DOI:
10.1039/C5RA21466A
(Paper)
RSC Adv., 2015,
5, 101616-101622
Synthesis, crystal structure and magnetic properties of the complex [ReCl3(tppz)]·MeCN†
Received
16th October 2015
, Accepted 17th November 2015
First published on 18th November 2015
Abstract
The reaction of the starting materials [ReIIICl3(MeCN)(PPh3)2] or [ReVOCl3(PPh3)2] with 2,3,5,6-tetrakis(2-pyridyl)pyrazine (tppz) in acetonitrile yielded the Re(III) complex [ReCl3(tppz)]·MeCN (1). This complex crystallizes in the monoclinic space group P21/n and its crystal structure consists of neutral mononuclear entities with meridional geometry of the chloride ligands, and the six-coordination of the Re(III) ion being completed by the tridentate tppz ligand. Each metal centre exhibits a highly distorted octahedral coordination with Re–Cl and Re–Ntppz bond lengths covering the ranges 2.3590(9)–2.3606(8) and 1.971(2)–2.096(2) Å, respectively. The magnetic properties of 1 have been investigated in the temperature range 1.9–290 K. They are characteristic of a six-coordinate Re(III) mononuclear complex with d4 low-spin (3T1 ground state). The magnetic data of 1 are discussed through a deep analysis of the influence of the ligand-field, spin–orbit coupling, tetragonal distortion and covalency effects. The second-order Zeeman effect between the non-magnetic ground state (MJ = 0) and higher energy levels (MJ ≠ 0) determines the magnetic susceptibility of 1, the value of the temperature-independent paramagnetic susceptibility being 3378 × 10−6 cm3 mol−1. This value compares well with those reported for other structurally characterized Re(III) complexes.
Introduction
2,3,5,6-Tetrakis(2-pyridyl)pyrazine (tppz), synthesized by Goodwin and Lions in 1959,1 is a remarkably versatile high-electron donor ligand offering a wide variety of binding modes for the construction of novel systems. It has evidenced that tppz can act as a bidentate (α, β and γ), tridentate, bis-bidentate, tris-bidentate and bis-tridentate ligand2 as well as a counterion in its diprotonated form.3 Up to now, 206 compounds incorporating tppz have been reported. Among them, 59 complexes are mononuclear and 147 are multinuclear (including bi-, tri-, tetranuclear compounds).4
A scientific interest in tppz complexes can be attributed to rich photophysical and redox properties associated with these compounds making them useful for analytical purposes and probes for biologically relevant molecules such as DNA.5 More recently, tppz has been examined as fluorescence sensor for series of metal ions and off–off–on switching of fluorescence depending on stepwise complex formation with tppz has been reported.6 A particular emphasis has been paid to studies of the homo- and hetero-polynuclear ruthenium complexes which have shown that tppz is an effective mediator for intermetallic coupling nearly of the order of the Creutz–Taube ion.7
Another relevant aspect of the coordination chemistry of tppz concerns the ability of this polydentate organic ligand as a bridge to mediate magnetic interactions between paramagnetic centers separated by more than 6.4 Å. The compounds incorporating the [M2(tppz)]4+ unit [M = Ni(II) and Cu(II)] were found to exhibit significant antiferromagnetic interactions between the paramagnetic metal ions involved.2b,8 Interestingly, the relatively good efficiency of the tppz bridge in transmitting magnetic interactions between paramagnetic centers contrasts with the much poorer ability of the related bridging pyrazine molecule in its metal complexes, where negligible or weak antiferromagnetic interactions were observed (J values ranging from −7.4 to 0 cm−1).9 Finally, mononuclear complexes of Co(II) of formula [Co(tppz)2]2+ with the tppz ligands coordinated in a tridentate coordination mode deserve to be mentioned. These compounds attract scientific interest due to the thermally induced spin crossover behavior from a high-spin SCo = 3/2 at high temperatures to a low-spin SCo = 1/2 at lower temperatures.2b,8b,10
The present contribution covers the synthesis and magneto-structural characterization of the new mononuclear rhenium(III) complex [ReCl3(tppz)]·MeCN (1). The coordination chemistry of Re(III) is dominated by the diamagnetic Re26+ core.11 Examples of mononuclear Re(III) complexes incorporating phosphorus,12 sulphur13 and less frequently nitrogen14 or oxygen15 donor ligands have also been described but their magnetic behavior, with compounds exhibiting different degrees of paramagnetism and even diamagnetism,14h,m,15d can still be considered as largely unexplored.
