DOI:
10.1039/C5DT04062K
(Paper)
Dalton Trans., 2016,
45, 1638-1647
Synthesis and reactions of N-heterocycle functionalised variants of heterometallic {Cr7Ni} rings†
Received
20th October 2015
, Accepted 20th November 2015
First published on 22nd December 2015
Abstract
Here we present a series of linked cage complexes of functionalised variants of the octametallic ring {Cr7Ni} with the general formula [nPr2NH2][Cr7NiF8(O2CtBu)15(O2CR)], where HO2CR is a N-heterocycle containing carboxylic acid. These compounds are made by reacting [nPr2NH2][Cr7NiF8(O2CtBu)15(O2CR)] with a variety of simple metal salts and metal dimers. The carboxylic acids studied include iso-nicotinic acid, 3-(4-pyridyl)acrylic acid and 4-pyridazine carboxylic acid. These new linked cage complexes have been studied structurally and the study highlights the versatility of functionalised {Cr7Ni} as a Lewis base ligand. As {Cr7Ni} is a putative molecular electron spin qubit this work contributes to our understanding of the chemistry that might be required to assemble molecular spin qubits.
Introduction
Several strategies have been proposed to create qubits that could act as the fundamental unit of a quantum computer; these include quantum dots,1 semiconductor nanowires,2 large atomic ensembles,3 ion traps,4 defects in solids,5 carbon nanotubes,6 nuclear spin7 and molecular nanomagnets.8 Molecular nanomagnets have one major advantage over most other strategies, which is that through chemistry it is possible to engineer the interaction between individual qubits. We have been studying heterometallic rings as possible qubits and have shown they have reasonable coherence times, and that they can be linked so that spin on individual rings entangle with neighbours.9 Here we report coordination chemistry to produce a series of functionalised rings.
Functionalisation of [nPr2NH2][Cr7NiF8(O2CtBu)16)] (hereafter {Cr7Ni}, Fig. 1) rings with N-heterocycle functional groups allows the ring to act as a Lewis base towards metal sites, essentially acting as a bulky N-donor ligand.10 By synthesising the functionalised {Cr7Ni} rings prior to binding to another metal or simple dimer, we produce a controlled approach to synthesis of linked systems. This should allow the design of appropriate linkers with the capability of providing switchable communication between qubits. The functionalised rings have the general formula [nPr2NH2][Cr7NiF8(O2CtBu)15(O2CR)], herein {Cr7Ni(O2CR)}, where HO2CR is either iso-nicotinic acid (HO2C5H4N, herein HO2C-py), 3-(4-pyridyl)acrylic acid (HO2C2H2C5H4N, herein HO2C-4pyac) or 4-pyridazine carboxylic acid (HO2C4H3N2, herein HO2C-pyd). These complex N-donor ligands have remarkable solubility in non-polar solvents and are very sterically demanding. In the following we discuss the diversity of structures that can be formed from reactions with simple metal salts and metal dimers. The range of metal used represents a wide selection from across the periodic table including 3d, 4d and 5d transition metals with a range of anions, including chelating and bridging ligands. The resulting complexes are discussed in terms of their structure and crystal packing.
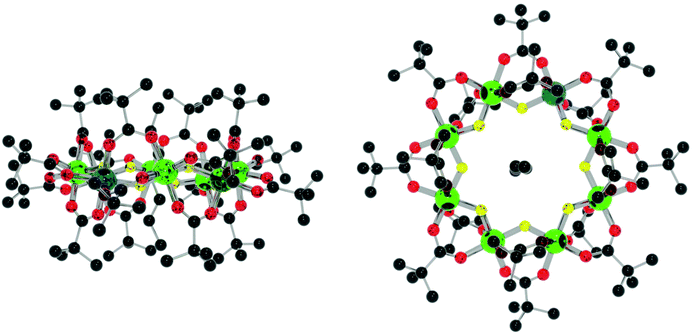 |
| Fig. 1 Side and top views of {Cr7Ni} in the crystal. Cr = green, Ni = turquoise, C = black, O = red, N = blue, F = yellow. H-atoms omitted for clarity. | |
Experimental
Column chromatography was carried out using Silica 60A (particle size 35–70 μm, Fisher, UK) as the stationary phase, and TLC was performed on pre-coated silica gel plates (0.25 mm thick, 60 F254, Merck, Germany). Elemental analyses were performed by departmental services at The University of Manchester. Carbon, nitrogen and hydrogen analysis was performed using a Flash 200 elemental analyser. Metal analysis was performed by Thermo iCap 6300 Inductively Coupled Plasma Optical Emission Spectroscopy (ICP-OES).
Starting materials
All reagents and solvents used to synthesise the functionalised rings and linkers were commercially available and used as received. [nPr2NH2][Cr7NiF8(O2CtBu)16],11 [nPr2NH2][Cr7NiF8(O2CtBu)15(O2CC5H4N)], 1,9c [Ni2(μ-OH2)(O2CtBu)4(HO2CtBu)4]12 and [Co2(μ-OH2)(O2CtBu)4(HO2CtBu)4]13 were prepared by published methods. Copper(II) pivalate, [Cu2(O2CtBu)4(HO2CtBu)2], was synthesised in an analogous procedure to [Ni2(μ-OH2)(O2CtBu)4(HO2CtBu)4]. [nPr2NH2][Cr7NiF8(O2CtBu)15(O2CC2H2C5H4N)], 2, and [nPr2NH2][Cr7NiF8(O2CtBu)15(O2CC4H3N2)], 3, were synthesised by an analogous procedure to 1, the details of which are in the ESI.†
Coordination compounds with rings as ligands
The linked ring systems are obtained from the reaction of the corresponding N-heterocycle functionalized {Cr7Ni} ring, in slight excess, and the link of choice in an appropriate solvent, usually hot acetone or toluene. The reaction mixture is then stirred for anywhere between 5 and 20 minutes (with the exception of ReCl(CO)5 and Rh2(O2CCH3)4 due to their inertness) during which time a precipitate may have formed. If a precipitate forms, the solution is cooled, the precipitate collected, washed with the same solvent used in the reaction and recrystallized from an appropriate solvent mix. If no precipitate forms the reaction solution is left to cool and in most cases the desired product will crystallise in 24–48 hours. An example is given for compound 4 below and in detail for all compounds in the ESI.† The reagents involved, yield and elemental analysis are listed in Table 1.
