DOI:
10.1039/C6CE00240D
(Paper)
CrystEngComm, 2016,
18, 4971-4976
Conformational changes in Cmethyl-resorcinarene pyridine N-oxide inclusion complexes in the solid state†
Received
29th January 2016
, Accepted 26th February 2016
First published on 26th February 2016
Abstract
Aromatic N-oxides interact with Cmethyl-resorcinarene resulting in marked changes in the conformation of the host resorcinarene. In the solid state, 2- and 3-methylpyridine N-oxides form pseudo-capsular 2
:
2 endo host–guest complexes with Cmethyl-resorcinarene stabilized by C–H⋯π interactions. The Cmethyl-resorcinarene·2-methylpyridine N-oxide complex has a C4v crown conformation, while the Cmethyl-resorcinarene·3-methylpyridine N-oxide complex has a slightly open C2v boat conformation. On the contrary, other para-substituted and benzo-fused pyridine N-oxides form only exo complexes with Cmethyl-resorcinarene. In the exo complexes, the asymmetry of the guest, conformational flexibility and preferred inter-host hydrogen bonding of Cmethyl-resorcinarenes exclude endo complexation. All the exo complexes form robust 1-D hydrogen bonded chains between the host hydroxyl groups assisted by the guest N–O groups, resulting in a C2v boat conformation.
Introduction
With flexible host molecules, several factors such as substituents, size and electronic properties of the guest, solvents, temperature or even reaction conditions can induce conformational changes in the host.1 The understanding of the interplay of host–guest interactions, both in the solid state and in solution, is a very important research area in supramolecular chemistry due to its major impact on the potential applications of host–guest systems as functional materials.2 Many host and receptor molecules adopt specific geometries and conformations. Amongst these compounds are resorcinarenes and pyrogallarenes which natively occur in the crown C4v conformation, and this favourable conformation allows them to act as hosts for a variety of guest molecules.2 The versatility of these host systems originates from the synthetically easy modifications either at the upper or lower rim of these macrocycles.2 However, it is known that core (unsubstituted) resorcinarene conformations are quite easily modulated by the reaction conditions when the host is synthesized. The most common resorcinarene and pyrogallarene conformations are crown, boat, chair, diamond and saddle.3 Other unusual conformations such as the rarely observed rcct-diamond Cmethyl-resorcinarene,3 the rcct-boat Cmethyl-2-nitroresorcinarene4 and the rcct-crown C4t-pyrogallarene (4t = tert-butyl)5 have been reported. In the C4v conformation, intramolecular circular hydrogen bonds (HB) between adjacent phenolic hydroxyl groups preserve the crown structure.5 In this conformation, the π-rich cavity is suitable for inclusion of spherical and planar guests such as ammonium and phosphonium cations, the main complexation force being the cation⋯π interactions.6 With resorcinarenes, these cationic guests play a key role in numerous solid-state architectures such as open inclusion complexes,7 dimers,8 hexamers,9 and tubular assemblies.10 Though inclusion complexes of resorcinarenes with cationic compounds are prevalent, there are several reports of complexes with neutral N-heteroaromatic five- and six-membered planar guests as well.11N-Aromatic compounds such as 2,2′- and 4,4′-bipyridines, due to their size and strong hydrogen bond acceptor properties, have been particularly effective in inducing conformational changes in resorcinarene complexes.12 A Cambridge Structural Database (CSD)13 search using Cmethyl-resorcinarene 1 as the host revealed 34 crystal structures with 4,4′-bipyridine as the guest molecule.14,12a–c
Aromatic N-oxides are well-known synthetic intermediates for functionalization of the pyridine ring in organic synthesis,15 and as ligands in supramolecular chemistry.16 The oxygen in the N-oxide group can exhibit hyper-dentate coordination behaviour with metal cations, resulting in interesting supramolecular architectures.16 Most recently, pyridine N-oxides (PyNOs) have been shown to be excellent halogen bond acceptors and thus form very strong halogen bonds.17 The N-oxide, viz.+N–O−, group makes the aromatic ring electron deficient but the electron deficiency can be modulated by the substituents on the aromatic ring, and due to this, aromatic N-oxides can act as guest molecules with calixarenes and cavitands.18 Recently, we have reported dimeric pseudo-capsular assemblies with aromatic N-oxides using Cethyl-2-methylresorcinarene as the host.19 The C–H⋯π and π⋯π interactions20 have proven to be the governing factors in the recognition of electron-deficient N-oxides by π-rich host systems.
