DOI:
10.1039/C6DT01288D
(Paper)
Dalton Trans., 2016,
45, 8422-8427
Surface functionalization of dinuclear clathrochelates via Pd-catalyzed cross-coupling reactions: facile synthesis of polypyridyl metalloligands†
Received
4th April 2016
, Accepted 16th April 2016
First published on 25th April 2016
Abstract
Dinuclear clathrochelate complexes are easily accessible by reaction of zinc(II) triflate or cobalt(II) nitrate with arylboronic acids and phenoldioximes. The utilization of brominated arylboronic acids and/or brominated phenoldioximes allows preparing clathrochelates with two, three, five or seven bromine atoms on the outside. These clathrochelates can undergo Pd-catalyzed cross-coupling reactions with 3- and 4-pyridylboronic acid to give new metalloligands featuring up to seven pyridyl groups. The pyridyl-capped clathrochelates display characteristics which make them interesting building blocks for structural supramolecular chemistry: they are rigid, large (up to 2.7 nm), luminescent (for M = Zn), and anionic. The pentatopic pyridyl ligands display an unusual trigonal bipyramidal geometry.
Introduction
A metalloligand is a metal complex appended with functional groups capable of binding to other metal ions. Metalloligands have been used extensively in structural supramolecular chemistry.1–3 Compared to standard organic ligands, metalloligands have potential advantages. The internal metal ions can add novel functions such as redox-activity, color, magnetism, molecular recognition sites or catalytic activity. In addition, the metal ions can simplify the synthesis of the ligand if metal-templated reactions are employed. Metalloligands featuring two or more pyridyl groups have received particular interest. For example, metalloporphyrin ligands with terminal pyridyl groups have been used extensively for the construction of molecular and polymeric nanostructures.2 Pyridyl-functionalized salen-type complexes have also found widespread applications, notably because the salen complexes can be used for catalytic reactions.3
We have recently described the synthesis of metalloligands which feature two pyridyl groups attached to a dinuclear clathrochelate core containing either Zn2+ or Co2+ ions (Fig. 1).4 A special feature of these metalloligands is their negative charge, which can impart an increased stability to metallasupramolecular assemblies. Furthermore, they are very robust compounds, tolerating the rather harsh reaction conditions which are needed for the synthesis of metal–organic frameworks (MOFs). Capitalizing on the inert character of the clathrochelate, we now demonstrate that it is possible to post-functionalize these complexes via Pd-catalyzed cross-coupling reactions. This synthetic strategy enabled us to prepare extended metalloligands containing two, three, five or seven pyridyl groups.
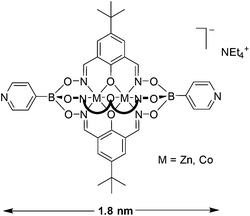 |
| Fig. 1 Dinuclear clathrochelates with terminal 4-pyridyl groups. | |
Results and discussion
Ditopic metalloligands
The dipyridyl ligands depicted in Fig. 1 were obtained by reaction of a Zn- or Co-salt with 4-pyridylboronic acid and a phenoldioxime.4 The synthesis of clathrochelates with other functional groups (e.g. carboxylic acids) in the periphery can be achieved by using different boronic acids and/or phenoldioximes.5 For mononuclear clathrochelates based on glyoximato ligands and Fe(II) centers, it had been reported that metal-catalyzed reactions can be used for the post-functionalization of the clathrochelate skeleton.6,7 We were wondering if a similar strategy could be employed for the post-modification of dinuclear clathrochelates. To investigate this question, we have prepared the Zn(II) complex 1a and the Co(II) complex 1b having two bromine atoms in apical position following a standard protocol (Scheme 1).
