DOI:
10.1039/C4SC02596B
(Edge Article)
Chem. Sci., 2015,
6, 1265-1271
Atom-economic generation of gold carbenes: gold-catalyzed formal [3+2] cycloaddition between ynamides and isoxazoles†
Received
26th August 2014
, Accepted 12th November 2014
First published on 12th November 2014
Abstract
The generation of gold carbenes via the gold-catalyzed intermolecular reaction of nucleophiles containing relatively labile N–O or N–N bonds with alkynes has received considerable attention during recent years. However, this protocol is not atom-economic as the reaction produces a stoichiometric amount of pyridine or quinoline waste, the cleaved part of the N–O or N–N bonds. In this article, we disclose an unprecedented gold-catalyzed formal [3+2] cycloaddition between ynamides and isoxazoles, allowing rapid and practical access to a wide range of synthetically-useful 2-aminopyrroles. Most importantly, mechanistic studies and theoretical calculations revealed that this reaction presumably proceeds via an α-imino gold carbene pathway, thus providing a strategically novel, atom-economic route to the generation of gold carbenes. Other significant features of this approach include the use of readily-available starting materials, high flexibility, simple procedure, mild reaction conditions, and in particular, no need to exclude moisture or air (“open flask”).
Introduction
Catalytic transformations involving gold carbenes are arguably the most important aspect of homogeneous gold catalysis.1 Recently, the possibility of forming an α-oxo gold carbenoid species via gold-catalyzed intramolecular or intermolecular alkyne oxidation by a N–O bond oxidant (initially a sulfoxide), pioneered by Toste and Zhang,2a,b represents a significant advance in gold carbene chemistry, and various efficient synthetic methods have been developed based on this strategy.2 Compared with intramolecular alkyne oxidation, the intermolecular approach offers much greater flexibility as no tethering of the oxidant is required, and therefore it is more synthetically useful.3 However, this intermolecular approach is obviously not atom-economic as the reaction produces a stoichiometric amount of pyridines or quinolines, the reduced form of the corresponding pyridine N-oxides or quinoline N-oxides, as waste (eqn (1)),4 which may even deactivate the gold catalyst via coordination.5 | 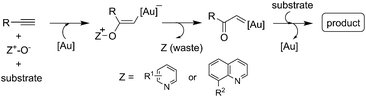 | (1) |
Access to the related α-imino gold carbenes via gold-catalyzed nitrene transfer to alkynes, however, remains a highly challenging task. Here, it should be noted that: (1) the nitrene moiety is delivered via an outer sphere attack and no gold nitrene complex6 is involved in this case; this mode of nitrene transfer is distinctively different from many well-established nitrene transfer reactions;7 (2) this protocol would present alkynes as equivalents of α-diazo imines, which are difficult to access as α-diazo imines can readily cyclize into the corresponding 1,2,3-triazoles. To date, only limited success has been achieved in this type of gold-catalyzed nitrene transfer, mainly by the intramolecular reaction of alkyne and azide.8 For example, Toste and co-workers used azide as an effective nitrene equivalent and realized the first protocol for the generation of α-imino gold carbenes in 2005.8a Later, elegant studies on the synthesis of indoles from alkynyl azides were demonstrated by Gagosz8c and Zhang,8d independently. Recently, several studies have invoked the intermolecular transfer of nitrene to alkynes by the use of iminopyridinium ylides as nitrene-transfer reagents, as disclosed by the groups of Zhang,9a Davies,9b,c and Liu.9d However, similar to those of the above-mentioned gold-catalyzed intermolecular alkyne oxidations, a stoichiometric amount of pyridine was produced as the waste in these cases. Therefore, the exploration of intermolecular approaches to the generation of α-imino gold carbenes, especially in an atom-economic way, is very attractive to researchers. We envisioned that the α-imino gold carbene intermediate B might be generated through the gold-catalyzed intermolecular reaction of ynamides 110 with isoxazoles 2, which could be obtained in an efficient and modular manner following the synthetic routes shown in eqn (3) and (4) in Scheme 1.11 The carbene B, likely highly electrophilic, could then undergo an electrophilic cyclization to yield the final 2-aminopyrroles 3, thus constituting a gold-catalyzed formal [3+2] cycloaddition (Scheme 1, eqn (2)). Herein, we report the successful implementation of this mechanistic design to a facile and practical synthesis of a wide range of polysubstituted 2-aminopyrroles, which are common structural motifs found in natural products and pharmacologically active molecules (Fig. 1)12 and are difficult to access via traditional methods for pyrrole synthesis.13 Most importantly, an α-imino gold carbene is most likely generated as the key intermediate on the basis of both mechanistic studies and theoretical calculations, thereby providing a strategically-novel, atom-economic route to the generation of gold carbenes.
