DOI:
10.1039/C5RA19635C
(Paper)
RSC Adv., 2015,
5, 100856-100864
Aluminium borate whiskers grafted with boric acid containing poly(ether ether ketone) as a reinforcing agent for the preparation of poly(ether ether ketone) composites
Received
23rd September 2015
, Accepted 10th November 2015
First published on 13th November 2015
Abstract
A new soluble boron-containing poly(ether ether ketone) (B-PEEK) was synthesized through iridium-catalyzed C–H borylation of soluble meta-poly(ether ether ketone) and grafted on the surface of aluminum borate whiskers as the coupling agent between the whiskers and poly(ether ether ketone) (PEEK) matrix. The grafted aluminum borate whisker (g-W) reinforced PEEK composites were then prepared via a melt blending method. The coupling effect of B-PEEK was investigated by the interface micromorphology analysis and performance evaluation of PEEK composites. After grafting treatment with B-PEEK, aluminum borate whiskers were found to be dispersed evenly in the PEEK matrix without apparent aggregation. Ascribed to the strong interaction between whisker grafted chains and the PEEK matrix, g-W/PEEK composites show better mechanical properties and izod impact strength, higher crystallization temperature and crystalline fraction (χc) than those composites without grafting modification, indicating B-PEEK is an effective coupling reagent for PEEK and aluminum borate whiskers.
1. Introduction
Poly(ether ether ketone) (PEEK) is a semi-crystalline engineering thermoplastic with outstanding properties, such as superior mechanical properties, thermal stability and solvent resistance, which has been widely applied in aerospace technology, energy fields and chemical industry.1–3 To further improve the mechanical strength and heat resistance of PEEK, some materials with high mechanical performance such as carbon fibers, glass fibers and inorganic fillers are often introduced into PEEK matrix.4–6 Aluminum borate whisker is an acicular crystal mineral with high aspect ratio, stiffness and strength. It is almost free from internal flaws and the yield strength tends to approach the maximum theoretical value expected from the theory of elasticity.7,8 Using aluminum borate whisker as a reinforcing filler in polymer is especially suitable for those which are generally difficult to be reinforced by fibers or large size fillers, and those which are small but have high-performance and high-dimension precision parts.9
It is well known that besides the characteristics, dimensions, and shapes of inorganic fillers, the properties of polymer composites strongly depend on the interfacial bonding strength between fillers and polymer matrix. Therefore, inorganic fillers are usually first treated by coupling agents before compounding to promote the dispersion and improve the interfacial adhesion with polymer matrix.10–13 Composites modified by coupling agents exhibit improved performance in many aspects, such as mechanical properties, thermal conductivity, crystalline ability and so on.14–17 For polymer/aluminum borate whisker composites, organic borate coupling agents are generally used because boron oxygen framework in coupling agents has good interaction with borate whiskers. In recent years, grafting similar polymer segments to the whisker surface with the matrix becomes an effective way to improve the interface compatibility between them by polymer chains entanglement and/or through chemical bonding.18,19 Therefore, covalent and noncovalent grafting reactions have been used to modify inorganic particles, which was beneficial to the design of new functional organic–inorganic hybrid materials.
In addition, coupling agent which has good compatibility with resin could play a good modification effect, but the solvent resistance of PEEK is not conducive to the preparation of modified agents.19–21 Borate ester coupling agents were usually used as the interfacial adhesion between the polymer matrix and aluminum borate whisker.22,23 However, the conventional coupling agents are seldom used in high performance polymer composites due to their bad thermal stability, which can't meet the high temperature requirement for polymer processing, such as PEEK, PES, TPI, etc. Recently, Bae et al. discovered that aromatic main chain polymers such as PSU can be functionalized in a controlled manner using an iridium-catalyzed C–H borylation. Bpin group along the polymer chain could be conveniently transformed to more polar boronic acid.24 Otherwise, we previously reported that the boron-containing poly(arylene ether ketone) was successfully prepared by iridium-catalyzed C–H borylation.25 Because boric acid-containing poly(aryl ether ketone) own boric acid side chain which could form covalent bond with aluminum borate whisker and aromatic main chain which are structurally similar to PEEK, so it may be an outstanding interfacial adhesion. In the present study, a novel boric acid containing poly(arylene ether ketone) was first prepared according to previous literatures. And then the boron-containing poly(arylene ether ketone) were grafted on the surface of whisker to obtain grafted whisker (g-W), and the g-Ws were incorporated into the PEEK matrix. So aluminum borate whisker/PEEK composites were prepared. The reinforcing effects and mechanisms of coupling agents were studied in detail. In the process, the mechanical, thermal properties and crystallization behavior of the composites were investigated.