Results and discussion
Synthesis
The complex [ReCl3(tppz)]·MeCN (1) was prepared by treating [ReOCl3(PPh3)2] or [ReCl3(MeCN)(PPh3)2] with 2,3,5,6-tetra-(2-pyridyl)pyrazine (tppz) in refluxing acetonitrile: |
[ReIIICl3(MeCN)(PPh3)2] + tppz → [ReIIICl3(tppz)] + 2PPh3 + MeCN
| (1) |
|
[ReVOCl3(PPh3)2] + tppz → [ReIIICl3(tppz)] + OPPh3 + PPh3
| (2) |
A relatively high yield of [ReCl3(tppz)] (ca. 70%) in the reaction (2) indicates that the tridentate nitrogen donor tppz facilitates the substitution of the phosphine ligands and then the abstraction of the oxo group and reduction to the [ReIIICl3] unit. A similar trend was confirmed by Zubieta et al. for bifunctional chelators based on pyridyl, imidazole- and carboxylate-derivatized amino acids.14f In general, the use of additional PPh3 is necessary to abstract the oxo group and reduce the [ReV
O]3+ core of the [ReOCl3(PPh3)2] complex to the [ReIIICl3] unit.14m,16
Crystal structure
Compound 1 crystallizes in the monoclinic space group P21/n (Table 1) and its crystal structure consists of neutral [ReCl3(tppz)] units and solvated acetonitrile molecules. A perspective view of the asymmetric unit of 1 together with the atom numbering is shown in Fig. 1. No intermolecular interactions with enough strength to govern the crystal packing or molecule conformation were found in the structure of 1 except for weak intra- and intermolecular C–H⋯N and C–H⋯Cl type interactions [C(6)–H(6)⋯N(4) with D⋯A distance = 2.570 Å and D–H⋯A angle = 114.0°, C(6)–H(6)⋯Cl(1)a with D⋯A distance = 2.760 Å and D–H⋯A angle = 139.0° and C(22)–H(22)⋯N(99)b with D⋯A distance = 2.560 Å and D–H⋯A angle = 134.0°; symmetry code: (a) = −x, 1 − y, −z and (b) = 1 − x, 2 − y, −z] and Re–Cl⋯π contacts [Re1–Cl1⋯Cg1a (N4–C10–C11–C12–C13–C14) with a distance of 3.761(2) Å and an angle (between X(I) → Cg(J) vector and normal to plane J) of 10.99°] (Fig. 2).
Table 1 Crystal data and structure refinement for complex 1
|
1 |
Empirical formula |
C26H19Cl3N7Re |
Formula weight (g mol−1) |
722.03 |
Temperature (K) |
293(2) |
Wavelength (Å) |
0.71073 |
Crystal system |
Monoclinic |
Space group |
P21/n |
Unit cell dimensions (Å, °) |
a = 14.9278(4) |
b = 10.2675(2) |
c = 17.3725(5) |
β = 99.442(3) |
Volume (Å3) |
2626.63(12) |
Z |
4 |
ρcalcd (Mg m−3) |
1.826 |
Absorption coefficient (mm−1) |
4.962 |
F(000) |
1400 |
Crystal size [mm] |
0.051 × 0.088 × 0.104 |
θ range for data collection [°] |
3.34 to 25.05 |
Index ranges |
−17 ≤ h ≤ 17, −12 ≤ k ≤ 12, −20 ≤ l ≤ 20 |
Reflections collected |
14 243 |
Independent reflections |
4633 [Rint = 0.0296] |
Completeness to 2θ = 25° |
99.8% |
Min. and max. transm. |
0.617 and 1.000 |
Data/restraints/parameters |
4633/0/335 |
R indices (all data) |
R1 = 0.0272, wR2 = 0.0453 |
Largest diff. peak and hole (e Å−3) |
0.560 and −0.375 |
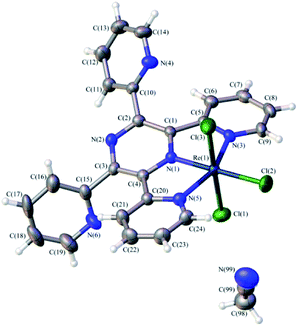 |
| Fig. 1 Perspective view of the molecular structure of [ReCl3(tppz)]·MeCN (1) showing the atom numbering. Displacement ellipsoids are drawn at 50% probability level. | |
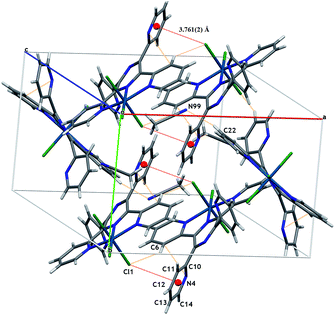 |
| Fig. 2 View of the molecular packing of 1 with marked Re–Cl⋯π as well as C–H⋯N and C–H⋯Cl type interactions. | |
The Re(III) ion is six-coordinate in a highly distorted octahedral geometry defined by three nitrogen atoms of the tridentate coordinated tppz ligand and three chloride donors, meridionally arranged. The major angular distortions from the idealized octahedral geometry, reflected in the cisoid [77.45(9)–103.44(7)°] and transoid [154.98(10)–179.08(7)°] angles, are attributed to geometrical constraints issued from the occurrence of two fused five-member chelate rings of the tridentate tppz ligand, resulting in N(2)–Re(1)–N(1) and N(2)–Re(1)–N(3) angles of 77.45(9) and 77.58(9)°, respectively (Table 2). The tppz molecule as a whole is far from planarity. The dihedral angles between the mean planes of the two coordinated pyridyl rings and the pyrazine ring are 12.41(14) and 22.41(11)°, while the uncoordinated pyridyl rings are inclined to the pyrazine ring by 67.46(8) and 38.25(12)°. Low energy conformations of the free tppz are predicted to have each pyridyl ring twisted from coplanarity with the pyrazine by roughly 50°.2f
Table 2 The selected bond lengths (Å) and angles (°) for 1
Bond lengths |
Bond angles |
Re(1)–N(1) |
1.971(2) |
N(1)–Re(1)–N(3) |
77.58(9) |
Re(1)–N(3) |
2.096(2) |
N(1)–Re(1)–N(5) |
77.45(9) |
Re(1)–N(5) |
2.084(2) |
N(1)–Re(1)–Cl(1) |
91.44(7) |
Re(1)–Cl(1) |
2.3606(8) |