Table 1 Reactants, yields and elemental analyses for compounds 4–21
Linker |
Ring |
Product |
Yield/%a |
Elemental analysis/% |
Calculated (found) |
All yields based on linker.
|
Cu(NO3)2·2.5H2O |
1
|
4
|
71.7 |
Calc. for: C174H312Cr14Cu1F16N6Ni2O71 |
C43.20 H6.50 N1.74 Cr15.05 Ni2.43 Cu1.31 |
(C43.05 H6.54 N1.66 Cr15.25 Ni2.42 Cu1.26) |
AgNO3 |
1
|
5
|
6.7 |
Calc. for: C174H310AgCr14F16N5Ni2O67 |
C43.59 H6.52 N1.46 |
(C43.65 H6.63 N1.40) |
Cu(NO3)2·2.5H2O |
2
|
6
|
52.0 |
Calc. for: C178H316Cr14CuF16N6Ni2O71 |
C43.73 H6.51 N1.72 Cr14.89 Ni2.40 Cu1.30 |
(C43.51 H6.55 N1.71 Cr14.94 Ni2.35 Cu1.23) |
Ni(acac)2(H2O)2 |
1
|
7
|
38.0 |
Calc. for: C184H324Cr14F16N4Ni3O68 |
C45.21 H6.68 N1.15 Cr14.89 Ni3.60 |
(C44.92 H6.86 N1.21 Cr14.77 Ni3.53) |
Ni(F3acac)2(H2O)2 |
1
|
8
|
45.7 |
Calc. for: C184H318Cr14F22N4Ni3O68 |
C44.23 H6.42 N1.12 Cr14.57 Ni3.52 |
(C43.95 H6.57 N1.18 Cr14.52 Ni3.62) |
Ni(Hfac)2(H2O)2 |
1
|
9
|
42.0 |
Calc. for: C184H312Cr14F28N4Ni3O68 |
C43.30 H6.16 N1.09 Cr14.26 Ni3.45 |
(C43.02 H6.37 N1.08 Cr14.02 Ni3.36) |
Cu(Hfac)2(H2O)2 |
1
|
10
|
42.9 |
Calc. for: C184H312Cr14CuF28N4Ni2O68 |
C43.25 H6.15 N1.10 Cr14.25 Ni2.30 Cu 1.24 |
(C43.15 H6.18 N1.11 Cr13.92 Ni2.29 Cu 1.23) |
Mn(Hfac)2(H2O)2 |
1
|
11
|
48.6 |
Calc. for: C184H312Cr14F28MnN4Ni2O68 |
C43.33 H6.17 N1.10 Cr14.27 Ni2.30 Mn1.08 |
(C43.12 H6.23 N1.09 Cr14.08 Ni2.33 Mn1.02) |
Ni(Hfac)2(H2O)2 |
3
|
12
|
39.0 |
Calc. for: C182H310Cr14F28N6Ni3O68 |
C42.81 H6.12 N1.65 Cr14.26 Ni3.45 |
(C42.47 H6.00 N1.59 Cr13.95 Ni3.46) |
Mn(Hfac)2(H2O)2 |
3
|
13
|
47.0 |
Calc. for: C182H310Cr14MnF28N6Ni2O68 |
C43.27 H6.22 N1.61 Cr13.95 Mn1.05 |
(C43.15 H6.19 N1.66 Cr13.64 Mn0.80) |
ReCl(CO)5 |
1
|
14
|
62.7 |
Calc. for: C177H310ClCr14F16N4Ni2O67Re |
C43.06 H6.33 N1.13 Cr14.74 Re3.77 |
(C42.77 H6.32 N1.07 Cr14.46 Re3.85) |
Cu2(O2CtBu)4(HO2CtBu)2 |
1
|
15
|
89.9 |
Calc. for: C194H346Cr14Cu2F16N4Ni2O72 |
C45.13 H6.75 N1.09 Cr14.10 Ni2.27 Cu2.46 |
(C44.98 H6.87 N1.07 Cr14.39 Ni2.23 Cu2.43) |
[Ni2(μ-H2O)(O2CtBu)4(HO2CtBu)4] |
1
|
16
|
38.5 |
Calc. for: C196H336Cr14F16N6Ni4O72 |
C45.21 H6.77 N1.09 Cr14.13 Ni4.56 |
(C44.99 H6.67 N1.01 Cr13.71 Ni4.78) |
[Co2(μ-H2O)(O2CtBu)4(HO2CtBu)4] |
1
|
17
|
89.6 |
Calc. for: C196H336CoCr14F16N6Ni2O72 |
C45.21 H6.77 N1.08 Cr14.12 Ni2.28 Co2.29 |
(C45.15 H6.74 N1.11 Cr14.03 Ni2.17 Co2.42) |
[Ru2(O2CtBu)4]BF4 |
1
|
18
|
65.4 |
Calc. for: C194H346B1Cr14F20N4Ni2O72Ru2 |
C43.76 H6.55 Cr13.67 Ni2.20 |
(C43.75 H6.60 Cr13.40 Ni2.12) |
Rh2(O2CCH3)4 |
1
|
19
|
89.9 |
Calc. for: C182H322Cr14F16N4Ni2O72Rh2 |
C43.08 H6.40 N1.10 Cr14.35 Ni2.31 Rh4.06 |
(C43.43 H6.56 N1.09 Cr14.38 Ni2.25 Rh3.96) |
Cu2(O2CtBu)4(HO2CtBu)2 |
2
|
20
|
14.0 |
Calc. for: C198H350Cr14Cu2F16N4Ni2O72 |
C45.60 H6.76 N1.07 Cr13.96 Cu2.44 |
(C45.70 H6.85 N1.02 Cr13.59 Cu2.48) |
Cu2(O2CtBu)4(HO2CtBu)2 |
3
|
21
|
44.0 |
Calc. for: C192H344Cr14Cu2F16N6Ni2O72 |
C44.65 H6.71 N1.63 Cr14.09 Cu2.46 |
(C44.78 H6.84 N1.51 Cr13.27 Cu2.47) |
{[nPr2NH2][Cr7NiF8(O2CtBu)15(O2CC5H4N)]}2[Cu(NO3)2(OH2)] 4.