2. Experimental
2.1 Materials
PEEK with a melt-flow index of 25 g/10 min at 400 °C was purchased from Jilin University Super Engineering Plastics Research Co., Ltd (Changchun, P. R. China), the structure of PEEK as shown below:
The needle-shaped aluminum borate whisker with average length of 10–30 μm, average diameter of 0.5–1 μm, density of 2.93 g cm−3 and specific surface of 2.3 m2 g−1 were provided by Shanghai NP Trade CO., Ltd. (Shanghai, China). m-Dihydroxybenzene was purchased from Tianjin Guangfu Fine Chemical Research Institute (Tianjin, China). 4,4-Difluorobenzophenone (DFBP) (Yanji Chemical Plant, China) was purified before use. Bis(pinacolato)diboron (B2pin2) (98%) was provided by Energy Chemical, China. Chloro-1,5-cyclooctadiene iridium(I) dimer ([Ir(COD)Cl]2) (98%), 4,4′-ditert-butyl-2,2′-dipyridyl (dtbpy) and sodium periodate were obtained from Sigma-Aldrich Co. LLC. N,N-Dimethylacetamide (DMAc), toluene and other chemical reagents were purchased from Beijing Chemical Reagent, China. K2CO3 was obtained from Sinopharm Chemical Reagent Co., Ltd, (China) and dried at 120 °C for 24 h before polymerization.
2.2 Synthesis of poly(ether ether ketone) containing meta-phenyl links (m-PEEK)
m-Dihydroxybenzene (11.01 g, 0.1 mol), 4,4′-difluorobenzophenone (21.82 g, 0.1 mol), anhydrous K2CO3 (15.20 g, 0.11 mol), DMAc (130 mL) and toluene (35 mL) were added to a 250 mL three-necked flask equipped with a mechanical stirrer, a nitrogen inlet with a thermometer, and a Dean–Stark trap with a condenser. The system was heated to reflux for 2.5 h in order to remove the water. After distillation of toluene, the reaction mixture was heated at 160 °C for 4 h to finish the polymerization. The viscous solution was then poured into water and discontinuous threadlike polymer trips were obtained. The polymer was pulverized into powders and washed with hot water and ethanol subsequently several times. After dried at 120 °C for 24 h, poly(ether ether ketone) with meta-phenyl links (m-PEEK) was obtained.
2.3 Synthesis of boron-containing poly(aryl ether ether ketone)
The preparation of borylated m-PEEK (PEEK-Bpin) was carried out according to the synthetic pathway shown in Scheme 2. m-PEEK (8.1496 g, 0.02 mmol of polymer repeating unit), B2pin2 (1.3544 g, 0.0054 mmol, 4/15 equiv.), [IrCl(COD)]2 (0.0538 g, 1.5 mol% based on the amount of B2pin2), dtbpy (0.043 g, 3 mol% based on the amount of B2pin2), and a magnetic stirring bar were placed into a 250 mL round-bottom flask fitted with a reflux condenser. THF (200 mL) was then injected into the reaction system and the system was stirred in an oil bath at 80 °C for 10 h under the nitrogen atmosphere. After cooling to room temperature, the reaction solution was diluted with 200 mL THF and filtered through a short plug of silica gel to remove the catalyst. The filtrate was concentrated using a rotary evaporator and poured into 500 mL methanol to precipitate the polymer. The dissolution and precipitation process was repeated several times. The borylated polymer was isolated as white fibers after drying under vacuum at 80 °C for 12 h (6.85 g). The mol% of Bpin was estimated based on the relative intensity of resonances of hydrogen around ketone in the polymer main chain (at 7.6–7.7 ppm) and the four methyl groups of the Bpin (at 1.0–1.2 ppm).
2.4 Preparation of PEEK-B(OH)2
15% PEEK-Bpin (6.1609 g, 3 mmol Bpin) was dissolved in 250 mL dioxane, and then NaIO4 (9.63 g, 45 mmol, 15 equiv.) was added. Distilled water (9 mL) was added dropwise into the polymer solution and the reaction mixture was stirred at 90 °C for 8 h. After the solvent was evaporated thoroughly by using the rotary evaporator, the residual polymer was washed with methanol and water several times, filtered and dried at 80 °C under vacuum for 12 h (5.50 g).
2.5 Modification of aluminum borate whiskers
The modification scheme of aluminum borate whisker was shown in Scheme 1. The whiskers were added into 1 g/200 mL solution of PEEK-B(OH)2 mixed with CHCl3, the mass fraction of PEEK-B(OH)2 is 2%, 4%, 6% and 10% of the whiskers. The mixture solution was heated to reflux for 2 h in the nitrogen atmosphere. The grafted whiskers were collected by filtration, then washed by cold CHCl3 and dried in a vacuum oven at 80 °C for 48 h. At last, the grafted whiskers were treated at 120 °C for 12 h, 150 °C for 12 h, 180 °C for 12 h and 210 °C for 12 h. Four kinds of grafted whiskers with different PEEK-B(OH)2 content were obtained and named g-W-1, g-W-2, g-W-3 and g-W-4, respectively.