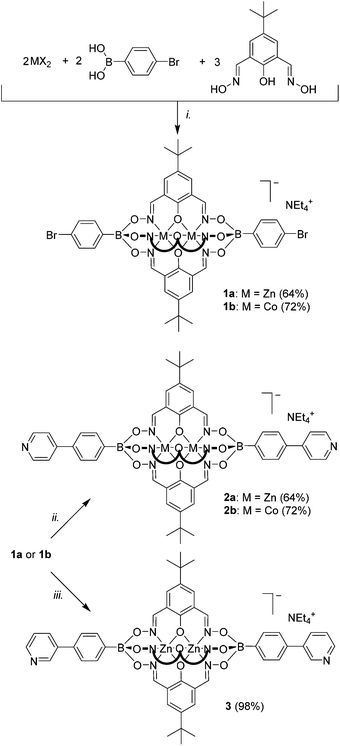 |
| Scheme 1 Synthesis of the clathrochelates 1–3. Reagents and conditions: (i): 4-bromophenylboronic acid, Zn(OTf)2 or [Co(H2O)6](NO3)2, MeOH, 70 °C, then NEt4OH; (ii): 1a or 1b (1 eq.), 4-pyridylboronic acid (6 eq.), K3PO4 (3 eq.), Pd2(dba)3 (5 mol%), SPhos (10 mol%), n-BuOH/toluene (1 : 1), 120 °C, 12 h, then NEt4OH; (iii): 1a (1 eq.), 3-pyridylboronic acid (6 eq.), K3PO4 (3 eq.), Pd2(dba)3 (5 mol%), SPhos (10 mol%), n-BuOH/toluene (1 : 1), 120 °C, 12 h, then NEt4OH. | |
The complexes 1a and 1b were then used as substrates for a Pd-catalyzed Suzuki–Miyaura cross-coupling reaction with 3- or 4-pyridylboronic acid using Buchwald's ligand SPhos.8,9 The reaction was found to give the desired coupling products 2a, 2b, and 3 in good to excellent yields (Scheme 1).
Subsequently, we have examined whether coupling reactions in meta position with respect to the boronate ester function are possible. We have therefore prepared clathrochelate 4 (Scheme 2) using 3-bromophenylboronic acid (yield: 67%). This complex was then subjected to the Buchwald-type coupling conditions used before. The desired dipyridyl ligand 5 could be isolated in 91% yield (Scheme 2).
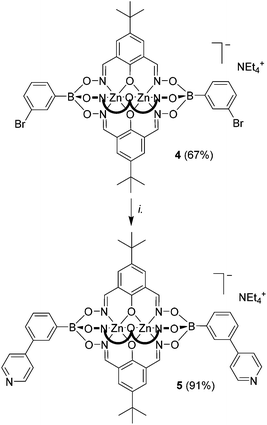 |
| Scheme 2 Synthesis of the bent metalloligand 5. Reagents and conditions: (i): 4 (1 eq.), 4-pyridylboronic acid (6 eq.), K3PO4 (3 eq.), Pd2(dba)3 (5 mol%), SPhos (10 mol%), n-BuOH/toluene (1 : 1), 120 °C, 12 h, then NEt4OH. | |
The metalloligands 2a/b, 3, and 5 are well soluble in polar organic solvents such as DMSO, MeOH, acetonitrile or CH2Cl2. In line with the depicted structure, the NMR spectra of the diamagnetic Zn complexes 2a, 3, and 5 show one set of signals for the three lateral oximato ligands and one set of signals for the two terminal phenylpyridyl groups. The successful coupling of two pyridyl groups to the clathrochelates was also confirmed by high-resolution mass spectrometry. As observed for other Zn-based clathrochelates,4 solutions of 2a, 3, or 5 are luminescent, with an emission maximum of 445 nm (DMF, λex = 330 nm). The excitation and the emission spectrum of a representative complex, clathrochelate 3, are shown in Fig. 2, and additional spectra are depicted in the ESI.†
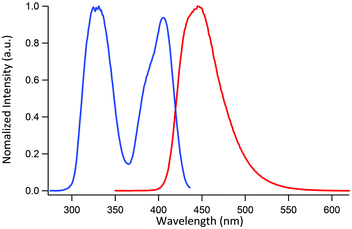 |
| Fig. 2 Excitation spectrum (blue curve, λem = 445 nm) and emission spectrum (red curve, λex = 330 nm) of complex 3 in DMF (0.5 mM). | |
