DOI:
10.1039/C5RA20449F
(Paper)
RSC Adv., 2015,
5, 100579-100588
Chemistry of heterocyclic-2-thiones: in situ generated 1-(4,5-dihydro-3-alkyl-imidazolidin-2-yl)-3-alkyl-imidazolidine-2-thione and triiodide form novel mixed valent CuI–II/CuII complexes†
Received
2nd October 2015
, Accepted 13th November 2015
First published on 16th November 2015
Abstract
Equimolar reaction of copper(I) iodide with N-methyl-imidazolidine-2-thione (imdzSH-Me) in acetonitrile under aerobic conditions involved C–S rupture and formation of C–N bond between two heterocyclic rings giving rise to the formation of 1-(4,5-dihydro-3-methyl-imidazolidin-2-yl)-3-methyl-imidazolidine-2-thione (L-NMe) which has yielded black prismatic crystals of mixed valent trinuclear complex, [CuI2CuII(κ2-N,S-L-NMe)(κ1N,μ-S-L-NMe)(μ-I)2(κ1-I)2] 1 along with formation of CuSO4·5H2O. A similar reaction of N-n-propyl-imidazolidine-2-thione (imdzSH-Prn) with copper(I) iodide has formed white {Cu(μ-S-imdzSH-Prn)2Cu(μ-I)2}n 2 and brown [Cu3(κ1N,μ-S-L-NPrn)2(μ-I)2(κ1-I3)2]n 3 polymers. The N-n-propyl-imidazolidine-2-thione ligand is unchanged in 2, but it changed into 1-(4,5-dihydro-3-propyl-imidazolidin-2-yl)-3-propyl-imidazolidine-2-thione (L-NPrn) in polymer 3. Finally, in reaction of copper(I) iodide with N-n-butyl-imidazolidine-2-thione (imdzSH-Bun), the thio-ligand changed into 1-(4,5-dihydro-3-butyl-imidazolidin-2-yl)-3-butyl-imidazolidine-2-thione (L-NBun) which coordinated to the metal centre and formed black compound [Cu(κ2-N,S-L-NBun)2](I3)2 4. The formation of in situ generated new ligands, L-NR (R = Me, Prn and Bun) coordinated in 1, 3 and 4 occurs in the same manner involving C–S rupture, fusion of two N-alkyl-imidazolidine-2-thione rings and formation of new C–N bonds. Compounds 1–4 have been characterized with the help of analytical data, IR spectroscopy, X-ray crystallography and ESI-mass studies.
Introduction
Coordination chemistry of heterocyclic-2-thiones such as pyridine-2-thiones, pyrimidine-2-thiones, 1,3-thiazolidine-2-thiones, imidazolidine-2-thione etc. has been intensively explored and is well reviewed by Raper and other authors.1–6 The impetus to research in this area is owing to the structural diversity engineered due to the presence of both hard Lewis base (N) and soft Lewis base donor atoms in the thio-pyridine or heterocyclic thione rings,1–14 and relevance of their metal complexes in the biological systems.1–7,15 The thio-ligands based on imidazolidine-2-thione are an interesting class of coordinating ligands towards copper(I). They have formed mononuclear,16–23 dinuclear,21–23 and polynuclear10,24 complexes with copper(I) halides and the geometry around each metal center is either distorted trigonal planar or distorted tetrahedral.
Recently it was observed that imidazolidine-2-thione (imdzSH, Scheme 1) reacted with copper(I) chloride/bromide in dimethyl sulfoxide (or DMF) and was converted into 2,2′-thio-di-2-imidazoline involving C–S rupture which subsequently formed a sulfate bridged polymer, [CuII{κ2-N,N-L1}(μ-O,OSO2)(κ1-OH2)]n (Scheme 1, L1).25 Similarly, copper(I) bromide converted 1-methyl-imidazoline-2-thione in dimethyl sulfoxide into 1,1′-dimethyl-2,2′-di-imidazolylsulfide which formed a sulfate chelated paramagnetic complex, [CuII{κ2-N,N-L2}(κ2-O,OSO2)(κ1-OH2)] (Scheme 1, L2).25 Furthermore, reaction of copper(I) bromide with 1-methyl-imidazoline-2-thione in acetonitrile–chloroform converted thio-ligand into 2-bromo-1-methyl-imidazole (L3) which then coordinated to CuII in its cluster [Cu4II(κ1-N-L3)4(μ4-O)(μ-Br)6].26 The thio-ligand 1,3-thiazolidine-2-thione (tzdSH, Scheme 1) in acetonitrile in reaction with copper(I) chloride/bromide or CuBF4 was transformed into 3-(2-thiazolin-2-yl)thiazolidine-2-thione (Scheme 1, L4) through C–S bond cleavage and formation of a C–N bond between two heterocyclic rings. The ligand L4 yielded three coordinate, [CuX(κ2-N,S-L4)] (X = Cl, Br) and four coordinate, [Cu(κ2-N,S-L4)2](BF4) complexes.27
 |
| Scheme 1 (a) Heterocyclic-2-thione ligands; (b) in situ generated thio-ligands. | |
The activation, cleavage and transformation of C–S bonds into new set of ligands using transition metal compounds have found wide interest in petroleum industry, in the synthetic organic chemistry, and also shown relevance in the biological reactions.28–30 In continuation of our recent research reports in the chemistry of reactivity of imidazolidine-2-thione, 1-methyl-imidazoline-2-thione and 1,3-thiazolidine-2-thione towards copper especially related to C–S rupture,21,25–27,31,32 in this paper chemical reactivity of N-alkyl-imidazolidine-2-thiones (imdzSH-Me, imdzSH-Prn and imdzSH-Bun, Scheme 1) towards copper(I) iodide is investigated. It is added here that imidazolidine-2-thione (imdzSH) with copper(I) iodide in acetonitrile–chloroform yielded polymeric products displaying bond isomerism with no C–S rupture.33a However, under treatment of mild oxidizing agents, imidazolidine-2-thione (imdzSH, Scheme 1) gives analogue 1-(4,5-dihydro-1H-imidazolidin-2-yl)imidazolidine-2-thione.33b In this paper, novel compounds (1, 3, 4) with in situ generated new thio-ligands L-NR (Scheme 2) as well as complex 2 (unchanged imdzSH-Prn) are reported. Compounds are characterized with the help of analytical data, IR spectroscopy, X-ray crystallography and ESI-mass studies.