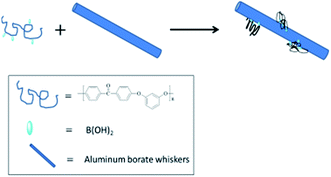 |
| Scheme 1 Modification of aluminum borate whisker. | |
2.6 Preparation of composites
First of all, PEEK resin and whiskers were mixed with a high speed mixer, the mass fraction of whisker was 10, 20 and 30 wt% in the composites. Composites were further compounded using a HAAKE PTW16/25p co-rotating twin-screw extruder with a screw speed of 120 rpm. The temperature profiles of the barrel were 320–340–355–360–355–340 °C from the hopper to the die. All the composites were dried in an oven at 120 °C for 3 h prior to injection molding. Standard test bars were injected at a barrel temperature of 380 °C and mold temperature of 180 °C by an HAAKE MiniJet II (HAAKE, German). The size of bars was 2.0 mm × 5.0 mm × 75.0 mm for tensile tests and 4 mm × 10 mm × 78 mm for three-point flexural tests. With 10 wt% whisker content, above four kinds of whiskers/PEEK composites were named as g-W-1/PEEK10, g-W-2/PEEK10, g-W-3/PEEK10 and g-W-4/PEEK10. For comparison, the composite without grafting treatment was prepared and named as W/PEEK10. Some PEEK composites with 20 wt% and 30 wt% whisker contents were also prepared and named as W/PEEK20, g-W-2/PEEK20, W/PEEK30 and g-W-2/PEEK30, separately.
2.7 Characterization
FT-IR spectra were measured on a Bruker Victor22 Fourier-transform infrared spectrometer. 1H-NMR experiments were carried out on a Bruker 510 spectrometer (500 MHz for 1H) using DMSO-d6 or CDCl3 as solvents. The morphology of W, g-W and PEEK composites were observed by SEM (HITACHI, SU8020) with quenched samples in liquid nitrogen. Mechanical testing was carried out with extensometer by a Shimadzu AG-I instrument at room temperature. Before test, all samples were treated at 200 °C for 2 h. The thermal stability of whisker and composites were measured by Netzsch Sta449C thermogravimetric analysis under nitrogen or air atmosphere with a heating rate at 10 °C min−1. The notch impact strength properties were measured using XJ-6 cantilever beam impactor. Mettler Tolledo DSC821e instrument was used to evaluate the crystallization behavior of composites. Cooling scans were performed at 2 °C min−1, whereas heating scans were carried out at 10 °C min−1. Crystallization peak temperature (Tc) and crystallization enthalpy (ΔHc) were determined on the first cooling scan, while the temperature of melting peaks (Tm) were obtained on the second heating scan. The isothermal crystallization kinetics of the composites were first held at 400 °C for 5 min, then decreased to the desired crystallization temperature with a cooling rate of 100 °C min−1 and held for 30 min.
3. Results and discussion
3.1 Molecular weight and structure of PEEK-B(OH)2
PEEK-B(OH)2 has been synthesized via the iridium-catalyzed C–H borylation method. As shown below: Scheme 2
The number-average molecular weight (Mn) of PEEK-B(OH)2 was 1.1 × 104 with a polydispersity index of 1.63 determined by GPC. The Tg of PEEK-B(OH)2 was 139 °C, which was higher than that of m-PEEK. The 1H-NMR spectra of PEEK-Bpin and PEEK-B(OH)2 were shown in Fig. 1. PEEK-Bpin exhibited signals of hydrogen g in boronate pinacol pendants at 1.16–1.25 ppm, and there were no signals at 1.16–1.25 ppm in PEEK-B(OH)2. The results demonstrated that pinacol had been taken off successfully. On the basis of the integral ratio of a and c, the molar percentage of the pinacol phenyl boronate in PEEK-Bpin was calculated to be 15%. In order to prove the existence of the boric acid, the PEEK-B(OH)2 was fabricated into a thin membrane, and then the cross section of the membrane was measured by EDX. The results were shown in Fig. 2, it can be seen that boron was evenly distributed in the polymer, which confirmed the existence of phenylboronic acid in the PEEK-B(OH)2.
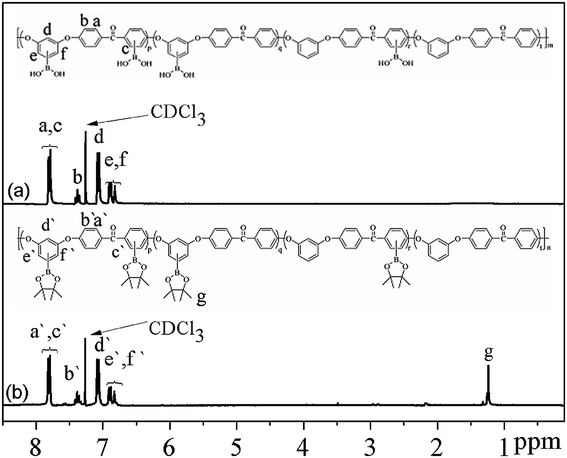 |
| Fig. 1 1H-NMR spectra of the PEEK-Bpin and PEEK-B(OH)2. | |
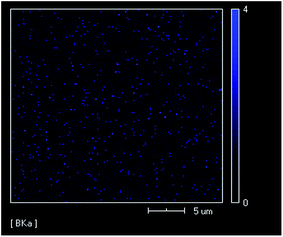 |
| Fig. 2 EDX image of PEEK-BOH membrane cross section. | |
3.2 Grafted whiskers thermal treatment
The g-Ws were formed by coordination-bonding interactions between boronic acid groups of PEEK-B(OH)2 and surface hydroxyl groups of whiskers, as shown in Scheme 1. PEEK-B(OH)2 can strengthen interface properties between g-Ws and PEEK due to the similar structure of PEEK-B(OH)2 and PEEK. Heat treatment temperature and time were important for the coupling agent combined with the surface of whisker, grafted whiskers were treated in 120 °C for 12 h, 150 °C for 12 h, 180 °C for 12 h and 210 °C for 12 h. TGA was used to evaluate the effect of heat treatment. As summarized in Fig. 3. With the increase of heat treatment temperature, the weight loss of coupling agent at 180 °C was close to that at 210 °C. So the heat treatment temperature and time was identified as 180 °C for 12 h.
