time as a within factor and treatment as a between factor. The portal hormone concentrations were analyzed using a regular two-way ANOVA. Conditional taste aversion, pica behavior, 12-hour cumulative food intake inhibition, gastric emptying rate and gene expression results were analyzed using a one-way ANOVA or Student's t-test, as appropriate. Bonferroni post-hoc tests were performed to assess the differences between groups after significant ANOVA. The statistical analyses were performed with IBM SPSS Statistics 22. P-values < 0.05 were considered significant in all cases.
Conclusions
A grape-seed proanthocyanidin extract reduces food intake in rats when it is intragastrically administered at a dose of 423 mg phenolics per kg BW or higher, preferably before meal initiation. This effect could be partially explained by its interaction with the enteroendocrine system and its effects on reducing gastric emptying. Further work is needed to clearly define the possible role of the enteroendocrine system in this satiating effect. This treatment could be recommended for individuals with difficulties in food intake control, who usually have concomitant defective glucose tolerance.
Conflict of interest
The authors declare that there are no conflicts of interest.
Acknowledgements
This work has been supported by a grant (AGL2014-55347-R) from the Spanish government. Àngela Casanova-Martí and Katherine Gil-Cardoso received a grant for Ph.D. students from Universitat Rovira i Virgili. Joan Serrano received a pre-doctoral fellowship from the Government of Catalonia. Montserrat Pinent is a Serra Húnter fellow. The authors declare that they have no conflict of interest. We would like to express our thanks to Niurka Llopiz and Yaiza Tobajas for their technical support. Cocoanox (Cocoa derived extract) was kindly provided by Nutrafur S.A. (http://www.nutrafur.com).
References
- C. Hawkes, Global Health, 2006, 2, 4 CrossRef PubMed.
- L. Trigueros, S. Peña, A. V. Ugidos, E. Sayas-Barberá, J. A. Pérez-Álvarez and E. Sendra, Crit. Rev. Food Sci. Nutr., 2013, 53, 929–942 CrossRef CAS PubMed.
- N. D. Yuliana, H. Korthout, C. H. Wijaya, H. K. Kim and R. Verpoorte, Crit. Rev. Food Sci. Nutr., 2012, 54, 373–388 CrossRef PubMed.
- R. B. Birari and K. K. Bhutani, Drug Discovery Today, 2007, 12, 879–889 CrossRef CAS PubMed.
- A. G. Dulloo, Obes. Rev., 2011, 12, 866–883 CrossRef CAS PubMed.
- J. C. G. Halford and J. A. Harrold, Proc. Nutr. Soc., 2012, 71, 350–362 CrossRef PubMed.
- C. Bladé, L. Arola and M.-J. Salvadó, Mol. Nutr. Food Res., 2010, 54, 37–59 Search PubMed.
- M. Pinent, L. Cedó, G. Montagut, M. Blay and A. Ardévol, Crit. Rev. Food Sci. Nutr., 2012, 52, 569–584 CrossRef CAS PubMed.
- M. Pinent, M. Bladé, M. Salvadó, M. Blay, G. Pujadas, J. Fernàndez-Larrea, L. Arola and A. Ardévol, Crit. Rev. Food Sci. Nutr., 2006, 46, 543–550 CrossRef CAS PubMed.
- N. Martinez-Micaelo, N. González-Abuín, A. Ardèvol, M. Pinent and M. Blay, BioFactors, 2012, 38, 257–265 CrossRef CAS PubMed.
- M. Pinent, M. Blay, J. Serrano and A. Ardévol, Crit. Rev. Food Sci. Nutr., 2015 Search PubMed , in press.
- M. Vadillo, A. Ardévol, J. Fernández-Larrea, G. Pujadas, C. Bladé, M. J. Salvadó, L. Arola and M. Blay, J. Nutr. Biochem., 2006, 17, 139–142 CrossRef PubMed.
- K. Tebib, P. Besançon and J. M. Rouanet, Nutr. Res., 1996, 16, 105–110 CrossRef CAS.
- K. Ohyama, C. Furuta, Y. Nogusa, K. Nomura, T. Miwa and K. Suzuki, Ann. Nutr. Metab., 2011, 58, 250–258 CrossRef CAS PubMed.
- X. Terra, V. Pallarés, A. Ardèvol, C. Bladé, J. Fernández-Larrea, G. Pujadas, J. Salvadó, L. Arola and M. Blay, J. Nutr. Biochem., 2011, 22, 380–387 CrossRef CAS PubMed.
- A. Caimari, J. M. del Bas, A. Crescenti and L. Arola, Int. J. Obes., 2012, 37, 576–583 CrossRef PubMed.
- N. Vogels, I. M. T. Nijs and M. S. Westerterp-Plantenga, Eur. J. Clin. Nutr., 2004, 58, 667–673 CrossRef CAS PubMed.
- K. S. Panickar, Mol. Nutr. Food Res., 2012, 1–14 Search PubMed.
- F. M. Gribble, Proc. Nutr. Soc., 2012, 71, 456–462 CrossRef CAS PubMed.
- N. González-Abuín, N. Martínez-Micaelo, M. Blay, G. Pujadas, S. Garcia-Vallvé, M. Pinent and A. Ardévol, J. Agric. Food Chem., 2012, 60, 9055–9061 CrossRef PubMed.
- N. González-Abuín, N. Martínez-Micaelo, M. Margalef, M. Blay, A. Arola-Arnal, B. Muguerza, A. Ardévol and M. Pinent, Food Funct., 2014, 5, 2357–2364 Search PubMed.