N(1)–Re(1)–Cl(2) |
179.08(7) |
Re(1)–Cl(2) |
2.3590(9) |
N(1)–Re(1)–Cl(3) |
90.93(7) |
Re(1)–Cl(3) |
2.3597(8) |
N(3)–Re(1)–Cl(1) |
90.69(7) |
|
|
N(3)–Re(1)–Cl(2) |
101.53(7) |
|
|
N(3)–Re(1)–Cl(3) |
90.97(7) |
|
|
N(5)–Re(1)–N(3) |
154.98(10) |
|
|
N(5)–Re(1)–Cl(1) |
88.19(7) |
|
|
N(5)–Re(1)–Cl(2) |
103.44(7) |
|
|
N(5)–Re(1)–Cl(3) |
91.17(7) |
|
|
Cl(2)–Re(1)–Cl(1) |
88.82(3) |
|
|
Cl(2)–Re(1)–Cl(3) |
88.83(3) |
|
|
Cl(3)–Re(1)–Cl(1) |
177.36(3) |
The Re–Cl distances [2.3606(8), 2.3590(9) and 2.3597(8) Å] compare well with the values reported for the related Re(III) complexes with the six-coordinate metal centre in a distorted ReN3Cl3 octahedral geometry (Table S1†). The bond length of Re(III) to the middle tppz-nitrogen atom N(2) [1.971(2) Å] is shorter than the Re(1)–N(1) and Re(1)–N(3) distances [2.084(2) and 2.096(2) Å], a pattern which is usually observed in complexes incorporating tppz-like ligands.14a,17 The shortness of Re–Npyrazine distance within the tppz unit is attributed to a more efficient overlap of the t2g metal orbitals with the π* orbitals of the central pyrazine ring compared to the distal pyridyl groups.
Magnetic properties
It is well known that the octahedral ligand field for 5d metal ions is always strong and so the d4 electronic configuration of the six-coordinate Re(III) ion is defined by a low-spin state 3T1 term (t42), which, in the case of 1 is split by the spin–orbit coupling (SOC) and an axial ligand field as shown in Fig. 3 (hereafter the symbol of the parity will be omitted). SOC splits the 3T1 term into three spin–orbit energy levels (J = 0, 1 and 2, as shown in the central part of Fig. 3), being the non-magnetic singlet, J = 0, the ground state for λ < 0 (λ is the spin–orbit coupling parameter). In the absence of SOC, a tetragonal distortion splits the 3T1 term into an orbital singlet (3A2) and an orbital doublet (2E) separated by an energy gap (Δ) which is defined as positive if the orbital singlet is the lowest (left of Fig. 3) or negative in the reverse situation (right of Fig. 3). Due to the strong spin–orbit coupling and ligand-field acting in the 5d metal ions, both perturbations have to be taken into account simultaneously.
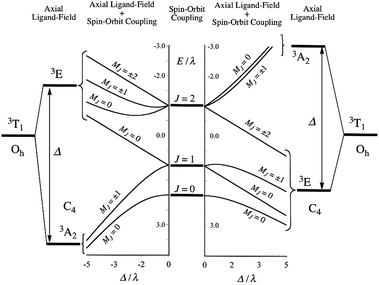 |
| Fig. 3 Splitting of the 3T1 term of the t42 electronic configuration by spin–orbit coupling and a tetragonal ligand-field component. | |
Having these considerations in mind and in order to interpret the magnetic properties of 1, we used the Hamiltonian of eqn (3) (see Appendix)
|
Ĥ = −κλ Ŝ + Δ( z2 − 2/3) + βH(−κ + 2Ŝ)
| (3) |
where the first term is the spin–orbit coupling, the second one accounts for the orbital distortion of the triplet T
1 (T
1 breaks its degeneracy giving an orbital doublet E and an orbital singlet A
2 under a
C4 symmetry group, which are separated by an energy gap
Δ).
κ is the orbital reduction due to covalency.
In the case of a strict octahedral symmetry (Δ = 0), an exact analytical equation for the magnetic susceptibility can be deduced [eqn (4)].
|
 | (4) |
where
x =
λ/
kT.
18 When a lower symmetry is present, the dependence of the susceptibility on temperature is complicated and no analytical expression can be obtained. As shown in
Fig. 3, nine non-degenerate energy levels appear (|
J,
MJ ≥ functions) under a tetragonal ligand-field distortion. The relevant determinants and the energy values for each level are given in the Appendix.
19 These levels are plotted in
Fig. 3 as a function of the
Δ/
λ ratio.
From Fig. 3 and for λ < 0, one can see that the singlet MJ = 0 is always the ground state for any value of Δ (positive or negative) and also that the energy gap between this non-magnetic ground state and the first magnetic excited state (MJ = ±1) is of the same order as |λ|. Due to the strong SOC (λ ≈ −1250 cm−1),18 one can assume that the ground state is the only populated one at room temperature and below (kT < λ). Therefore, the magnetic susceptibility for 1 arises only from the second-order Zeeman effect between the non-magnetic ground state (MJ = 0) and higher magnetic levels (MJ ≠ 0), that is, a temperature-independent paramagnetic susceptibility (χTIP). In this respect, from eqn (4) and for |λ|/kT ≫ 1, one obtains eqn (5):
|
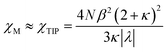 | (5) |
Then, the experimental thermal dependence of the χMT product of 1 below room temperature is expected to follow a straight line which tends to zero when the temperature vanishes (χMT = constant value × T).