Cu(NO3)2·2.5H2O (0.011 g, 0.049 mmol) was added to a solution of 2 (0.3 g, 0.13 mmol) in hot acetone (30 mL) with stirring. The solution was refluxed for 5 minutes before cooling and left undisturbed in a sealed flask at room temperature. Dark green crystals suitable for single crystal XRD formed after 1 week and were collected by filtration and washed with acetone.
X-ray crystallography
Structures 2, 3, 6, and 17 were collected on a Bruker X8 Prospector 3-circle diffractometer with a copper microfocus source and an APEX II CCD detector. Structure 21 were collected on an Enraf Nonius FR590 4-circle diffractometer with a molybdenum sealed-tube, fine focus molybdenum source, structures 1, 5, 10, 11, 12, 14, 16, 18, 19 and 20 were collected on Advanced Light Source station 11.3.1. Structures were solved and refined using SHELX97 and SHELX-2013. Structures 4, 7, 8, 9, 12 and 15 have already been communicated previously.9c,10d Full crystallographic details for all crystal structures are included in Table S1.† CCDC numbers 1424323–1424336 contain the supplementary crystallographic data for the new structures reported in this paper.
Results and discussion
In all the structures discussed here the heterometallic ring is unchanged from the parent unsubstituted {Cr7Ni} ring.11 The ring contains an octagon of metal sites, with each edge bridged internally by a fluoride and by two carboxylates. On each edge, one carboxylate lies close to the plane of the metal ring while the second carboxylate is perpendicular to the plane of eight metals; this site is labelled as the axial carboxylate, with the in-plane carboxylates described as equatorial.
In the functionalised derivatives the nickel(II) site is always found in the edge that is substituted and is disordered between the two sites in that edge. The incoming carboxylate (e.g. iso-nicotinate) is found in an axial site in the molecules described below and this is always the more common isomer.
Functionalised {Cr7Ni} derivatives
Iso-nicotinate {Cr7Ni}, [nPr2NH2][Cr7NiF8(O2CtBu)15(O2CC5H4N)], 1 (Fig. 2).
Functionalising {Cr7Ni} with iso-nicotinic acid provides the ring with a very sterically congested pyridyl moiety, with the nitrogen of the pyridyl barely protruding beyond the neighbouring pivalate groups. This provides a pathway for spin propagation from the ring to whatever it may bind through the conjugated π-system.
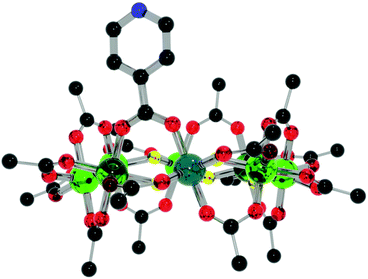 |
| Fig. 2 Structure of 1 in the crystal viewed into the plane of the {Cr7Ni} ring. Colour code: Cr = green, Ni = turquoise, C = black, O = red, N = blue, F = yellow. H-atoms, methyl groups of {Cr7Ni} pivalates and central cation omitted for clarity. | |
3-(4-Pyridyl)acrylate {Cr7Ni}, [nPr2NH2][Cr7NiF8(O2CtBu)15(O2CC2H2C5H4N)], 2 (Fig. 3).
The introduction of the 3-(4-pyridyl)acrylate group provides a longer, less sterically demanding linker whilst still maintaining the potential for spin propagation from the ring to whatever it may bind to through the conjugated π-system.
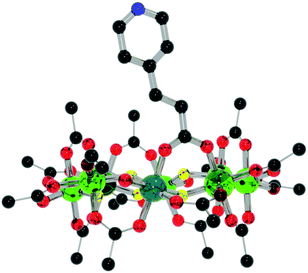 |
| Fig. 3 Structure of 2 in the crystal. Colours and omissions as Fig. 2. | |
4-Pyridazine carboxylate {Cr7Ni}, [nPr2NH2][Cr7NiF8(O2CtBu)15(O2CC4H3N2)], 3 (Fig. 4).
The 4-pyridazine carboxylate group provides a very sterically congested pyridazine moiety thorough which spin propagation can occur with the potential for different coordination modes and strength of coupling compared to 1.
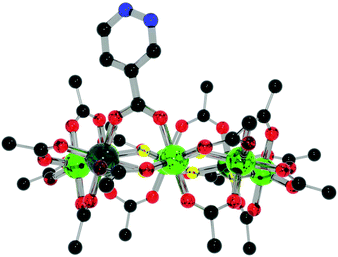 |
| Fig. 4 Structure of 3 in the crystal. Colours and omissions as Fig. 2. | |
Linked {Cr7Ni} systems
The following structures are described using three metric parameters; the torsion angle between the centre of the Cr–Ni edges and the centre of each ring, the N–M–N bond angle (in the case of dimetallic linkers the centroid between the metals is used) and the deviation from co-planar of the two rings. Schematics for the torsion and deviation from co-planar metrics are shown in Fig. 5. If the torsion angle is 180°, the rings are considered staggered and if the angle is 0° they are considered eclipsed and co-planar if there is 0° deviation between the planes.
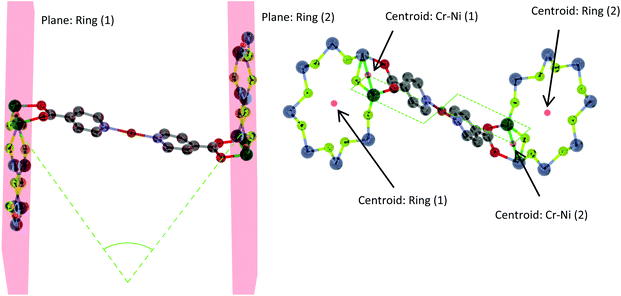 |
| Fig. 5 Schematics for the deviation from co-planar and torsion metrics of the rings. | |
Simple metal salt linked structures
Reactions with group 11 metal nitrates.