In addition, halogen bond breaking using Cethyl-2-methylresorcinarene in halo-substituted pyridine N-oxide systems was an unexpected result of the conformational change in the host.21 While our previous work has focused on Cethyl-2-methylresorcinarene,19,21 there exists one study with 4,4′-bipyridine N,N′-dioxide and Cmethyl-resorcinarene as the host.22 In this work, we report solid-state complexes with Cmethyl-resorcinarene 1 as the host and seven PyNOs (Fig. 1) as the guests, and the conformational behaviour of the host upon complexation.
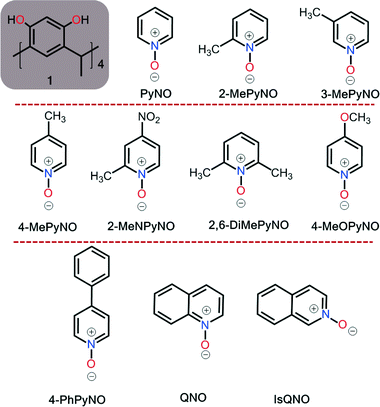 |
| Fig. 1 The chemical structures of Cmethyl-resorcinarene (1), pyridine N-oxide (PyNO), 2-methylpyridine N-oxide (2-MePyNO), 3-methylpyridine N-oxide (3-MePyNO), 4-methylpyridine N-oxide (4-MePyNO), 2-methyl-4-nitropyridine N-oxide (2-MeNPyNO), 2,6-dimethylpyridine N-oxide (2,6-DiMePyNO), 4-methoxypyridine N-oxide (4-MeOPyNO), 4-phenylpyridine N-oxide (4-PhPyNO), quinoline N-oxide (QNO), and isoquinoline N-oxide (IsQNO). | |
Results and discussion
X-ray crystallography
Single crystals of the complexes were obtained from slow evaporation of the methanolic solution of a 1
:
1 mixture of the host and guest molecules. Attempts to crystallize PyNO, 2,6-DiMePyNO and QNO with 1 were unsuccessful. In the endo complexes, the N-oxide oxygen atoms point away from the cavity (Fig. 2). Complexes of 2-MePyNO and 3-MePyNO with host 1 resulted in mixed endo/exo complexes, 2-MePyNO·1 and 3-MePyNO·1 (Fig. 2), which are remarkably similar to our previous results with Cethyl-2-methylresorcinarene.21 Complex 2-MePyNO·1 [formally 1
:
(2-MePyNO)endo
:
3(2-MePyNO)exo] can be described as a discrete dimeric pseudo-capsular 2
:
2 complex which is then hydrogen bonded to six exo-cavity 2-MePyNO molecules, as shown in Fig. 2b. In complex 2-MePyNO·1, host 1 retains the crown conformation (centroid-to-centroid distances being nearly equal, ca. 6.8/7.0 Å, Fig. 3), encapsulating one 2-MePyNO molecule. The guest, 2-MePyNO, resides 3.2 Å from the centroid of the lower rim carbon atoms (calculated from the PyNO benzene ring carbon deepest in the cavity). The hydrogen atoms para to the N–O and –CH3 groups of 2-MePyNO manifest C–H⋯π interactions with the aromatic rings of host 1 with distances ranging between 2.7 Å and 3.1 Å (Fig. 3a). Complex 3-MePyNO·1 [formally 1
:
(3-MePyNO)endo
:
(3-MePyNO)exo] forms a similar dimeric pseudo-capsular 2
:
2 complex to 2-MePyNO (Fig. 2c). These dimers are hydrogen-bonded to each other by bridging exo3-MePyNO molecules as shown in Fig. 2d. In complex 3-MePyNO·1, the cavity of host 1 is elongated so that the centroid-to-centroid distances are 5.8 and 7.5 Å to accommodate the slightly bigger 3-MePyNO molecule, as shown in Fig. 3d. The endo guest in 3-MePyNO·1 is situated 3.2 Å from the centroid of the lower rim carbon atoms, exactly the same distance as that in 2-MePyNO·1 and previously studied 3-MePyNO·Cethyl-2-methylresorcinarene.21 In 3-MePyNO·1, the guest is situated near the center of the cavity between the benzene rings with no apparent aromatic