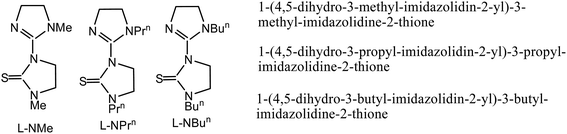 |
| Scheme 2 In situ generated thio-ligands. | |
Results and discussion
Synthesis and IR spectroscopy
Equimolar reaction of copper(I) iodide with N-methyl-imidazolidine-2-thione (Scheme 1) in acetonitrile under aerobic conditions formed a polymer, {Cu4(μ3-I)2(μ-I)2(μ-S-imdzSH-Me)2(κ1-S-imdzSH-Me)2}n, after a few days as reported earlier.27 In this polymer, imdzSH-Me ligand coordinates to the metal center through its sulfur donor atom. After separation of the polymer, the color of the filtrate changed to brownish green in next 2–4 days and black prismatic crystals of [CuI2CuII(κ2-N,S-L-NMe)(κ1N,μ-S-L-NMe)(μ-I)2(κ1-I)2] 1 were obtained along with the formation of CuSO4·5H2O. It is interesting to note here that the thio-ligand, N-methyl-imidazolidine-2-thione involves in situ generation of 1-(4,5-dihydro-3-methyl-imidazolidin-2-yl)-3-methyl-imidazolidine-2-thione (L-NMe) which then coordinates to copper metal center in compound 1 (Scheme 3). A similar reaction of N-n-propyl-imidazolidine-2-thione(imdzSH-Prn) with copper(I) iodide has formed polymers, {Cu(μ-S-imdzSH-Prn)2Cu(μ-I)2}n 2(white) and [Cu3(κ1N,μ-S- L-NPrn)2(μ-I)2(κ1-I3)2]n 3 (brown). The N-n-propyl-imidazolidine-2-thione ligand is unchanged in 2 (Scheme 1), but it changed into 1-(4,5-dihydro-3-propyl-imidazolidin-2-yl)-3-propyl-imidazolidine-2-thione (L-NPrn) which then formed polymer 3 (Scheme 3). Finally in reaction of copper(I) iodide with N-n-butyl-imidazolidine-2-thione (imdzSH-Bun), the thio-ligand changed into 1-(4,5-dihydro-3-butyl-imidazolidin-2-yl)-3-butyl-imidazolidine-2-thione (L-NBun) which formed black compound [Cu(κ2-N,S-L-NBun)2]·2I3 4 (Scheme 3). The formation of in situ generated new ligands, L-NR (R = Me, Prn and Bun) coordinated in 1, 3 and 4 occurs in the same manner involving C–S rupture, and formation of C–N bonds via fusion of two N-alkyl-imidazolidine-2-thione rings.
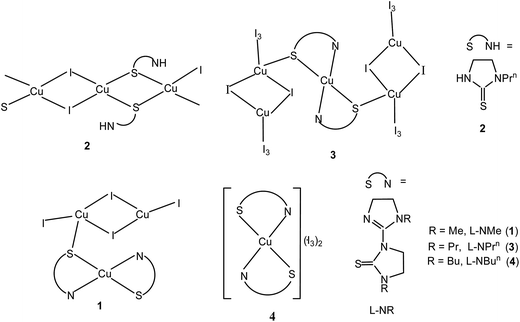 |
| Scheme 3 A view of bonding pattern of thio-ligands in complexes 1–4. | |
The IR spectra of free N-alkyl-imidazolidine-2-thiones (imdzSH-Me, imdzSH-Prn, imdzSH-Bun) (Scheme 1 for thio-ligands) reveal characteristic broad peaks due to ν(N–H) group at 3196m, 3204m and 3193m cm−1 respectively. In complex 2 it appears at 3278s cm−1 suggesting the ligand HL-Prn coordinating to the metal center as neutral ligand, while in other complexes 1, 3 and 4, the absence of ν(N–H) bands indicates deprotonation of –NH hydrogen of thio-ligands. Another diagnostic band is due to ν(C–S) which shifts to low energy regions at 1114s, 1190m, 1134m and 1113m cm−1 in complexes 1–4 respectively (cf. free ligands: imdzSH-Me, 1200s; imdzSH-Prn, 1195s and imdzSH-Bun, 1190m cm−1). The greater ν(C–S) shifts in complexes 1, 3 and 4 is due to the transformation of original ligands into new type of ligands as shown in Scheme 3. The low ν(C–S) shift in complex 2 supports that imdzSH-Prn thio-ligand is unchanged.
Molecular structures
The crystal data of complexes 1–4 are given in Table 1 while important bond parameters are placed in Table 2. Complexes crystallized in orthorhombic (1), monoclinic (2) and triclinic (3, 4) crystal systems in space groups Pbca (1), P21/n (2) and P
(3, 4) respectively. The structures of these compounds are described as follows.