Fig. 4 shows a family of χMT curves as a function of temperature for different values of the λ, Δ and κ parameters. These curves are obtained through the Hamiltonian of eqn (3) and using the VPMAG program.20 In all these curves, one can see that the χMT values tend linearly to zero at low temperatures. It is interesting to note that ligand fields of low symmetry whose values are of the same order of magnitude as (or less than) the spin–orbit coupling constant (|Δ| ≤ |λ|) do not much affect the average paramagnetism of the t42 configuration, so that one may largely neglect them in dealing with regular octahedral complexes and use eqn (5) to evaluate the temperature independent paramagnetism. So, if we assume the free–ion parameter λ0 ≈ 1250 cm−1 and an orbital reduction parameter κ ≈ 0.75 (value commonly observed for 5d metal ions),18,21 a value of the χTIP about 3000 × 10−6 cm3 mol−1 for six-coordinate Re(III) complexes can be expected.
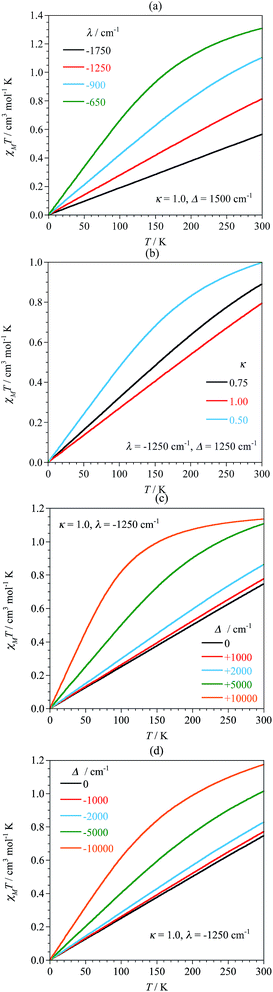 |
| Fig. 4 Theoretical χMT vs. T plots as a function of (a) the spin–orbit parameter (λ), (b) reduction parameter (κ), (c), positive tetragonal field (Δ > 0) and (d) negative tetragonal field (Δ < 0). | |
The χMT versus T plot for 1 (χM is the magnetic susceptibility per mol of Re(III) ions] is shown in Fig. 5. A linear plot is observed, as expected. At room temperature, χMT is equal to 0.93 cm3 mol−1 K and it decreases linearly with temperature, as predicted.
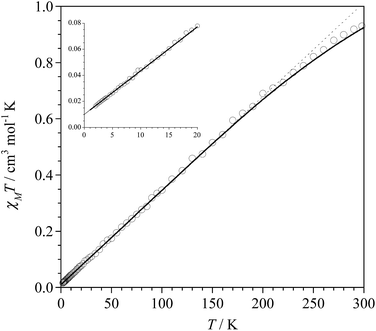 |
| Fig. 5 Temperature dependence of the χMT product for 1: (o) experimental; (solid line) theoretical curve through Hamiltonian of eqn (3) and the best-fit parameters (see text); (dotted line) linear fit from equation χMT = A + BT (see text). The inset shows a detail of the low temperature domain. | |
However, it does not tend to zero when temperature vanishes, but to ca. 0.010 cm3 mol−1 K. This feature may be attributed to the presence of some paramagnetic impurity, most likely due to an oxidation of a very small amount of Re(III) to Re(IV). A satisfactory match of the experimental χMT values is achieved through eqn (3) with the introduction of a paramagnetic impurity (ρ) in the form of eqn (6).
|
χTexp = (1 − ρ)χTRe(III) + ρχTRe(IV)
| (6) |
where

(for
S = 3/2 and
g = 1.85).
Least-squares best-fit parameters through the VPMAG program20 were: λ = −1190(20) cm−1, Δ = 1010(25) cm−1, κ = 0.720(5) and ρ = 0.00625(2) [that is ca. 0.63% of Re(IV) is present in 1]. The corresponding value of the χTIP is 3080 × 10−6 cm3 mol−1. This value is very close to that calculated by eqn (5) and it is also similar to those observed in other Re(III) complexes.18,22 However, in the temperature range explored (1.9–290 K), all these parameters (λ, Δ and κ) are very correlated and it is not possible to obtain unambiguous values for them. Even, the sign for Δ cannot be unambiguously determined. The values of the parameters given above are one of the more reliable set of the best fits. Moreover, because of the large correlation existing between diamagnetism of the ligands (χdia) and the χTIP, the experimental magnetic susceptibilities obtained for 1 were carefully corrected for the diamagnetism of the constituent atoms [χdia = −397 × 10−6 cm3 mol−1] and also for the sample holder.
Finally, it is interesting to note that, for this type of Re(III) complexes, which have large values of λ (λ ≫ kT), the experimental thermal dependence of the χMT product is expected to follow a straight line, χMT = A + BT, where A is the value of χMT corresponding to the occurrence of a small paramagnetic impurity and B is the temperature-independent paramagnetism (χTIP) of Re(III) defined by eqn (5) (the octahedral distortion Δ being neglected). In the case of 1, a fit to a linear plot yields A = 0.00998 cm3 mol−1 K [which corresponds to 0.63% of Re(IV) in 1] and B = 0.003110 cm3 mol−1 (χTIP = 3110 × 10−6 cm3 mol−1). These values are practically the same than those obtained through eqn (3).