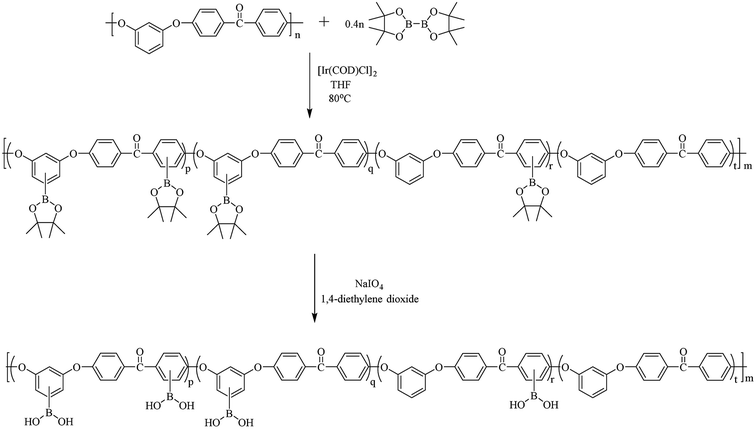 |
| Scheme 2 Synthesis of PEEK-B(OH)2. | |
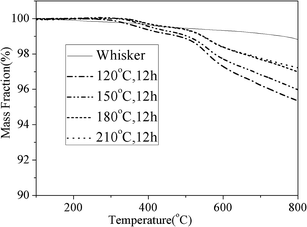 |
| Fig. 3 TGA Curves of aluminum borate whisker modified by PEEK-B(OH)2 at 120 °C, 12 h, 150 °C, 12 h, 180 °C, 12 h and 210 °C, 12 h in N2. | |
3.3 Characterization of modified aluminum borate whiskers
The process of grafting polymers on the surface of whisker was carried out according to Scheme 2. The FT-IR spectra of pure W and g-Ws were shown in Fig. 4. As shown in Fig. 4b–d, the peaks appeared at 1500, 1600, 3030 and 1680 cm−1 were attributed to the in-plane vibration of benzene and C
O stretching vibration of PEEK.21,26 This indicated that PEEK-B(OH)2 had been grafted on the surface of whiskers. Fig. 5 depicted the morphology of pure W and g-Ws. Compared to the pure W (Fig. 6a), the g-W-1 and g-W-2 exhibited a dispersed state, but g-W-3 and g-W-4 had some agglomeration (Fig. 5b–e). The results further demonstrated that PEEK-B(OH)2 was coated on the surface of whisker. Further, DSC (Fig. 6) was used to study the effect of interface bonding. It was obvious that the Tg of coupling agent in g-W was higher than that of PEEK-B(OH)2. Because the interaction between boric acid and surface of whisker restricted polymer chain mobility. Meanwhile, chain entanglement of the polymer on the surface leads to high grafting density. Fig. 7 showed TGA curves of W and g-Ws. As can be seen, at 375 °C, which was just the extrusion temperature, TGA curves were nearly flat. This indicated that there was no obvious thermal degradation of the polymers during the unabiding resident time of processing.27 In addition, it can be known from Fig. 7 that the grafting percentage of g-W-1, g-W-2, g-W-3 and g-W-4 was 1.06, 2.66, 3.98 and 7.47 wt%, respectively.