Table 1 Crystallographic data for complexes 1–4
|
1 |
2 |
3 |
4 |
Empirical formula |
C16H28Cu3I4N8S2 |
C6H12CuIN2S |
C24H44Cu3I8N8S2 |
C28H52CuI6N8S2 |
Molecular mass |
1094.80 |
334.68 |
1714.61 |
1389.83 |
T (K) |
100(2) |
110(2) |
100(2) |
100(2) |
Crystal system |
Orthorhombic |
Monoclinic |
Triclinic |
Triclinic |
Space group |
Pbca |
P21/n |
P![[1 with combining macron]](https://www.rsc.org/images/entities/char_0031_0304.gif) |
P![[1 with combining macron]](https://www.rsc.org/images/entities/char_0031_0304.gif) |
a (Å) |
15.797(1) |
5.659(1) |
7.8636(4) |
8.3323(4) |
b (Å) |
18.075(1) |
13.432(3) |
9.7353(5) |
11.8411(5) |
c (Å) |
19.987(1) |
13.249(3) |
15.513(1) |
12.396(1) |
α (°) |
90 |
90 |
76.049(2) |
65.562(2) |
β (°) |
90 |
100.013(3) |
84.558(2) |
81.020(2) |
γ (°) |
90 |
90 |
69.582(2) |
74.418(2) |
V (Å3) |
5706.8(5) |
991.7(4) |
1080.06(10) |
1071.13(9) |
Z |
8 |
4 |
1 |
1 |
D (Mg m−3) |
2.548 |
2.242 |
2.636 |
2.155 |
Reflections collected |
173 860 |
11 382 |
19 316 |
60 825 |
Unique reflections |
5851 [Rint = 0.0662] |
2041 [Rint = 0.0458] |
8249 [Rint = 0.0483] |
5360 [Rint = 0.0495] |
Reflns. with [I > 2σ(I)] |
4923 |
1871 |
6657 |
4531 |
R1 |
0.0239 |
0.0279 |
0.0369 |
0.0253 |
wR2 (Rindices) |
0.0519 |
0.0716 |
0.0764 |
0.0512 |
R1 |
0.0329 |
0.0313 |
0.0526 |
0.0353 |
wR2 (all data) |
0.0545 |
0.0726 |
0.0831 |
0.0556 |
Table 2 Bond distances (Å) and bond angles (°) in complexes
[CuI2CuII(κ2-N,S-L-NMe)(κ1N,μ-S-L-NMe)(μ-I)2(κ1-I)2] 1 |
Cu1–N20 |
1.956(3) |
Cu2–I1 |
2.759(1) |
Cu1–N30 |
1.956(3) |
Cu2–I4 |
2.695(1) |
Cu1–S11 |
2.334(1) |
Cu3–I3 |
2.522(1) |
Cu1–S21 |
2.380(1) |
Cu3–I4 |
2.566(1) |
Cu2–S21 |
2.387(1) |
Cu3–I1 |
2.578(1) |
Cu2–I2 |
2.545(1) |
Cu2⋯Cu3 |
2.871(1) |
I1⋯I4 |
4.3199(4) |
S–C1; S–C21 |
1.692(4), 1.713(4) |
N20–Cu1–N30 |
178.26(12) |
I2–Cu2–I4 |
113.73(2) |
N20–Cu1–S11 |
90.20(9) |
I2–Cu2–I1 |
113.10(2) |
N30–Cu1–S21 |
88.91(9) |
I4–Cu2–I1 |
104.77(2) |
N20–Cu1–S21 |
90.73(9) |
Cu1–S21–Cu2 |
90.63(3) |
N30–Cu1–S11 |
90.26(9) |
I3–Cu3–I4 |
124.16(2) |
S11–Cu1–S21 |
176.73(4) |
I3–Cu3–I1 |
120.40(2) |
S21–Cu2–I2 |
122.00(3) |
I4–Cu3–I1 |
114.25(2) |
S21–Cu2–I4 |
94.94(3) |
Cu3–I1–Cu2 |
64.99(1) |
S21–Cu2–I1 |
105.77(3) |
Cu3–I4–Cu2 |
66.10(2) |
![[thin space (1/6-em)]](https://www.rsc.org/images/entities/char_2009.gif) |
[Cu(μ-S-imdzSH-Prn)(μ-I)]n 2 |
Cu1–S1 |
2.333(1); 2.426(2) |
Cu1⋯Cu1′ |
2.735(1) (Cu2I2) |
Cu1–I1 |
2.631(1); 2.669(1) |
Cu1⋯Cu1′′ |
2.948(2) (Cu2S2) |
C–S |
1.713(5) |
S1⋯S1′′ |
3.737(3) |
I1⋯I1′ |
4.5399(11) |
S1–Cu1–S1′′ |
103.48(5) |
S1′′–Cu1–I1′ |
102.39(4) |
S1–Cu1–I1 |
106.42(4) |
I1–Cu1–I1′ |
117.86(3) |
S1–Cu1–I1′ |
119.3(4) |
Cu1–S1–Cu1′′ |
76.52(5) |
S1′′–Cu1–I1 |
105.95(4) |
Cu1–I1–Cu1′ |
62.14(3) |
![[thin space (1/6-em)]](https://www.rsc.org/images/entities/char_2009.gif) |
[Cu3(κ1N,μ-S-L-NPrn)2(μ-I)2(κ1-I3)2]n (3) |
Cu1–N10 |
1.961(5) |
Cu2–I1 |
2.636(1) |
Cu1–N10a |
1.961(5) |
Cu2–I1b |
2.653(1) |
Cu1–S1 |
2.3824(16) |
Cu2–S1c |
2.390(2) |
Cu1–S1a |
2.3824(16) |
Cu2⋯Cu2b |
2.887(2) |
Cu1⋯I1 |
3.2855(4) |
I2–I3 |
3.039(1) |
Cu1⋯I1a |
3.2855(4) |
I3–I4 |
2.816(1) |
Cu2–I2 |
2.5934(10) |
C1⋯S1c |
1.711(6) |
N10–Cu1–N10a |
180 |
S1c–Cu2–I2 |
113.88(5) |
S1–Cu1–S1a |
180 |
S1c–Cu2–I1b |
97.83(5) |
I1⋯Cu1⋯I1a |
180 |
S1c–Cu2–I1 |
105.10(5) |
N10–Cu1–S1 |
89.59(16) |
I2–Cu2–I1 |
110.56(3) |
N10a–Cu1–S1a |
89.59(16) |
I2–Cu2–I1b |
114.41(3) |
N10a–Cu1–S1 |
90.41(16) |
I1–Cu2–I1b |
113.83(3) |
N10–Cu1–S1a |
90.41(16) |
Cu2–I1–Cu2b |
66.17(3) |
![[thin space (1/6-em)]](https://www.rsc.org/images/entities/char_2009.gif) |
[Cu(κ2-N,S-L-NBun)2]·2I3 4 |
Cu1–N21 |
1.945(2) |
Cu1–S1 |
2.378(1) |
Cu1–N21a |
1.945(2) |
Cu1–S1a |
2.378(1) |
C–S1 |
1.691(3) |
C–S1 |
1.691(3) |
N21–Cu1–S1 |
88.45(7) |
N21a–Cu1–S1 |
88.45(7) |
N21–Cu1–S1a |
91.55(7) |
N21–Cu1–N21a |
180 |
N21a–Cu1–S1a |
91.55(7) |
S1–Cu1–S1a |
180 |
I1–I2 |
2.938(1) |
I2–I3 |
2.906(1) |
Mono-and tri-nuclear complexes (1, 4)
