DOI:
10.1039/C6CC07627K
(Communication)
Chem. Commun., 2016,
52, 12364-12367
Metal-free C–H thioarylation of arenes using sulfoxides: a direct, general diaryl sulfide synthesis†
Received
19th September 2016
, Accepted 27th September 2016
First published on 27th September 2016
Abstract
Metal-free C–H thioarylation of arenes and heteroarenes using methyl sulfoxides constitutes a general protocol for the synthesis of high value diaryl sulfides. The coupling of arenes and heteroarenes with in situ activated sulfoxides is regioselective, uses readily available starting materials, is operationally simple, and tolerates a wide range of functional groups.
Diaryl sulfides are prevalent in important and high value chemical structures such as biologically active compounds,1 organic materials2 and ligands3 that mediate an array of chemical transformations (Scheme 1A). In addition, they are precursors to other higher oxidation state sulfur containing compounds that are found in similarly important chemicals.4
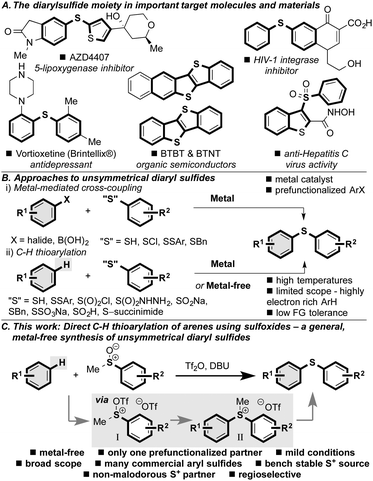 |
| Scheme 1 (A) The importance of unsymmetrical diaryl sulfides. (B) Conventional metal-mediated and metal-free approaches. (C) The metal-free C–H thioarylation of arenes provides convenient and general access to unsymmetrical diarylsulfides. Tf2O = trifluoromethanesulfonic anhydride, DBU = 1,8-diazabicycloundec-7-ene. | |
Due to the importance of unsymmetrical diaryl sulfides, their synthesis has garnered much attention. For example, in transition metal-mediated processes,5 using Pd,6 Ni,7 Rh,8 Cu,9 In,10 Fe11 and Co,12 the coupling of aryl halides (or pseudohalides) with various sulfur based partners has been described (Scheme 1Bi).13,14 In an attempt to improve reaction efficiency by abolishing the need for a prefunctionalized arene (e.g. aryl halide), C–H thioarylation mediated by transition metals has received significant attention (Scheme 1Bii).15 However, the use of metals raises issues of supply risk16 and contamination of products.17 In addition, these metal-catalyzed procedures for the synthesis of diaryl sulfides typically require elevated temperatures, that limit their general applicability. More recently, metal-free18 C–H thioarylation of highly electron rich arenes and heteroarenes using electrophilic sulfur reagents and precursors, including N-(thio)succinimides,19 sulfonyl hydrazines,20 sulfonyl chlorides,21 sodium sulfinates,22 thiols,23 disulfides,24 and others25 has emerged, and circumvents some of the problems associated with transition metal-mediated transformations (Scheme 1Bii). In these reactions the active sulfur electrophile, which is often formed in situ, is at the sulfide oxidation level and therefore has poor electrophilicity, limiting these methods to electron rich aryl coupling partners (typically indole or phenol/aniline derivatives) and the requirement for high temperatures.
Herein, we report the development of a simple, general, metal-free method for the synthesis of unsymmetrical diaryl sulfides that exploits a bench-stable, convenient sulfur electrophile, which has broad scope and high functional group tolerance (Scheme 1C). The method exploits the reactivity of activated sulfoxides, which we show to be excellent electrophiles, and as such allows the straightforward reaction to proceed under mild conditions with a variety of arene coupling partners in a highly regioselective manner.