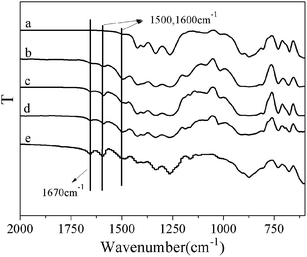 |
| Fig. 4 FT-IR spectra of the (a) Ws, (b) g-W-1s, (c) g-W-2s, (d) g-W-3s and (e) g-W-4s. | |
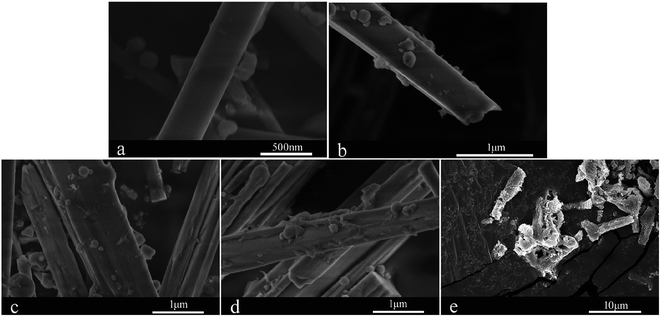 |
| Fig. 5 SEM micrographs of (a) Ws, (b) g-W-1s, (c) g-W-2s, (d) g-W-3s and (e) g-W-4s. | |
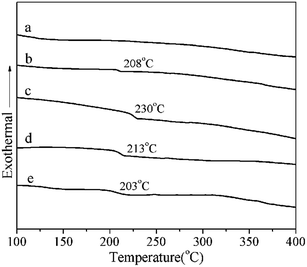 |
| Fig. 6 DSC curves of (a) Ws, (b) g-W-1s, (c) g-W-2s, (d) g-W-3s and (e) g-W-4s. | |
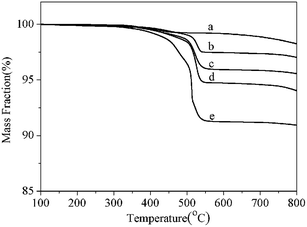 |
| Fig. 7 TGA curves of (a) Ws, (b) g-W-1s, (c) g-W-2s, (d) g-W-3s and (e) g-W-4s. | |
3.4 Mechanical properties of the composites
Table 1 showed the mechanical properties of PEEK composites with W and g-W loadings of 10, 20 and 30 wt%. It can be seen that the modulus and strength of composites with whisker were enhanced in comparison with the pure PEEK. However, introducing whisker into the composites resulted in some drop of elongation at break. The Young's modulus, flexural modulus and flexural strength of the composites with g-W increased more than the values of the composites with W. The mechanical performance of the four composites with g-W did not show remarkable difference, but the mechanical performance of the g-W-2/PEEK was a little better than the others. In general, mechanical performance of the composites basically depended on the efficiency of interfacial stress transfer. The PEEK chain had good compatibility with the g-W and could diffuse into the g-W during melt blending and establish a strong interaction due to the similar molecular structure. The strong interfacial interaction and compatibility increased stress transfer to the g-W particles, which resulted in high mechanical performance of the composites.
Table 1 The mechanical properties of PEEK and composites with W and g-W
Sample |
Flexural modulus (GPa) |
Flexural strength (MPa) |
Young's modulus (MPa) |
Tensile strength (MPa) |
Strain at break (%) |
PEEK |
3.422 |
122.6 |
1.821 |
96.00 |
62.00 |
W/PEEK |
4.138 |
132.9 |
2.247 |
100.6 |
20.52 |
g-W-1/PEEK |
4.273 |
139.7 |
2.462 |
103.9 |
22.52 |
g-W-2/PEEK |
4.365 |
140.7 |
2.807 |
104.2 |
24.68 |
g-W-3/PEEK |
4.013 |
136.5 |
2.511 |
103.9 |
18.03 |
g-W-4/PEEK |
3.898 |
133.1 |
2.499 |
102.3 |
13.81 |
20%W/PEEK |
5.010 |
150.1 |
3.063 |
109.4 |
10.53 |
20%g-W-2/PEEK |
5.672 |
160.4 |
3.132 |
110.6 |
13.06 |
30%W/PEEK |
8.184 |
178.3 |
4.355 |
110.2 |
7.732 |
30%g-W-2/PEEK |
8.406 |
183.1 |
4.773 |
116.7 |
8.216 |
3.5 Izod impact strength of the composites
Fig. 8 showed the izod impact strength of PEEK composites with W and g-W loadings of 10, 20 and 30 wt%. It can be seen that the izod impact strength of composites with whisker decreased gradually with the increase of the whisker content. The toughness decline range of the g-W/PEEK composites reduced less than that of W/PEEK composites. The decline range of g-W-2/PEEK10 composite was the most significant, because the g-W of the g-W-2/PEEK10 composites dispersed more uniform and interface binding force increased at the same time, thus the composites owned enhanced ability to resist impact. However, as the content of whisker further increase, coupling agent is not enough to make whisker disperse adequately, so the decline range of g-W-2/PEEK30 consistent with W/PEEK30. Boric acid containing poly(aryl ether ketone) in a certain range can effectively improve interface bonding force between the whiskers and resin.
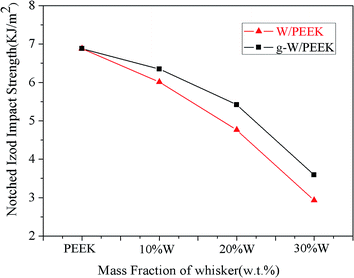 |
| Fig. 8 Notched izod impact strength of PEEK, W/PEEK, g-W/PEEK. | |
3.6. Crystallization behavior of the composites
Besides reinforcement and interfacial interactions, whiskers had an effect on the crystallization behavior of PEEK. DSC was applied to estimate the effects of the W and g-W on the crystallization and melting behavior of the composites. The parameters including Tccold, Tc, Tm, and χc of the composites were shown in Table 2. The crystallization peak temperature (Tc) shifted to a lower temperature for both W and g-W-reinforced composites in comparison with neat PEEK. The Tc of g-W-filled PEEK composites was slightly higher than that of the W-filled composite, but the trend of the Tccold was on the contrary. Furthermore, there was no significant change in the melting points (Tm) for both filled and unfilled specimens, as shown in Table 2. From the figure of DSC, the crystalline fraction χc could be estimated by relating it to the heat of fusion of an infinitely thick PEEK crystal, ΔH0f, as28,29 |
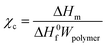 | (1) |
where ΔH0f is 130 J g−1 and Wpolymer is the weight fraction of polymer matrix. All of the five composites had a higher χc than neat PEEK, and the crystalline fraction of the composites filled by g-W was slightly higher than that of composites with W. When whiskers were used to reinforce PEEK, it decreased the entanglement of the polymer chains, so the χc increased slightly. Due to the large size of whisker, the Tc of W-filled PEEK composites shifted to the lower temperature, which showed that the whisker did not play the role of heterogeneous nucleation. The higher Tccold implied that the whisker restricted molecular mobility of the polymer matrix. When the polymers were grafted onto the surface of whisker, there would be good compatibility between fillers and PEEK matrix due to the similar molecular structure. This caused a lubricating effect that made the molecular mobility of polymer matrix easier, so the Tc of g-W-filled PEEK composites were higher and the Tccold were lower than that of the W-filled composite. This showed the g-W could make the nucleation quicker and molecular mobility of the polymer matrix easier in comparison with the W.