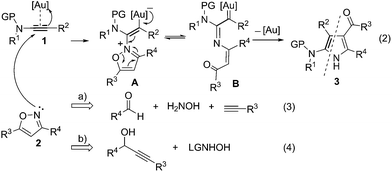 |
| Scheme 1 Synthetic design for the atom-economic generation of α-imino gold carbenes: formation of 2-aminopyrroles 3 through gold-catalyzed formal [3+2] cycloaddition between ynamides 1 and isoxazoles 2. | |
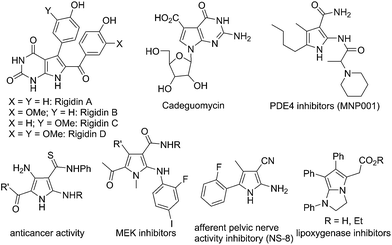 |
| Fig. 1 2-Aminopyrrole subunit in natural products and bioactive molecules. | |
Results and discussion
At the outset, ynamide 1a and 3,5-dimethylisoxazole 2a were used as the reacting species and a series of experiments were performed in order to validate our approach. To our delight, the expected product 3a was indeed formed in 70% 1H NMR yield in the presence of 5 mol% IPrAuNTf2 (Table 1, entry 1). Then, various typical gold catalysts with a range of electronic and steric characteristics were screened (Table 1, entries 2–7), and (ArO)3PAuNTf2 (Ar = 2,4-di-tert-butylphenyl) gave the best yield of the desired product (Table 1, entry 7). Somewhat surprisingly, AgNTf2 could also catalyze this reaction in 50% yield (Table 1, entry 8). Notably, without a metal catalyst, the reaction failed to give even a trace of 3a, and PtCl2 and Zn(OTf)2 were not effective in promoting this reaction (Table 1, entries 9–10).14 The reaction proved to be less efficient when it was performed at a reduced temperature (Table 1, entry 11). In addition, the use of 2 equiv. of 2a also gave the desired pyrrole 3a in 90% yield (Table 1, entry 12).
Table 1 Optimization of reaction conditionsa
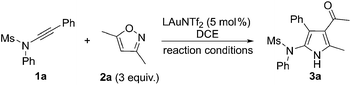
|
Entry |
Metal catalyst |
Conditions |
Yieldb (%) |
Reaction conditions: [1a] = 0.05 M; DCE = 1,2-dichloroethane.
Measured by 1H NMR using diethyl phthalate as the internal standard.
Dichloro(2-picolinato)gold(III).
Ar = 2,4-di-tert-butylphenyl.
1a was decomposed.
2.0 equiv. of 2a was used.
|
1 |
IPrAuNTf2 |
DCE, 80 °C, 3 h |
70 |
2 |
Ph3PAuNTf2 |
DCE, 80 °C, 3 h |
69 |
3 |
Et3PAuNTf2 |
DCE, 80 °C, 3 h |
54 |
4 |
Cy-JohnPhosAuNTf2 |
DCE, 80 °C, 3 h |
71 |
5 |
BrettPhosAuNTf2 |
DCE, 80 °C, 12 h |
27 |
6 |
Au(III)c |
DCE, 80 °C, 3 h |
34 |
7 |
(ArO)3PAuNTf2d |
DCE, 80 °C, 3 h |
95 |
8 |
AgNTf2 |
DCE, 80 °C, 3 h |
50 |
9e |
PtCl2 |
toluene, 80 °C, 3 h |
<5 |
10e |
Zn(OTf)2 (10 mol%) |
DCE, 80 °C, 3 h |
<5 |
11 |
(ArO)3PAuNTf2d |
DCE, 60 °C, 5 h |
75 |
12f |
(ArO)3PAuNTf2d |
DCE, 80 °C, 3 h |
90 |
With the optimized reaction conditions in hand, the scope of the transformation was explored. As seen from the results collected in Table 2, the reaction proceeded smoothly with various ynamide substrates 1, and the yields ranged from 58% to 96%. For example, ynamides with different protecting groups, even the Ns group (Table 2, entries 4–5), readily gave the desired 2-aminopyrroles 3a–f (Table 2, entries 1–6). Of note, an excellent yield could be achieved in the case of an ynamide with an oxazolidinone moiety and no dimerization reaction was observed (Table 2, entry 6).15 When R1 is an allyl group, the desired 3j could also be formed in 86% yield, and no cyclopropanation product was formed (Table 2, entry 10).16,5g Other aryl-substituted ynamides were also suitable substrates for this reaction, giving the corresponding functionalized pyrroles 3k–l in excellent yields (Table 2, entries 11–12). Interestingly, for styryl or cyclopropyl-substituted ynamides, this reaction still led to 75% yield and 58% yield, respectively (Table 2, entries 13–14). The molecular structure of 3a was further confirmed by X-ray diffraction (Fig. 2).17
Table 2 Reaction scope for different ynamides 1a

|
Reactions run in vials; [1] = 0.05 M; isolated yields are reported.
|
|
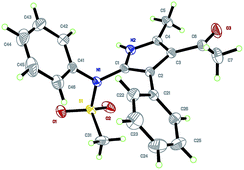 |
| Fig. 2 Crystal structure of compound 3a. | |
We next extended the reaction to different 3,5-disubstituted isoxazoles 2. To our delight, the reaction of ynamide 1i with various isoxazole substrates 2 worked well under the above optimized reaction conditions, giving versatile polysubstituted 2-aminopyrroles 3o–z in generally good to excellent yields. As summarized in Table 3, a range of aryl-substituted isoxazoles 2c–g were successful (R2 = aryl), delivering the desired 3p–t in 72–96% yield (Table 3, entries 2–6). In addition, when R1 is an aryl group, the reaction also worked well to afford the corresponding pyrroles 3v–w in excellent yields (Table 3, entries 8–9). Pleasingly, methyl 3-pyrrolecarboxylate 3x was formed in 90% yield from the corresponding isoxazole (Table 3, entry 10). It should be mentioned that 3-formylpyrroles 3y–z could also be prepared in serviceable yields (Table 3, entries 11–12).
