DOI:
10.1039/C6CE02123A
(Paper)
CrystEngComm, 2016,
18, 8794-8802
Intercalated soft-crystalline mesophase exhibited by an unsymmetrical twist-bend nematogen†
Received
4th October 2016
, Accepted 29th October 2016
First published on 31st October 2016
Abstract
A number of new states of matter have been reported in recent years for liquid crystal dimers, most notably the twist-bend nematic phase which exhibits spontaneous breaking of symmetry through the emergence of chiral structures in an achiral fluid. In this communication we report on an unsymmetrical liquid crystal dimer that exhibits a transition from the spontaneously chiral twist-bend nematic phase into a novel smectic liquid crystal phase.
Introduction
The observation of a lower temperature mesophase lacking lamellar or positional molecular order (i.e. a nematic-like mesophase),1 later identified as the ‘twist-bend nematic’ phase (NTB),2–10 has motivated a great deal of study into the properties of this intriguing state of matter by a wide range of techniques.11–18 In a typical nematic phase rod-like molecules are orientated, on average, parallel to one another along a vector termed the director, n (Fig. 1a). In the case where the rod-like molecules are chiral the structure twists forming a left or right-handed helix (Fig. 1c). Although the helicoidal model of the NTB phase, proposed independently by Meyer and Dozov and depicted in Fig. 1b,19,20 is supported by experimental data, alternate models have also been proposed.21–24 The observation of direct isotropic liquid to NTB phase transitions, first reported for a mixed dimer/chiral-dopant system25 and then later in pure materials,26 providing a challenge for future theoretical treatment.
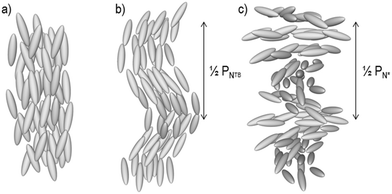 |
| Fig. 1 Local structures and director arrangement in (a) the uniaxial nematic phase, (b) the oblique helicoidal model of the NTB phase and (c) the chiral nematic phase. | |
That the NTB phase has been observed in bent-core systems,8 ether linked dimers,27,28 trimers,29–32 oligomers33 and even polymers34 hints at this mesophase being far more universal than was perhaps first thought. A comprehensive structure property relationship remains elusive despite the ever growing number of compounds reported in the literature to exhibit this state of matter.35–44 The observation of spontaneous breaking of mirror symmetry in the twist-bend nematic phase leads to the emergence of helical nano-structures in an achiral fluid;10,45,46 questions concerning the mechanism of this phenomenon are of fundamental importance to a range of scientific disciplines,47–51 and are likely to motivate further study into the NTB phase (and analogous states of matter such as splay-bend modulated phases52 or possible smectic twist-bend mesophases) for some time to come. Possible applications of dimers and oligomesogens that exhibit twist-bend phases include tuneable selective reflection, presenting another motivation for study of these fascinating materials.53,54
In this work we report an unsymmetrical methylene linked bimesogen containing one polar rigid aromatic unit and one ‘apolar’ unit. In addition to exhibiting nematic and twist-bend nematic mesophases this material also exhibits what we believe to be a previously unobserved smectic mesophase.
Experimental
Compound 1 was synthesised, as shown in Scheme 1, via the EDAC/DMAP mediated Steglich esterification between (4-(9-(4-hydroxyphenyl)nonyl)phenyl 4-cyanobenzoate), prepared as described previously,29 and 4-pentyloxybenzoic acid.
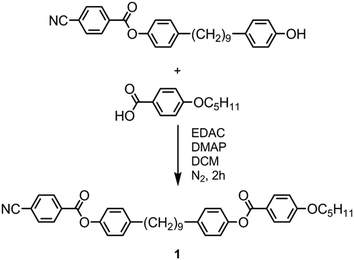 |
| Scheme 1 | |
Small angle X-ray scattering was performed on a Bruker D8 Discover using copper Kα radiation (λ = 0.15418 nm) equipped with a temperature controlled, bored graphite rod furnace. Patterns were collected on a VANTEC 500 area detector (2048 × 2048 pixels) set at a distance of 121 mm from the sample, allowing simultaneous observation of wide angle and small angle scattering data. Computational chemistry was performed at the B3LYP/6-31G(d) level of DFT in Gaussian G09 revision e01,55 with Gaussian output files rendered using Qutemol.56 Full experimental details, including chemical characterisation and descriptions of instrumentation used, are available in the ESI† to this article.
Results
The transition temperatures along with the associated enthalpies and entropies of transition for compound 1 are given in Table 1. Transition temperatures, along with associated enthalpies and entropies were determined by differential scanning calorimetry at a heat/cool rate of 10 °C min−1 and are the mean of 10 cycles; standard deviations are given in parenthesis. The associated enthalpies and entropies of transition are not especially characteristic and are of a comparable magnitude to those reported previously for methylene linked phenylbenzoate dimers.38,39,41,43 Both the onset temperature and associated enthalpy of the SmX–NTB transition temperature were found to be invariant when subjected to different heat/cool rates in the range of 1–20 °C min−1, indicating that the transition is not dependent on kinetic processes; contrast this to the NTB–N transition which was previously shown to show a strong dependence on the heat/cool rate.9 The magnitude of the enthalpy (Table 1) associated with this transition to the SmX phase suggests that the phase is highly ordered. Whereas crystalline phases show thermal hysteresis (i.e. supercooling), liquid-crystalline or soft-crystalline states of matter do not, and cannot typically be supercooled more than a few tens of mK. In order to demonstrate that the SmX phase is a bona fide liquid-crystalline (or possibly soft-crystalline) phase a DSC study was performed whereby the sample was first heated into the isotropic liquid, then cooled into the SmX phase and reheated back into the NTB phase. The lack of thermal hysteresis (Fig. 2) confirms that the transition into the SmX phase is not simply a recrystallization event, but rather a transition to a mesophase.