Table 2 The DSC data of the PEEK and composites with W and g-W
Sample |
Tccold (°C) |
Tc (°C) |
Tm (°C) |
χc (%) |
PEEK |
173.1 |
293.9 |
339.5 |
22.2 |
W/PEEK |
176.2 |
286.0 |
338.1 |
23.4 |
g-W-1/PEEK |
175.1 |
286.6 |
338.6 |
24.1 |
g-W-2/PEEK |
175.0 |
287.5 |
339.2 |
25.3 |
g-W-3/PEEK |
174.3 |
286.2 |
339.1 |
23.6 |
g-W-4/PEEK |
173.8 |
285.1 |
338.3 |
23.2 |
The isothermal crystallization behaviors of the composites were studied by DSC, with the samples held at 400 °C for 5 min and cooled from 400 °C to 291, 289, 287 and 285 °C at a cooling rate of 100 °C·min−1, respectively, and held at the temperature for 30 min. The result was analyzed by means of the Avrami equation.30
|
1 − Xt = exp −ktn
| (2) |
Eqn (2) is often written in the logarithmic form:
where
k is the rate constant of crystallization and
n is the Avrami exponent, which can be related to the type of nucleation and the geometry of crystal growth. The log[−ln(1 −
Xt)]
vs. log
t for composites showed an initial linear portion, followed by a gradual roll-off. From the intercept and slope of the linear part, the values of
k and
n were calculated, respectively (
Table 3). The half time of crystallization
t1/2, can be calculated by using the follow equations:
Table 3 The isothermal crystallization kinetics of PEEK and its composites
Samples |
Crystallization temperature (°C) |
n |
K (min−1) |
t1/2cal (min) |
t1/2exp (min) |
PEEK |
291 |
2.24 |
3.59 |
0.48 |
0.48 |
289 |
2.36 |
4.81 |
0.44 |
0.44 |
287 |
2.12 |
4.15 |
0.43 |
0.43 |
285 |
2.05 |
5.04 |
0.38 |
0.39 |
W/PEEK |
291 |
2.78 |
1.72 |
0.72 |
0.69 |
289 |
2.53 |
2.32 |
0.62 |
0.61 |
287 |
2.51 |
3.25 |
0.54 |
0.54 |
285 |
2.33 |
2.91 |
0.54 |
0.52 |
g-W-1/PEEK |
291 |
2.16 |
2.33 |
0.57 |
0.57 |
289 |
2.06 |
2.47 |
0.54 |
0.53 |
287 |
2.01 |
2.79 |
0.50 |
0.48 |
285 |
1.97 |
3.49 |
0.44 |
0.43 |
g-W-2/PEEK |
291 |
2.42 |
3.54 |
0.51 |
0.51 |
289 |
1.98 |
2.85 |
0.49 |
0.47 |
287 |
1.93 |
3.24 |
0.45 |
0.45 |
285 |
2.02 |
4.20 |
0.41 |
0.41 |
g-W-3/PEEK |
291 |
2.62 |
2.14 |
0.65 |
0.64 |
289 |
2.43 |
2.72 |
0.57 |
0.57 |
287 |
2.14 |
2.81 |
0.52 |
0.52 |
285 |
2.27 |
4.25 |
0.45 |
0.45 |
g-W-4/PEEK |
291 |
2.66 |
1.93 |
0.68 |
0.68 |
289 |
2.54 |
2.64 |
0.61 |
0.59 |
287 |
2.35 |
2.82 |
0.55 |
0.55 |
285 |
2.24 |
3.58 |
0.49 |
0.48 |
The values of n, k, t1/2cal (t1/2exp) were listed in Table 3. The values of n were around 2 or 3, and hardly dependent on the components of the composites and the crystallization temperature. The values of k were influenced by the components of the composites and the crystallization temperature. The experimental values (t1/2exp) were close to the calculated values (t1/2cal), indicated that the Avrami equation could be described as the preliminary crystallization of PEEK and its composites with whisker. As shown in Table 3, the rate constant of crystallization decreased as the crystallization temperature increased, and the k of the composites was lower than that of pure PEEK at a given crystallization temperature. The composites with g-W showed a higher value of k in comparison with that of the composites with W, This phenomenon was consistent with the trend of the Tccold and Tc. Furthermore, as seen in Fig. 9, the crystallization rate of the g-W-reinforced composites was lower than that of the pure PEEK but higher than that of the W-reinforced composites. These suggested that there was an interfacial layer between filler and polymer matrix which played the role of a lubricant and made transfer of the polymer chain easier.18 From the crystallization behavior results of composites, the composite with g-W-2 showed the best crystallization ability.