Based on the seminal studies by Balenkova26 and recent work by us27 and others28 on the reactivity of sulfoxonium salts with a variety of nucleophiles,29 we postulated that activated aryl methyl sulfoxides (I) would serve as efficient sulfur based electrophiles for the C–H thioarylation of arenes to afford sulfonium salts II (Scheme 1C). The intermediates II could then be demethylated in situ to afford a straightforward, metal-free synthesis of unsymmetrical diaryl sulfides. Thus, we treated methyl phenyl sulfoxide 1a with trifluoromethanesulfonic anhydride, which formed the activated sulfoxide (cf.I). Addition of p-xylene 2a led to the formation of an isolable sulfonium salt (cf.II),30 that smoothly underwent demethylation with DBU to yield diaryl sulfide 3a in 91% isolated yield (Scheme 2).31
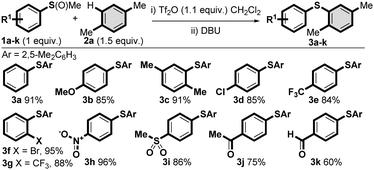 |
| Scheme 2 Scope of the methyl aryl sulfoxide coupling partner in the metal-free C–H thioarylation approach to diaryl sulfides. Isolated yields stated. | |
We furthered investigated the scope of the metal-free C–H thioarylation process with regard to the aryl methyl sulfoxide partner (Scheme 2). A range of methyl sulfoxides 1, commercially available or readily prepared from the corresponding commercial sulfides, were employed in the study. Electron-donating (3b and 3c) and electron-withdrawing (3d-k) substituents were well tolerated and diaryl sulfides were obtained in excellent isolated yields. More hindered ortho substituted sulfoxides (forming 3c, 3f and 3g), as well as a variety of important and/or functionalizable groups, such as methoxyl (3b), chloro (3d), trifluoromethyl (3e and 3g), bromo (3f), nitro (3h), sulfone (3i), ketone (3j) and aldehyde (3k) were amenable to the process. It is important to note that the presence of halides would typically be incompatible with metal-mediated diaryl sulfide syntheses.
Next we turned to investigating the scope with regards to the aryl–H partner 2 and found that the metal-free C–H thioarylation embraced a variety of aromatics with complete regioselectivity observed in all cases (Scheme 3). Less activated arenes, such as toluene (3l), iodobenzene (3m) and even benzene (3n) underwent smooth C–H thioarylation, in stark contrast to other metal-free C–H thioarylation approaches to diaryl sulfides. More hindered m-xylene (3o), naphthalene (3p) and 1-bromo naphthalene (3q) were well tolerated, as well as arenes containing oxygen substitution (3r-w and 3ab), including those with versatile bromo (3s and 3t), acetal (3t), alkenyl (3ab), ester (3u, 3w and 3ab) and aldehyde (3v) functionality. Heteroaromatics, such as indole (3x), dibenzothiophene (3y), benzothiophene (3z and 3aa), thiophene (3ac) and furan (3ad) were also successfully applied. We have used benzothiophene 3z in a formal synthesis of an anti-hepatitis-C drug candidate (see Scheme 1A for structure).32 Also of note, 3aa is a known precursor15a to BTBT organic semiconductors (see Scheme 1A for structure).
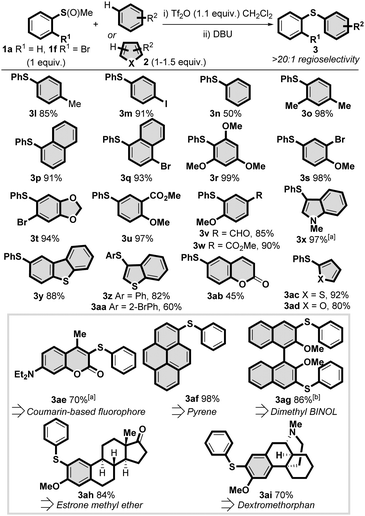 |
| Scheme 3 Scope of the arene and heteroarene coupling partner in the metal-free C–H thioarylation approach to diaryl sulfides and metal-free C–H thioarylation for scaffold construction and late-stage modification. Isolated yields stated. a TFAA used as activator. b 2 equiv. of 1a and 2.1 equiv. of Tf2O. | |
We further evaluated the efficacy of the process in the late-stage diversification of a range of molecular scaffolds. Aryl sulfide 3ae was formed from an established coumarin based fluorophore, useful in the study of biological systems.33 Pyrene, a common motif in dyes, also efficiently formed diaryl sulfide 3af, an intermediate in the synthesis of fluorescent chemosensors.34 Dimethyl BINOL, bearing the ubiquitous binaphthyl chiral scaffold, smoothly underwent a one-pot double C–H thioarylation with two equivalents of activated sulfoxide without reduction in efficiency (3ag). The metal-free protocol was applied to estrone methyl ether, an estrogenic hormone derivative, leading selectively to sulfide 3ah. In addition, dextromethorphan,35 a drug of the morphinan class commonly sold in over-the-counter cold and cough medicines, led to the corresponding diaryl sulfide 3ai in good yield.