The molecular structure of complex 2a in the crystal was determined by single crystal X-ray crystallography (Fig. 3). The two Zn2+ ions are coordinated in a trigonal prismatic fashion by three nitrogen (Zn–Nav. = 2.13 Å) and three oxygen atoms (Zn–Oav. = 2.12 Å). The oxygen atoms bridge the two metal ions resulting in a M⋯M distance of 2.971(1) Å. The terminal nitrogen atoms of the two pyridyl groups are 2.7 nm apart from each other. This metalloligand is thus substantially longer than the previously reported clathrochelate based on 4-pyridylboronic acid (1.8 nm; Fig. 1),4 and it is comparable in size to pyridyl-capped double clathrochelates introduced by our group (2.7 nm)6 and to bis-(4′-ethynylpyridyl)-functionalized salen ligands (2.4 nm).10
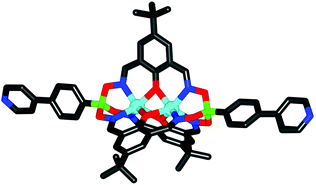 |
| Fig. 3 Molecular structure of 2a in the crystal. Hydrogen atoms, the counterion (NEt4+), and solvent molecules have been omitted for clarity. Color coding: C: grey; O: red; N: blue; B: green; Zn: cyan. | |
Polytopic metalloligands
Next, we explored the reactivity of clathrochelates having bromo-substituents on the lateral oximato ligands. Reaction of 2,6-diformyl-4-bromophenol dioxime with zinc(II) triflate or cobalt(II) nitrate and the respective boronic acid afforded the complexes 6–8 in good yields (Scheme 3). The clathrochelates 6 and 7 have three lateral bromo atoms, whereas complex 8 features a total of five arylbromide groups.
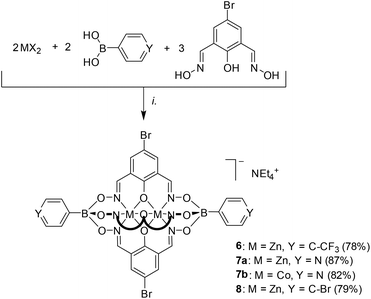 |
| Scheme 3 Synthesis of the polybrominated clathrochelates 6–8. Reagents and conditions: (i): 4-bromophenylboronic acid, Zn(OTf)2 or [Co(H2O)6](NO3)2, MeOH, 70 °C, then NEt4OH. | |
Subsequent Pd-catalyzed cross-coupling with 4-pyridylboronic acid afforded the tripyridyl ligand 9 as well as the pentatopic metalloligands 10a/b and 11 (Scheme 4).
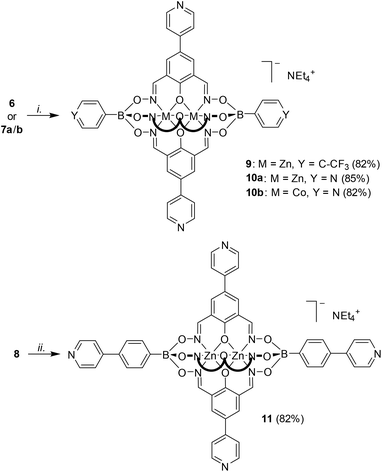 |
| Scheme 4 Synthesis of the tri- and pentatopic metalloligands 9–11. Reagents and conditions: (i): 6 or 7a/b (1 eq.), 4-pyridylboronic acid (15 eq.), K3PO4 (7.5 eq.), Pd2(dba)3 (5 mol%), SPhos (10 mol%), n-BuOH/toluene (1 : 1), 120 °C, 12 h, then NEt4OH; (ii): 8 (1 eq.), 4-pyridylboronic acid (20 eq.), K3PO4 (10 eq.), Pd2(dba)3 (7.5 mol%), SPhos (15 mol%), n-BuOH/toluene (1 : 1), 120 °C, 12 h, then NEt4OH. | |
The polypyridyl ligands 9–11 are well soluble in DMSO and moderately soluble in CH2Cl2 and acetonitrile. The solution-based analysis by NMR spectroscopy and high resolution mass spectrometry confirmed the exhaustive replacement of the bromo atoms with 4-pyridyl groups. The luminescent properties of the Zn complexes 9, 10a, and 11 were in line with what was observed previously, with emission maxima at 445 nm (DMF, λex = 330 nm; Fig. S33†).