The elemental analysis of complex 1 conformed to the composition C16H28Cu3I4N8S2 and its X-ray crystal structure determination has revealed the formation of a mixed-valent(CuI/CuII) trinuclear cluster [CuI2CuII(κ2-N,S-L-NMe)(κ1N,μ-S-L-NMe)(μ-I)2(κ1-I)2] 1. In this cluster, three copper metal centers are differently coordinated: Cu1 is bonded to two N,S-chelating L-NMe thio-ligands with N and S donor atoms in trans-coordination; Cu2 is bonded to one μ-S, two μ-I and one terminal iodine atoms and Cu3 is bonded to two μ-I, one terminal iodine atoms. It shows Cu1 and Cu2 are tetracoordinated and Cu3 is 3-coordinated (Fig. 1). Hence compound 1 can be considered to consist of four coordinated mononuclear [CuII(κ2-N,S-L-NMe)2]2+ and three coordinated dinuclear [CuI2I4]2− species which are joined together through one coordinated sulfur donor atom. In Cu2I2 core, Cu2⋯Cu3 contact is 2.871(7) Å, which is close to twice the sum of the van der Waals radius of Cu atoms, viz. 2.80 Å.34 The Cu–I bond distances in Cu(μ-I)2Cu core {2.759(1), 2.695(1), 2.578(1), 2.566(1) Å} are unequal and the 4-membered ring does not form a parallelogram. Terminal Cu–I bond distances, 2.545(1) and 2.522(1) Å, as expected are shorter than bridging Cu–I distances. These Cu–I bond distances are smaller than the sum of the ionic radii of copper and iodine {Cu+, I−, 2.97 Å}.34 The geometry around Cu2 is distorted trigonal planar (bond angles, 114 to 124°) and that around Cu3 is distorted tetrahedral (bond angles, 94 to 122°). The angles within the Cu(μ-I)2Cu core are 65–66° and 104–114° at bridging iodine (I1/I4) and copper metal (Cu2/Cu3) centers respectively.
 |
| Fig. 1 Molecular structure of [CuI2CuII(κ2-N,S-L-NMe)(κ1N,μ-S-L-NMe)(μ-I)2(κ1-I)2] 1. | |
At four-coordinated Cu1, the Cu1–S11 terminal bond distance is 2.334(1), the Cu–S distances with bridging sulfur are unequal {Cu1–S21, 2.380(1); S21–Cu2, 2.387(1) Å} but Cu1–N distances 1.956(3) Å are same. The trans bond angles {S–Cu1–S, 176.34(4)°, N–Cu1–N, 178.26(12)°} are close to 180° and various angles around Cu1 vary in the range, 88.91(9)–90.73(9)° which suggest that the geometry around Cu1 is nearly square planar. The angle Cu1–S21–Cu2 of 90.63(3)° suggests that the plane of Cu(μ-I)2Cu core is nearly parallel to that of square planar trans-CuN2S2 core.
The in situ generated thio-ligand, 1-(4,5-dihydro-3-butyl-imidazolidin-2-yl)-3-butyl-imidazolidine-2-thione(L-NBun) gave mononuclear CuII complex, [Cu(κ2-N,S-L-NBun)2]·2I3 4 (Fig. 2). Copper is bonded to two N,S – chelated trans-related L-NBun ligands at equal Cu–S and Cu–N distances of 2.378(1) and 1.945(2) Å respectively. The trans bond angles, N–Cu–N and S–Cu–S, of 180° each as well as the around Cu in the range, 88.45(7) to 91.55(7)° reveal nearly square planar geometry around the metal center. The tri-iodide ion is lying outside the coordination sphere and has I1–I2 and I2–I3 bond distances of 2.938(1) and 2.906(1) Å respectively which are longer than twice the sum of covalent radius of iodine atom, 2.66 Å.34 The I1–I2–I3 angle of 176.65(1)° suggest nearly linear triiodide anion. The bond parameters of [Cu(κ2-N,S-L-NBun)2]2+ cation are similar to that of complex 1.
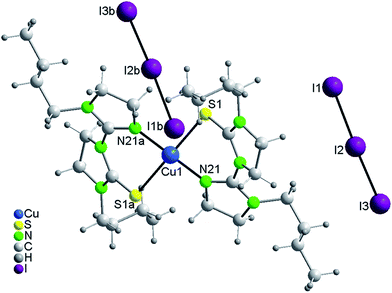 |
| Fig. 2 Molecular structure of [Cu(κ2-N,S-L-NBun)2]·2I3 4. | |
Polynuclear complexes (2, 3)
Complex 2 has empirical composition {CuI(imdzSH-Prn)} and its X-ray structure determination has shown that it is a 1D polymer, {Cu(μ-S-imdzSH-Prn)2Cu(μ-I)2}n (Fig. 3). The thio-ligand imdzSH-Prn and iodide ligand coordinate as μ-S bridging or μ-I bridging modes respectively. This polymer is built by alternate Cu(μ-I)2Cu and Cu(μ-S)2Cu cores with the Cu⋯Cu distances of 2.735(1) and 2.948(2) Å respectively in iodine and sulfur bridged cores. The Cu⋯Cu distance in Cu(μ-I)2Cu core is less than twice the sum of van der Waals radius of Cu atoms (viz. 2.80 Å),34 and it is longer in Cu(μ-S)2Cu core.25 The S⋯S and I⋯I contacts of 3.737(3) and 4.540(1) Å respectively are more than twice the sum of van der Waals' radius of S(1.80 + 1.80 = 3.60 Å) or iodine (3.90 to 4.24 Å).34 Each of four membered Cu2I2 and Cu2S2 rings is centrosymmetric and mutually perpendicular to each other and the polymer extends to give 1D, twisted ribbon like chains running parallel to the a-axis. The unequal Cu–S distances {2.427(1), 2.333(1) Å} of Cu(μ-S)2Cu core form a parallelogram. The angles at sulfur and Cu metal atoms within the Cu(μ-S)2Cu core {76.52(5) and 103.48(5)° respectively} are different from those found in similar polymer, viz.,{Cu(μ-S-imzSH)2Cu(μ-I)2}n (cf.71.95(3)° at S; 108.03(3)° at Cu; imzSH = imidazoline-2-thione) reported in literature.35 Similarly, the unequal Cu–I bond distances {2.669(1), 2.631(1) Å} in Cu(μ-I)2Cu core form a parallelogram and the angles of 62.14(3), 117.86(3)° at I and Cu atoms respectively within the Cu(μ-I)2Cu core are comparable to those found in the polymer, {Cu(μ-S-imzSH)2Cu(μ-I)2}n as mentioned above.35