- N. González-Abuín, N. Martínez-Micaelo, M. Blay, B. D. Green, M. Pinent and A. Ardévol, Am. J. Physiol.: Cell Physiol., 2014, 306, C485–C492 CrossRef PubMed.
- N. González-Abuín, N. Martínez-Micaelo, M. Blay, A. Ardévol, M. Pinent, N. Gonzalez-Abuin, N. González-Abuín, N. Martínez-Micaelo, M. Blay, A. Ardévol and M. Pinent, J. Agric. Food Chem., 2014, 62, 1066–1072 CrossRef PubMed.
- R. Törrönen, E. Sarkkinen, T. Niskanen, N. Tapola, K. Kilpi and L. Niskanen, Br. J. Nutr., 2011, 107, 1445–1451 CrossRef PubMed.
- Y. Yamashita, M. Okabe, M. Natsume and H. Ashida, Biosci. Biotechnol. Biochem., 2013, 77(4), 888–891 CrossRef CAS PubMed.
- H. J. A. Hollis, J. H. Blumberg JB, R. D. Mattes, J. H. Hollis, J. A. Houchins, J. B. Blumberg and R. D. Mattes, J. Am. Coll. Nutr., 2009, 28, 574–582 CrossRef PubMed.
- I. K. Hwang, D. W. Kim, J. H. Park, S. S. Lim, K.-Y. Yoo, D. Y. Kwon, D.-W. Kim, W.-K. Moon and M.-H. Won, Phytother. Res., 2009, 23, 1182–1185 CrossRef PubMed.
- S. Bentivegna and K. Whitney, Food Chem. Toxicol., 2002, 40, 1731–1743 CrossRef CAS PubMed.
- S. V. E. Prigent, A. G. J. Voragen, G. A. van Koningsveld, A. Baron, C. M. G. C. Renard and H. Gruppen, J. Dairy Sci., 2015, 92, 5843–5853 CrossRef PubMed.
- Z. Glick, J. Nutr., 1981, 111, 1910–1916 CAS.
- S. C. Woods and W. Langhans, Am. J. Physiol.: Endocrinol. Metab., 2012, 303, E1408–E1418 CrossRef CAS PubMed.
- M. Quiñones, L. Guerrero, M. Suarez, Z. Pons, A. Aleixandre, L. Arola and B. Muguerza, Food Res. Int., 2013, 51, 587–595 CrossRef.
- G. Montagut, I. Baiges, J. Valls, X. Terra, J. M. del Bas, X. Vitrac, T. Richard, J.-M. Mérillon, L. Arola, M. Blay, C. Bladé, J. Fernández-Larrea, G. Pujadas, J. Salvadó and A. Ardévol, Food Chem., 2009, 116, 265–270 CrossRef CAS.
- L. Gu, M. Kelm, J. Hammerstone, G. Beecher, J. Holden, D. Haytowitz, S. Gebhardt and R. Prior, J. Nutr., 2004, 134, 613–617 CAS.
- M. Quinones, M. Miguel, B. Muguerza and A. Aleixandre, Food Funct., 2011, 2, 649–653 CAS.
- S. Soares, S. Kohl, S. Thalmann, N. Mateus, W. Meyerhof and V. De Freitas, J. Agric. Food Chem., 2013, 61, 1525–1533 CrossRef CAS PubMed.
- O. Chaudhri, C. Small and S. Bloom, Philos. Trans. R. Soc. London, Ser. B, 2006, 361, 1187–1209 CrossRef CAS PubMed.
- M. D. Gahete, D. Rincón-Fernández, A. Villa-Osaba, D. Hormaechea-Agulla, A. Ibáñez-Costa, A. J. Martínez-Fuentes, F. Gracia-Navarro, J. P. Castaño and R. M. Luque, J. Endocrinol., 2014, 220, R1–R24 CrossRef CAS PubMed.
-
K. Toshinai, in The Conference on Bioactive Peptides for Cell–Cell Communication 2014 - The 30th Anniversary of ANP (Atrial Natriuretic Peptide) Discovery -, 2014.
- A. Sclafani and C. N. Berner, Physiol. Behav., 1976, 16, 355–363 CrossRef CAS PubMed.
- N. Ortega, M.-P. Romero, A. Macià, J. Reguant, N. Anglès, J.-R. Morelló and M.-J. Motilva, J. Agric. Food Chem., 2008, 56, 9621–9627 CrossRef CAS PubMed.
- C. Selman and D. J. Withers, Philos. Trans. R. Soc. London, Ser. B, 2010, 366, 99–107 CrossRef PubMed.
- J. Ribot, A. M. Rodriguez, E. Rodriguez and A. Palou, Obesity, 2008, 16, 723–730 CrossRef CAS PubMed.
- J. Sánchez, T. Priego, M. Palou, A. Tobaruela, A. Palou and C. Picó, Endocrinology, 2008, 149, 733–740 CrossRef PubMed.
- E. M. Fekete, K. Inoue, Y. Zhao, J. E. Rivier, W. W. Vale, A. Szucs, G. F. Koob and E. P. Zorrilla, Neuropsychopharmacology, 2006, 32, 1052–1068 CrossRef PubMed.
- M. G. Hotchkiss, D. S. Best, R. L. Cooper and S. C. Laws, Neurotoxicol. Teratol., 2012, 34, 295–302 CrossRef CAS PubMed.
Footnotes |
† Electronic supplementary information (ESI) available. See DOI: 10.1039/C5FO00892A |
‡ These authors contributed equally to this work. |
|
This journal is © The Royal Society of Chemistry 2016 |
Click here to see how this site uses Cookies. View our privacy policy here.