Table 1 Transition temperatures (°C), associated enthalpies of transition (kJ mol−1) and dimensionless associated entropy of transition (ΔS/R) for compound 1. Note, the SmX–NTB transition is monotropic, i.e. it occurs below the melting point of the sample. Enthalpies and entropies were are the mean of 10 cycles as determined by DSC at a heat/cool rate of 10 °C min−1, with standard deviations given in parenthesis. Tabulated DSC data is presented in the ESI

|
|
MP |
SmX–NTB |
NTB–N |
N–Iso |
T (°C) |
81.4 (0.027) |
56.1 (0.009) |
91.9 (0.007) |
110.8 (0.007) |
ΔH (kJ mol−1) |
35.83 (0.194) |
10.30 (0.004) |
0.31 (0.002) |
0.61 (0.002) |
ΔS/R |
12.15 (0.065) |
3.76 (0.001) |
0.10 (0.001) |
0.19 (0.001) |
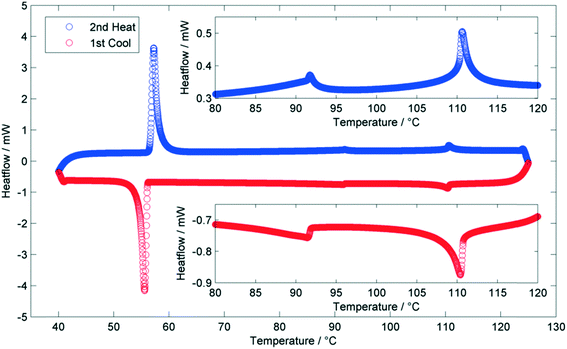 |
| Fig. 2 DSC thermogram of compound 1 obtained at 10 °C min−1 on the first cooling cycle (red circles) and second heating cycle (blue circles), with two expansions showing the region between 80–120 °C which contains the NTB–N and N–Iso transitions. | |
On cooling from the isotropic liquid it is trivial to identify the first mesophase as the nematic, based on its fluidity and characteristic schlieren texture. The identification of the mesophase immediately below the nematic as the twist-bend nematic is also straightforward due to its characteristic optical textures (Fig. 3) and the shape of the peak obtained in DSC thermograms (Fig. 2).9 As discussed by Zhu et al.,13 small-angle X-ray scattering can be used to discriminate between the experimentally observed NTB phase and the theoretically predicted splay-bend nematic, NSB, as the electron density modulation in the former does not lead to Bragg scattering whereas it is expected to in the later. We observe no Bragg scattering at Q values as low as 0.06 in the phase immediately below the nematic (Fig. SI-6 in the ESI†) and so we can be confident in our assignment of this mesophase as the twist-bend modulated nematic.
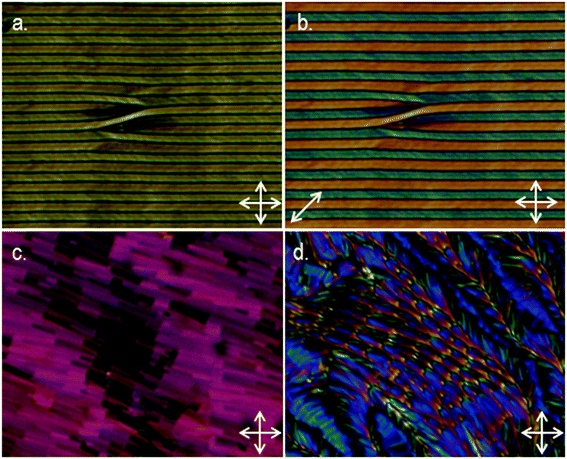 |
| Fig. 3 Photomicrographs (×100) of the twist bend phase of compound 1. (a) The well-aligned rope texture of the NTB phase at 86.5 °C confined in a 5 ± 0.1 μm cell with antiparallel buffed polyimide alignment layers, (b) the same conditions as (a) but with a λ waveplate inserted, (c) the ‘blocky’ texture of the NTB phase at 89.4 °C in a sample on untreated glass, (d) parabolic defects and the ‘blocky’ texture on untreated glass at 87.3 °C. | |
As shown in Fig. 3(a) and (b) a number of crossover points, reminiscent of braids in rope, were seen in the aligned rope texture of the NTB phase, with the ‘length’ of the rope defects being parallel to the rubbing direction of the cell. Insertion of a λ waveplate lead to different colours in adjacent stripes (Fig. 3b), indicating that the optical azimuthal angle alternates between stripes.21 Additionally clear striations can be seen within the stripes (Fig. 3b), these being are offset from the length of the rope defects by an average of ±30° with the direction alternating in adjacent stripes. Further cooling of compound 1 below 56.1 °C leads to a reversible (see DSC trace in Fig. 2) transition from the NTB phase into to the unknown mesophase, denoted SmX presently. When confined in a 5 ± 0.1 μm cell with antiparallel buffed polyimide alignment layers the NTB phase of 1 gives a well aligned rope texture (Fig. 3a and 4a), and at the phase transition the rope texture is partially preserved, either through paramorphosis or this being a characteristic texture of the SmX phase, however the birefringence changes markedly (Fig. 4b and c). If the sample is reheated across the SmX–NTB phase transition then the original rope texture returns (Fig. 4d), however, the interface between adjacent stripes now appear buckled and the striations set at 30° to the rope length, which persist after cooling into the SmX phase, are more pronounced than they are in the virginal texture (compare Fig. 4a–d).