Single crystal X-ray structural analyses of 10a and 11 confirmed the presence of five 4-pyridyl groups with divergent coordinate vectors (Fig. 4). The five peripheral nitrogen atoms are arranged in a distorted trigonal bipyramidal fashion. The distances between the two axial nitrogen atoms are 1.8 nm (10a) and 2.7 nm (11), and thus similar to what was found for the ditopic metalloligands described above. The average N⋯N distances for pairs of lateral pyridyl substituents are 1.7 nm (10a) and 1.7 nm (11) and the angles between the oximato ligands are 117.22°, 117.22°, and 125.57° for 10a, and 100.15°, 126.60° and 138.25° for 11. The deviations from the ideal 120° angle is likely due to packing effects in the crystal. The bond lengths and angles observed for the bimetallic core of the clathrochelates are within the expected range.
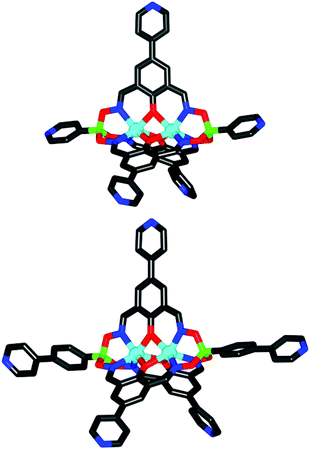 |
| Fig. 4 Molecular structures of the pentatopic metalloligands 10a (top) and 11 (bottom) in the crystal. Hydrogen atoms, counterions (NEt4+), and solvent molecules have been omitted for clarity. Color coding: C: gray; O: red; N: blue; B: green; Zn: cyan. | |
The good success of the five-fold coupling reactions with precursor 7a/b prompted us to push the synthetic concept even further. We thus synthesized clathrochelate 12 having seven bromine atoms. The complete surface functionalization of this complex was achieved under slightly more forcing coupling conditions (10 mol% Pd; Scheme 5). The resulting heptapyridyl ligand 13 was characterized by NMR spectroscopy and high resolution mass spectrometry. Attempts to obtain single crystals of 13 were unfortunately not successful. In order to obtain an estimate of the dimensions of this metalloligand, we have performed an exploratory computational study of its geometry using Density Functional Theory (DFT)11 with the PBE0 exchange–correlation functional.12 The robustness of the method was first evaluated by comparing the XRD structure of clathrochelate 2a with the computed geometry of 2a, which resulted in a good match. We then calculated the minimum-energy structure of 13, and the result is shown in Fig. 5.
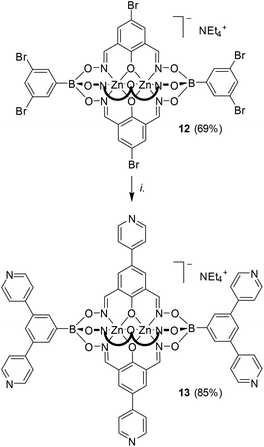 |
| Scheme 5 Synthesis of the heptatatopic metalloligand 13. Reagents and conditions: (i): 12 (1 eq.), 4-pyridylboronic acid (28 eq.), K3PO4 (14 eq.), Pd2(dba)3 (10 mol%), SPhos (20 mol%), n-BuOH/toluene (1 : 1), 120 °C, 12 h, then NEt4OH. | |
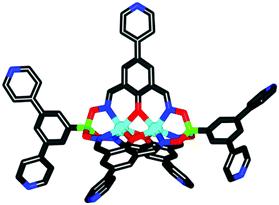 |
| Fig. 5 Minimum-energy structure of the heptatatopic metalloligand 13 obtained by DFT/PBE0. Only the anionic clathrochelate was calculated. Hydrogen atoms have been omitted for clarity. Color coding: C: gray; O: red; N: blue; B: green; Zn: cyan. | |
For clathrochelate 13, the computed average N⋯N distance for pairs of lateral pyridyl substituents is 1.7 nm, a value which is in line with the measured values for 10a and 11. The maximum N⋯N distance between opposite pyridyl groups of the terminal 3,5-(dipyridin-4-yl)phenyl moieties is 2.3 nm. These values give an idea about the size of this unusual heptatopic metalloligand.