π⋯π interaction, but manifesting a weak methyl C–H⋯O hydrogen bond to the host O–H group (C–H⋯O distance of 2.51 Å) and C–H⋯π interactions from the hydrogen atoms meta to the N–O group (Fig. 3c). These C–H⋯π distances range from 2.8 Å to 2.9 Å. The increased conformational flexibility of host 1 and the larger guest size in the endo complexation via the C–H⋯π interactions between the host and the guest distort the parent C4v crown conformation to a nearly ideal C2v boat conformation (Fig. 3d). With our previous work on resorcinarene endo PyNO complexes,21 the PyNOs located deep in the cavity, viz. <2.9 Å from the centroid of the lower rim carbon atoms, usually show short C–H⋯π(centroid) contacts. However in 3-MePyNO·1, where the 3-MePyNO guest is situated higher in the cavity (3.2 Å), the shortest C–H⋯π(centroid) distance of ca. 2.5 Å was observed. Comparing the host–guest chemistry of 2-MePyNO·1 and 3-MePyNO·1 with that of Cethyl-2-methylresorcinarene,21 both host systems show a similar encapsulation profile to 2-MePyNO and 3-MePyNO. In general, the formation of endo complexes with host 1 is considerably more difficult than that with conformationally more rigid host molecules due to the stronger influence of the intermolecular hydrogen bonding between the hosts, which can lead to the conformational change and subsequent formation of host-to-host 1-D, 2-D or 3-D networks.
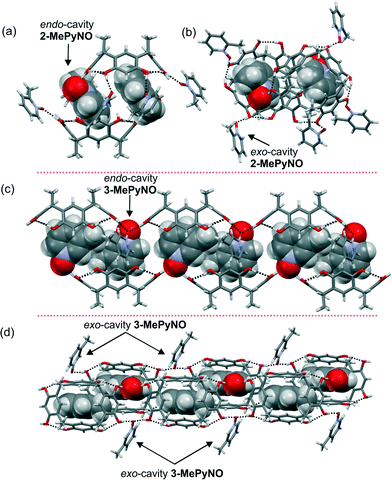 |
| Fig. 2 (a) Side view of the discrete dimeric structure of 2-MePyNO·1, and (b) top view showing exo-cavity HB monodentate decorated 2-MePyNO with host 1. (c) Section of the 1-D polymeric crystal packing in 3-MePyNO·1 showing endo3-MePyNO as a bidentate HB acceptor, and the respective (d) side view showing the exo-cavity HB bidentate 3-MePyNO molecules. | |
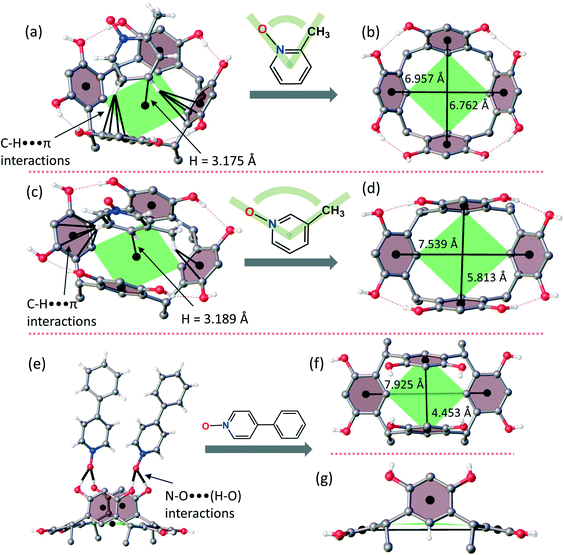 |
| Fig. 3 (a) C–H⋯π interactions in 2-MePyNO·1. (b) The cavity of host 1 in 2-MePyNO·1 highlighting the flexibility. (c) C–H⋯π interactions in 3-MePyNO·1. (d) The cavity of host 1 in 3-MePyNO·1 highlighting the flexibility. (e) Complex 4-PhPyNO·1 displaying bidentate bridging 4-PhPyNO molecules through (N–O)⋯(H–O) interactions. (f) Top view of the C2v boat conformer in complex 4-PhPyNO·1. (g) Side view of the C2v boat conformer in complex 4-PhPyNO·1. Black broken lines in Fig. 3e represent (N–O)⋯(H–O) interactions. | |
The conformational flexibility of resorcinarenes is due to their lower rim R chains. The methyl chain is smaller, thus allowing more different conformations for the resorcinarene skeleton. The ethyl chain is sterically more bulky and thus results in conformationally less flexible resorcinarenes.2,5 Sterically suitable PyNOs like 2-MePyNO and 3-MePyNO which do form endo complexes with conformationally flexible host systems are also proven to form endo complexes with more rigid systems; this we have recently shown with Cethyl-2-methylresorcinarene.21 The question arises if the larger PyNOs21 would also behave similarly to the more flexible host 1. As such, larger PyNOs were utilized to probe their behaviour.