 |
| Fig. 3 Molecular structure of polymeric {Cu(μ-S-imdzSH-Prn)2Cu(μ-I)2}n 2. | |
The analytical data of complex 3 supported the composition C24H44Cu3I8N8S2 and its X-ray crystal structure revealed it to be a mixed-valent polynuclear complex, [Cu3(κ1N,μ-S-L-NPrn)2(μ-I)2(κ1-I3)2]n 3 (Fig. 4). The formation of new ligand in this polymer occurs in the same way as it does in complexes 1 or 4 described above. Polymer 3 appears to be formed through interlinking of two basic moieties: [CuII(κ1N,κ1S-L-NPrn)2]2+ (A) and {CuI2(μ-I)2(κ1-I3)2}2− (B). Here the coordinated sulfur atom of moiety A binds to one Cu atom of B moiety and second Cu atom of B moiety binds to sulfur atom another A moiety (ABAB…pattern) and this process continues for the generation of polymer 3. Thus the polymer 3 has μ3-N,S-L-NPrn, μ-I, and κ1-I3 coordinating moieties (see Scheme 3). From Fig. 4, it can be seen that there are two basic four-coordinated CuN2(μ-S)2 and four coordinated Cu(μ-I)2(κ1-I3)(μ-S) cores. In the CuN2(μ-S)2 core metal is divalent (CuII) and in Cu(μ-I)2(κ1-I3)(μ-S) core metal is univalent (CuI). The divalent CuII is bonded to two nitrogen and two sulfur atoms at same bond distances in each case {Cu1–N10 = 1.961(5); Cu1–S1 = 2.3824(16). Similarly the trans bond angles are equal: S–Cu1–S = 180; N–Cu1–N = 180°, while other bond angles around Cu1 metal center fall in the close range, 89 to 90° suggesting nearly square planar geometry. The univalent CuI is bonded to two μ-I, one κ1-I3 and one μ-sulfur atoms at bond distances of 2.636(1)[Cu2–I1], 2.653(1)[Cu2–I1b], 2.593(1)[Cu2–I2] and 2.390(2) Å[Cu2–S1c] and the angles around Cu2 in the range, 97–115° suggest a distorted tetrahedral geometry. The coordination of triiodide affects its distances, namely, I2–I3 gets longer {3.039(1) Å} while I3–I4 gets shorter {2.816(1) Å} than the uncoordinated triiodide as observed in complex 4. The Cu⋯Cu distance {Cu2⋯Cu2b = 2.887(2) Å} in Cu(μ-I)2Cu core is close to twice the sum of the van der Waals radius of Cu atoms (viz. 2.80 Å).34 The angles within the Cu(μ-I)2Cu core are 66.17(3) and 113.83(3)° at I and Cu atoms respectively which are similar to those found in compound 2.
 |
| Fig. 4 Molecular structure of polymeric [Cu3(κ1N,μ-S-L-NPrn)2(μ-I)2(κ1-I3)2]n 3. | |
ESI-mass studies
An aliquot of reaction mixture for the preparation of complex 1 was studied using ESI-mass technique. The spectrum showed several peaks, the most significant ones correspond to the chemical species: [Cu(κ2-N,S-L-NMe)Cu]+ (A), [Cu(κ2-N,S-L-NMe) (κ1-S-imdzSH-Me)]+ (B), [Cu(κ2-N,S-L-NMe)2CuI2+2H]+ (C), [Cu(κ2-N,S-L-NMe)2Cu2I3 + 2H]+ (D) and [Cu(κ2-N,S-L-NMe)2Cu3I4+2H]+ (E). All the five species support the formation of the new thio-ligand, N,S-L-NMe in solution followed by coordination to the CuI ion. The most abundant species happens to be A whose isotopic pattern is given in Fig. 5 and chemical species D corresponds to [M − I]+ which means loss of one iodide ion from the molecular ion of complex 1 (Fig. 6).
 |
| Fig. 5 ESI-mass of species [Cu(κ2-N,S-L-NMe)Cu]+ (A) (complex 1). | |
 |
| Fig. 6 ESI-mass of species [Cu(κ2-N,S-L-NMe)2Cu2I3 + 2H]+ D (complex 1). | |
Similar to complex 1, the ESI-mass studies of reaction mixture for the preparation of complex 4, the spectrum showed several peaks, the most significant ones correspond to the chemical species: [Cu(κ2-N,S-L-NBun)Cu]+ (F), [Cu(κ2-N,S-L-NBun)2]+ (G), [Cu(κ2-N,S-L-NBun)2CuI + H]+ (H), [Cu(κ2-N,S-L-NBun)2CuI2]+ (I) and [Cu(κ2-N,S-L-NBun)2Cu2I3]+ (J). All the five species support the formation of the new thio-ligand, N,S-L-NBun in solution followed by coordination to the CuI ion. The isotopic patterns of chemical species [Cu(κ2-N,S-L-NBun)2]+ G and [Cu(κ2-N,S-L-NBun)2Cu2I3]+ J are given in Fig. 7 and 8 respectively.