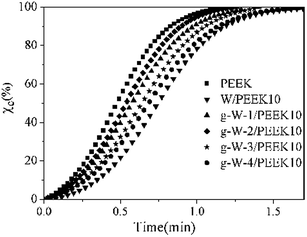 |
| Fig. 9 The plot of crystallinity vs. time for PEEK and its composites at 291 °C. | |
3.7 Morphology of the composites
From Fig. 10, there was no bulk agglomeration of whisker in composite with the W or g-W except for g-W-3 and g-W-4. This may be due to good compatibility of whisker and polymer as well as the large size of the whisker. However, coupling regent of g-W-3 and g-W-4 was too more to lead to agglomeration of whisker. As shown in Fig. 10, the surface of the g-W was relatively coarse and some polymer matrix could be seen on the surface of the whisker, implying the strong interfacial adhesion. Whereas the surface of the W was relatively smooth, due to the poor interfacial adhesion. This phenomenon may be the source of the improvement of mechanical properties in the present composites. Furthermore, the amount of polymer matrix which adhered to the g-W-2 was superior to the amounts adhered to the g-W-1, the g-W-3 and the g-W-4, indicating that the interfacial interaction between g-W-2 and PEEK matrix was stronger. Besides, as shown in Fig. 11a, c and e, the bulk agglomeration of whisker was increasingly severe. However, Whisker evenly dispersed in the g-W/PEEK composite from Fig. 11a, d and f. It indicated the coupling reagent played a vital role.
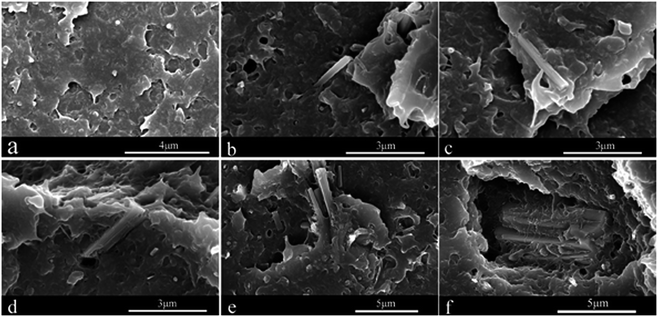 |
| Fig. 10 SEM images of (a) PEEK, (b) W/PEEK10, (c) g-W-1/PEEK10, (d) g-W-2/PEEK10, (e) g-W-3/PEEK10 and (f) g-W-4/PEEK10. | |
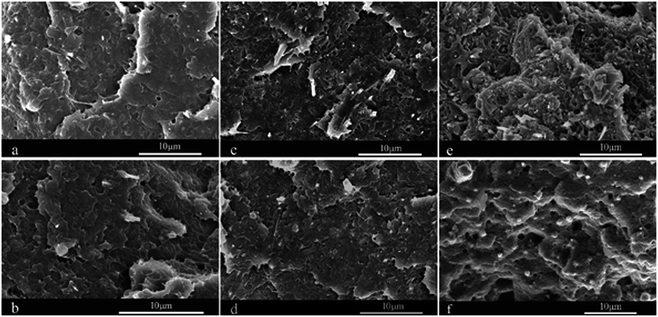 |
| Fig. 11 SEM images of (a) W/PEEK10, (b) g-W-2/PEEK10, (c) W/PEEK20, (d) g-W-2/PEEK20, (e) W/PEEK30 and (f) g-W-2/PEEK30. | |
4. Conclusion
In consideration that the conventional and commercially important PEEKs are generally not soluble in common organic solvents. We used the m-PEEK as the modification of aluminium borate whiskers. New boron-containing poly(ether ether ketone)s were successfully synthesized and grafted on the surface of whisker. FT-IR and DSC results showed that the PEEK-B(OH)2 had been successfully grafted on the surface of whiskers. The TGA curves showed that the g-W met the high melting processing temperature of PEEK. The composites with g-W showed better mechanical properties than those of the composites with W, because of the enhanced interfacial interaction between the g-W and the PEEK matrix. Whisker and grafted whiskers resulted in different effects on the crystallization behavior of PEEK. The composites with g-W had higher Tc, lower Tccold and faster crystallization rate compared with the composite with W. These results suggested that grafting polymer on the surface of whisker could improve the crystallization ability of the composites. This was also confirmed by the SEM images. Therefore, this new PEEK-B(OH)2 was a good modification agent and showed potential use for preparing high-performance composites.
References
- T. E. Attwood, P. C. Dawson and J. L. Freeman, et al., Polymer, 1981, 22, 1096–1103 CrossRef CAS.
- P. T. McGrail, Polym. Int., 1996, 41, 103–121 CrossRef CAS.