Complexes 4-MePyNO·1 [formally 1
:
2(4-MePyNO)exo
:
CH3OH], 4-MeOPyNO·1 [formally 1
:
2(4-MeOPyNO)exo], 2-MeNPyNO·1 [formally 1
:
2(2-MeNPyNO)exo
:
4CH3OH
:
H2O], 4-PhPyNO·1 [formally 1
:
2(4-PhPyNO)exo], and IsQNO·1 [formally 1
:
2(IsQNO)exo
:
4CH3OH
:
4H2O] manifest only exo complexes with a prominent feature being the formation of 1-D tubular hydrogen-bonded chains with a C2v boat conformation of the host (Fig. 3f and g). The asymmetric structure and larger size of these PyNOs prevent the formation of endo complexes and the ease of intermolecular hydrogen bond formation leads to the formation of host-to-host 1-D tubular chains in all complexes, as shown in Fig. 4. During crystallization, the host interactions with themselves, the guest and the solvent molecules (methanol and water) change the conformation from the C4v crown to C2v boat and provide possibilities for better intermolecular hydrogen bonding between the hydroxyl groups of the hosts. Two types of 1-D chains were observed, only PyNOs (type 1) and a combination of PyNOs and solvent molecules (type 2). The type 1 situation appears in complexes 4-MeOPyNO·1 and 4-PhPyNO·1, while type 2 is observed in complexes 2-MeNPyNO·1 and IsQNO·1 (Fig. 5 and ESI,† Fig. S6).
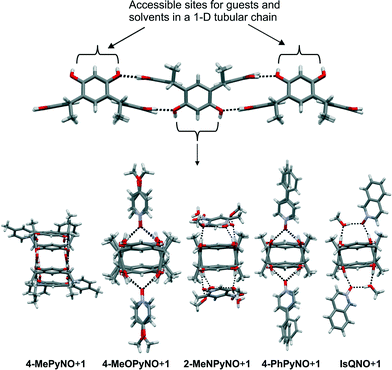 |
| Fig. 4 Repeating units of the crystal packing viewed along the 1-D chains of complexes in 4-MePyNO·1, 4-MeOPyNO·1, 2-MeNPyNO·1, 4-PhPyNO·1, and IsQNO·1 (left to right). | |
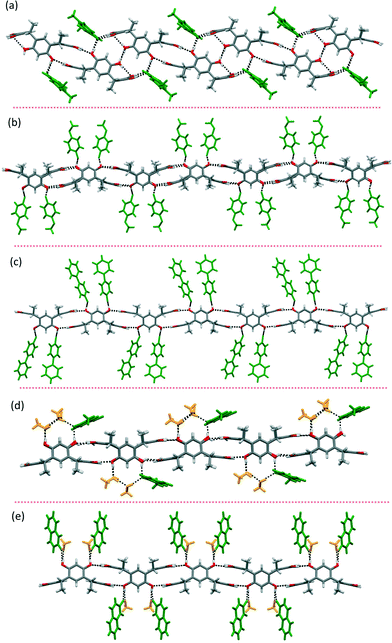 |
| Fig. 5 Side view of the 1-D HB chains of host 1. (a) 4-MePyNO·1, (b) 4-MeOPyNO·1, (c) 4-PhPyNO·1, (d) 2-MeNPyNO·1 and (e) IsQNO·1. The PyNO molecules are shown in green. The solvent molecules are shown in orange in figures (d) and (e). | |
In complex 4-MePyNO·1, the 4-MePyNO molecules bridge the hosts via the N-oxide oxygen atom (O–H)host⋯(O–N)⋯(O–H)host with distances of 2.60 and 2.67 Å, generating a zigzag 1-D tubular chain (Fig. 5a). A closer look at the 3-D crystal packing reveals that the 4-MePyNO molecules of the 1-D polymeric chain are additionally stabilised by π⋯π interactions at centroid-to-centroid distances of ca. 3.9 Å (ESI,† Fig. S1b). Both 4-MeOPyNO·1 and 4-PhPyNO·1 manifest a regular 1-D chain joined by the bidentate N-oxide oxygen atom with (O–H)host⋯(O–N)⋯(O–H)host distances of 2.64 Å (4-MeOPyNO·1), and ca. 2.66–2.72 Å (4-PhPyNO·1) as shown in Fig. 5b and c. Further analysis of the crystal packing