Table 3 Reaction scope for different isoxazoles 2a
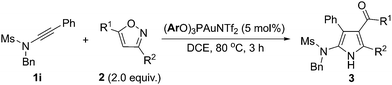
|
Reactions run in vials; [1i] = 0.05 M; isolated yields are reported.
|
|
Interestingly, when the scope of the method was extended to fully-substituted isoxazoles 4, the reaction also proceeded well, allowing the convenient synthesis of deacylated polysubstituted 2-aminopyrroles 5. A series of readily-available substituted ynamides was first examined. The corresponding pyrroles 5a–d were obtained in 72–85% yield (Table 4, entries 1–4). Then, isoxazoles 4 with substituents at the 4-position were also investigated, giving the products 5e–m in mostly good to excellent yields (Table 4, entries 5–13). Notably, methyl 3-pyrrolecarboxylate 5n could also be obtained in 77% yield from the corresponding 4-substituted isoxazole, which is complementary to the above protocol based on the 3,5-disubstituted isoxazoles 2 (Table 4, entry 14 vs.Table 3, entry 10). In particular, the 3,4-diphenyl substituted isoxazole also reacted smoothly, delivering the 3,4,5-triphenyl substituted pyrrole 5o in a respectable 60% yield (Table 4, entry 15).
Table 4 Reaction scope for different ynamides 1 and 4-substituted isoxazoles 4a

|
Reactions run in vials; [1] = 0.05 M; isolated yields are reported.
|
|
To further test the practicality of the current catalytic system, a gram-scale reaction of 1.36 g of 1a and 1.07 g of 2a was carried out with a much lower catalyst loading (1 mol%), and 1.72 g of the desired pyrrole 3a was formed in 85% yield, highlighting the synthetic utility of this chemistry (eqn (5)). Interestingly, the reaction could also be performed well even in water to afford the desired product 3a in 80% yield and no hydration of the ynamide was observed (eqn (6)),10a–c thus making this protocol more practical and environmentally benign.
| 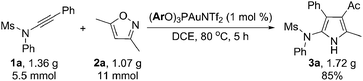 | (5) |
|  | (6) |
This chemistry can also be used to construct N-heteropyrrolizines, which are present in a variety of bioactive molecules.18,12k For example, treatment of ynamide 1p with isoxazole 4a under the optimized reaction conditions gave the pyrrole 5p, which could be converted into fused 2-aminopyrrole 6 in basic conditions in a one-pot process (63% two-step overall yield, eqn (7)). Compound 6 might serve as a precursor for the synthesis of lipoxygenase inhibitors (Fig. 1).12k
| 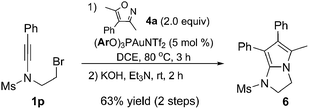 | (7) |
The sulfonamide could be readily transformed into a free amine (Scheme 2). For example, the reaction of ynamide 1d with isoxazole 2c under the optimized reaction conditions furnished pyrrole 7 in 81% yield. Nitrogen protection of 7 with a methyl group and subsequent removal of the Ns group using the standard conditions (PhSH, K2CO3) resulted in the formation of species 7a (53% two-step overall yield). Subsequent deprotection of the benzyl group in 7a could be realized by performing MnO2-mediated oxidation followed by hydrolysis to afford 7b in 56% yield.13e
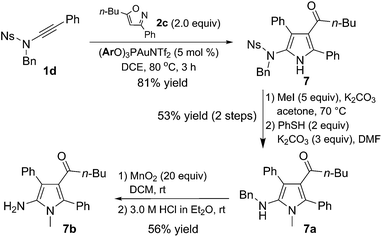 |
| Scheme 2 Transformation of a sulfonamide into an amine. | |
To probe the mechanism of this reaction, we first synthesized the alkyl-substituted ynamide 1q as the alkyl-substituted gold carbene is well-known in the gold-catalyzed cycloisomerizations of enynes; [1,2] hydride shift followed by elimination of the gold catalyst was involved as the critical deauration step.1e,19 Indeed, as depicted in eqn (8), when ynamide 1q reacted with 2a under the standard reaction conditions, none of the desired pyrrole was detected and α,β-unsaturated amide 8 was isolated in 25% yield. Amide 8 is supposed to be derived from [1,2] hydride shift followed by elimination of the gold catalyst and subsequent hydrolysis. This result indicated that a gold carbene is most likely generated as the key intermediate in this process. On the other hand, the low chemoselectivity in the case of n-butyl substituted ynamide shows the importance of aryl substituents on the ynamides to keep a high reactivity for the reactions in Tables 2–4.20
| 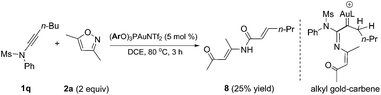 | (8) |
In addition, it was found that a key intermediate 3H-pyrrole 5l′ could be detected and isolated in the case of the reaction of ynamide 1i with fully-substituted isoxazole 4i (Table 4, entry 12). To further demonstrate this process, we monitored the reaction by 1H NMR spectroscopy, as depicted in Fig. 3. Here, the reaction was performed in the presence of 2 mol% (ArO)3PAuNTf2 in CDCl3 in order to better track the reaction intermediates. At the early stage of the reaction, we could clearly observe the formation of the 3H-pyrrole 5l′, which was gradually transformed into the final 1H-pyrrole 5l.