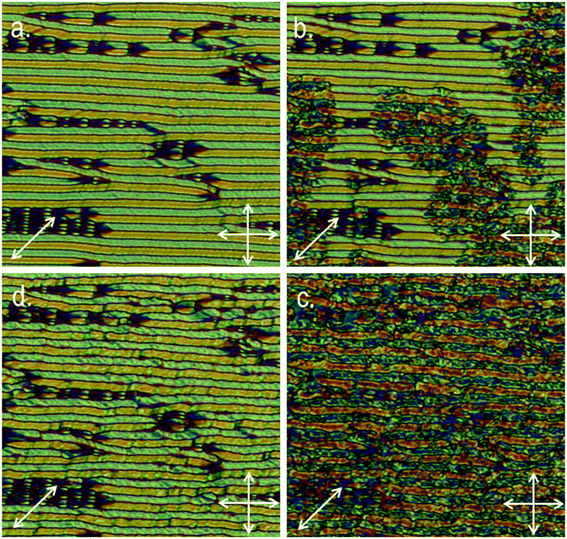 |
| Fig. 4 Photomicrographs, taken with a λ waveplate inserted, of compound 1 (×100) confined in a 5 ± 0.1 μm cell with antiparallel buffed polyimide alignment layers. The rope texture of 1 at 60.0 °C (a), the onset of the SmX–NTB transition at 56.0 °C (b), the rope texture of the SmX phase at 54.5 °C (c), the rope texture of the NTB phase restored after heating through the SmX–NTB transition, 59.2 °C (d). Photomicrographs correspond to approximately the same area of the sample. | |
The behaviour of 1 in a cell was studied under applied electric fields. In the nematic phase at 95 °C a Fréedericksz transition could be observed at low frequency (<50 Hz) with an applied field of above 1 VRMS. The sample was cooled into the NTB phase to 85 °C where it was possible to observe some form of electrooptic response at an applied voltage of 60 VRMS. With a higher applied voltage (100 VRMS) the sample was reversibly into a reasonably uniform homeotropic state, removal of the field lead to the regrowth of the rope-texture over a period of minutes. When cooled into the SmX mesophase we did not observe any electrooptic response at amplitudes of up to 100 VRMS across a range of frequencies (1 mHz to 100 KHz). Therefore we conclude that the SmX phase does not exhibit any electrooptic behaviour in the voltage regime used in this work.
We next elected to study the nematic, NTB and SmX mesophases of 1 by small angle X-ray scattering using copper K alpha radiation on magnetically aligned samples. The nematic phase was well aligned by the external magnetic field and gave the expected diffuse scattering pattern shown in Fig. 5a. This alignment was partially lost on entering the NTB phase (Fig. 5b). For the nematic phase the orientational order parameter P2 (Fig. 5c) was calculated as follows: integration of the wide-angle scattering as a function of the angle χ followed by averaging of the two signals gave Fig. 5d, from this the order parameter could be calculated according to Davidson et al.57 The alignment of the NTB phase was not sufficient to obtain order parameters, however in the nematic phase the order parameter increases from a value of 0.30 to a maximum of 0.39, these values being comparable to those measured for the nematic phase of methylene linked bimesogens.11
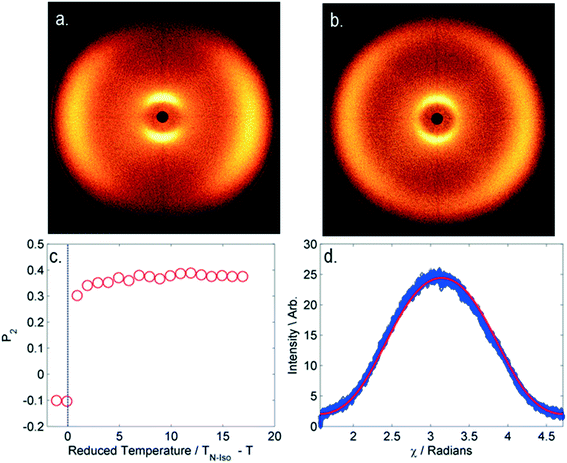 |
| Fig. 5 Two dimensional small-angle X-ray scattering patterns for the nematic phase of 1 at 109 °C (a), the NTB phase of 1 at 89 °C (b), plot of the order parameter P2vs. reduced temperature (c), plot of scattered X-ray intensity (blue diamonds) fitted using as described by Davidson et al. (red line) as a function of the angle χ for the wide-angle (2θ = 15–25) region of compound 1 in the nematic phase at 99 °C (d). | |
As shown in Fig. 6, in the nematic and twist-bend mesophases only diffuse scattering peaks are seen, with a single wide angle peak and a single small angle peak. These scattering peaks corresponding to the side-to-side and end-to-end separation of molecules respectively, however, as the nematic and NTB phases lack positional ordering the small angle peak occurs at roughly ½ of the molecular length (37.2 Å at the B3LYP/6-31G(d) level of DFT). Upon cooling into the SmX phase the diffraction pattern changes markedly in both the small and wide angle regions. The small-angle scattering peak grows in intensity and becomes significantly more defined, with the average full-width at half-maximum (FWHM) being an order of magnitude larger in the NTB phase than in the SmX phase (0.348 Å−1 and 0.035 Å−1 respectively). The out-of-plane correlation length (ξ, defined as ξ = 2π/ΔQ increases from an average of 18.1 Å in the NTB phase to 182.1 Å in the SmX mesophase. The SmX phase also exhibits an scattering peak at Q ≈ 0.17 Å−1 (d ≈ 3.7 nm) that is both more diffuse and less intense than the larger of the two small angle peaks (Q ≈ 0.34 Å−1, d ≈ 2.0 nm).