The reaction of 1 in a 2.6
:
1 ratio (to provide a slight excess of 1) with Cu(NO3)2·2.5H2O, refluxing in hot acetone for a few minutes and allowing to cool produces {[nPr2NH2][Cr7NiF8(O2CtBu)15(O2C-Py)]}2[Cu(NO3)2(H2O)] 4 with a 72% yield (Fig. 6). The central copper(II) adopts a 7-coordinated geometry that could be described like a distorted pentagonal bipyramid, with two pyridine nitrogen atoms from two different iso-nicotinic groups of two 1 in a trans arrangement and two bidentate nitrate counter ions in a trans disposition and a water molecule completing the pentagonal basal plane. Similar coordination geometries have been seen previously, chiefly in coordination polymers.14 The rings themselves adopt a staggered geometry with a torsion angle of 176.7(2)°. This is most likely due to the short distance between the rings binding through a single metal ion with an eclipsed geometry i.e. with 0° rotation resulting in a steric clash. The rings lie almost parallel with a deviation of 7.2(2)° from co-planarity, with the N–Cu–N angle being 176.3(10)°.
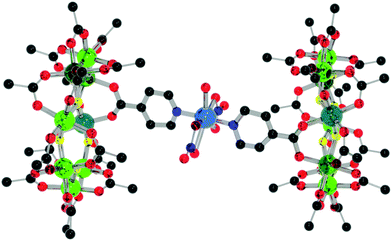 |
| Fig. 6 Structure of 4 in the crystal. Colours and omissions as Fig. 2, Cu = light blue. | |
Reacting 1 with silver nitrate gives {[nPr2NH2][Cr7NiF8(O2CtBu)15(O2C-Py)]}2[AgNO3], 5, with the silver adopting a distorted four-coordinate geometry, similar to other examples of N-donor groups bound to silver nitrate (Fig. 7).15 Unlike 4, the rings adopt an eclipsed geometry with a torsion angle of 8.3(2)°. However, the rings as are not parallel with an angle of 49.8(2)° from planar between the plane of the rings. The iso-nicotinates bind to the silver(I) with an N–Ag–N angle of 145.1(6)° and N–Ag distances of N–Ag 2.246(15) Å and 2.284(15) Å.
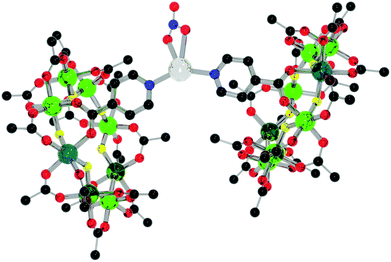 |
| Fig. 7 Structure of 5 in the crystal. Colours and omissions as Fig. 2, Ag = white. | |
The reaction of 2 with hydrated copper(II) nitrate gives [{Cr7Ni(O2C-4pyac)}2Cu(NO3)2(OC3H6)] 6 (Fig. 8). The ethene-link to the pyridyl group in 2 leads to different crystal packing in 6 compared with 4; the rings adopt an eclipsed, rather than staggered geometry with a torsion angle of 19.49(2)° and with a 34.65(2)° deviation from co-planarity. The Cu–N bond distances of 2.146(6) Å for 6 are slightly longer than literature examples of similar motifs (the CSD average is 1.978(4) Å for 3-(4-pyridyl) acrylic moieties bound to {Cu(NO3)2}).14 As with 4, the central copper adopts a 7-coordinate geometry, with the rings binding in a trans disposition with two nitrates and a water molecule in the pentagonal basal plane.
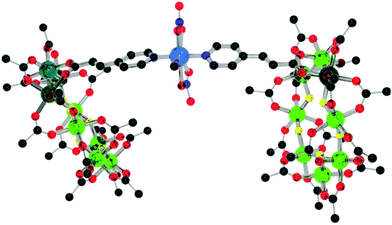 |
| Fig. 8 Structure of 6 in the crystal. Colours and omissions as Fig. 2, Cu = light blue. | |
The reaction of 3 with copper(II) nitrate proved unsuccessful, which was unexpected as 4-pyradizine has previously been shown to coordinate to copper(II) nitrate.16
Reactions of with M(II) acetylacetonate derivatives.
Compound 1 reacts with M(II) acetylacetonate derivatives, including acetylacetonate ([M(II)(acac)2]), 1,1,1-trifluoroacetylacetonate ([M(II)(F3acac)2)]) and 1,1,1,6,6,6-hexafluoroacetylacetonate ([M(II)(Hfac)2]) salts. This produces compounds {[nPr2NH2][Cr7NiF8(O2CtBu)15(O2C-Py)]}2[ML2], (L = acac, M = Ni 7; L = F3acac, M = Ni 8; L = Hfac, M = Ni 9; L = Hfac, M = Cu 10; L = Hfac, M = Mn 11) (9 is shown in Fig. 9). In all cases the central metal lies on an inversion centre with a trans disposition of the ligands. The presence of electron withdrawing F atoms in the acac ligand leads to a shortening of the N–M bond distances from 2.104(7) and 2.122(7) in 7 to 2.075(9) in 8 and 2.074(9) Å in 8 and 9 respectively. In 7, the rings are not co-planar with a deviation of 9.8(2)°, with a torsion angle of 178.9(2)° and a N–Ni–N bond angle of 177.5(3). In contrast, 8 and 9 exhibit perfect co-planarity of the rings, a torsion angle of 180° and an N–M–N bond angle of 180.0°.
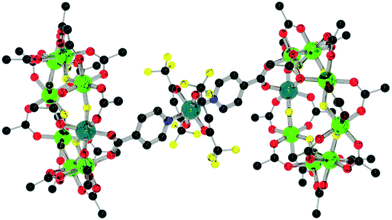 |
| Fig. 9 Structure of 9 in the crystal. Colours and omissions as Fig. 2. | |
The other examples of M(Hfac)2 linked rings (10 and 11) crystallise in a triclinic P
rather than monoclinic C2/c. They have the same disposition of rings as 9, with a torsion angle of 180° with 0° deviation from co-planar, and a N–M–N bond angle of 180. Interestingly, 10 does not appear to display a significant Jahn–Teller elongation, as might be expected from copper(II). However, this could be due to its position on an inversion centre averaging the Cu–O bond lengths. In any case, the Cu–N bond distance from the centre to the ring is shorter than the two Cu–O bond lengths, which could imply that the rings coordinate in the x–y plane of the central copper (Cu–N = 1.993(3) Å, Cu–O1 = 2.065(4) Å and Cu–O2 = 2.178(3) Å). In the case of 11, the central manganese adopts a slightly distorted octahedral geometry with the coordinating nitrogen atoms exhibiting a slightly longer bond length to the oxygen atoms of the Hfac (2.150(3) Å vs. 2.085(3) and 2.089(3) Å).