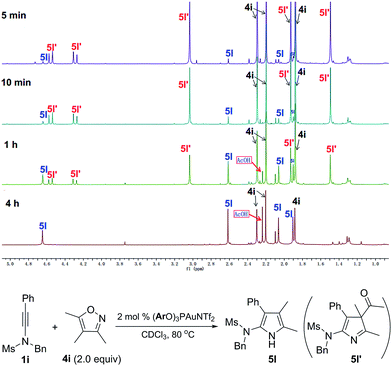 |
| Fig. 3
1H NMR monitoring of the reaction of ynamide 1i with fully-substituted isoxazole 4i. | |
A plausible mechanism to rationalize the formation of pyrrole 3 or 5 is illustrated in Scheme 3, in light of the above experimental observations and density functional theory (DFT) computations (see ESI† for details).21 Initially, nucleophilic attack of isoxazole 2 or 4 to the Au(I)-ligated alkyne of ynamide 1 forms vinyl gold intermediate A by overcoming a moderate barrier (12.2 kcal mol−1). Intermediate A isomerizes into the gold carbene intermediate B upon breakage of the isoxalic N–O bond,22 again requiring an activation energy around 12.0 kcal mol−1. Subsequent 1,5-cyclization23 within intermediate B readily occurs to afford the Au(I)-ligated 3H-pyrrole C, which upon ligand exchange with another ynamide 1 releases 3H-pyrrole D. The whole process is highly exothermic with free energy release amounting to 52 kcal mol−1. For 3,5-disubstituted isoxazoles 2, 3H-pyrrole D readily isomerizes into the final aromatic 1H-pyrrole 3 by sigmatropic H-migrations.24 In the case of fully-substituted isoxazole substrates 4, D is ultimately transformed into the final 1H-pyrrole 5, presumably by a water-assisted deacylative aromatization.25
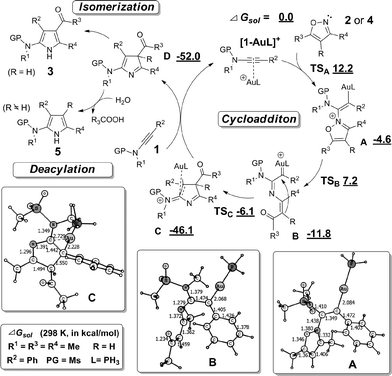 |
| Scheme 3 Plausible reaction mechanism. Theoretical investigations on the reaction pathways for the formation of product 3g (Table 2, entry 7): relative free energies (ΔGsol, in kcal mol−1) of key intermediates and transition states were computed at the M06/6-31+G(d)/SDD level in 1,2-dichloroethane at 298 K. | |
Conclusions
In summary, we have developed a novel gold-catalyzed formal [3+2] cycloaddition between ynamides and isoxazoles, leading to the concise and flexible synthesis of polysubstituted 2-aminopyrroles. This methodology makes it possible to introduce four substituents onto a pyrrole ring very freely with high efficiency. Of particular interest, fully substituted isoxazoles also react under deacylation, closing a further gap in the reaction scope. Moreover, an α-imino gold carbene is the most likely intermediate based on both mechanistic studies and theoretical calculations, thus providing a new strategy for the generation of gold carbenes, especially in an atom-economic way. Studies to elucidate the detailed mechanism and further synthetic applications of the current protocol are in progress in our laboratory.
Acknowledgements
We are grateful for the financial support from NSFC (no. 21102119, 21273177 and 21272191), RFDP (20130121110004), NFFTBS (no. J1310024), and the Program for Changjiang Scholars and Innovative Research Team in University.
Notes and references
- For reviews, see:
(a) L. Fensterbank and M. Malacria, Acc. Chem. Res., 2014, 47, 953 CrossRef CAS PubMed;
(b) C. Obradors and A. M. Echavarren, Acc. Chem. Res., 2014, 47, 902 CrossRef CAS PubMed;
(c) C. Obradors and A. M. Echavarren, Chem. Commun., 2014, 50, 16 RSC;
(d) A. S. K. Hashmi, Angew. Chem., Int. Ed., 2010, 49, 5232 CrossRef CAS PubMed;
(e) E. Jiménez-Núñez and A. M. Echavarren, Chem. Rev., 2008, 108, 3326 CrossRef PubMed;
(f) A. S. K. Hashmi, Chem. Rev., 2007, 107, 3180 CrossRef CAS PubMed;
(g) A. Fürstner and P. W. Davies, Angew. Chem., Int. Ed., 2007, 46, 3410 CrossRef PubMed.