Conclusions
The preparative routes of complex 1 whose crystal structure and magnetic properties are described herein demonstrate that tridentate nitrogen donors such as tppz make easy the substitution of the phosphine ligands and the release of the oxo group with reduction of the [ReV
O]3+ core from the [ReOCl3(PPh3)2] complex to the [ReCl3] entity. A deep analysis of the variable-temperature magnetic data of 1 reveals that it is a d4 low-spin species (3T1 ground state) whose magnetic behaviour is dictated by a large spin–orbit coupling [λ = −1190(20) cm−1]. Therefore, the magnetic susceptibility of 1 arises only from the second-order Zeeman effect between the non-magnetic ground state and the higher magnetic levels, the value of the TIP being 3110× 10−6 cm3 mol−1.
Experimental section
Materials
The reagents used to the synthesis were commercially available and they were used without further purification. The [ReCl3(MeCN)(PPh3)2]23 and [ReOCl3(PPh3)2]24 complexes were prepared according to the literature method.
Instrumentation
IR spectrum was recorded on a Nicolet iS5 spectrophotometer in the spectral range 4000–400 cm−1 with the sample in the form of KBr pellets [Fig. S1 in the ESI†]. X-ray powder diffraction (XRPD) measurements [Fig. S2 in the ESI†] were performed on a PANalytical Empyrean X-ray diffractometer using Cu-Kα radiation (λ = 1.5418 Å), in which the X-ray tube was operated at 40 kV and 30 mA ranging from 5 to 50°. The 1H NMR and 13C NMR spectra were recorded at 295 K on a Bruker Advance 500 NMR spectrometer with resonance frequencies of 500 MHz for 1H NMR spectra and 125 MHz for 13C NMR spectra by using DMSO-d6 as solvent and TMS as an internal solvent [Fig. S3 in the ESI†]. The X-ray diffraction data of 1 were collected on Oxford Diffraction four-circle diffractometer Gemini A Ultra with Atlas CCD detector using graphite monochromated Mo Kα radiation (λ = 0.71073 Å) at room temperature. Details concerning crystal data and refinement are given in Table 1. Lorentz, polarization and empirical absorption correction using spherical harmonics implemented in SCALE3 ABSPACK scaling algorithm25 were applied. The structure was solved by the Patterson method using SHELXS97 and refined by full-matrix least-squares on F2 using SHELXL97.26 All the non-hydrogen atoms were refined anisotropically, and hydrogen atoms were placed in calculated positions refined using idealized geometries (riding model) and assigned fixed isotropic displacement parameters, d(C–H) = 0.93 Å, Uiso(H) = 1.2 Ueq(C) (for aromatic); and d(C–H) = 0.96 Å, Uiso(H) = 1.5 Ueq(C) (for methyl). The methyl groups were allowed to rotate about their local threefold axis.
CCDC-1431249 (complex 1) contain the supplementary crystallographic data for this paper.†
Synthesis of the rhenium(III) complex
Preparation of [ReCl3(tppz)]·MeCN (1).
Method A. [ReCl3(MeCN)(PPh3)2] (0.50 g, 0.60 mmol) was added to tppz (0.23 g, 0.60 mmol) in acetonitrile (100 mL) and the reaction mixture was refluxed for 4 h. The resulting solution was reduced in volume to 10 mL and allowed to cool at room temperature. A brown crystalline precipitate of 1 was filtered off and dried in the air. X-ray quality crystals of 1 as brown prisms were obtained by slow recrystallization from acetonitrile, and collected by filtration. Yield: ca. 85%.
Method B. [ReOCl3(PPh3)2] (0.50 g, 0.60 mmol) was added to tppz (0.23 g, 0.60 mmol) in acetonitrile (100 mL) and the reaction mixture was refluxed for 4 h. The resulting solution was reduced in volume to 10 mL and allowed to cool at room temperature. A brown crystalline precipitate of 1 was filtered off and dried in the air. X-ray quality crystals of 1 were obtained by slow recrystallization from acetonitrile, and collected. Yield: ca. 70%.Anal. calcd for C21H16ClN2O6Re (1): C, 41.08; H, 5.77; N, 4.56. Found: C, 40.94; H, 5.71; N 4.64%. 1H NMR (500 MHz, DMSO-d6): δ = 7.69–7.56 (m, 4H), 7.52 (d, J = 19.0 Hz, 2H), 7.43 (d, J = 21.3 Hz, 2H), 7.35 (s, 4H), 7.27 (s, 4H), 2.08 (s, 3H) ppm. 13C NMR (125 MHz, DMSO-d6): δ = 133.7, 133.6, 132.9, 132.7, 132.5, 132.0, 131.9, 130.7, 129.3, 129.2, 118.6, 1.6 ppm. IR (KBr, cm−1): 1598w, 1586w and 1562w [ν(C
Ntppz)] and ν(C
Ctppz)].
Conflict of interest
The authors declare no competing financial interest.
Acknowledgements
This work was supported by the Polish National Science Centre (Grant No. DEC-2012/05/N/ST5/00743 and DEC-2012/07/N/ST5/02213) and the Spanish MICINN (Project CTQ-2013-44844P). Joanna Palion-Gazda is grateful for a scholarship from the DoktoRIS project co-financed by the European Social Fund.
References
- H. A. Goodwin and F. Lions, J. Am. Chem. Soc., 1959, 81, 6415 CrossRef CAS.