shows that the 1-D chains in 4-MeOPyNO·1 and 4-PhPyNO·1 interdigitate to form 2-D supramolecular sheets (see the ESI,† Fig. S2). These interdigitated 1-D chains are stabilized by C–H⋯π and π⋯π interactions. During interdigitation, the aromatic rings of host 1, and the hydrogen atoms of the methoxy group in 4-MeOPyNO, and the para hydrogen atoms of 4-PhPyNO have short C–H⋯π contacts with distances of ca. 2.8 Å and 2.7 Å, respectively (see the ESI,† Fig. S2 and S3).
The host molecules in complex 2-MeNPyNO·1 also form a 1-D hydrogen bonded chain as shown in Fig. 5d. However, the hydrogen bonding via the hydroxyl groups of the host and the crystal packing differ due to interactions with solvent molecules and a second 2-MeNPyNO molecule in the asymmetric unit. Two methanol molecules, a water molecule and a 2-MeNPyNO molecule hydrogen bond to three host hydroxyl groups of the 1-D tubular chain in a continuous fashion (O–H)host⋯(O–H)CH3OH⋯(O–H)H2O⋯(O–H)CH3OH⋯(O–N)PyNO (see the ESI,† Fig. S4a). Also, the second 2-MeNPyNO molecule hydrogen bonds to water and resides close to the host aromatic ring at centroid-to-centroid distances of ca. 3.7 Å. The crystal packing of 2-MeNPyNO·1 shows that the 1-D chains are connected by two methanol molecules to give a 2-D sheet motif (see the ESI,† Fig. S4c).
The mode of action of the host 1 hydroxyl groups in the 1-D polymeric chain of complex IsQNO·1 (Fig. 5e) is different from other complexes. The host hydroxyl groups are connected by methanol and IsQNO molecules through hydrogen bonds, (O–H)host⋯(O–H)CH3OH⋯(O–N)IsQNO⋯(O–H)host with distances of ca. 2.61, 2.64 and 2.63 Å, respectively. Once again, the 1-D chains interdigitate by extensive C–H⋯π and π⋯π interactions between IsQNO molecules to give a 2-D sheet (see the ESI,† Fig. S5) motif. During interdigitation, the aromatic rings of IsQNO molecules have centroid-to-centroid distances of ca. 3.9 Å, and C–H⋯π contacts with the host aromatic rings at distances of ca. 2.8 Å.
Solution studies
Solution studies between host 1 and PyNO, 2-MePyNO, 3-MePyNO, 4-MePyNO, 2-MeNPyNO, 2,6-DiMePyNO, 4-MeOPyNO, 4-PhPyNO, QNO and IsQNO as guests were conducted via1H NMR experiments in CD3OD at 293 K. In these experiments, the host and guests were mixed in a 1
:
1 (6.6 mM on both molecules) ratio in CD3OD; the 1H NMR spectra were measured and the results were compared with the free host (6.6 mM) and free guests (6.6 mM).