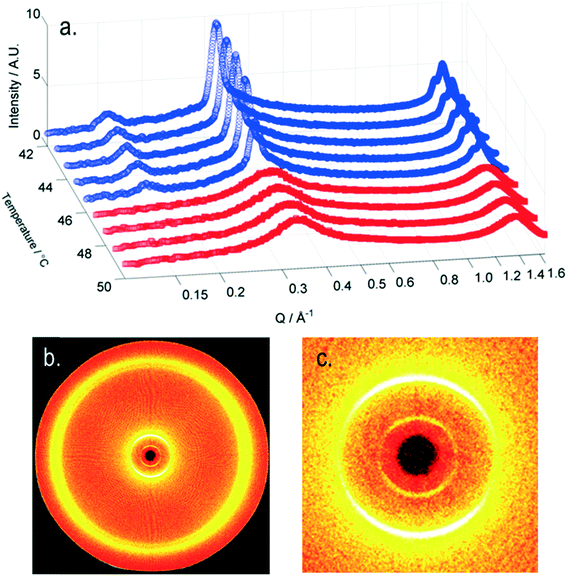 |
| Fig. 6 Small angle X-ray scattering data for compound 1. (a) Plot of diffraction intensity (arbitrary units) versus Q (Å−1) as a function of temperature (42–50 °C, 1 °C steps), with red circles corresponding to the NTB phase and blue circles to the SmX phase. (b) two dimensional diffraction pattern of the SmX mesophase exhibited by 1 at 47 °C and (c) an expansion of the two-dimensional diffraction pattern showing scattering at small angles (2θ = 3–10, Q = 0.1–0.6 Å−1). | |
The diffuse wide-angle peak present in the twist-bend phase sharpens and splits into two peaks that are not fully resolved at the SmX–NTB transition. The increase in scattered intensity in this region at the SmX–NTB phase transition is further evidence for the large increase in the in-plane correlation length. The wide-angle scattering data, which corresponds to the average lateral separation between molecules, was deconvoluted into two Lorentzian peaks with maxima (Fig. 7) at Q = 1.3296 Å−1 (d ≈ 4.73 Å) and Q = 1.4114 Å−1 (d ≈ 4.45 Å). In the more ordered SmX phase we propose that there are two different average lateral intermolecular distances, one for the polar phenyl 4-cyanobenzoate unit and one for the ‘apolar’ phenyl 4-pentyloxybenzoate group.
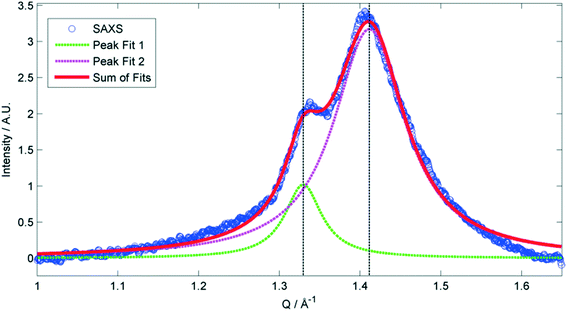 |
| Fig. 7 Plot of scattered X-ray intensity for compound 1 in the SmX mesophase at 50 °C between Q 1.0 and Q 1.65. The overlapping peaks were deconvoluted into two Lorentzian peaks (dashed lines, Imax at Q = 1.3296 Å−1 and Q = 1.4114 Å−1 respectively) which sum to give the solid red line, giving an R2 value of >0.99. | |
From experimental data it is known that the SmX phase is intercalated, with a layer spacing of ½ the molecular length (i.e. d/l ratio of 0.5). The in-plane correlation length is relatively large (average of 18 nm) and although we do not observe evidence of hexagonal close packing of molecules this cannot be excluded as the sample is not aligned during SAXS on the SmX phase (Fig. 6b). Assuming the polar phenyl 4-cyanobenzoate units form antiparallel correlated pairs with adjacent polar units, mirroring what is observed in calamitic systems such as 8CB,58,59 interdigitated smectic layers will result. The antiparallel correlation between adjacent polar phenyl 4-cyanobenzoates is possibly also the origin of the two distinct spacings seen in the wide angle X-ray scattering experiment (Fig. 7). Additionally this mixed polar/apolar molecular structure will lead to segregation of polar and apolar mesogenic units into adjacent layers. We propose that the scattering peak in the small angle at Q ≈ 0.17 Å−1 (d ≈ 3.7 nm) is the distance between two ‘like’ (e.g. polar–polar and apolar–apolar) layers, which is roughly the molecular length, while the layer spacing at Q ≈ 0.34 Å−1 (d ≈ 2.0 nm) is the average layer spacing of the two layers and is approximately ½ of the molecular length.