-
(a) B. Machura, J. Palion, J. Mroziński, B. Kalinska, M. Amini, M. M. Najafpour and R. Kruszynski, Polyhedron, 2013, 53, 132 CrossRef CAS;
(b) C. Yuste, L. Cañadillas-Delgado, C. Ruiz-Pérez, F. Lloret and M. Julve, Dalton Trans., 2010, 39, 167 RSC;
(c) L. M. Callejo, G. Madariaga, L. Lezama, L. Fidalgo, N. De la Pinta and R. Cortés, Inorg. Chem., 2010, 49, 5353 CrossRef CAS PubMed;
(d) M. Trivedi, D. S. Pandey and N. P. Rath, Inorg. Chim. Acta, 2009, 362, 284 CrossRef CAS;
(e) L. M. Toma, D. Armentano, G. De Munno, J. Sletten, F. Lloret and M. Julve, Polyhedron, 2007, 26, 5263 CrossRef CAS;
(f) C. W. Padgett, W. T. Pennington and T. W. Hanks, Cryst. Growth Des., 2005, 5, 737 CrossRef CAS;
(g) M. Maekawa, T. Minematsu, H. Konaka, K. Sugimoto, T. Kuroda-Sowa, Y. Suenaga and M. Munakata, Inorg. Chim. Acta, 2004, 357, 3456 CrossRef CAS.
- I. Gryca, J. Palion-Gazda, B. Machura, M. Penkala, R. Kruszyński, J. Cano, F. Lloret and M. Julve, Dalton Trans., 2015, 44, 17118 RSC.
- F. H. Allen, Acta Crystallogr., 2002, B58, 380 CAS.
-
(a) S. Rubino, P. Portanova, A. Girasolo, G. Galvaruso, S. Orecchio and G. C. Stoco, Eur. J. Med. Chem., 2009, 44, 1041 CrossRef CAS PubMed;
(b) F. H. Haghighi, H. Hadadzadeh, F. Darabi, Z. Jannesari, M. Ebrahimi, T. Khayamian, M. Salimi and H. A. Rudbari, Polyhedron, 2013, 65, 16 CrossRef CAS.
-
(a) M. Maskus and H. D. Abruña, Langmuir, 1996, 12, 4455 CrossRef CAS;
(b) J. Yuasa and S. Fukuzumi, J. Am. Chem. Soc., 2006, 128, 15976 CrossRef CAS PubMed;
(c) J. Yuasa and S. Fukuzumi, J. Am. Chem. Soc., 2008, 130, 566 CrossRef CAS PubMed;
(d) H. Hadadzadeh, S. R. Hosseinian and S. J. A. Fatemi, Polyhedron, 2009, 28, 2776 CrossRef CAS;
(e) I. Nawrot, B. Machura and R. Kruszyński, CrystEngComm, 2015, 17, 830 RSC.
-
(a) R. Ruminski, J. Kiplinger, T. Cockroft and C. Chase, Inorg. Chem., 1989, 28, 370 CrossRef CAS;
(b) J. P. Collin, P. Lainé, J. P. Sauvage and A. Sour, J. Chem. Soc., Chem. Commun., 1993, 434 RSC;
(c) L. M. Vogler, B. Scott and K. J. Brewer, Inorg. Chem., 1993, 32, 898 CrossRef CAS;
(d) L. M. Vogler and K. J. Brewer, Inorg. Chem., 1996, 35, 818 CrossRef CAS PubMed;
(e) J. D. Lee, L. M. Vrana, E. R. Bullock and K. J. Brewer, Inorg. Chem., 1998, 37, 3575 CrossRef CAS PubMed;
(f) C. M. Hartshorn, N. Daire, V. Tondreau, B. Loeb, T. J. Meyer and P. S. White, Inorg. Chem., 1999, 38, 3200 CrossRef CAS;
(g) C. Metcalfe, S. Spey, H. Adams and J. A. Thomas, J. Chem. Soc., Dalton Trans., 2002, 4732 RSC;
(h) N. Chanda, R. H. Laye, S. Chakraborthy, R. L. Paul, J. C. Jeffery, M. Ward and G. K. Lahiri, J. Chem. Soc., Dalton Trans., 2002, 3496 RSC.
-
(a) M. Graf, H. Stoekli-Evans, A. Escuer and R. Vicente, Inorg. Chim. Acta, 1997, 257, 89 CrossRef CAS;
(b) C. S. Campos-Fernández, B. W. Smucker, R. Clérac and K. R. Dunbar, Isr. J. Chem., 2001, 41, 207 CrossRef;
(c) J. Carranza, C. Brennan, J. Sletten, J. M. Clemente-Juan, F. Lloret and M. Julve, Inorg. Chem., 2003, 42, 8716 CrossRef CAS PubMed;
(d) J. Carranza, J. Sletten, C. Brennan, F. Lloret, J. Cano and M. Julve, Dalton Trans., 2004, 3997 RSC;
(e) G.-H. Hsu, C.-W. Cheng, S.-C. Cheng, S.-H. Lin, H.-H. Wei and C.-J. Lee, Polyhedron, 2005, 24, 487 CrossRef CAS;
(f) C. Yuste, D. Armentano, N. Marino, L. Cañadillas-Delgado, F. S. Delgado, C. Ruiz-Pérez, D. P. Rillema and M. Julve, Dalton Trans., 2008, 1583 RSC.