 |
| Fig. 7 ESI-mass of species [Cu(κ2-N,S-L-NBun)2]+ F (complex 4). | |
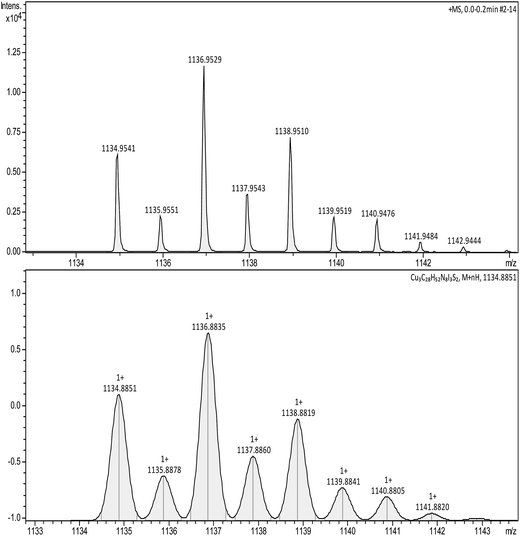 |
| Fig. 8 ESI-mass of species [Cu(κ2-N,S-L-NBun)2Cu2I3]+ (J) (complex 4). | |
Suggested possible mechanism of C–S rupture and complex formation. The formation of thio-ligand L4 occurred when copper(I) chloride/bromide were reacted with 1,3-thiazolidine-2-thione in acetonitrile under aerobic conditions. The mechanism of formation of L4 and its copper(I) complexes [CuX(N,S-L4)] (X = Cl, Br) have been reported from our laboratory.27 Scheme 4 depicts formation of L-NMe, L-NPrn and L-NBun substituted imidazolidine-2-thiones from reactions of copper(I) iodide on the pattern similar to formation of L4. Scheme 5 suggests mechanistic aspects of formation of complexes 1, 3 and 4 which involved C–S rupture.
 |
| Scheme 4 The in situ generated thio-ligands L4, and L-NR, R = Me, Prn and Bun. | |
 |
| Scheme 5 Suggested pathways for the formation of complexes: (a) 1 (b) 3, (c) 4. | |
Conclusion
It is interesting to note that imidazolidine-2-thione (imdzSH, Scheme 1) on reaction with copper(I) chloride/bromide in dimethyl sulfoxide (or DMF) was converted into 2,2′-thio-di-2-imidazoline (L1 ligand, Scheme 1).25 The reaction of copper(I) iodide with imdzSH in acetonitrile did not involve C–S rupture and formed polymeric products.33 The presence of methyl, n-propyl or n-butyl groups at one N atom of imidazolidine-2-thione yielded thio-ligands, imdzSH-Me, imdzSH-Prn and imdzSH-Bun, which reacted with copper(I) iodide in acetonitrile and were converted into 1-(4,5-dihydro-3-alkyl-imidazolidin-2-yl)-3-alkyl-imidazolidine-2-thiones (Scheme 2: L-NR, R = Me, n-Pr, n-Bu). These ligands formed mixed valent CuI–II/CuII complexes (1, 3, 4). The generation of triiodide is also an interesting feature and it either showed coordination to CuI in polymer 3 or was counter anion as in complex 4. The N-alkyl-imidazolidine-2-thiones (imdzSH-Me, imdzSH-Prn, imdzSH-Bun) have shown behavior similar to that of 1,3-thiazolidine-2-thione (tzdSH) in acetonitrile which was transformed into 3-(2-thiazolin-2-yl)thiazolidine-2-thione (L4, Scheme 1).
Experimental
Materials and techniques
Copper(I) iodide was prepared by the reduction of CuSO4·5H2O using SO2 in the presence of stoichiometric amounts of sodium iodide in water.36 The N-alkyl-imidazolidine-2-thiones (N-methyl-imidazolidine-2-thione, N-n-propyl-imidazolidine-2-thione and N-n-butyl-imidazolidine-2-thione) were prepared as per the literature methods.37 Elemental analysis for C, H and N were carried out using Thermoelectron FLASHEA1112 analyzer. Infrared (IR) spectra were recorded using KBr pellets on a Pye Unicam SP-3-300 or Varian 660-IR Fourier transform infrared spectrophotometer in 4000–400 cm−1 range. The melting points were determined with a Gallen Kamp electrically heated apparatus. The ESI-mass spectra were recorded in CH3CN using Bruker Daltonik LS-MS high resolution microTOF-Q II 10356. The single crystals of compounds 1–4 were mounted on glass fibers and data were collected using a Bruker X8 Kappa APEXII (1, 3, 4) and Bruker SMART CCD 1000 (2) diffractometers, each equipped with a graphite monochromator and Mo-Kα radiation (λ = 0.71073 Å). The unit cell dimensions and intensity data were measured at 100(2) (1, 3, 4), 110(2) (2) K. The data were processed (data collection, refinement and reduction) with APEX2 (1–4)38,39 and corrected for absorption using SADABS.40 The structures were solved by direct methods using the program SHELXS-97 (ref. 41) and refined by full-matrix least-squares techniques against F2 using SHELXL-97,41 PLATON (molecular graphics). Positional and anisotropic atomic displacement parameters were refined for all non-hydrogen atoms. Hydrogen atoms were located in difference maps and included as fixed contributions riding on attached atoms with isotropic thermal parameters 1.2 times those of their carrier atoms.
Synthesis of complexes
[CuI2CuII(κ2-N,S-L-NMe)(κ1N,μ-S-L-NMe)(μ-I)2(κ1-I)2] (1). To a solution of copper(I) iodide (0.025 g, 0.13 mmol) in acetonitrile was added a solution of N-methyl-imidazolidine-2-thione (0.015 g, 0.13 mmol) in acetonitrile. After a few days, colorless prismatic crystals of complex {Cu4(μ3-I)2(μ-I)2(μ-S-imdzSH-Me)2(η1-S-imdzSH-Me)2}n were obtained as reported earlier.27 Analysis of filtrate: the color of the filtrate changed to brownish green in next 2–4 days and black prismatic crystals of [CuI2CuII(κ2-N,S-L-NMe)(κ2-N,S-L-NMe)(μ-I)2(η1-I)2] 1 were obtained along with CuSO4·5H2O. Yield of 1 (relative to CuI): 0.005 g, 18%, m.p. 215–217 °C. Anal. calcd for C16H28Cu3I4N8S2 (1094.80): C, 17.53; H, 2.56; N, 10.23; found: C, 17.45; H, 2.44; N, 10.32%. Main IR peaks (KBr, cm−1): ν(C–H), 2919w, 2871w; ν(C–N) + δ(C–H), 1587–1528s; ν(C
S) 1114s; δ(N–CH3), 971m. Compound is poorly soluble in acetonitrile and chloroform. Magnetic moment: 1.791 BM. ESI-mass data: obsvd m/z 324.99; calcd 324.96, formula, Cu2C8H14N4S, species, [Cu(κ2-N,S-L-NMe)Cu]+ (A); obsvd m/z 441.04, calcd 441.00, formula, CuC12H22N6S2, species, [Cu(κ2-N,S-L-NMe)(κ1-S-imdzSH-Me)]+ (B); obsvd m/z 778.89, calcd 778.86, formula, Cu2C16H28N8S2I2 + 2H species, [Cu(κ2-N,S-L-NMe)2CuI2+2H]+ (C); obsvd m/z 968.73, calcd 968.69, formula, Cu3C16H28N8S2I3 + 2H species, [Cu(κ2-N,S-L-NMe)2Cu2I3 + 2H]+ (D) and obsvd m/z 1158.58, calcd 1158.52, formula, Cu4C16H28N8S2I4 + 2H species, [Cu(κ2-N,S-L-NMe)2Cu3I4 + 2H]+ (E).