Conclusions
We have prepared and characterised a novel unsymmetrical liquid crystalline dimer which consists of one polar and one non-polar mesogenic unit. In addition to exhibiting the highly topical twist-bend nematic phase this compound also exhibits a smectic mesophase. Transitions from the NTB phase to smectic mesophases still remain rare (we are aware of only a handful of examples) and present rich opportunities for physical study and theoretical treatment of both the local structure, and the processes occurring at the phase transition between, these two mesophases. We note that the lower-temperature phase observed in compound 1 appears unique – based on small angle X-ray scattering data we propose that the local structure is lamellar, with alternating blocks of ‘polar’ and ‘apolar’ mesogenic units. It is believed that this mesophase arises due to the strong intermolecular self-association of nitrile units. With this point in mind it seems logical that other groups prone to self-association will also support the formation of this mesophase. Studies of the phase by microscopy, calorimetry, SAXS and under applied fields indicate it is soft-crystalline in nature; however the phase does not exhibit thermal hysteresis and thus can be classified as a mesophase rather than a true crystalline state of matter.
Acknowledgements
We thank the Engineering and Physical Sciences Research Council (EPSRC) for support of this work via grant codes EP/K039660/1 and EP/M020584/1. Raw data are available upon request from the University of York data catalogue.
References
- M. Šepelj, A. Lesac, U. Baumeister, S. Diele, H. Loc Nguyen and D. W. Bruce, Intercalated liquid-crystalline phases formed by symmetric dimers with an α,ω-diiminoalkylene spacer, J. Mater. Chem., 2006, 17, 1154–1165 RSC
.
- V. P. Panov, M. Nagarai, J. K. Vij, Y. P. Panarin, A. Kohlmeier, M. G. Tamba, R. A. Lewis and G. H. Mehl, Spontaneous Periodic Deformations in Nonchiral Planar-Aligned Bimesogens with a Nematic-Nematic Transition and a Negative Elastic Constant, Phys. Rev. Lett., 2010, 105, 167701 CrossRef PubMed
.
- M. Cestari, S. Diez-Berart, D. A. Dunmur, A. Ferrarini, M. R. de la Fuente, D. J. B. Jackson, D. O. Lopez, G. R. Luckhurst, M. A. Perez-Jubindo, R. M. Richardson, J. Salud, B. A. Timimi and H. Zimmermann, Phase Behaviour and Properties of the Liquid-Crystal Dimer 1′′-7′′-bis(4-cyanobiphenyl4′-yl) heptane: A Twist-Bend Nematic Liquid Crystal, Phys. Rev. E: Stat., Nonlinear, Soft Matter Phys., 2011, 84, 031704 CrossRef CAS PubMed
.
- C. S. P. Tripathi, P. Losada-Pérez, C. Glorieux, A. Kohlmeier, M. G. Tamba, G. H. Mehl and J. Leys, Nematic-Nematic Phase Transition in the Liquid Crystal Dimer CB9CB and its Mixtures with 5CB: A High-Resolution Adiabatic Scanning Calorimetric Study, Phys. Rev. E: Stat., Nonlinear, Soft Matter Phys., 2011, 84, 041707 CrossRef PubMed
.
- V. P. Panov, R. Balachandran, M. Nagaraj, J. K. Vij, M. G. Tamba, A. Kohlmeier and G. H. Mehl, Microsecond Linear Optical Response in the Unusual Nematic Phase of Achiral Bimesogens, Appl. Phys. Lett., 2011, 99, 261903 CrossRef
.
- V. Borshch, Y.-K. Kim, J. Xiang, M. Gao, A. Jákli, V. P. Panov, J. K. Vij, C. T. Imrie, M. G. Tamba, G. H. Mehl and O. D. Lavrentovich, Nematic Twist-Bend Phase with Nanoscale Modulation of Molecular Orientation, Nat. Commun., 2013, 4, 2635 CAS
.
- D. Chen, J. H. Porda, J. B. Hooper, A. Klittnic, Y. Shen, M. R. Tuchband, E. Korblova, D. Bedrov, D. M. Walba, M. A. Glaser, J. E. Maclennan and N. A. Clark, A Twist-Bend Chiral Helix of 8 nm Pitch in a Nematic Liquid Crystal of Achiral Molecular Dimers, Proc. Natl. Acad. Sci. U. S. A., 2013, 110, 15931–15936 CrossRef CAS PubMed
.
- D. Chen, M. Nakata, R. Shao, M. R. Tuchband, M. Shuai, U. Baumeister, W. Weissflog, D. M. Walba, M. A. Glaser, J. E. Maclennan and N. A. Clark, Twist-Bend Heliconical Chiral Nematic Liquid Crystal Phase of an Achiral Rigid Bent-Core Mesogen, Phys. Rev. E: Stat., Nonlinear, Soft Matter Phys., 2014, 89, 022506 CrossRef PubMed
.
- R. J. Mandle, E. J. Davis, C. T. Archbold, S. J. Cowling and J. W. Goodby, Microscopy Studies of the Nematic NTB phase of 1,11-Di-(1′′-cyanobiphenyl-4-yl)undecane, J. Mater. Chem. C, 2014, 2, 556–566 RSC
.
- C. Meyer, G. R. Luckhurst and I. Dozov, The temperature dependence of the heliconical tilt angle in the twist-bend nematic phase of the odd dimer CB7CB, J. Mater. Chem. C, 2015, 3, 318–328 RSC
.