Conclusions
We have shown that brominated clathrochelate complexes of Zn and Co can be used as substrates for Pd-catalyzed cross-coupling reactions. As nucleophilic reaction partner, we have employed 3- or 4-pyridylboronic acid. The poly-cross-coupling reactions are remarkably efficient and give the desired functionalized clathrochelates in high yields. In view of the characteristics of the new polypyridyl ligands (large, relatively rigid, anionic, and luminescent in case of M = Zn), we expect that they will be of interest as building blocks for supramolecular chemistry and materials science. The ditopic metalloligands 2, 3, and 5 could be used for the construction of molecularly defined nanostructures, as already demonstrated for shorter analogues.4,5,13 The polytopic metalloligands 9–11 and 13, on the other hand, appear to be more suited for the construction of polymeric structures such as metal–organic frameworks (MOFs). The penta- and heptatopic ligands 10, 11, and 13 are particularly interesting in this context, because MOFs based on ligands with five or seven donor groups have hardly been studied.14,15
For the present investigation, we have focused on the preparation of polypyridyl ligands. However, it appears likely that brominated clathrochelate complexes can be coupled in a similar fashion to boronic acids featuring other functional groups (e.g. cyano groups). We thus predict that brominated clathrochelates should become a versatile and easy-to-access scaffold for the synthesis of novel metalloligands with an unusual trigonal bipyramidal geometry.
Acknowledgements
The work was supported by the Swiss National Science Foundation and by the Ecole Polytechnique Fédérale de Lausanne (EPFL). We are grateful to the Swiss-Norwegian Beamline Consortium for providing access to synchrotron radiation.
Notes and references
-
(a) L. LI, D. J. Fanna, N. D. Shepherd, L. F. Lindoy and F. Li, J. Inclusion Phenom. Macrocyclic Chem., 2015, 82, 3 CrossRef CAS;
(b) H. Li, Z.-J. Yao, D. Liu and G.-X. Jin, Coord. Chem. Rev., 2015, 293–294, 139 CrossRef CAS;
(c) G. Kumar and R. Gupta, Chem. Soc. Rev., 2013, 42, 9403 RSC;
(d) E. C. Constable, Chimia, 2013, 67, 388 CrossRef CAS PubMed;
(e) M. C. Das, S. Xiang, Z. Zhang and B. Chen, Angew. Chem., Int. Ed., 2011, 50, 10510 CrossRef CAS PubMed;
(f) E. C. Constable, Coord. Chem. Rev., 2008, 252, 842 CrossRef CAS.
-
(a) E. Iengo, P. Cavigli, D. Milano and P. Tecilla, Inorg. Chim. Acta, 2014, 417, 59 CrossRef CAS;
(b) I. Beletskaya, V. S. Tyurin, A. Y. Tsivadze, R. Guilard and C. Stern, Chem. Rev., 2009, 109, 1659 CrossRef CAS PubMed;
(c) S. J. Lee and J. T. Hupp, Coord. Chem. Rev., 2006, 250, 1710 CrossRef CAS;
(d) E. Iengo, E. Zangrando and E. Alessio, Acc. Chem. Res., 2006, 39, 841 CrossRef CAS PubMed;
(e) K. S. Suslick, P. Bhyrappa, J. H. Chou, M. E. Kosal, S. Nakagaki, D. W. Smithenry and S. R. Wilson, Acc. Chem. Res., 2005, 38, 283 CrossRef CAS PubMed.