-
(a) N. D. Shapiro and F. D. Toste, J. Am. Chem. Soc., 2007, 129, 4160 CrossRef CAS PubMed;
(b) G. Li and L. Zhang, Angew. Chem., Int. Ed., 2007, 46, 5156 CrossRef CAS PubMed ; for reviews, see: ;
(c) J. Xiao and X. Li, Angew. Chem., Int. Ed., 2011, 50, 7226 CrossRef CAS PubMed;
(d) L. Zhang, Acc. Chem. Res., 2014, 47, 877 CrossRef CAS PubMed;
(e) H.-S. Yeom and S. Shin, Acc. Chem. Res., 2014, 47, 966 CrossRef CAS PubMed.
- For recent representative examples on gold-catalyzed intermolecular alkyne oxidation, see:
(a) J. Schulz, L. Jašíková, A. Škríba and J. Roithová, J. Am. Chem. Soc., 2014, 136, 11513 CrossRef CAS PubMed;
(b) L. Li, C. Shu, B. Zhou, Y.-F. Yu, X.-Y. Xiao and L.-W. Ye, Chem. Sci., 2014, 5, 4057 RSC;
(c) F. Pan, S. Liu, C. Shu, R.-K. Lin, Y.-F. Yu, J.-M. Zhou and L.-W. Ye, Chem. Commun., 2014, 50, 10726 RSC;
(d) S. N. Karad and R.-S. Liu, Angew. Chem., Int. Ed., 2014, 53, 5444 CrossRef CAS PubMed;
(e) T. Wang, S. Shi, M. M. Hansmann, E. Rettenmeier, M. Rudolph and A. S. K. Hashmi, Angew. Chem., Int. Ed., 2014, 53, 3715 CrossRef CAS PubMed;
(f) T. Wang, S. Shi, M. Rudolph and A. S. K. Hashmi, Adv. Synth. Catal., 2014, 356, 2337 CrossRef CAS;
(g) M. D. Santos and P. W. Davies, Chem. Commun., 2014, 50, 6001 RSC;
(h) J. Li, K. Ji, R. Zheng, J. Nelson and L. Zhang, Chem. Commun., 2014, 50, 4130 RSC;
(i) G. Wu, R. Zheng, J. Nelson and L. Zhang, Adv. Synth. Catal., 2014, 356, 1229 CrossRef CAS;
(j) P. Nösel, L. N. dos Santos Comprido, T. Lauterbach, M. Rudolph, F. Rominger and A. S. K. Hashmi, J. Am. Chem. Soc., 2013, 135, 15662 CrossRef PubMed;
(k) L. Wang, X. Xie and Y. Liu, Angew. Chem., Int. Ed., 2013, 52, 13302 CrossRef CAS PubMed;
(l) S. K. Pawar, C.-D. Wang, S. Bhunia, A. M. Jadhav and R.-S. Liu, Angew. Chem., Int. Ed., 2013, 52, 7559 CrossRef CAS PubMed;
(m) K. Ji, Y. Zhao and L. Zhang, Angew. Chem., Int. Ed., 2013, 52, 6508 CrossRef CAS PubMed;
(n) G. Henrion, T. E. J. Chava, X. Le Goff and F. Gagosz, Angew. Chem., Int. Ed., 2013, 52, 6277 CrossRef CAS PubMed;
(o) S. Ghorpade, M.-D. Su and R.-S. Liu, Angew. Chem., Int. Ed., 2013, 52, 4229 CrossRef CAS PubMed.
- For examples on the utilization of the side products, see:
(a) E. P. A. Talbot, M. Richardson, J. M. McKenna and F. D. Toste, Adv. Synth. Catal., 2014, 356, 687 CrossRef CAS PubMed;
(b) D. B. Huple, S. Ghorpade and R.-S. Liu, Chem.–Eur. J., 2013, 19, 12965 CrossRef CAS PubMed;
(c) A. Mukherjee, R. B. Dateer, R. Chaudhuri, S. Bhunia, S. N. Karad and R.-S. Liu, J. Am. Chem. Soc., 2011, 133, 15372 CrossRef CAS PubMed.