- Z. Zhang, V. P. Panov, M. Nagaraj, R. J. Mandle, J. W. Goodby, G. R. Luckhurst, J. C. Jones and H. F. Gleeson, Raman scattering studies of order parameters in liquid crystalline dimers exhibiting the nematic and twist-bend nematic phases, J. Mater. Chem. C, 2015, 3, 10007–10016 RSC
.
- J. P. Jokissari, G. R. Luckhurst, B. A. Timimi, J. Zhu and H. Zimmerman, Twist-bend nematic phase of the liquid crystal dimer CB7CB: orientational order and conical angle determined by 129Xe and 2H NMR spectroscopy, Liq. Cryst., 2015, 42, 708–721 Search PubMed
.
- C. Zhu, M. R. Tuchband, A. Young, M. Shuai, A. Scarrough, D. M. Walba, J. E. Maclennan, C. Wang, A. Hexemer and N. A. Clark, Resonant Carbon K-Edge Soft X-Ray Scattering from Lattice-Free Heliconical Molecular Ordering: Soft Dilative Elasticitiy of the Twist-Bend Liquid Crystal Phase, Phys. Rev. Lett., 2016, 116, 147803 CrossRef PubMed
.
- J. W. Emsley, M. Lelli, H. Joy, M.-G. Tamba and G. H. Mehl, Similarities and differences between molecular order in the nematic and twist-bend nematic phases of a symmetric liquid crystal dimer, Phys. Chem. Chem. Phys., 2016, 18, 9419–9430 RSC
.
- S. A. Pardaev, S. M. Shamid, M. G. Tamba, C. Welch, G. H. Mehl, J. T. Gleeson, D. W. Allender, J. V. Selinger, B. Ellman, A. Jakli and S. Sprunt, Second harmonic light scattering induced by defects in the twist-bend nematic phase of liquid crystal dimers, Soft Matter, 2016, 12, 4472–4482 RSC
.
- B. Robles-Hernandez, N. Sebastian, M. Rosario de la Fuente, D. O. Lopez, S. Diez-Berart, J. Salud, M. B. Ros, D. A. Dunmur, G. R. Luckhurst and B. A. Timimi, Twist, tilt, and orientational order at the nematic to twist-bend nematic phase transition of 1′′,9′′-bis(4-cyanobiphenyl-4′-yl) nonane: A dielectric, 2H NMR, and calorimetric study, Phys. Rev. E: Stat., Nonlinear, Soft Matter Phys., 2015, 92, 062505 CrossRef PubMed
.
- C. T. Archbold, J. L. Andrews, R. J. Mandle, S. J. Cowling and J. W. Goodby, Effect of the Linking Unit on the Twist-Bend Nematic Phase in Liquid Crystal Dimers: A Comparative Study of Two Homologous Series of Methylene and Ether Linked Dimers, Liq. Cryst., 2016 DOI:10.1080/02678292.2016.1240247
, in press.
- J. W. Emsley, M. Lelli, A. Lesage and G. R. Luckhurst, A Comparison of the Conformational Distributions of the Achiral Symmetric Liquid Crystal Dimer CB7CB in the Achiral Nematic and Chiral Twist-Bend Nematic Phases, J. Phys. Chem. B, 2013, 117, 6547–6557 CrossRef CAS PubMed
.
-
R. B. Meyer, Structural Problems in Liquid Crystal Physics, in Les Houches Summer School in Theoretical Physics, 1973. Molecular Fluids, ed. R. Balian and G. Weil, Gordon and Breach, New York, 1976, pp. 273–373 Search PubMed
.
- I. Dozov, On the Spontaneous Symmetry Breaking in the Mesophases of Achiral Banana-Shaped Molecules, Europhys. Lett., 2001, 56, 247–253 CrossRef CAS
.
- E. Gorecka, M. Salmonczyk, A. Zep, D. Pociecha, C. Welch, Z. Ahmed and G. H. Mehl, Do the Short Helices Exist in the Nematic TB Phase, Liq. Cryst., 2015, 42, 1–7 CrossRef CAS
.
- A. Hoffmann, A. G. Vanakaras, A. Kohlmeier, G. H. Mehl and D. J. Photinos, On The Structure of the NX Phase of Symmetric Dimers: Inferences from NMR, Soft Matter, 2015, 11, 850–855, 10.1039/c4sm02480j
.
- A. G. Vanakaras and D. J. Photinos, A molecular theory of nematic-nematic phase transitions in mesogenic dimers, Soft Matter, 2016, 12, 2208–2220, 10.1039/C5SM02505B
.
- T. Ivšić, M. Vinković, U. Baumeister, A. Mikleušević and A. Lesac, Towards understanding the NTB phase: a combined experimental, computational and spectroscopic study, RSC Adv., 2016, 6, 5000–5007, 10.1039/C5RA26078G
.
- C. T. Archbold, E. J. Davis, R. J. Mandle, S. J. Cowling and J. W. Goodby, Chiral dopants and the twist-bend nematic phase – induction of novel mesomorphic behaviour in an apolar bimesogen, Soft Mater., 2015, 11, 7547–7557 RSC
.
- A. A. Dawood, M. C. Grossel, G. R. Luckhurst, R. M. Richardson, B. A. Timimi, N. J. Wells and U. Z. Yousif, On the twist-bend nematic phase formed directly from the isotropic phase, Liq. Cryst., 2016, 43, 2–12 CrossRef CAS
.