While 3 did not coordinate to copper(II) nitrate, it acts as a ligand towards M(Hfac)2 derivatives (M = Ni and Mn); again there was no reaction with Cu(Hfac)2. {[nPr2NH2][Cr7NiF8(O2CtBu)15(O2C-pyd)]}2[M(Hfac)2], (M = Ni 12; M = Mn 13) form in decent yields; unfortunately 13 does not crystallise, therefore we are unsure which isomer forms. The structure of 12 shows the Ni(Hfac)2 adopting the cis conformation (Fig. 10), contrasting with the trans-geometry of 9. The rings are eclipsed, with a torsion angle of 26.93(3)° and the rings lie with a 53.73(3)° deviation from co-planar. The Ni–N distance is 2.073(6) Å and the N–Ni–N bond angle is 90.0(3)°. It is unclear why the 4-pyridazinecarboxylate favours a cis-geometry, which seems more sterically demanding. It is also intriguing that 3 seems to bind to Ni(II) but not Cu(II).
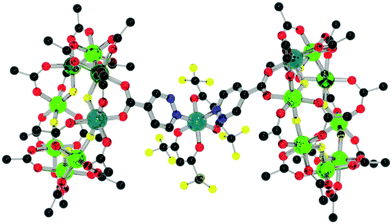 |
| Fig. 10 Structure of 12 in the crystal. Colours and omissions as Fig. 2. | |
Reactions with 5d metals.
We have also fully characterised one example of {Cr7Ni} bound to rhenium by reacting 2 with ReCl(CO)5 to give {[nPr2NH2][Cr7NiF8(O2CtBu)15(O2C-Py)]}2[ReCl(CO)3], 14 (Fig. 11). The rings are half eclipsed with a torsion angle of 72.3(2)° with an angle of 55.2(2)° from co-planarity. The Re–N are 2.13(3) Å and 2.16(3) Å, shorter than the average of 2.23(3) Å found in the CSD for similar ReCl(CO)3(py)2 complexes.17
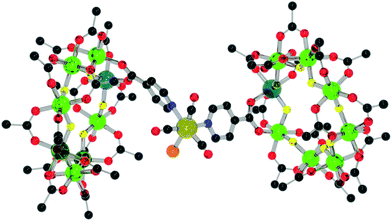 |
| Fig. 11 Structure of 14 in the crystal. Colours and omissions as Fig. 2, Re = dark yellow, Cl = orange. | |
Dimetallic tetracarboxylates
Compound 2 was reacted with a range of dimetallic tetracarboxylates; [Cu2(O2CtBu)4(HO2CtBu)2], [Rh(O2CMe)4] and [Ru2(O2CCtBu)4]BF4, and water-bridged metal carboxylate dimers [M2(μ-OH2)(O2CtBu)4(HO2CtBu)4] (M = Ni or Co). Similar results are found in each case with compounds {[nPr2NH2][Cr7NiF8(O2CtBu)15(O2C-Py)]}2[M2(O2CtBu)4], (M = Cu 15; M = Ni, 16; M = Co, 17; M = Ru 18) and {[nPr2NH2][Cr7NiF8(O2CtBu)15(O2C-Py)]}2[Rh2(O2CMe)4]M = Rh 19) forming in good yields (see Table 2). The water is displaced from the two water-bridged dimers. In all these compounds the N-donor from iso-nicotinate binds to the apical sites of the metal dimers. In the five crystal structures, three crystallise in monoclinic settings (15, 18 and 19) with similar unit cells and with half the assembly in the asymmetric unit. Compound 16, has the highest molecular symmetry with 1/4 of the assembly present in the asymmetric unit in the orthorhombic Pnnm space group. Compound 17, crystallises in tetragonal I41/a and features half the assembly in the asymmetric unit. The crystal for 16 contains huge solvent channels throughout the crystal with total solvent accessible voids of 8579.4 Å3, making the crystal 41.3% solvent accessible voids with a unit cell of volume of 20795 Å3. PLATON calculates the largest nine cavities to have radii ranging from 7.4–5.2 Å. It is unclear why 16 packs in a very different way to the other compounds.
Table 2 Selected metric parameters for compounds 15–19
Compound |
15
|
16
|
17
|
18
|
19
|
M–M bond length/Å |
2.606(2) |
2.542(5) |
2.607(3) |
2.2657(14) |
2.4038(10) |
CSD average M–M bond lengths for similar/Å |
2.604(20) |
2.596(11) |
2.72(4) |
2.279(3) |
2.398(4) |
M–N bond distance/Å |
2.146(6) |
1.97(2) |
2.061(12) |
2.285(9) |
2.223(7) |
CSD average M–N bond lengths for similar/Å |
2.165(16) |
1.993(20) |
2.066(9) |
2.276(6) |
2.231(15) |
The assemblies adopt two distinct geometries. For the compounds with [M2(O2CtBu)4] as linker (15–18), the rings are perfectly staggered with respect to one another and are also completely co-planar (Fig. 12a). The only differences from 15 to 18 are the M–M bond distances, the M–N bond distances, the ring centroid distances and the ring torsion angle (Table 2). For compound 19, where the link is [Rh2(O2CMe)4], the rings are eclipsed with a torsion angle of 47.55° and a deviation of 50.48° from co-planarity (Fig. 12b). The N–M bond length is 2.223(7) Å, slightly shorter than the average found in the CSD of 2.231(15) Å for similar complexes. The N–M2–N bond angle is 177.26(4)°, measured through the dimer centroid and the M–M bond distance is 2.4038(10) Å, slightly longer than the CSD average of 2.398(4) Å found for related compounds.18
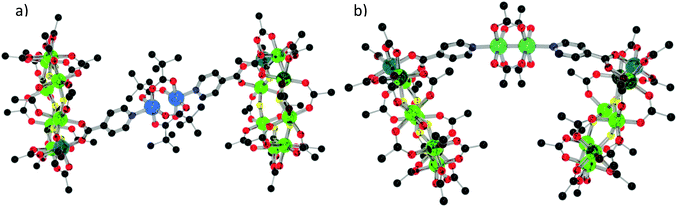 |
| Fig. 12 (a) Structure of 15 in the crystal. (b) Structure of 19 in the crystal. Colours and omissions as Fig. 2 (a) Cu = light blue, (b) Rh = emerald. | |
Whilst 15, 18 and 19 exhibit similar M–M distances to other similar complexes found in the CSD, 16 and 17 exhibit a significant shortening of the M–M distances. In the case of 16, only one similar compound from the CSD has a bond length significantly less than 2.6 Å; one with a {Cr7Ni} bound through a pyridyl group on the thread.10b It would appear that the bulky nature of the coordinating ligand forces the Ni⋯Ni and Co⋯Co bonds to shorten. For 15, favourable 5-coordinate geometry may explain a lack of shortening, while in the case of 18 and 19 the bond order of the metal–metal bond is presumably the controlling factor. For 16, 17 and 19 the central dimetallic link has all carboxylate ligands around 45° from the plane of the iso-nicotinate ligands, while in 15 and 18 two of the carboxylates are almost co-planar with the iso-nicotinates (Fig. 13).