- To avoid catalyst deactivation by the byproduct pyridine or quinoline, acid has to be used as the additive in some cases. See:
(a) C. Shu, L. Li, X.-Y. Xiao, Y.-F. Yu, Y.-F. Ping, J.-M. Zhou and L.-W. Ye, Chem. Commun., 2014, 50, 8689 RSC;
(b) C. Shu, L. Li, Y.-F. Yu, S. Jiang and L.-W. Ye, Chem. Commun., 2014, 50, 2522 RSC;
(c) S. Shi, T. Wang, W. Yang, M. Rudolph and A. S. K. Hashmi, Chem.–Eur. J., 2013, 19, 6576 CrossRef CAS PubMed;
(d) A. S. K. Hashmi, T. Wang, S. Shi and M. Rudolph, J. Org. Chem., 2012, 77, 7761 CrossRef CAS PubMed;
(e) M. Xu, T.-T. Ren and C.-Y. Li, Org. Lett., 2012, 14, 4902 CrossRef CAS PubMed;
(f) W. He, L. Xie, Y. Xu, J. Xiang and L. Zhang, Org. Biomol. Chem., 2012, 10, 3168 RSC;
(g) D. Qian and J. Zhang, Chem. Commun., 2011, 47, 11152 RSC;
(h) Y. Luo, G. Zhang, E. S. Hwang, T. A. Wilcoxon and L. Zhang, Beilstein J. Org. Chem., 2011, 7, 596 CrossRef CAS PubMed;
(i) L. Ye, W. He and L. Zhang, J. Am. Chem. Soc., 2010, 132, 8550 CrossRef CAS PubMed;
(j) L. Ye, L. Cui, G. Zhang and L. Zhang, J. Am. Chem. Soc., 2010, 132, 3258 CrossRef CAS PubMed.
-
(a) Z. Li, D. A. Capretto, R. O. Rahaman and C. He, J. Am. Chem. Soc., 2007, 129, 12058 CrossRef CAS PubMed;
(b) Z. Li, X. Ding and C. He, J. Org. Chem., 2006, 71, 5876 CrossRef CAS PubMed.
- For selected reviews, see:
(a) S. Fantauzzi, A. Caselli and E. Gallo, Dalton Trans., 2009, 5434 RSC;
(b) M. M. Diaz-Requejo and P. J. Perez, Chem. Rev., 2008, 108, 3379 CrossRef CAS PubMed;
(c) P. Mueller and C. Fruit, Chem. Rev., 2003, 103, 2905 CrossRef CAS PubMed.
-
(a) D. J. Gorin, N. R. Davis and F. D. Toste, J. Am. Chem. Soc., 2005, 127, 11260 CrossRef CAS PubMed;
(b) N. D. Shapiro and F. D. Toste, J. Am. Chem. Soc., 2007, 129, 4160 CrossRef CAS PubMed;
(c) A. Wetzel and F. Gagosz, Angew. Chem., Int. Ed., 2011, 50, 7354 CrossRef CAS PubMed;
(d) B. Lu, Y. Luo, L. Liu, L. Ye, Y. Wang and L. Zhang, Angew. Chem., Int. Ed., 2011, 50, 8358 CrossRef CAS PubMed;
(e) Y. Xiao and L. Zhang, Org. Lett., 2012, 14, 4662 CrossRef CAS PubMed;
(f) Z.-Y. Yan, Y. Xiao and L. Zhang, Angew. Chem., Int. Ed., 2012, 51, 8624 CrossRef CAS PubMed;
(g) Y. Tokimizu, S. Oishi, N. Fujii and H. Ohno, Org. Lett., 2014, 16, 3138 CrossRef CAS PubMed;
(h) During the preparation of this manuscript, an intramolecular reaction between alkyne and 2H-azirine groups was reported: A. Prechter, G. Henrion, P. F. dit Bel and F. Gagosz, Angew. Chem., Int. Ed., 2014, 53, 4959 CrossRef CAS PubMed.
-
(a) C. Li and L. Zhang, Org. Lett., 2011, 13, 1738 CrossRef CAS PubMed;
(b) P. W. Davies, A. Cremonesi and L. Dumitrescu, Angew. Chem., Int. Ed., 2011, 50, 8931 CrossRef CAS PubMed;
(c) E. Chatzopoulou and P. W. Davies, Chem. Commun., 2013, 49, 8617 RSC;
(d) H.-H. Hung, Y.-C. Liao and R.-S. Liu, J. Org. Chem., 2013, 78, 7970 CrossRef CAS PubMed.
- For recent reviews on ynamide reactivity, see:
(a) X.-N. Wang, H.-S. Yeom, L.-C. Fang, S. He, Z.-X. Ma, B. L. Kedrowski and R. P. Hsung, Acc. Chem. Res., 2014, 47, 560 CrossRef CAS PubMed;
(b) K. A. DeKorver, H. Li, A. G. Lohse, R. Hayashi, Z. Lu, Y. Zhang and R. P. Hsung, Chem. Rev., 2010, 110, 5064 CrossRef CAS PubMed;
(c) G. Evano, A. Coste and K. Jouvin, Angew. Chem., Int. Ed., 2010, 49, 2840 CrossRef CAS PubMed ; for recent selected examples on the gold-catalyzed reactions of ynamides, see: ;
(d) S. K. Pawar, D. Vasu and R.-S. Liu, Adv. Synth. Catal., 2014, 356, 2411 CrossRef CAS;
(e) E. Rettenmeier, A. M. Schuster, M. Rudolph, F. Rominger, C. A. Gade and A. S. K. Hashmi, Angew. Chem., Int. Ed., 2013, 52, 5880 CrossRef CAS PubMed;
(f) S. J. Heffernan, J. M. Beddoes, M. F. Mahon, A. J. Hennessy and D. R. Carbery, Chem. Commun., 2013, 49, 2314 RSC;
(g) S. N. Karad, S. Bhunia and R.-S. Liu, Angew. Chem., Int. Ed., 2012, 51, 8722 CrossRef CAS PubMed;
(h) R. B. Dateer, B. S. Shaibu and R.-S. Liu, Angew. Chem., Int. Ed., 2012, 51, 113 CrossRef CAS PubMed;
(i) R. B. Dateer, K. Pati and R.-S. Liu, Chem. Commun., 2012, 48, 7200 RSC.