- R. J. Mandle, E. J. Davis, S. A. Lobato, C. C. A. Vol, S. J. Cowling and J. W. Goodby, Characterisation of an Unsymmetrical, Ether-linked, Fluorinated Bimesogen Exhibiting a New Polymorphism Containing the NTB or ‘Twist-Bend’ Phase, Phys. Chem. Chem. Phys., 2014, 16, 6907–6915 RSC
.
- R. J. Mandle, C. C. A. Voll, D. J. Lewis and J. W. Goodby, Etheric Bimesogens and the Twist-Bend Nematic Phase, Liq. Cryst., 2015, 43, 13–21, DOI:10.1080/02678292.2015.1091095
.
- S. M. Jansze, A. Martínez-Felipe, J. D. M. Storey, A. T. M. Marcelis and C. T. Imrie, A Twist-Bend Nematic Phase Driven by Hydrogen Bonding, Angew. Chem., Int. Ed., 2015, 54, 643–646 CAS
.
- D. A. Paterson, A. Martinez-Felipe, S. M. Jansze, A. T. M. Marcelis, J. M. D. Storey and C. T. Imrie, New insights into the liquid crystal behaviour of hydrogen-bonded mixtures provided by temperature-dependent FTIR spectroscopy, Liq. Cryst., 2015, 42, 928–939 CAS
.
- A. Martinez-Felipe and C. T. Imrie, The role of hydrogen bonding in the phase behaviour of supramolecular liquid crystal dimers, J. Mol. Struct., 2015, 1100, 429–437 CrossRef CAS
.
- Y. Wang, G. Singh, D. M. Agra-Kooijman, M. Gao, H. K. Bisoyi, C. Xue, M. R. Fisch, S. Kumar and Q. Li, Room temperature heliconical twist-bend nematic liquid crystal, CrystEngComm, 2015, 1, 2778–2782 RSC
.
- R. J. Mandle and J. W. Goodby, A Liquid Crystalline Oligomer Exhibiting Nematic and Twist-Bend Nematic Mesophases, ChemPhysChem, 2016, 7, 967–970, DOI:10.1002/cphc.201600038
.
- G. Ungar, V. Percec and M. Zuber, Liquid crystalline polyethers based on conformational isomerism. 20. Nematic-nematic transition in polyethers and copolyethers based on 1-(4-hydroxyphenyl)2-(2-R-4-hydroxyphenyl)ethane with R = fluoro, chloro and methyl and flexible spacers containing an odd number of methylene units, Macromolecules, 1992, 25, 75–80 CrossRef CAS
.
- R. J. Mandle, The dependency of twist-bend nematic liquid-crystals on molecular structure: a progression from dimers to trimers, oligomers and polymers, Soft Matter, 2016, 12, 7883–7901 RSC
.
- E. Gorecka, N. Vaupotič, A. Zep, D. Pochiecha, J. Yoshioka, J. Yamamoto and H. Takazoe, A Twist-Bend Nematic (NTB) Phase of Chiral Materials, Angew. Chem., Int. Ed., 2015, 54, 10155–10159 CrossRef CAS PubMed
.
- D. A. Paterson, J. Xiang, G. Singh, R. Walker, D. M. Agra-Kooijman, A. Martinez-Felipe, M. Gao, J. M. D. Storey, S. Kumar, O. D. Larentovich and C. T. Imrie, J. Am. Chem. Soc., 2016, 138, 5283–5289 CrossRef CAS PubMed
.
- R. J. Mandle, E. J. Davis, C. C. A. Voll, C. T. Archbold, J. W. Goodby and S. J. Cowling, The Relationship between Molecular Structure and the Incidence of the NTB Phase, Liq. Cryst., 2015, 42, 688–703, DOI:10.1080/02678292.2014.974698
.
- R. J. Mandle, E. J. Davis, C. T. Archbold, C. C. A. Voll, J. L. Andrews, S. J. Cowling and J. W. Goodby, Apolar Bimesogens and the Incidence of the Twist-Bend Nematic Phase, Chem. – Eur. J., 2015, 21, 8158–8167 CrossRef CAS PubMed
.
- Z. Ahmed, C. Welch and G. H. Mehl, The design and investigation of the self-assembly of dimers with two nematic phases, RSC Adv., 2015, 5, 93513–93521 RSC
.
- R. J. Mandle and J. W. Goodby, Progression from Nano to Macro Science in Soft Matter Systems: Dimers to Trimers and Oligomers in Twist-Bend Liquid Crystals, RSC Adv., 2016, 6, 34885–34893 RSC
.
- R. J. Mandle and J. W. Goodby, A Twist-bend Nematic to an Intercalated, Anticlinic, Biaxial Phase Transition in Liquid Bimesogens, Soft Matter, 2016, 12, 1436–1443 RSC
.
- R. J. Mandle and J. W. Goodby, Dependence of Mesomorphic Behaviour of Methylene-Linked Dimers and the Stability of the NTB/NX Phase upon Choice of Mesogenic Units and Terminal Chain Length, Chem. – Eur. J., 2016, 22, 9366–9374 CrossRef CAS PubMed
.
- A. Zep, S. Aya, K. Aihara, K. Ema, D. Pociecha, K. Madrak, P. Bernatowicz, H. Takezoe and E. Gorecka, Multiple nematic phases observed in chiral mesogenic dimers, J. Mater. Chem. C, 2013, 1, 46–49 RSC
.
- J. W. Emsley, P. Lesot, G. R. Luckhurst, A. Meddour and D. Merlet, Chiral solutes can seed the formation of enantiomorphic domains in a twist-bend nematic liquid crystal, Phys. Rev. E: Stat., Nonlinear, Soft Matter Phys., 2013, 87, 040501 CrossRef PubMed
.