Complexation-induced shielding of the guest proton resonances was observed in all cases except with 4-PhPyNO. The shielding effects of the aromatic rings of the bowl-shaped host cavity upon addition of the guest are responsible for this upfield shift and indicate that guest exchange is fast on the NMR time scale. Taking the 1
:
1 mixture between 1 and 3-MePyNO as an example (Fig. 6, I), the aromatic protons (g, f) are the most shielded (0.16 ppm and 0.17 ppm). These shift changes confirm the orientation of the guest deep in the host cavity, supporting the X-ray crystal structure (Fig. 3). Analysis of the 1
:
1 mixture between 1 and QNO (Fig. 6, II) reveals the aromatic protons (f, g) to be the most shielded (0.14–0.15 ppm), once again suggesting the localization of QNO within the host cavity. The analyses of the 1H NMR results between the host and the other guests (PyNO, 2-MePyNO, 4-MePyNO, 2-MeNPyNO, 2,6-DiMePyNO, 4-MeOPyNO, and IsQNO) also indicate the localization of the guest in the host cavity (Fig. S7–S16†). No shift changes were observed with 4-PhPyNO, implying that in solution no host–guest complex is formed (Fig. S14†).
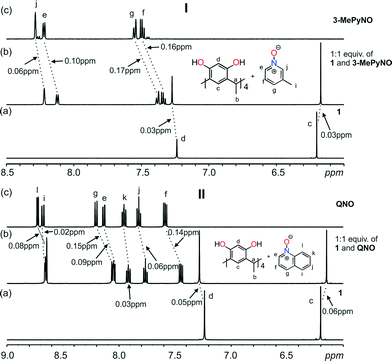 |
| Fig. 6 Selected region of the 1H NMR (CD3OD, 293 K) of: (I) a mixture of 3-MePyNO and 1, (a) 1 (6.6 mM), (b) 1+3-MePyNO (1 : 1), (c) 3-MePyNO (6.6 mM) and (II) a mixture of QNO and 1, (a) 1 (6.6 mM), (b) 1+QNO (1 : 1), (c) QNO (6.6 mM). The shift changes in the guest and host signals of the complexes in ppm are highlighted. | |
Variable temperature (233–333 K) 1H NMR experiments were done to probe the conformational changes of host 1 in the presence of the different guests (Fig. S17–S18†). No obvious changes in the host 1 signals were observed, implying that the conformational changes observed in the solid state with the N-oxide guests are caused by multiple intermolecular interactions existing only in the solid state.
Conclusions
The X-ray structures of seven PyNO complexes led to the conclusion that there is either endo complexation via C–H⋯π interactions or exo complexation via (N–O)⋯(H–O)host hydrogen bonds between the aromatic N-oxides and Cmethyl-resorcinarene. Due to the rarity of Cmethyl-resorcinarene·pyridine N-oxide complexes, the endo host–guest complexes 2-MePyNO·1 and 3-MePyNO·1 were compared with our previous results with Cethyl-2-methylresorcinarene,21 which allowed us to probe factors such as the conformational flexibility of the cavity, the strength of C–H⋯π interactions and the localization of the guest molecule in the cavity. Despite the enhanced conformational flexibility, Cmethyl-resorcinarene forms a pseudo-capsular 2
:
2 endo complex with 2-MePyNO and 3-MePyNO stabilized by C–H⋯π interactions in the solid state. The larger overall size of 3-MePyNO forces the C4v crown conformation to open, very close to the ideal C2v boat conformation, but still having the guest inside the cavity. Additionally, the strong C–H⋯π interactions found in the endo complex 3-MePyNO·1 hint that the guest similar to 3-MePyNO with Cmethyl-resorcinarene could show interesting host–guest interactions. The larger guests 4-MePyNO, 2-MeNPyNO, 4-MeOPyNO, 4-PhPyNO and IsQNO do not form endo complexes, as the exo complexes are preferred due to the strong intermolecular hydrogen bonding between the host 1 molecules leading to 1-D chains. All exo complexes show very similar overall crystal packings. The complementary solution studies show that host–guest complexes between host 1 and all the N-oxide molecules, except 4-PhPyNO, are formed in solution. Furthermore, the variable temperature 1H NMR measurements proved that the conformational changes in host 1, observed in the solid state upon complexation, were not observed in solution.
Acknowledgements
The Academy of Finland (K. R.: grant no. 265328 and 263256; N. K. B.: grant no. 258653), the University of Jyväskylä and Aalto University are gratefully acknowledged for financial support.