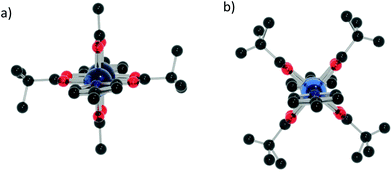 |
| Fig. 13 Orientation of the central metal dimer looking down the coordinating iso-nicotinate groups (a) 15 and 18 and (b) 16 and 17. Colours as Fig. 2, M = light blue. | |
Compounds 2 and 3 also reacts with [Cu2(O2CtBu)4(HO2CtBu)2] to give {[nPr2NH2][Cr7NiF8(O2CtBu)15(O2C-4pyac)]}2[Cu2(O2CtBu)4] 20 (Fig. 14) and {[nPr2NH2][Cr7NiF8(O2CtBu)15(O2C-pyd)]}2[Cu2(O2CtBu)4] 21 (Fig. 15). In 20, the rings bind to the apical positions of the copper sites and the packing is similar to that of 15, with the rings in a perfectly staggered geometry. However, the central bridging unit in 20 adopts the same as that in 16 and 17, with the bridging pivalates out of the plane of the pyridyl groups. The Cu–N bond length is 2.146(6) Å, which is similar to that in 15, but the Cu–Cu distance is 2.5865(14) Å, slightly shorter than 15 and the CSD average.19
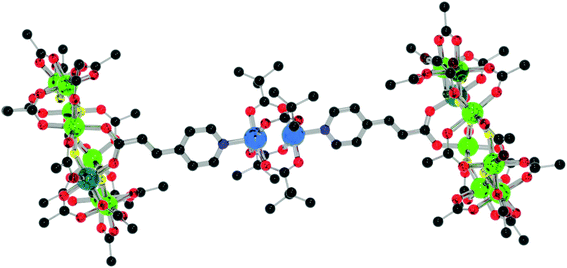 |
| Fig. 14 Structure of 20 in the crystal. Colours and omissions as Fig. 2, Cu = light blue. | |
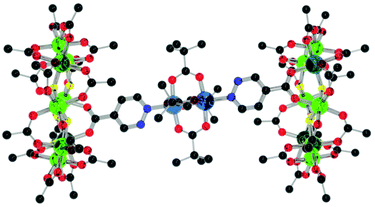 |
| Fig. 15 Structure of 21 in the crystal. Colours and omissions as Fig. 2, Cu = light blue. | |
The structure of 21 is similar to that of 15, with the carboxylates of the central copper in plane with the pyridyl moieties and similar Cu–N bond lengths (2.15(2) and 2.160(12) Å). The Cu–N bond distances are shorter than that for pyridazine bound to copper(II) acetate (2.211(3) Å), implying that 3 is a better donor than plain pyridazine. This could either be due to the presence of the ring or the carboxylate group in the para-position. Unfortunately, no structure is known for 4-pyridazinecarboxylic acid bound to a dimetalic copper pivalate complex, so a direct comparison with the free carboxylic acid is not possible.
It adopts the same perfectly staggered geometry as 15 with the central dimer also adopting the same geometry with respect to the iso-nicotinate groups with a similar ring centroid – dimer centroid angle of 77.13(3)° and a slightly longer Cu–Cu distance of 2.612(4) Å.
The focus of this paper is the structural chemistry of these materials. Magnetic studies of the substituted rings and linked arrays show variable temperature susceptibility behaviour that matches that of the sum of components. As we have reported previously9c,10 interactions between such components tend to too weak to measure using conventional magnetometers and very low temperatures are required to measure the exchange interactions directly. Such measurements are only useful if new physics is to be uncovered. We have recently reported the use of double electron–electron resonance spectroscopy20,21 to study such interactions in other supramolecular species; such studies will be undertaken for some of the compounds reported here in the future.
Conclusions and future perspectives
In summary, we have shown that N-heterocycle functionalised {Cr7Ni} rings can act as Lewis bases towards simple metal salts and metal carboxylate dimers to form linked ring systems. The simplicity of the method, using some of the most basic principles of simple coordination chemistry, coupled with a diverse range of metal salts and carboxylate dimers, means we can produce a range of coordination complexes where functionalised {Cr7Ni} act as bulky ligands. We have established that {Cr7Ni} rings functionalised with different N-heterocycles can all act as bulky Lewis bases, with some subtle variations based on the heterocycle present. This allows a degree of control over the synthesis of linked {Cr7Ni} systems and allows us to work towards the design and realisation of a quantum gate.
This approach to linking {Cr7Ni} furthers its potential for use as a qubit in quantum information processing and quantum simulation applications by addressing one DiVincenzo criteria that {Cr7Ni} does not fulfil.22 Future work will look to the introduction of fully switchable linkers with a look to entanglement of linked {Cr7Ni} in order to implement quantum gate operations. We will also look at introducing hetero-functionality to the {Cr7Ni} in order to fulfil the schematic for a quantum simulator put forward by Santini et al.23
Acknowledgements
This work was supported by the EPSRC(UK) through the Centre for Doctoral Training – NoWNANO and the European Commission (Marie Curie Intra-European Fellowship 622659 to J. F. S.). We also thank EPSRC (UK) for funding an X-ray diffractometer (grant number EP/K039547/1). The ALS is supported by the Director, Office of Science, Office of Basic Energy Sciences, of the US Department of Energy under contract no. DE-AC02-05CH11231.