These late stage modifications (3ae-ai), general scope of the sulfoxide 1 (3a-k) and aryl–H coupling partner 2 (3l-ad) underscore some key features of this metal-free C–H thioarylation protocol: (1) the reaction has broad functional group tolerance, including halides, methoxy, carbonyl derivatives, nitro, sulfone, and basic nitrogen atoms; (2) electron withdrawing groups on the aryl–H partner and electron neutral aryl–H partners are well tolerated in contrast to other metal-free protocols; (3) the reaction yields diaryl sulfides with complete regioselectivity; and (4) over C–H thioarylation is impossible due to intermediate sulfonium salt II being deactivated to further SEAr with another molecule of activated sulfoxide I. These facets are made possible by the superior reactivity of activated sulfoxides as sulfur electrophiles, meaning the reaction does not require harsh conditions and elevated temperatures.
The chemical union of two biologically active molecules is of significant interest to medicinal chemists.36 We reasoned that we could use our approach in an iterative C–H thioarylation to link two biologically active aryl–H partners via a sulfur atom (Scheme 4). In order to explore iterative C–H thioarylation we reacted estrone methyl ether 2ah with activated DMSO,26 which after dealkylation and oxidation gave estrone derived methyl sulfoxide 1ah in high yield over two steps (79%). The generated sulfoxide 1ah then served as the sulfur partner in the metal-free C–H thioarylation of another molecule of estrone methyl ether 2ah, giving dimeric estrone methyl ether 3aj in a convenient three-step, metal-free procedure from two non-prefunctionalized aryl–H coupling partners and DMSO37 as the sulfur source. This strategy would also be useful when the required methyl aryl sulfoxide 1 (or corresponding methyl sulfide) is not commercially available.38
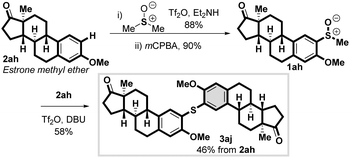 |
| Scheme 4 Metal-free, iterative C–H thioarylation in the synthesis of diaryl sulfides from the union of two aryl–H coupling partners and DMSO as the sulfur source. | |
In summary, we have developed a general, operationally simple metal-free synthesis of diaryl sulfides that proceeds under mild conditions. The C–H thioarylation process is made possible by the use of readily available sulfoxides that once activated, form sulfoxonium salts that serve as excellent sulfur electrophiles for coupling with a variety of aryl–H partners, including those that are not electronically biased, with universally high regioselectivity. The method allows the incorporation of a variety of functional groups in either coupling partner, and as such is suitable for the construction of complex diaryl sulfides that have broad applications. In addition, we have described an iterative C–H thioarylation approach to complex diaryl sulfides utilising two aryl–H partners and DMSO as the sulfur source.
We thank EPSRC (Postdoctoral Fellowship to J. A. F.-S.; Established Career Fellowship to D. J. P.) and The University of Manchester (Lectureship to A. P. P.).
Notes and references
- For recent examples, see:
(a) H. Wu, S. Bock, M. Snitko, T. Berger, T. Weidner, S. Holloway, M. Kanitz, W. E. Diederich, H. Steuber, C. Walter, D. Hofmann, B. Weißbrich, R. Spannaus, E. G. Acosta, R. Bartenschlager, B. Engels, T. Schirmeister and J. Bodemb, Antimicrob. Agents Chemother., 2015, 59, 1100 CrossRef PubMed;
(b) Y. Mao, L. Jiang, T. Chen, H. He, G. Liu and H. Wang, Synthesis, 2015, 1387 CAS.
- For a recent example, see: H. Iino, T. Usui and J.-i. Hanna, Nat. Commun., 2015, 6, 6828 CrossRef CAS PubMed.
- For a recent review, see: R. G. Arrayás and J. C. Carretero, Chem. Commun., 2011, 47, 2207 RSC.