-
(a) A. K. Crane and M. J. MacLachlan, Eur. J. Inorg. Chem., 2012, 1, 17 CrossRef;
(b) M. J. Wiester, P. A. Ulmann and C. A. Mirkin, Angew. Chem., Int. Ed., 2011, 50, 114 CrossRef CAS PubMed;
(c) S. J. Wezenberg and A. W. Kleij, Angew. Chem., Int. Ed., 2008, 47, 2354 CrossRef CAS PubMed.
- M. Pascu, M. Marmier, C. Schouwey, R. Scopelliti, J. J. Holstein, G. Bricogne and K. Severin, Chem. – Eur. J., 2014, 20, 5592 CrossRef CAS PubMed.
- M. Marmier, M. D. Wise, J. J. Holstein, P. Pattison, K. Schenk, E. Solari, R. Scopelliti and K. Severin, Inorg. Chem., 2016, 55, 4006 CrossRef CAS PubMed.
- M. D. Wise, A. Ruggi, M. Pascu, R. Scopelliti and K. Severin, Chem. Sci., 2013, 4, 1658 RSC.
-
(a) O. A. Varzatskii, I. N. Denisenko, S. V. Volkov, A. S. Belov, A. V. Dolganov, A. V. Vologzhanina, V. V. Novikov, Y. N. Bubnov and Y. Z. Voloshin, Eur. J. Inorg. Chem., 2013, 18, 3178 CrossRef;
(b) A. S. Belov, A. I. Prikhod'ko, V. V. Novikov, A. V. Vologzhanina, Y. N. Bubnov and Y. Z. Voloshin, Eur. J. Inorg. Chem., 2012, 28, 4507 CrossRef;
(c) Y. Z. Voloshin, O. A. Varzatskii, S. V. Shul'ga, I. N. Denisenko, A. V. Vologzhanina and Y. N. Bubnov, Inorg. Chem. Commun., 2012, 17, 128 CrossRef CAS;
(d) O. A. Varzatskii, Y. Z. Voloshin, S. V. Korobko, S. V. Shulga, R. Krämer, A. S. Belov, A. V. Vologzhanina and Y. N. Bubnov, Polyhedron, 2009, 28, 3431 CrossRef CAS.
- T. E. Barder, S. D. Walker, J. R. Martinelli and S. L. Buchwald, J. Am. Chem. Soc., 2005, 127, 4685 CrossRef CAS PubMed.
- For the synthesis of Ru-based metalloligands via Pd-catalyzed cross coupling reactions see: J. Yang, J. K. Clegg, Q. Jiang, X. Lui, H. Yan, W. Zhong and J. E. Beves, Dalton Trans., 2013, 42, 15625 RSC.
-
(a) G. Li, C. Zhu, X. Xi and Y. Cui, Chem. Commun., 2009, 2118 RSC;
(b) S.-S. Sun, C. L. Stern, S. T. Nguyen and J. T. Hupp, J. Am. Chem. Soc., 2004, 126, 6314 CrossRef CAS PubMed.
-
(a) P. Hohenberg and W. Kohn, Phys. Rev. B: Condens. Matter, 1964, 136, B864 Search PubMed;
(b) W. Kohn and L. J. Sham, Phys. Rev., 1965, 140, 1133 CrossRef.
-
(a) J. P. Perdew, M. Ernzerhof and K. Burke, J. Chem. Phys., 1996, 105, 9982 CrossRef CAS;
(b) M. Ernzerhof and G. E. Scuseria, J. Chem. Phys., 1999, 110, 5029 CrossRef CAS;
(c) C. Adamo and V. Barone, J. Chem. Phys., 1999, 110, 6158 CrossRef CAS.