References
- C. B. Simmons, J. R. Prance, B. J. Van Bael, T. S. Koh, Z. Shi, D. E. Savage, M. G. Lagally, R. Joynt, M. Friesen, S. N. Coppersmith and M. A. Eriksson, Phys. Rev. Lett., 2011, 106, 156804 CrossRef CAS PubMed.
- V. Mourik, K. Zuo, S. M. Frolov, S. R. Plissard, E. P. A. M. Bakkers and L. P. Kouwenhoven, Science, 2012, 336, 1003 CrossRef CAS PubMed.
- R. McConnell, H. Zhang, J. Hu, S. Ćuk and V. Vuletić, Nature, 2015, 519, 439 CrossRef CAS PubMed.
- D. J. Wineland, M. Barrett, J. Britton, J. Chiaverini, B. DeMarco, W. M. Itano, B. Jelenković, C. Langer, D. Leibfried, V. Meyer, T. Rosenband and T. Schätz, Phil. Trans. R. Soc. Lond. A, 2003, 361, 1349–1361 CrossRef CAS PubMed.
- W. F. Koehl, B. B. Buckley, F. J. Heremans, G. Calusine and D. D. Awschalom, Nature, 2011, 479, 84 CrossRef CAS PubMed.
- W. L. Yang, Z. Y. Xu, H. Wei, M. Feng and D. Suter, Phys. Rev. A, 2010, 81, 032303 CrossRef.
- J. J. L. Morton, A. M. Tyryshkin, R. M. Brown, S. Shankar, B. W. Lovett, A. Ardavan, T. Schenkel, E. E. Haller, J. W. Ager and S. A. Lyon, Nature, 2008, 455, 1085 CrossRef CAS.
-
(a) M. Leuenberger and D. Loss, Nature, 2001, 410, 789 CrossRef CAS PubMed;
(b) F. Meier, J. Levy and D. Loss, Phys. Rev. Lett., 2003, 90, 47901 CrossRef PubMed;
(c) J. Lehmann, A. Gaita-Ariño, E. Coronado and D. Loss, Nat. Nanotechnol, 2007, 2, 312 CrossRef CAS PubMed;
(d) F. Troiani and M. Affronte, Chem. Soc. Rev., 2011, 40, 3119 RSC;
(e) S. Nakazawa, S. Nishida, T. Ise, T. Yoshino, N. R. Mori, D. Rahimi, K. Sato, Y. Morita, K. Toyota, D. Shiomi, M. Kitagawa, H. Hara, P. Carl, P. Höfer and T. Takui, Angew. Chem., Int. Ed., 2012, 51, 9860 CrossRef CAS PubMed;
(f) D. Aguilà, L. A. Barrios, V. Velasco, O. Roubeau, A. Repollés, P. J. Alonso, J. Sesé, S. J. Teat, F. Luis and G. Aromí, J. Am. Chem. Soc., 2014, 136, 14215 CrossRef PubMed.
-
(a) F. Troiani, A. Ghirri, M. Affronte, S. Carretta, P. Santini, G. Amoretti, S. Piligkos, G. Timco and R. E. P. Winpenny, Phys. Rev. Lett., 2005, 94, 207208(1) Search PubMed;
(b) C. J. Wedge, G. A. Timco, E. T. Spielberg, R. E. George, F. Tuna, S. Rigby, E. J. L. McInnes, R. E. P. Winpenny, S. J. Blundell and A. Ardavan, Phys. Rev. Lett., 2012, 108, 107204(1) CrossRef PubMed;
(c) G. A. Timco, S. Carretta, F. Troiani, F. Tuna, R. J. Pritchard, C. A. Muryn, E. J. L. McInnes, A. Ghirri, A. Candini, P. Santini, G. Amoretti, M. Affronte and R. E. P. Winpenny, Nat. Nanotechnol., 2008, 4, 173 CrossRef PubMed.
-
(a) G. F. S. Whitehead, F. Moro, G. A. Timco, W. Wernsdorfer, S. J. Teat and R. E. P. Winpenny, Angew. Chem., Int. Ed., 2013, 52, 9932 CrossRef CAS PubMed;
(b) G. F. S. Whitehead, B. Cross, L. Carthy, V. A. Milway, H. Rath, A. Fernandez, S. L. Heath, C. A. Muryn, R. G. Pritchard, S. J. Teat, G. A. Timco and R. E. P. Winpenny, Chem. Commun., 2013, 49, 7195 RSC;
(c) G. F. S. Whitehead, J. Ferrando-Soria, L. G. Christie, N. F. Chilton, G. A. Timco, F. Moro and R. E. P. Winpenny, Chem. Sci., 2014, 5, 235 RSC;
(d) A. Chiesa, G. F. S. Whitehead, S. Carretta, L. Carthy, G. A. Timco, S. J. Teat, G. Amoretti, E. Pavarini, R. E. P. Winpenny and P. Santini, Sci. Rep., 2014, 4, 7423 CrossRef CAS PubMed;
(e) J. Ferrando-Soria, A. Fernandez, E. Moreno Pineda, S. A. Varey, R. W. Adams, I. J. Vitorica-Yrezabal, F. Tuna, G. A. Timco, C. A. Muryn and R. E. P. Winpenny, J. Am. Chem. Soc., 2015, 137, 7644 CrossRef CAS PubMed.
-
(a) F. K. Larsen, E. J. L. McInnes, M. H. El, J. Overgaard, S. Piligkos, G. Rajaraman, E. Rentschler, A. A. Smith, G. M. Smith, V. Boote, M. Jennings, G. A. Timco and R. E. P. Winpenny, Angew. Chem., Int. Ed., 2003, 42, 101 CrossRef CAS;
(b) E. J. L. McInnes, G. A. Timco, G. F. S. Whitehead and R. E. P. Winpenny, Angew. Chem., Int. Ed., 2015, 54, 14244 CrossRef CAS PubMed.
- G. Chaboussant, R. Basler, H.-U. Güdel, S. Ochsenbein, A. Parkin, S. Parsons, G. Rajaraman, A. Sieber, A. A. Smith, G. A. Timco and R. E. P. Winpenny, Dalton Trans., 2004, 2758 RSC.