-
K.-M. Roy, Sulfones and Sulfoxides, Ullmann's Encyclopedia of Industrial Chemistry, Wiley-VCH, Weinheim, 2000 Search PubMed.
- For reviews on metal catalysed C–S bond formation, see:
(a) I. P. Beletskaya and V. P. Ananikov, Chem. Rev., 2011, 9, 1596 CrossRef PubMed;
(b) I. P. Beletskaya and V. P. Ananikov, Eur. J. Org. Chem., 2007, 3431 CrossRef CAS;
(c) C.-F. Lee, Y.-C. Liu and S. S. Badsara, Chem. – Asian J., 2014, 9, 706 CrossRef CAS PubMed;
(d) C. Shen, P. Zhang, Q. Sun, S. Bai, T. S. A. Hor and X. Liu, Chem. Soc. Rev., 2015, 44, 291 RSC . For a review on metal-catalysed synthesis of diaryl sulfides, see: ;
(e) C. C. Eichman and J. P. Stambuli, Molecules, 2011, 16, 590 CrossRef CAS PubMed.
- For selected references, see:
(a) M. A. Fernández-Rodríguez, Q. Shen and J. F. Hartwig, J. Am. Chem. Soc., 2006, 128, 2180 CrossRef PubMed;
(b) G. Bastug and S. P. Nolan, J. Org. Chem., 2013, 78, 9303 CrossRef CAS PubMed;
(c) M. Sayah and M. G. Organ, Chem. – Eur. J., 2011, 17, 11719 CrossRef CAS PubMed;
(d) M. Murata and S. L. Buchwald, Tetrahedron, 2004, 60, 7397 CrossRef CAS;
(e) J. Mao, T. Jia, G. Frensch and P. J. Walsh, Org. Lett., 2014, 16, 5304 CrossRef CAS PubMed;
(f) M. Kosugi, T. Shimizu and T. Migita, Chem. Lett., 1978, 13 CrossRef CAS.
- For selected references, see:
(a) Y. Zhang, K. C. Ngeow and J. Y. Ying, Org. Lett., 2007, 9, 3495 CrossRef CAS PubMed;
(b) O. Baldovino-Pantaleón, S. Hernández-Ortega and D. Morales-Morales, Adv. Synth. Catal., 2006, 348, 236 CrossRef;
(c) X.-B. Xu, J. Liu, J.-J. Zhang, Y.-W. Wang and Y. Peng, Org. Lett., 2013, 15, 550 CrossRef CAS PubMed;
(d) H. J. Cristau, B. Chabaud, A. Chene and H. Christol, Synthesis, 1981, 892 CrossRef CAS.
- M. Arisawa, T. Suzuki, T. Ishikawa and M. Yamaguchi, J. Am. Chem. Soc., 2008, 130, 12214 CrossRef CAS PubMed.
- For reviews, see:
(a) A. Sujatha, A. M. Thomas, A. P. Thankachan and G. Anilkumar, ARKIVOC, 2015, 1 Search PubMed;
(b) S. V. Ley and A. W. Thomas, Angew. Chem., Int. Ed., 2003, 42, 5400 CrossRef CAS PubMed. For a recent example, see: Z. Qiao, N. Gea and X. Jiang, Chem. Commun., 2015, 51, 10295 RSC.
- V. P. Reddy, A. V. Kumar, K. Swapna and K. R. Rao, Org. Lett., 2009, 11, 1697 CrossRef CAS PubMed.
- A. Correa, M. Carril and C. Bolm, Angew. Chem., Int. Ed., 2008, 47, 2880 CrossRef CAS PubMed.
- Y.-C. Wong, T. T. Jayanth and C.-H. Cheng, Org. Lett., 2006, 8, 5613 CrossRef CAS PubMed.
- For an example of a photoredox based diaryl sulfide synthesis using 3-diazopyridines and aryl thiosulfate, see:
(a) Y. Li, W. Xie and X. Jiang, Chem. – Eur. J., 2015, 21, 16059 CrossRef CAS PubMed . For methods involving benzynes and sulfoxides, see: ;
(b) Y. Li, D. Qiu, R. Gu, J. Wang, J. Shi and Y. Li, J. Am. Chem. Soc., 2016, 138, 10814 CrossRef CAS PubMed;
(c) M.-M. Lou, H. Wang, L. Song, H.-Y. Liu, Z.-Q. Li, X.-S. Guo, F.-G. Zhang and B. Wang, J. Org. Chem., 2016, 81, 5915 CrossRef CAS PubMed.