[Cu(μ-S-imdzSH-Prn)(μ-I)]n (2). To a solution of copper(I) iodide (0.025 g, 0.13 mmol) in acetonitrile was added a solution of N-propyl-imidazolidine-2-thione (0.019 g, 0.13 mmol) in acetonitrile. After 2–4 days, colorless needles of complex 2 were obtained. Yield (relative to CuI): 0.008 g, 31%, m.p. 143–145 °C. Anal. calcd for C6H12CuIN2S (334.68): C, 21.51; H, 3.58; N, 8.37; found: C, 21.37; H, 3.35; N, 8.51. Main IR peaks (KBr, cm−1): ν(N–H), 3278s; ν(C–H), 2954–2870m; ν(C–N), 1534s; δ(C–H), 1467m; ν(C
S), 1190(m).
[Cu3(κ1N,μ-S-L-NPrn)2(μ-I)2(κ1-I3)2]n (3). The color of the filtrate from reaction for compound 2 above changed to greenish yellow in 2–3 days and brown needles of 3 were obtained along with CuSO4·5H2O. Yield of 3 (relative to CuI): 0.005 g, 19%, m.p. 161–163 °C. Anal. calcd for C24H44Cu3I8N8S2 (1714.61): C, 16.80; H, 2.57; N, 6.53; found: C, 16.74; H, 2.02; N, 6.68. Main IR peaks (KBr, cm−1): ν(C–H), 2956m, 2868m; ν(C–N) + δ(C–H) 1586s, 1514s; ν(C
S) 1134m. It is poorly soluble in acetonitrile and chloroform. Magnetic moment: 1.892.
[Cu(μ-N,S-L-NBun)2]·2I3 (4). To a solution of copper(I) iodide (0.025 g, 0.13 mmol) in acetonitrile was added a solution of N-butyl-imidazolidine-2-thione (0.021 g, 0.13 mmol) in acetonitrile. After 2–3 days color of solution changed to greenish brown and black prismatic crystals of complex 4 were obtained along with the formation of CuSO4·5H2O. Yield of 4 (relative to CuI): 0.015 g, 53%, m.p. 155–157 °C. Anal. calcd for C28H52CuI6N8S2 (1389.84): C, 24.17; H, 3.74; N, 8.06; found: C, 24.12; H, 3.66; N, 8.15. Main IR peaks (KBr, cm−1): ν(C–H), 2956–2870m-w; ν(C–N) + δ(C–H), 1587s, 1509m; ν(C
S) 1113m. It is very poorly soluble in acetonitrile and chloroform. Magnetic moment: 1.832 BM. ESI-mass data: obsvd m/z 4.09.09; calcd 409.05, formula, Cu2C14H26N4S, species, [Cu(κ2-N,S-L-NBun)Cu]+ (F); obsvd m/z 627.36, calcd 627.30, formula, CuC28H52N8S2, species, [Cu(κ2-N,S-L-NBun)2]+ (G); obsvd m/z 819.23, calcd 819.15, formula, Cu2C28H53N8S2I species, [Cu(κ2-N,S-L-NBun)2CuI + H]+ (H); obsvd m/z 945.10, calcd 945.01, formula, Cu2C28H52N8S2I2 species, [Cu(κ2-N,S-L-NBun)2CuI2]+ (I) and obsvd m/z 1134.95, calcd 1134.88, formula, Cu3C28H52N8S2I3 species, [Cu(κ2-N,S-L-NBun)2Cu2I3]+ (J).
Acknowledgements
Financial assistance from the Council of Scientific and Industrial Research (CSIR), New Delhi for grant [09/254(0202)/2010-EMR-I] as well as Emeritus Grant [21(0904)/12-EMR-II] to TSL are gratefully acknowledged. The authors thank Jaspreet Kaur, JRF, for assistance in ESI-mass studies.
References
- E. S. Raper, Coord. Chem. Rev., 1985, 61, 115–184 CrossRef CAS.
- E. S. Raper, Coord. Chem., Rev., 1994, 129, 91–156 CrossRef CAS.
- E. S. Raper, Coord. Chem. Rev., 1996, 153, 199–255 CrossRef CAS.
- E. S. Raper, Coord. Chem. Rev., 1997, 165, 475–567 CrossRef CAS.
- J. A. Garcia-Vazquez, J. Romero and A. Sousa, Coord. Chem. Rev., 1999, 193, 691–745 CrossRef.
- P. D. Akrivos, Coord. Chem. Rev., 2001, 213, 181–210 CrossRef CAS.
- D. Li, W.-J. Shi and L. Hou, Inorg. Chem., 2005, 44, 3907–3913 CrossRef CAS PubMed.
- L. Han, B. Wu, Y. Xu, M. Wu, Y. Gong, B. Lou, B. Chen and M. Hong, Inorg. Chim. Acta, 2005, 358, 2005–2013 CrossRef CAS.
- M. Hong, Y. Zhao, W. Su, R. Cao, M. Fujita, X. Zhou and A. S. C. Chan, Angew. Chem., Int. Ed., 2000, 39, 2468–2470 CrossRef CAS.