-
(a) T. M. V. D. Pinho e Melo, Curr. Org. Chem., 2005, 9, 925 CrossRef CAS;
(b) T. V. Hansen, P. Wu and V. V. Fokin, J. Org. Chem., 2005, 70, 7761 CrossRef CAS PubMed;
(c) S. Tang, J. He, Y. Sun, L. He and X. She, Org. Lett., 2009, 11, 3982 CrossRef CAS PubMed;
(d) O. Debleds, E. Gayon, E. Ostaszuk, E. Vrancken and J.-M. Campagne, Chem.–Eur. J., 2010, 16, 12207 CrossRef CAS PubMed;
(e) A. G. Griesbeck, M. Franke, J. Neudörfl and H. Kotaka, Beilstein J. Org. Chem., 2011, 7, 127 CrossRef CAS PubMed.
- For selected examples, see:
(a) T. B. Kumar, C. Sumanth, S. Vaishaly, M. S. Rao, K. B. C. Sekhar, C. L. T. Meda, A. Kandale, D. Rambabu, G. R. Krishna, C. M. Reddy, K. S. Kumar, K. V. L. Parsa and M. Pal, Bioorg. Med. Chem. Lett., 2012, 22, 5639 CrossRef PubMed;
(b) L. V. Frolova, N. M. Evdokimov, K. Hayden, I. Malik, S. Rogelj, A. Kornienko and I. V. Magedov, Org. Lett., 2011, 13, 1118 CrossRef CAS PubMed;
(c) H. B. Jalani, A. N. Pandya, A. B. Baraiya, J. C. Kaila, D. H. Pandya, J. A. Sharma, V. Sudarsanam and K. K. Vasu, Tetrahedron Lett., 2011, 52, 6331 CrossRef CAS PubMed;
(d) M. B. Wallace, M. E. Adams, T. Kanouni, C. D. Mol, D. R. Dougan, V. A. Feher, S. M. O'Connell, L. Shi, P. Halkowycz and Q. Dong, Bioorg. Med. Chem. Lett., 2010, 20, 4156 CrossRef CAS PubMed;
(e) V. Onnis, A. De Log, M. T. Cocco, R. Fadda, R. Meleddu and C. Congiu, Eur. J. Med. Chem., 2009, 44, 1288 CrossRef CAS PubMed;
(f) M. T. Migawa, J. C. Drach and L. B. Townsend, J. Med. Chem., 2005, 48, 3840 CrossRef CAS PubMed;
(g) A. Lauria, M. Bruno, P. Diana, P. Barraja, A. Montalbano, G. Cirrincione, G. Dattolo and A. M. Almerico, Bioorg. Med. Chem., 2005, 13, 1545 CrossRef CAS PubMed;
(h) M. T. Cocco, C. Congiu and V. Onnis, Bioorg. Med. Chem., 2003, 11, 495 CrossRef CAS;
(i) M. Tanaka, Y. Sasaki, Y. Kimura, T. Fukui and Y. Ukai, BJU Int., 2003, 92, 1031 CrossRef CAS PubMed;
(j) C. E. Stephens, T. M. Felder, J. W. Sowell Sr, G. Andrei, J. Balzarini, R. Snoeck and E. D. Clercq, Bioorg. Med. Chem., 2001, 9, 1123 CrossRef CAS;
(k)
S. Laufer, H. G. Striegel and G. Dannhardt, Ger. Offen. DE 44 19 315A1, 1995;
(l) S. M. Bennet, N. Nguyen-Ba and K. K. Ogilvie, J. Med. Chem., 1990, 33, 2162 CrossRef;
(m) J. Kobayshi, J. Cheng, Y. Kikuchi, M. Ishibashi, S. Yamamura, Y. Ohizumi, T. Ohta and S. Nozoe, Tetrahedron Lett., 1990, 31, 4617 CrossRef.