- For use of sulfenyl chlorides and stoichiometric organometallic reagents, see:
(a) J.-H. Cheng, C. Ramesh, H.-L. Kao, Y.-J. Wang, C.-C. Chan and C.-F. Lee, J. Org. Chem., 2012, 77, 10369 CrossRef CAS PubMed;
(b) I. M. Yonova, C. A. Osborne, N. S. Morrissette and E. R. Jarvo, J. Org. Chem., 2014, 79, 1947 CrossRef CAS PubMed.
- For a review, see reference 5d. For selected references, see:
(a) S. Vásquez-Céspedes, A. Ferry, L. Candish and F. Glorius, Angew. Chem., Int. Ed., 2015, 54, 5772 CrossRef PubMed;
(b) Y. Yang, W. Hou, L. Qin, J. Du, H. Feng, B. Zhou and Y. Li, Chem. – Eur. J., 2014, 20, 416 CrossRef CAS PubMed;
(c) L. Chu, X. Yue and F.-L. Qing, Org. Lett., 2010, 12, 1644 CrossRef CAS PubMed;
(d) P. Saravanan and P. Anbarasan, Org. Lett., 2014, 16, 848 CrossRef CAS PubMed;
(e) K. Inamoto, Y. Arai, K. Hiroya and T. Doi, Chem. Commun., 2008, 5529 RSC;
(f) P. Peng, J. Wang, C. Li, W. Zhu, H. Jiang and H. Liu, RSC Adv., 2016, 6, 57441 RSC;
(g) G. Yan, A. J. Borah and L. Wang, Org. Biomol. Chem., 2014, 12, 9557 RSC;
(h) Z. Li, J. Hong and X. Zhou, Tetrahedron, 2011, 67, 3690 CrossRef CAS;
(i) S. Zhang, P. Qian, M. Zhang, M. Hu and J. Cheng, J. Org. Chem., 2010, 75, 6732 CrossRef CAS PubMed;
(j) X.-L. Fang, R.-Y. Tang, X.-G. Zhang and J.-H. Li, Synthesis, 2011, 1099 CAS;
(k) T. Mukaiyama and K. Suzuki, Chem. Lett., 1993, 1 CrossRef CAS;
(l) J. Willwacher, R. Raj, S. Mohammed and B. G. Davis, J. Am. Chem. Soc., 2016, 138, 8678 CrossRef CAS PubMed;
(m) H. Tian, C. Zhu, H. Yanga and H. Fu, Chem. Commun., 2014, 50, 8875 RSC;
(n) P. Anbarasan, H. Neumann and M. Beller, Chem. Commun., 2011, 47, 3233 RSC.
- The Risk list. British Geological Survey (2012); http://https://www.bgs.ac.uk/news/NEWS/Risk_List_2012_Press_Release.pdf.
-
(a) ICH guideline Q3D on elemental impurities, European Medicines Agency, London, 2015;
(b) Ö. Usluer, M. Abbas, G. Wantz, L. Vignau, L. Hirsch, E. Grana, C. Brochon, E. Cloutet and G. Hadziioannou, ACS Macro Lett., 2014, 3, 1134 CrossRef.
- C.-L. Sun and Z.-J. Shi, Chem. Rev., 2014, 114, 9219 CrossRef CAS PubMed.
-
(a) T. Hostier, V. Ferey, G. Ricci, D. G. Pardo and J. Cossy, Chem. Commun., 2015, 51, 13898 RSC;
(b) H. Tian, H. Yang, C. Zhu and H. Fu, Adv. Synth. Catal., 2015, 357, 481 CrossRef CAS;
(c) T. Hostier, V. Ferey, G. Ricci, D. G. Pardo and J. Cossy, Org. Lett., 2015, 17, 3898 CrossRef CAS PubMed;
(d) M. Tudge, M. Tamiya, C. Savarin and G. R. Humphrey, Org. Lett., 2006, 8, 565 CrossRef CAS PubMed.