-
(a) H. W. Richardson and W. E. Hatfield, J. Am. Chem. Soc., 1976, 98, 835 CrossRef CAS;
(b) H. W. Richardson, J. R. Wasson and W. E. Hatfield, Inorg. Chem., 1977, 16, 484 CrossRef CAS;
(c) J. Darriet, M. S. Haddad, E. N. Duesler and D. N. Hendrickson, Inorg. Chem., 1979, 18, 2679 CrossRef CAS;
(d) M. Julve, M. Verdaguer, J. Faus, F. Tinti, J. Moratal, A. Monge and E. Gutiérrez-Puebla, Inorg. Chem., 1987, 26, 3520 CrossRef CAS;
(e) J. A. Real, G. De Munno, M. C. Muňoz and M. Julve, Inorg. Chem., 1991, 30, 2701 CrossRef CAS;
(f) T. Otieno and R. C. Thompson, Can. J. Chem., 1995, 73, 275 CrossRef CAS.
- J. Palion-Gazda, A. Świtlicka-Olszewska, B. Machura, T. Grancha, E. Pardo, F. Lloret and M. Julve, Inorg. Chem., 2014, 53, 10009 CrossRef CAS PubMed.
-
(a) F. A. Cotton, Inorg. Chem., 1998, 37, 5710 CrossRef CAS;
(b) J. Chantler, D. A. Kort, P. E. Fanwick and R. A. Walton, J. Organomet. Chem., 2000, 596, 27 CrossRef CAS;
(c) D. P. Lydon, T. R. Spalding and J. F. Gallagher, Polyhedron, 2003, 22, 1281 CrossRef CAS;
(d) M. Q. Dequeant, R. McGuire Jr, D. R. McMillin and T. Ren, Inorg. Chem., 2005, 44, 6521 CrossRef CAS PubMed;
(e) A. V. Shtemenko, P. Collery, N. I. Shtemenko, K. V. Domasevitch, E. D. Zabitskaya and A. A. Golichenko, Dalton Trans., 2009, 5132 RSC;
(f) M. Q. Dequeant and T. Ren, J. Cluster Sci., 2010, 21, 291 CrossRef CAS;
(g) J. Beck and G. Zink, J. Chem. Crystallogr., 2011, 41, 1185 CrossRef CAS.
-
(a) X. L. R. Fontaine, E. H. Fowles, T. P. Layzell, B. L. Shaw and M. Thornton-Pett, J. Chem.
Soc., Dalton Trans., 1991, 1519 CAS;
(b) C. Pearson and A. L. Beauchamp, Inorg. Chim. Acta, 1995, 237, 13 CrossRef CAS;
(c) F. Tisato, F. Refosco, C. Bolzati, A. Cagnolini, S. Gatto and G. Bandoli, J. Chem. Soc., Dalton Trans., 1997, 1421 RSC;
(d) V. Bertolasi, A. Marchi, L. Marvelli, R. Rossi, C. Bianchini, I. de los Rios and M. Peruzzini, Inorg. Chim. Acta, 2002, 327, 140 CrossRef CAS;
(e) B. Machura, J. O. Dzięgielewski and J. Kusz, Inorg. Chem. Commun., 2003, 6, 786 CrossRef CAS;
(f) B. Machura, R. Kruszyński and M. Jaworska, J. Mol. Struct., 2005, 740, 107 CrossRef CAS;
(g) I. Klopsch, M. Finger, C. Würtele, B. Milde, D. B. Werz and S. Schneider, J. Am. Chem. Soc., 2014, 136, 6881 CrossRef CAS PubMed.
-
(a) P. Perez-Lourido, J. Romero, J. A. Garcia-Vazquez, A. Sousa, K. P. Maresca and J. Zubieta, Inorg. Chem., 1999, 38, 1511 CrossRef CAS;
(b) D. Gambinoa, J. Beniteza, L. Oteroa, E. Kremera, E. J. Baranb and O. E. Piro, Polyhedron, 1999, 18, 2099 CrossRef;
(c) F. Mevellec, A. Roucoux, N. Noiret, H. Patin, F. Tisato and G. Bandoli, Inorg. Chem. Commun., 1999, 2, 230 CrossRef CAS;
(d) A. R. Cowley, J. R. Dilworth, P. S. Donnelly and J. Woollard-Shore, Dalton Trans., 2003, 748 RSC;
(e) N. Lepareur, F. Mevellec, N. Noiret, F. Refosco, F. Tisato, M. Porchia and G. Bandoli, Dalton Trans., 2005, 2866 RSC;
(f) A. Shellenbarger-Jones, T. Nicholson, W. M. Davis, A. Davison and A. G. Jones, Inorg. Chim. Acta, 2005, 358, 3559 CrossRef CAS;
(g) N. Salvarese, N. Morellato, A. Venzo, F. Refosco, A. Dolmella and C. Bolzati, Inorg. Chem., 2013, 52, 6365 CrossRef CAS PubMed;
(h) N. Salvarese, A. Dolmella, F. Refosco and C. Bolzati, Inorg. Chem., 2015, 54(4), 1634 CrossRef CAS PubMed.