Notes and references
-
J. W. Steed and P. A. Gale, Supramolecular Chemistry: From Molecules to Nanomaterials, 8 Volume Set, Wiley, 2012 Search PubMed.
-
W. Sliwa and C. Kozlowski, Calixarenes and Resorcinarenes, Wiley, 2009 Search PubMed.
- M. He, R. J. Johnson, J. O. Escobedo, P. A. Beck, K. K. Kim, N. N. St. Luce, C. J. Davis, P. T. Lewis, F. R. Fronczek, B. J. Melancon, A. A. Mrse, W. D. Treleaven and R. M. Strongin, J. Am. Chem. Soc., 2002, 124, 5000–5009 CrossRef CAS PubMed.
- N. K. Beyeh and K. Rissanen, Tetrahedron Lett., 2009, 50, 7369–7373 CrossRef CAS.
- P. Timmerman, W. Verboom and D. N. Reinhoudt, Tetrahedron, 1996, 52, 2663–2704 CrossRef CAS.
-
(a) J. L. Atwood and A. Szumna, J. Supramol. Chem., 2002, 2, 479–482 CrossRef CAS;
(b) N. K. Beyeh and K. Rissanen, Isr. J. Chem., 2011, 51, 769–780 CrossRef CAS;
(c) N. K. Beyeh, A. Valkonen and K. Rissanen, Supramol. Chem., 2009, 21, 142–148 CrossRef CAS;
(d) H. Mansikkamaki, M. Nissinen and K. Rissanen, CrystEngComm, 2005, 7, 519–526 RSC.
-
(a) N. K. Beyeh, D. P. Weimann, L. Kaufmann, C. A. Schalley and K. Rissanen, Chem. – Eur. J., 2012, 18, 5552–5557 CrossRef CAS PubMed;
(b) N. K. Beyeh, M. Göth, L. Kaufmann, C. A. Schalley and K. Rissanen, Eur. J. Org. Chem., 2014, 2014, 80–85 CrossRef.
-
(a) H. Mansikkamaki, C. A. Schalley, M. Nissinen and K. Rissanen, New J. Chem., 2005, 29, 116–127 RSC;
(b) M. Luostarinen, A. Åhman, M. Nissinen and K. Rissanen, Supramol. Chem., 2004, 16, 505–512 CrossRef CAS.
-
(a) N. K. Beyeh, M. Kogej, A. Åhman, K. Rissanen and C. A. Schalley, Angew. Chem., Int. Ed., 2006, 45, 5214–5218 CrossRef CAS PubMed;
(b) T. Gerkensmeier, W. Iwanek, C. Agena, R. Fröhlich, S. Kotila, C. Näther and J. Mattay, Eur. J. Org. Chem., 1999, 1999, 2257–2262 CrossRef;
(c) L. R. MacGillivray and J. L. Atwood, Nature, 1997, 389, 469–472 CrossRef CAS.
-
(a) H. Mansikkamäki, M. Nissinen and K. Rissanen, Angew. Chem., 2004, 116, 1263–1266 CrossRef;
(b) H. Mansikkamäki, S. Busi, M. Nissinen, A. Åhman and K. Rissanen, Chem. – Eur. J., 2006, 12, 4289–4296 CrossRef PubMed.
-
(a) M. Nissinen, E. Wegelius, D. Falabu and K. Rissanen, CrystEngComm, 2000, 2, 151–153 RSC;
(b) M. Nissinen and K. Rissanen, Supramol. Chem., 2003, 15, 581–590 CrossRef CAS.
-
(a) B.-Q. Ma and P. Coppens, Cryst. Growth Des., 2004, 4, 1377–1385 CrossRef CAS;
(b) B.-Q. Ma, Y. Zhang and P. Coppens, Cryst. Growth Des., 2001, 1, 271–275 CrossRef CAS;
(c) L. R. MacGillivray, P. R. Diamente, J. L. Reid and J. A. Ripmeester, Chem. Commun., 2000, 359–360 RSC;
(d) G. Ferguson, C. Glidewell, A. J. Lough, G. D. McManus and P. R. Meehan, J. Mater. Chem., 1998, 8, 2339–2345 RSC;
(e) P. Thuéry, M. Nierlich, Z. Asfari, J. Vicens, O. Morikawa and H. Konishi, Supramol. Chem., 2001, 13, 521–527 CrossRef;
(f) C. L. Raston and G. W. V. Cave, Chem. – Eur. J., 2004, 10, 279–282 CrossRef CAS PubMed;
(g) P. O. Brown, G. D. Enright and J. A. Ripmeester, CrystEngComm, 2006, 8, 381–383 RSC.