- For examples:
(a) F.-L. Yang and S.-K. Tian, Angew. Chem., Int. Ed., 2013, 52, 4929 CrossRef CAS PubMed;
(b) X. Kang, R. Yan, G. Yu, X. Pang, X. Liu, X. Li, L. Xiang and G. Huang, J. Org. Chem., 2014, 79, 10605 CrossRef CAS PubMed.
- For example: Q. Wu, D. Zhao, X. Qin, J. Lan and J. You, Chem. Commun., 2011, 47, 9188 RSC.
- For examples:
(a) F. Xiao, H. Xie, S. Liu and G.-J. Deng, Adv. Synth. Catal., 2014, 356, 364 CrossRef CAS;
(b) F. Xiao, S. Chen, J. Tian, H. Huang, Y. Liu and G.-J. Deng, Green Chem., 2016, 18, 1538 RSC.
- For examples:
(a) S. K. R. Parumala and R. K. Peddinti, Green Chem., 2015, 17, 4068 RSC;
(b) Y. Liao, P. Jiang, S. Chen, H. Qia and G.-J. Deng, Green Chem., 2013, 15, 3302 RSC;
(c) C. Ravi, D. C. Mohan and S. Adimurthy, Org. Lett., 2014, 16, 2978 CrossRef CAS PubMed;
(d) Z. Huang, D. Zhang, X. Qi, Z. Yan, M. Wang, H. Yan and A. Lei, Org. Lett., 2016, 18, 2351 CrossRef CAS PubMed.
- For examples:
(a) W. Ge and Y. Wei, Green Chem., 2012, 14, 2066 RSC;
(b) L.-H. Zou, J. Reball, J. Mottweiler and C. Bolm, Chem. Commun., 2012, 48, 11307 RSC;
(c) D. Huang, J. Chen, W. Dan, J. Ding, M. Liu and H. Wu, Adv. Synth. Catal., 2012, 354, 2123 CrossRef CAS;
(d) C. D. Prasad, S. J. Balkrishna, A. Kumar, B. S. Bhakuni, K. Shrimali, S. Biswas and S. Kumar, J. Org. Chem., 2013, 78, 1434 CrossRef CAS PubMed.
- For examples:
(a) H. Qi, T. Zhang, K. Wan and M. Luo, J. Org. Chem., 2016, 81, 4262 CrossRef CAS PubMed;
(b) T. Miao, P. Li, Y. Zhang and L. Wang, Org. Lett., 2015, 17, 832 CrossRef CAS PubMed.
-
(a) I. L. Baraznenok, V. G. Nenajdenko and E. S. Balenkova, Tetrahedron, 2000, 56, 3077 CrossRef CAS;
(b) V. G. Nenajdenko and E. S. Balenkova, Russ. J. Org. Chem., 2003, 39, 291 CrossRef;
(c) V. G. Nenajdenko, P. V. Vertelezkij and E. S. Balenkova, Sulfur Lett., 1996, 20, 75 CAS.
-
(a) A. J. Eberhart, C. Cicoira and D. J. Procter, Org. Lett., 2013, 15, 3994 CrossRef CAS PubMed;
(b) A. J. Eberhart, J. E. Imbriglio and D. J. Procter, Org. Lett., 2011, 13, 5882 CrossRef CAS PubMed;
(c) A. J. Eberhart and D. J. Procter, Angew. Chem., Int. Ed., 2013, 52, 4008 CrossRef CAS PubMed;
(d) A. J. Eberhart, H. J. Shrives, E. Álvarez, A. Carrër, Y. Zhang and D. J. Procter, Chem. – Eur. J., 2015, 21, 7428 CrossRef CAS PubMed;
(e) J. A. Fernández-Salas, A. J. Eberhart and D. J. Procter, J. Am. Chem. Soc., 2016, 138, 790 CrossRef PubMed . For a review of C–H coupling reactions directed by sulfoxides, see:;
(f) A. P. Pulis and D. J. Procter, Angew. Chem., Int. Ed., 2016, 55, 9842 CrossRef CAS PubMed.