- For examples see:
(a) S. Jansze, G. Cecot, M. D. Wise, K. O. Zhurov, T. K. Ronson, A. M. Castilla, A. Finelli, P. Pattison, E. Solari, R. Scopelliti, G. E. Zelinskii, A. V. Volgzhanina, Y. Z. Voloshin, J. R. Nitschke and K. Severin, J. Am. Chem. Soc., 2016, 138, 2046 CrossRef CAS PubMed;
(b) M. D. Wise, J. J. Holstein, P. Pattison, C. Besnard, E. Solari, R. Scopelliti, G. Bricogne and K. Severin, Chem. Sci., 2015, 6, 1004 RSC;
(c) A. Ardavan, A. M. Bowen, A. Fernandez, A. J. Fielding, D. Kaminski, F. Moro, C. A. Muryn, M. D. Wise, A. Ruggi, E. J. L. McInnes, K. Severin, G. A. Timco, C. R. Timmel, F. Tuna, G. F. S. Whitehead and R. E. P. Winpenny, NPJ Quantum Inf., 2015, 1, 15012 CrossRef;
(d) Y.-Y. Zhang, Y.-J. Lin and G.-X. Jin, Chem. Commun., 2014, 50, 2327 RSC.
- For recent reviews about polytopic ligands see:
(a) T.-H. Chen, I. Popov, W. Kaveevivitchai and O. Š. Miljanić, Chem. Mater., 2014, 26, 4322 CrossRef CAS;
(b) V. Guillerm, D. Kim, J. F. Eubank, R. Luebke, X. Liu, K. Adil, M. S. Lah and M. Eddaoudi, Chem. Soc. Rev., 2014, 43, 6141 RSC;
(c) Z.-J. Lin, J. Lü, M. Hong and R. Cao, Chem. Soc. Rev., 2014, 43, 5867 RSC;
(d) W. Lu, Z. Wei, Z.-Y. Gu, T.-F. Liu, J. Park, J. Park, J. Tian, M. Zhang, Q. Zhang, T. Gentle Iii, M. Bosch and H.-C. Zhou, Chem. Soc. Rev., 2014, 43, 5561 RSC;
(e) M. Li, D. Li, M. O'Keeffe and O. M. Yaghi, Chem. Rev., 2013, 114, 1343 CrossRef PubMed;
(f) T. R. Cook, Y. Zheng and P. J. Stang, Chem. Rev., 2013, 113, 734 CrossRef CAS PubMed.
- For examples of MOFs based on pentatopic ligands see:
(a) J. Li, G.-P. Yang, S.-L. Wei, R.-C. Gao, N.-N. Bai and Y.-Y. Wang, Cryst. Growth Des., 2015, 15, 5382 CrossRef CAS;
(b) M.-L. Ma, J.-H. Qin, C. Ji, H. Xu, R. Wang, B.-J. Li, S.-Q. Zang, H.-W. Hou and S. R. Batten, J. Mater. Chem. C, 2014, 2, 1086 Search PubMed;
(c) L. Bai, H.-B. Wang, D.-S. Li, Y.-P. Wu, J. Zhao and L.-F. Ma, Inorg. Chem. Commun., 2014, 44, 188 CrossRef CAS;
(d) D. Wang, T. Zhao, Y. Cao, S. Yao, G. Li, Q. Huo and Y. Liu, Chem. Commun., 2014, 50, 8648 RSC;
(e) X. Duan, R. Song, J. Yu, H. Wang, Y. Cui, Y. Yang, B. Chen and G. Qian, RSC Adv., 2014, 4, 36419 RSC;
(f) J. Park, J.-R. Li, Y.-P. Chen, J. Yu, A. A. Yakovenko, Z. U. Wang, L.-B. Sun, P. B. Balbuena and H.-C. Zhou, Chem. Commun., 2012, 48, 9995 RSC;
(g) P. Zhang, B. Li, Y. Zhao, X. Meng and T. Zhang, Chem. Commun., 2011, 47, 7722 RSC.
Footnote |
† Electronic supplementary information (ESI) available: Experimental details, analytical data and crystallographic data. CCDC 1453506–1453508. For ESI and crystallographic data in CIF or other electronic format see DOI: 10.1039/c6dt01288d |
|
This journal is © The Royal Society of Chemistry 2016 |