- J. W. Emsley, M. Lelli, A. Lesage and G. R. Luckhurst, A Comparison of the Conformational Distributions of the Achiral Symmetric Liquid Crystal Dimer CB7CB in the Achiral Nematic and Chiral Twist-Bend Nematic Phases, J. Phys. Chem. B, 2013, 117, 6547–6557 CrossRef CAS PubMed
.
- V. Avetisov and V. Goldanskii, Mirror symmetry breaking at the molecular level, Proc. Natl. Acad. Sci. U. S. A., 1996, 93, 11435–11442 CrossRef CAS
.
- S. J. Sowerby, W. H. Heckl and G. B. Petersen, Chiral Symmetry Breaking During the Self-Assemly of Monolayers from Achiral Purine Molecules, J. Mol. Evol., 1996, 43, 419–424 CrossRef CAS PubMed
.
- C. Viedma, Chiral Symmetry Breaking and Complete Chiral Purity by Thermodynamic-Kinetic Feedback Near Equilibrium: Implications for the Origin of Biochirality, Astrobiology, 2007, 7, 312–319 CrossRef CAS PubMed
.
- G. Tschierske and G. Ungar, Mirror Symmetry Breaking by Chirality Synchronisation in Liquids and Liquid Crystals of Achiral Molecules, ChemPhysChem, 2016, 17, 9–26 CrossRef PubMed
.
- M. Alaasar, M. Prehm, Y. Cao, F. Liu and C. Tschierske, Spontaneous Mirror Symmetry Breaking in Isotropic Liquid Phases of Photoisomerizable Achiral Molecules, Angew. Chem., Int. Ed., 2015, 55, 312–316 CrossRef PubMed
.
- I. Lelidis and G. Barbero, Nematic phases with spontaneous splay–bend deformation: standard elastic description, Liq. Cryst., 2016, 43, 208–215 CrossRef CAS
.
- J. Xiang, Y. Li, Q. Li, D. A. Paterson, J. M. D. Storey, C. T. Imrie and O. D. Lavrentovich, Electrically Tunable Selective Reflection of Light from Ultraviolet to Visible and Infrared by Heliconical Cholesterics, Adv. Mater., 2015, 27, 3014–3018 CrossRef CAS PubMed
.
- Y. Wang, Z. Zheng, H. K. Bisovi, K. G. Gutierrez-Cuevas, L. Wang, R. S. Zola and Q. Li, Thermally reversible full color selective reflection in a self-organized helical superstructure enabled by a bent-core oligomesogen exhibiting a twist-bend nematic phase, Mater. Horiz., 2016, 3, 442–446 RSC
.
-
M. J. Frisch, G. W. Trucks, H. B. Schlegel, G. E. Scuseria, M. A. Robb, J. R. Cheeseman, G. Scalmani, V. Barone, B. Mennucci, G. A. Petersson, H. Nakatsuji, M. Caricato, X. Li, H. P. Hratchian, A. F. Izmaylov, J. Bloino, G. Zheng, J. L. Sonnenberg, M. Hada, M. Ehara, K. Toyota, R. Fukuda, J. Hasegawa, M. Ishida, T. Nakajima, Y. Honda, O. Kitao, H. Nakai, T. Vreven, J. A. Montgomery Jr., J. E. Peralta, F. Ogliaro, M. Bearpark, J. J. Heyd, E. Brothers, K. N. Kudin, V. N. Staroverov, R. Kobayashi, J. Normand, K. Raghavachari, A. Rendell, J. C. Burant, S. S. Iyengar, J. Tomasi, M. Cossi, N. Rega, J. M. Millam, M. Klene, J. E. Knox, J. B. Cross, V. Bakken, C. Adamo, J. Jaramillo, R. Gomperts, R. E. Stratmann, O. Yazyev, A. J. Austin, R. Cammi, C. Pomelli, J. W. Ochterski, R. L. Martin, K. Morokuma, V. G. Zakrzewski, G. A. Voth, P. Salvador, J. J. Dannenberg, S. Dapprich, A. D. Daniels, Ö. Farkas, J. B. Foresman, J. V. Ortiz, J. Cioslowski and D. J. Fox, Gaussian 09, Revision E.01, Gaussian, Inc., Wallingford CT, 2009 Search PubMed
.
- M. Tarini, P. Cignoni and C. Montani, Ambient Occlusion and Edge Cueing for Enhancing Real Time Molecular Visualization, IEEE Trans. Vis. Comput. Graph., 2006, 12, 1237–1244 CrossRef PubMed
.
- P. Davidson, D. Petermann and A. Levelut, The Measurement of the Nematic Order Parameter by X-ray Scattering Reconsidered, J. Phys. II, 1995, 5, 113–131 CrossRef CAS
.
- G. W. Gray and J. E. Lydon, New Type of Smectic Mesophase?, Nature, 1974, 252, 221–222 CrossRef CAS
.
- M. Walker and M. R. Wilson, Simulation insights into the role of antiparallel molecular association in the formation of smectic A phases, Soft Matter, 2016 10.1039/C6SM01920J
, in press.
Footnote |
† Electronic supplementary information (ESI) available. See DOI: 10.1039/c6ce02123a |
|
This journal is © The Royal Society of Chemistry 2016 |
Click here to see how this site uses Cookies. View our privacy policy here.