- For intramolecular C–H thioarylations, see:
(a) A. Haryono, K. Miyatake, J. Natori and E. Tsuchida, Macromolecules, 1999, 32, 3146 CrossRef CAS;
(b) S. Yoshida, H. Yorimitsu and K. Oshima, Org. Lett., 2007, 9, 5573 CrossRef CAS PubMed;
(c) D. Vasu, H. Yorimitsu and A. Osuka, Angew. Chem., Int. Ed., 2015, 54, 7162 CrossRef CAS PubMed . For reactions of sulfoxonium salts with other carbon nucleophiles, see: ;
(d) Y. Endo, K. Shudo and T. Okamoto, Chem. Pharm. Bull., 1981, 29, 3753 CrossRef CAS;
(e) P. Cowper, Y. Jin, M. D. Turton, G. Kociok-Köhn and S. E. Lewis, Angew. Chem., Int. Ed., 2016, 55, 2564 CrossRef CAS PubMed;
(f) K. Hartke and D. Strangemann, Heterocycles, 1986, 24, 2399 CrossRef CAS;
(g) T. Hamashima, Y. Mori, K. Sawada, Y. Kasahara, D. Murayama, Y. Kamei, H. Okuno, Y. Yokoyama and H. Suzuki, Chem. Pharm. Bull., 2013, 61, 292 CrossRef CAS PubMed;
(h) S. Yoshida, H. Yorimitsu and K. Oshima, Org. Lett., 2009, 11, 2185 CrossRef CAS PubMed;
(i) G. Hu, J. Xu and P. Li, Org. Lett., 2014, 16, 6036 CrossRef CAS PubMed;
(j) K. Higuchi, M. Tayu and T. Kawasaki, Chem. Commun., 2011, 47, 6728 RSC;
(k) S. Akai, N. Kawashita, H. Satoh, Y. Wada, K. Kakiguchi, I. Kuriwaki and Y. Kita, Org. Lett., 2004, 6, 3793 CrossRef CAS PubMed;
(l) S. Akai, N. Kawashita, Y. Wada, H. Satoh, A. H. Alinejad, K. Kakiguchi, I. Kuriwaki and Y. Kita, Tetrahedron Lett., 2006, 47, 1881 CrossRef CAS;
(m) X. Huang, M. Patil, C. Farès, W. Thiel and N. Maulide, J. Am. Chem. Soc., 2013, 135, 7312 CrossRef CAS PubMed.
- For a recent review of Pummerer chemistry, see: L. H. S. Smith, S. C. Coote, H. F. Sneddon and D. J. Procter, Angew. Chem., Int. Ed., 2010, 49, 5832 CrossRef CAS PubMed.
- The sulfonium salt formed from 1a and 2a enroute to 3a, was obtained in 83% yield.
- See ESI† for details of optimisation.
- See ESI† and see: T. Ai, Y. Xu, L. Qiu, R. J. Geraghty and L. Chen, J. Med. Chem., 2015, 58, 785 CrossRef CAS PubMed.
- T. H. V. Huynh, B. Abrahamsen, K. K. Madsen, A. Gonzalez-Franquesa, A. A. Jensen and L. Bunch, Bioorg. Med. Chem., 2012, 20, 6831 CrossRef CAS PubMed.
- R. S. Kathayat and N. S. Finney, J. Am. Chem. Soc., 2013, 135, 12612 CrossRef CAS PubMed.
- H. Morris and J. Wallach, Drug Test. Anal., 2004, 6, 614 CrossRef PubMed.
- For examples, see:
(a)
S. Sarker and L. Nahar, Steroid Dimers: Chemistry and Applications in Drug Design and Delivery, John Wiley & Sons, Ltd, 2012 Search PubMed;
(b) H. A. Namanja, D. Emmert, D. A. Davis, C. Campos, D. S. Miller, C. A. Hrycyna and J. Chmielewski, J. Am. Chem. Soc., 2012, 134, 2976 CrossRef CAS PubMed.
- For a recent review of DMSO in synthesis, see: X.-F. Wu and K. Natte, Adv. Synth. Catal., 2016, 358, 336 CrossRef CAS.
- See ESI† for other examples of iterative C–H thioarylation using this method.
Footnote |
† Electronic supplementary information (ESI) available. See DOI: 10.1039/c6cc07627k |
|
This journal is © The Royal Society of Chemistry 2016 |
Click here to see how this site uses Cookies. View our privacy policy here.