- For recent examples on 2-aminopyrrole synthesis, see:
(a) X. Qi, H. Xiang, Q. He and C. Yang, Org. Lett., 2014, 16, 4186 CrossRef CAS PubMed;
(b) X. Wang, X.-P. Xu, S.-Y. Wang, W. Zhou and S.-J. Ji, Org. Lett., 2013, 15, 4246 CrossRef CAS PubMed;
(c) W. Yu, W. Chen, S. Liu, J. Shao, Z. Shao, H. Lin and Y. Yu, Tetrahedron, 2013, 69, 1953 CrossRef CAS PubMed;
(d) L. V. Frolova, N. M. Evdokimov, K. Hayden, I. Malik, S. Rogelj, A. Kornienko and I. V. Magedov, Org. Lett., 2011, 13, 1118 CrossRef CAS PubMed;
(e) P. Fontaine, G. Masson and J. Zhu, Org. Lett., 2009, 11, 1555 CrossRef CAS PubMed;
(f) E. Barnea, S. Majumder, R. J. Staples and A. L. Odom, Organometallics, 2009, 28, 3876 CrossRef CAS;
(g) T.-C. Chien, E. A. Meade, J. M. Hinkley and L. B. Townsend, Org. Lett., 2004, 6, 2857 CrossRef CAS PubMed;
(h) V. Nair, A. U. Vinod and C. Rajesh, J. Org. Chem., 2001, 66, 4427 CrossRef CAS.
- For recent Zn(OTf)2-catalyzed metathesis reactions between 3-en-1-ynamides and nitrosoarenes, see: S. A. Gawade, D. B. Huple and R.-S. Liu, J. Am. Chem. Soc., 2014, 136, 2978 CrossRef CAS PubMed.
- S. Kramer, Y. Odabachian, J. Overgaard, M. Rottländer, F. Gagosz and T. Skrydstrup, Angew. Chem., Int. Ed., 2011, 50, 5090 CrossRef CAS PubMed.
- For examples on gold-catalyzed oxidative-cyclopropanation, see:
(a) K.-B. Wang, R.-Q. Ran, S.-D. Xiu and C.-Y. Li, Org. Lett., 2013, 15, 2374 CrossRef CAS PubMed;
(b) D. Vasu, H.-H. Hung, S. Bhunia, S. A. Gawade, A. Das and R.-S. Liu, Angew. Chem., Int. Ed., 2011, 50, 6911 CrossRef CAS PubMed.
- ESI.†.
-
(a)
Z. Li, X. Xu, Z. Ye, X. Qian, X. Shao, Z. Xu, B. Zeng and G. Song, PCT Int. Appl. WO 2013007168A1, 2013;
(b)
H. B. Lowman and S. Liu, PCT Int. Appl. WO 2013192546A1, 2013;
(c)
W. G. Kerr, PCT Int. Appl. WO 2010045199A2, 2010;
(d)
A. Lagrange, PCT Int. Appl. WO 2007071686A1, 2007;
(e)
J. Cotteret and A. Lagrange, Eur. Pat. Appl. EP 1428510A1, 2004;
(f)
T. D. Mckee and R. K. Suto, PCT Int. Appl. WO 2003073999A2, 2003;
(g)
G. Lang, PCT Int. Appl. WO 2001066071A1, 2001;
(h)
M. P. Audousset, Eur. Pat. Appl. EP 1002520A1, 2000;
(i)
L. Vidal and G. Malle, PCT Int. Appl. WO 9735554A1, 1997.
-
(a) L. Zhang, J. Sun and S. A. Kozmin, Adv. Synth. Catal., 2006, 348, 2271 CrossRef CAS;
(b) P. W. Davies, A. Cremonesi and N. Martin, Chem. Commun., 2011, 47, 379 RSC;
(c) B. Lu, C. Li and L. Zhang, J. Am. Chem. Soc., 2010, 132, 14070 CrossRef CAS PubMed.
- The DFT studies on substituent effects are given in the ESI.†.
- For theoretical calculations involving α-oxo gold carbenes, see:
(a) J. Schulz, L. Jašíková, A. Škríba and J. Roithová, J. Am. Chem. Soc., 2014, 136, 11513 CrossRef CAS PubMed;
(b) B. Lu, Y. Li, Y. Wang, D. H. Aue, Y. Luo and L. Zhang, J. Am. Chem. Soc., 2013, 135, 8512 CrossRef CAS PubMed.
- C. Kashima, N. Mukai, Y. Yamamoto, Y. Tsuda and Y. Omote, Heterocycles, 1977, 7, 241 CrossRef CAS.
- J. R. Manning and H. M. L. Davies, J. Am. Chem. Soc., 2008, 130, 8602 CrossRef CAS PubMed.
- W. Friedrichsen, T. Traulsen, J. Elguero and A. R. Katritzky, Adv. Heterocycl. Chem., 2000, 76, 85 CrossRef CAS.
- For selected examples of acid-catalyzed deacylation of pyrroles, see:
(a) A. E. Ondrus, H. Ü. Kaniskan and M. Movassaghi, Tetrahedron, 2010, 66, 4784 CrossRef CAS PubMed;
(b) F. Barrero, J. F. Sánchez, J. E. Oltra and D. J. Teva, J. Heterocycl. Chem., 1991, 28, 939 CrossRef;
(c) K. M. Smith, M. Miura and H. D. Tabba, J. Org. Chem., 1983, 48, 4779 CrossRef CAS.
Footnote |
† Electronic supplementary information (ESI) available. CCDC 1009902. For ESI and crystallographic data in CIF or other electronic format see DOI: 10.1039/c4sc02596b |
|
This journal is © The Royal Society of Chemistry 2015 |
Click here to see how this site uses Cookies. View our privacy policy here.