- T. S. Lobana, R. Sultana, A. Castineiras and R. J. Butcher, Inorg. Chim. Acta, 2009, 362, 5265–5270 CrossRef CAS.
- J. J. M. Amoore, L. R. Hanton and M. D. Spicer, Dalton Trans., 2003, 1056–1058 RSC.
- R. Peng, D. Li, T. Wu, X. P. Zhou and S. W. Ng, Inorg. Chem., 2006, 45, 4035–4046 CrossRef CAS PubMed.
- T. Wu, B.-H. Yi and D. Li, Inorg. Chem., 2005, 44, 4130–4132 CrossRef CAS PubMed.
- J. A. Garcia-Vazquez, J. Romero, A. S. Pedrares, M. L. Louro, A. Sousa and J. Zubieta, J. Chem. Soc., Dalton Trans., 2000, 559–567 RSC.
- T. S. Lobana and P. K. Bhatia, J. Sci. Ind. Res., 1989, 48, 394–408 CAS.
- L. P. Battaglia, A. B. Corradi, F. A. Devillanova and G. Verani, Transition Met. Chem., 1979, 4, 264–266 CAS.
- C. Palivan, T. Berclaz, M. Geoffroy, S. Ramaprabhu and G. Bernardinelli, J. Chem. Soc. Faraday Trans., 1995, 91, 2155–2160 RSC.
- S. R. Ramaprabhu, E. A. C. Lucken and G. Bernardinelli, J. Chem. Soc. Dalton Trans., 1995, 115–121 RSC.
- E. S. Raper, J. R. Creighton, J. D. Wilson, W. Clegg and A. Milne, Inorg. Chim. Acta, 1989, 155, 85–89 CrossRef CAS.
- G. A. Bowmaker, J. V. Hanna, B. W. Skelton and A. H. White, Chem. Commun., 2009, 2168–2170 RSC.
- S. Walia, S. Kaur, J. Kaur, A. K. Sandhu, T. S. Lobana, G. Hundal and J. P. Jasinski, Z. Anorg. Allg. Chem., 2015, 641, 1728–1736 CrossRef CAS.
- T. S. Lobana, A. K. Sandhu, R. Sultana, A. Castineiras, R. J. Butcher and J. P. Jasinski, RSC Adv., 2014, 4, 30511–30522 RSC.
- L. P. Battaglia, A. B. Corradi, M. Nardelli and M. E. V. Tani, J. Chem. Soc. Dalton Trans., 1976, 143–146 RSC.
- T. S. Lobana, R. Sharma, G. Hundal and R. J. Butcher, Inorg. Chem., 2006, 45, 9402–9409 CrossRef CAS PubMed.
- T. S. Lobana, R. Sultana, G. Hundal and R. J. Butcher, Dalton Trans., 2010, 39, 7870–7872 RSC.
- T. S. Lobana, R. Sultana and R. J. Butcher, Dalton Trans., 2011, 40, 11382–11384 RSC.
- T. S. Lobana, R. Sultana, R. J. Butcher, A. Castineiras, T. Akitsu, F. J. Fernandez and M. C. Vega, Eur. J. Inorg. Chem., 2013, 5161–5170 CrossRef CAS.
- A. G. Dodge, J. E. Richman, G. Johnson and L. P. Wackett, Appl. Environ. Microbiol., 2006, 72, 7468–7476 CrossRef CAS PubMed.
- E. W. Ainscough, A. G. Bingham and A. M. Brodie, Inorg. Chim. Acta, 1985, 96, L47–L48 CrossRef CAS.
- L. Wang, H. Wei and Z. Yu, Chem. Soc. Rev., 2013, 42, 599–621 RSC.
- T. S. Lobana, A. Rani, Y. N. Butt and J. P. Jasinski, J. Sulfur Chem., 2015, 36, 251–256 CrossRef CAS.
- T. S. Lobana, A. Rani, A. K. Jassal and J. P. Jasinski, J. Chem. Sci., 2015, 127, 149–153 CrossRef CAS.
-
(a) T. S. Lobana, R. Sharma, G. Hundal and R. J. Butcher, Inorg. Chem., 2006, 45, 9402–9409 CrossRef CAS PubMed;
(b) Y. Thanyasirikul, C. Pakawatchai, M. L. Cole, P. C. Junk, B. W. Skelton and A. H. White, Org. Biomol. Chem., 2003, 1, 3217–3222 RSC.
- J. E. Huheey, E. A. Keiter and R. L. Keiter, Inorganic Chemistry: Principles of Structure and Reactivity, Harper Collins, 4th edn, New York, 1993 Search PubMed.
- T. S. lobana, R. Sultana, G. Hundal and A. Castineiras, Polyhedron, 2009, 28, 1573–1577 CrossRef CAS.
- G. Brauer, Handbook of Preparative Chemistry, Academic Press, New York, 2nd edn, 1965, vol. 2 Search PubMed.
- J. A. Garcia-Vazquez, A. Sousa-Pedrares, M. Carabel, J. Romero and A. Sousa, Polyhedron, 2005, 24, 2043–2054 CrossRef CAS.
- Bruker, APEX2 Software, Bruker AXS Inc., V2.0-1, Madison, Wisconsin, USA, 2005 Search PubMed.
- Bruker, SMART and SAINT, Area Detector Control and Integration Software, Bruker Analytical X-ray Instruments Inc., Madison, Wisconsin, 1997 Search PubMed.
- G. M. Sheldrick, SADABS. Program for Empirical Absorption Correction of Area Detector Data, University of Goettingen, Germany, 1997 Search PubMed.
- G. M. Sheldrick, Acta Crystallogr., Sect. A: Found. Crystallogr., 2008, 64, 112–122 CrossRef CAS PubMed.
Footnote |
† Electronic supplementary information (ESI) available: The detailed information regarding ESI-mass data in detail and Ortep diagrams are given in ESI. CCDC 1421005–1421008 for compounds 1 to 4 respectively. For ESI and crystallographic data in CIF or other electronic format see DOI: 10.1039/c5ra20449f |
|
This journal is © The Royal Society of Chemistry 2015 |