- K. O. Abdulwahab, M. A. Malik, P. O'Brien, G. A. Timco, F. Tuna, C. A. Muryn, R. E. P. Winpenny, R. A. D. Pattrick, V. S. Coker and E. Arenholz, Chem. Mater., 2014, 26, 999 CrossRef CAS.
-
(a) Q.-X. Liu, Z.-X. Zhao, X.-J. Zhao, Y. Bi, J. Yu and X.-G. Wang, CrystEngComm, 2014, 16, 7023 RSC;
(b) W.-J. Hu, L.-Q. Liu, M.-L. Ma, X.-L. Zhao, Y. A. Liu, X.-Q. Mi, B. Jiang and K. Wen, Inorg. Chem., 2013, 52, 9309 CrossRef CAS PubMed;
(c) X. Fang, X. Yuan, Y.-B. Song, J.-D. Wang and M.-J. Lin, CrystEngComm, 2014, 16, 9090 RSC;
(d) J. E. Beves, E. C. Constable, C. E. Housecroft, M. Neuburger and S. Schaffner, CrystEngComm, 2008, 10, 344 RSC;
(e) Q. Zhao, X.-M. Liu, W.-C. Song and X.-H. Bu, Dalton Trans., 2012, 41, 6683 RSC.
-
(a) C. Richardson and P. J. Steel, Inorg. Chem. Commun., 1998, 1, 260 CrossRef CAS;
(b) C. M. Fitchett and P. J. Steel, Inorg. Chim. Acta, 2000, 310, 127 CrossRef CAS;
(c) W.-L. Jia, R.-Y. Wang, D. Song, S. J. Ball, A. B. McLean and S. Wang, Chem. – Eur. J., 2005, 11, 832 CrossRef PubMed;
(d) B. Antonioli, D. J. Bray, J. K. Clegg, K. Gloe, K. Gloe, O. Kataeva, L. F. Lindoy, J. C. McMurtrie, P. J. Steel, C. J. Sumby and M. Wenzei, Dalton Trans., 2006, 4783 RSC;
(e) X. Ma, L.-H. Huo, Z.-P. Deng, T.-P. Liu, H. Zhao and S. Gao, Inorg. Chem. Commun., 2014, 43, 94 CrossRef CAS.
-
(a) L. Chen, L. K. Thompson and J. N. Bridson, Can. J. Chem., 1993, 71, 1086 CrossRef CAS;
(b) L. Carlucci, G. Ciani, M. Moret and A. Sironi, J. Chem. Soc., Dalton Trans., 1994, 2397 RSC.
-
(a) S. Belanger, J. T. Hupp, C. L. Stern, R. V. Slone, D. F. Watson and T. G. Carrell, J. Am. Chem. Soc., 1999, 121, 557 CrossRef CAS;
(b) N. M. Shavaleev, A. Barbieri, Z. R. Bell, M. D. Ward and F. Barigelletti, New. J. Chem., 2004, 28, 398 RSC;
(c) P. de Wolf, P. Waywell, M. Hanson, S. L. Heath, A. J. H. M. Meijer, S. J. Teat and J. A. Thomas, Chem. – Eur. J., 2006, 12, 2188 CrossRef CAS PubMed.
-
(a) F. A. Cotton and T. R. Felthouse, Inorg. Chem., 1981, 20, 600 CrossRef CAS;
(b) K. Aoki, M. Inaba, S. Teratani, H. Yamazaki and Y. Miyashita, Inorg. Chem., 1994, 33, 3018 CrossRef CAS;
(c) M. Handa, T. Nakao, M. Mikuriya, T. Kotera, R. Nukada and K. Kasuga, Inorg. Chem., 1998, 37, 149 CrossRef CAS PubMed;
(d) J. F. Berry, F. A. Cotton, C. Lin and C. A. Murillo, J. Cluster Sci., 2004, 15, 531 CrossRef CAS;
(e) D. Pogozhev, S. A. Baudron and M. W. Hosseini, Dalton Trans., 2011, 40, 7403 RSC.
-
(a) G. Speier and V. Fulop, J. Chem. Soc., Dalton Trans., 1989, 2331 RSC;
(b) C. B. Aakeröy, A. M. Beatty, J. Desper, M. O'Shea and J. Valdes-Martinez, Dalton Trans., 2003, 3956 RSC;
(c) A. Karmakar, K. Bania, A. M. Baruah and J. B. Baruah, Inorg. Chem. Commun., 2007, 10, 959 CrossRef CAS;
(d) A. V. Yakovenko, S. V. Kolotilov, O. Cador, S. Golhen, L. Ouahab and V. V. Pavlishchuk, Eur. J. Inorg. Chem., 2009, 2354 CrossRef CAS;
(e) M. Fontanet, A.-R. Popescu, X. Fontrodona, M. Rodriguez, I. Romero, F. Teixidor, C. Viñas, N. Aliaga-Alcalde and E. Ruiz, Chem. – Eur. J., 2011, 17, 13217 CrossRef CAS PubMed.
- A. Ardavan, A. Bowen, A. Fernandez, A. Fielding, D. Kaminski, F. Moro, C. A. Muryn, M. Wise, A. Ruggi, E. J. L. McInnes, K. Severin, G. A. Timco, C. Timmel, F. Tuna, G. F. S. Whitehead and R. E. P. Winpenny, NPJ Quantum Information, 2015, 1, 15012 CrossRef.
- A. Fernandez, J. Ferrando-Soria, E. M. Pineda, F. Tuna, I. J. Vitorica-Yrezabal, C. Knappke, J. Ujma, C. A. Muryn, G. A. Timco, P. E. Barran, A. Ardavan and R. E. P. Winpenny, Nat. Commun., 2015 DOI:10.1038/ncomms10240.
- D. Loss and D. P. DiVincenzo, Phys. Rev. A, 1998, 57, 120 CrossRef CAS.
- P. Santini, S. Carretta, F. Troiani and G. Amoretti, Phys. Rev. Lett., 2011, 107, 230502 CrossRef CAS PubMed.
|
This journal is © The Royal Society of Chemistry 2016 |
Click here to see how this site uses Cookies. View our privacy policy here.