-
(a) J. Rall, F. Weingart, D. M. Ho, M. J. Heeg, F. Tisato and E. Deutsch, Inorg. Chem., 1994, 33, 3442 CrossRef CAS;
(b) O. Arp and W. Preetz, Z. Anorg. Allg. Chem., 1996, 622, 219 CrossRef CAS;
(c) C. Pearson and A. L. Beauchamp, Can. J. Chem., 1997, 75, 220 CrossRef CAS;
(d) F. Tisato, F. Refosco, A. Dolmella and G. Bandoli, Polyhedron, 1998, 17, 3947 CrossRef CAS;
(e) S. Fortin, P.-L. Fabre, M. Dartiguenave and A. L. Beauchamp, J. Chem. Soc., Dalton Trans., 2001, 3520 RSC;
(f) S. H. Meiere, B. C. Brooks, T. B. Gunnoe, E. H. Carrig, M. Sabat and W. D. Harman, Organometallics, 2001, 20, 3661 CrossRef CAS;
(g) S. Bhattacharyya, I. Chakraborty, B. K. Dirghangi and A. Chakravorty, Inorg. Chem., 2001, 40, 286 CrossRef CAS PubMed;
(h) S. R. Banerjee, L. Wei, M. K. Levadala, N. Lazarova, V. O. Golub, C. J. O'Connor, K. A. Stephenson, J. F. Valliant, J. W. Babich and J. Zubieta, Inorg. Chem., 2002, 41, 5795 CrossRef CAS PubMed;
(i) S. Das, I. Chakraborty and A. Chakravorty, Polyhedron, 2003, 22, 901 CrossRef CAS;
(j) B. Machura, M. Jaworska and R. Kruszynski, Polyhedron, 2004, 23, 2005 CrossRef CAS;
(k) B. Machura, R. Kruszynski, M. Jaworska and P. Lodowski, Polyhedron, 2005, 24, 701 CrossRef CAS;
(l) M. N. Sokolov, N. E. Federova, E. V. Peresypkina, R. Pätow, V. E. Fedorov and D. Fenske, Inorg. Chim. Acta, 2005, 358, 3914 CrossRef CAS;
(m) B. Machura, R. Kruszyński, J. Kusz, J. Kłak and J. Mroziński, Polyhedron, 2007, 26, 4427 CrossRef CAS;
(n) B. Machura, M. Wolff and I. Gryca, Inorg. Chem. Commun., 2010, 13, 904 CrossRef CAS.
-
(a) F. Loiseau, F. Connac, Y. Lucchese, M. Dartiguenave, S. Fortin, A. L. Beauchamp and Y. Coulais, Inorg. Chim. Acta, 2000, 306, 94 CrossRef CAS;
(b) M. N. Sokolov, N. E. Fyodorova, R. Paetow, D. Fenske, A. G. Ravelo, D. Y. Naumov and V. E. Fedorov, Inorg. Chim. Acta, 2007, 360, 2192 CrossRef CAS;
(c) S. Basak, A. Mondal, D. Chopra and K. K. Rajak, Polyhedron, 2007, 26, 3465 CrossRef CAS;
(d) B. Machura, R. Kruszyński, J. Mroziński and J. Kusz, Polyhedron, 2008, 27, 1739 CrossRef CAS;
(e) P. Mayer, K. C. Potgieter and T. I. A. Gerber, Polyhedron, 2010, 29, 1423 CrossRef CAS.
- H. Spies, M. Glaser, F. E. Hahn, T. Lfigger and D. Scheller, Inorg. Chim. Acta, 1995, 232, 235 CrossRef CAS.
- G. Bohm, K. Wieghardt, B. Nuber and J. Weiss, Inorg. Chem., 1991, 30, 3464 CrossRef.
- A. Earnshaw, B. N. Figgis, J. Lewis and R. D. Peacock, J. Chem. Soc., 1961, 3132 RSC.
- B. N. Figgis, J. Lewis, F. E. Mabbs and G. A. Webb, J. Chem. Soc. A, 1966, 1411 RSC.
- J. Cano, VPMAG package, University of Valencia, Valencia, Spain, 2003 Search PubMed.
-
(a) A. B. P. Lever, Inorganic Electronic Spectroscopy, Elesevier, Amsterdam, 1968 Search PubMed;
(b) I. B. Bersuker, Electronic Structure and Properties of Transition Metal Ions, John Wiley & Sons, New York, 1996 Search PubMed.
-
(a) H. P. Gunz and G. J. Leigh, J. Chem. Soc. A, 1971, 2229 RSC;
(b) C. E. Uzelmeier, S. L. Bartley, M. Formigué, R. Rogers, G. Grandinetti and K. R. Dunbar, Inorg. Chem., 1998, 37, 6706 CrossRef CAS PubMed;
(c) S. R. Banerjee, L. Wei, M. K. Levadala, N. Lazarova, V. O. Golub, C. J. O'Connor, K. A. Stephenson, J. F. Valliant, J. W. Babich and J. Zubieta, Inorg. Chem., 2002, 41, 5795 CrossRef CAS PubMed;
(d) B. Machura, J. Mrozinski, R. Kruszenski and J. Kusz, Polyhedron, 2009, 28, 2821 CrossRef CAS.
- G. Rouschias and G. Wilkinson, J. Chem. Soc. A, 1967, 993 RSC.
- G. Rouschias and G. Wilkinson, J. Chem. Soc. A, 1966, 465 RSC.
- CrysAlis RED, Oxford Diffraction Ltd., 1.171.33.55 Search PubMed.
- G. M. Sheldrick, Acta Crystallogr., 2008, A64, 112 CrossRef PubMed.
Footnote |
† Electronic supplementary information (ESI) available: Magneto-structural data for Re(III) complexes (Table S1), IR (Fig. S1), 1H and 13C NMR spectra (Fig. S3), and XPRD (Fig. S2) for 1, Appendix [energy levels for six-coordinate Re(III)] and X-ray crystallographic tables (CIF for 1). CCDC 1431249. For ESI and crystallographic data in CIF or other electronic format see DOI: 10.1039/c5ra21466a |
|
This journal is © The Royal Society of Chemistry 2015 |