- CSD version 5.36 (November 2014), ConQuest Version 1.17; Search performed date, 17.12.2015. The CSD search was defined and limited to core Cmethyl-resorcinarene as the host molecule.
-
(a) P. O. Brown, G. D. Enright and J. A. Ripmeester, J. Supramol. Chem., 2002, 2, 497–500 CrossRef CAS;
(b) T. Friščić and L. R. MacGillivray, J. Organomet. Chem., 2003, 666, 43–48 CrossRef;
(c) B.-Q. Ma, Y. Zhang and P. Coppens, CrystEngComm, 2001, 3, 78–80 RSC;
(d) B.-Q. Ma, Y. Zhang and P. Coppens, J. Organomet. Chem., 2003, 68, 9467–9472 CrossRef CAS PubMed;
(e) B.-Q. Ma, Y. Zhang and P. Coppens, Cryst. Growth Des., 2002, 2, 7–13 CrossRef CAS;
(f) L. R. MacGillivray and J. L. Atwood, J. Am. Chem. Soc., 1997, 119, 6931–6932 CrossRef CAS;
(g) L. R. MacGillivray, K. T. Holman and J. L. Atwood, J. Supramol. Chem., 2001, 1, 125–130 CrossRef CAS;
(h) L. R. MacGillivray, G. S. Papaefstathiou, J. L. Reid and J. A. Ripmeester, Cryst. Growth Des., 2001, 1, 373–375 CrossRef CAS;
(i) L. R. MacGillivray, J. L. Reid and J. A. Ripmeester, Chem. Commun., 2001, 1034–1035 RSC;
(j) A. Nakamura, T. Sato and R. Kuroda, CrystEngComm, 2003, 5, 318–325 RSC;
(k) L. R. MacGillivray, J. L. Reid and J. A. Ripmeester, CrystEngComm, 1999, 1, 1–4 RSC;
(l) J. Zhang, M. Gembicky, M. Messerschmidt and P. Coppens, Chem. Commun., 2007, 2399–2401 RSC;
(m) Y. Zhang, C. D. Kim and P. Coppens, Chem. Commun., 2000, 2299–2300 RSC.
-
A. R. Katritzky, Handbook of heterocyclic chemistry, Pergamon Press, 1985 Search PubMed;
A. Albini, Heterocyclic N-oxides, Taylor & Francis, 1991 Search PubMed.
- R. Puttreddy and P. J. Steel, CrystEngComm, 2014, 16, 556–560 RSC (references therein).
- R. Puttreddy, O. Jurcek, S. Bhowmik, T. Makela and K. Rissanen, Chem. Commun., 2016, 52, 2338–2341 RSC.
-
(a) G. Zheng, Y.-Y. Li, H.-D. Guo, S.-Y. Song and H.-J. Zhang, Chem. Commun., 2008, 4918–4920 RSC;
(b) L. Adriaenssens and P. Ballester, Chem. Soc. Rev., 2013, 42, 3261–3277 RSC.
- N. K. Beyeh, R. Puttreddy and K. Rissanen, RSC Adv., 2015, 5, 30222–30226 RSC.
- C. Janiak, J. Chem. Soc., Dalton Trans., 2000, 3885–3896 RSC.
- R. Puttreddy, N. K. Beyeh and K. Rissanen, CrystEngComm, 2016, 18, 793–799 RSC.
-
Y. Zhang and P. Coppens, Private Commun., 2007 (CSD Code - IFOVIJ).
Footnote |
† Electronic supplementary information (ESI) available: X-ray crystallographic and NMR spectroscopic data. CCDC 1450582–1450588. For ESI and crystallographic data in CIF or other electronic format see DOI: 10.1039/c6ce00240d |
|
This journal is © The Royal Society of Chemistry 2016 |