- A. Jonas and R. kegras, Polymer, 1991, 32, 2691–2706 CrossRef CAS.
- M. F. Talbott, G. S. Springer and L. A. Berglund, J. Compos. Mater., 1987, 21, 1056–1081 CrossRef CAS.
- J. R. Sarasua, P. M. Remiro and J. Pouyet, J. Mater. Sci., 1995, 30, 3501–3508 CrossRef CAS.
- G. S. Zhuang, G. X. Sui, H. Meng, Z. S. Sun and R. Yang, Compos. Sci. Technol., 2007, 67, 1172–1181 CrossRef CAS.
- K. Tsugeki, T. Yoshikawa, H. Maeda, K. Kusakabe and S. Morooka, J. Am. Ceram. Soc., 1993, 76, 3061 CrossRef CAS.
- X. J. Zhang, X. Y. Zhang, B. S. Zhu, K. L. Lin and J. Chang, Dent. Mater. J., 2012, 31, 903–908 CrossRef.
- X. Y. Zhang, X. J. Zhang, Z. L. Huang, B. S. Zhu and R. R. Chen, Dent. Mater. J., 2014, 33, 141–146 CrossRef CAS.
- G. Hwang, Y. T. Shieh and K. C. Hwang, Adv. Funct. Mater., 2004, 14, 487–491 CrossRef CAS.
- X. Jin, N. Fu, H. L. Ding, N. Zhao, J. B. Sang, X. L. Li, S. Abbas, X. W. Xu, F. B. Meng, J. Li, Y. Fan and C. C. Tang, RSC. Adv., 2015, 558171–558175 Search PubMed.
- R. Blake, J. N. Coleman, M. T. Byrne, J. E. McCarthy, T. S. Perova and W. J. Blau, et al., J. Mater. Chem., 2006, 16, 4206–4213 RSC.
- A. A. Koval'chuk, V. G. Shevchenko, A. N. Shchegolikhin, P. M. Nedorezova, A. N. Klyamkina and A. M. Aladyshev, Macromolecules, 2008, 41, 7536–7542 CrossRef.
- V. A. Soloukhin, W. Posthumus, J. C. M. Brokken-Zijp, J. Loos and G. With, Polymer, 2002, 43, 6169–6181 CrossRef CAS.
- Y. H. Lai, M. C. Kuo, J. C. Huang and M. Chen, Mater. Sci. Eng., A, 2007, 458, 158–169 CrossRef.
- C. R. Rong, G. Ma, S. L. Zhang, L. Song, Z. Chen, G. B. Wang and P. M. Ajayan, Compos. Sci. Technol., 2010, 70, 380–386 CrossRef CAS.
- M. Zhang, D. L. Zang, J. Y. Shi, Z. X. Gao, C. Y. Wang and J. Li, RSC. Adv., 2015, 5, 67780–67786 RSC.
- S. Zulfiqar, I. Lieberwirth, Z. Ahmad and M. I. Sarwar, Acta Mater., 2008, 56, 4905–4912 CrossRef CAS.
- G. Ma, X. G. Yue, S. L. Zhang, C. R. Rong and G. B. Wang, High Perform. Polym., 2011, 23, 160–169 CrossRef CAS.
- A. X. Liu, Z. K. Hong, X. L. Zhuang, X. S. Chen, Y. Cui, Y. Liu and X. B. Jing, Acta Biomater., 2008, 4, 1005–1015 CrossRef CAS PubMed.
- T. Norio, Polym. J., 2007, 39, 83–100 Search PubMed.
- G. Z. Liang and X. L. Hu, J. Appl. Polym. Sci., 2004, 92, 1950–1954 CrossRef CAS.
- G. Z. Liang and X. L. Hu, Polym. Int., 2004, 53, 670–674 CrossRef CAS.
- Y. Chang, H. H. Lee, S. H. Kim, T. S. Jo and C. Bae, Macromolecules, 2013, 46, 1754–1764 CrossRef CAS.
- Y. P. Wang, M. Z. Liu, Y. X. Zhang, Y. J. Li, Y. Shang, X. B. Zhu, H. B. Zhang and Z. H. Jiang, Chem. Res. Chin. Univ., 2014, 5, 1086–1092 Search PubMed.
- D. B. Mawhinney, V. Naumenko, A. Kuznetsova, J. T. Yates, J. Liu and R. E. J. Am, Chem. Soc. Rev., 2000, 122, 2383–2384 CrossRef CAS.
- B. Zhou, X. B. Ji, Y. Sheng, L. F. Wang and Z. H. Jiang, Eur. Polym. J., 2004, 40, 2357–2363 CrossRef CAS.
- J. Sandlera, P. Wernerb, M. S. P. Shaffera, V. Demchukc, V. Altstadt and A. H. Windlea, Composites, Part A, 2002, 33, 1033–1039 CrossRef.
- Y. Peng, R. Liu and J. Z. Cao, Appl. Surf. Sci., 2015, 332, 253–259 CrossRef CAS.
- M. Avrami, J. Chem. Phys., 1940, 8, 212–224 CrossRef CAS.
|
This journal is © The Royal Society of Chemistry 2015 |