DOI:
10.1039/C5RA19468G
(Paper)
RSC Adv., 2015,
5, 97367-97380
Synthesis of 2-anilinopyridine–arylpropenone conjugates as tubulin inhibitors and apoptotic inducers†
Received
21st September 2015
, Accepted 19th October 2015
First published on 20th October 2015
Abstract
A series of new (Z)-3-(arylamino)-1-(2-(arylamino)pyridin-3-yl)prop-2-en-1-one conjugates 9a–p were synthesized and evaluated for their cytotoxic activity against some human cancer cell lines. Some of the treated compounds like 9a, 9g and 9j showed significant activity with IC50 values ranging from 0.51 to 1.29 μM. Flow cytometry results revealed that for A549 cells these compounds caused accumulation of cells in the G2/M phase. Interestingly, compound 9a demonstrated a considerable inhibitory effect on tubulin polymerization and showed significant inhibition of tubulin polymerization with an IC50 value of 1.34 μM, whereas nocodazole showed antitubulin activity with an IC50 value 2.64 μM. Further, Hoechst staining and activation of caspase-3 suggested that these conjugates induced cell death by apoptosis. Fluorescence based competitive colchicine binding assay and docking studies suggest that these conjugates 9a and 9g bind to the tubulin perfectly at the colchicine binding site.
Introduction
Microtubules are key components of eukaryotic cells in a variety of elemental cellular processes such as formation, cell division, maintenance of cell shape, regulation of motility, cell signaling, secretion and cytoplasmic transport.1–3 Microtubules have a fundamental role in cellular processes, which makes them an important target for anticancer drugs; consequently, the molecules that target tubulin also stop the mitosis of cancer cells.4–6 A number of small molecules have been described in the literature that inhibit polymerization of tubulin with subsequent arrest of cells during mitosis and thereby leading to apoptosis.7 Microtubule polymerization inhibitors are effective in the treatment of breast, lung, ovarian and other cancers. Consequently, microtubules have become an important target to discover new anticancer agents. However, peripheral neuropathy occurrence is a main complication in the development of microtubule targeting agents as anticancer drugs. To overcome this problem, the discoveries of new molecules to defeat neuropathies are required and some important ones are colchicine (I), combretastatin A-4 (II) nocodazole (III) and E-7010 (IV).8–12 Combretastatin A-4 (CA-4) (II) is one of the well-known natural product, isolated from the bark of the South African tree Combretum caffrum that inhibits tubulin polymerization at the colchicine binding site.13,14 CA-4 has shown significant cytotoxicity against a variety of human cancer cell lines, including multidrug resistant cell lines (Fig. 1).15
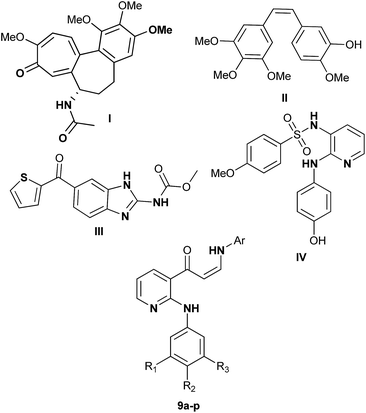 |
| Fig. 1 Chemical structures of microtubule targeting agents nocodazole (I), combretastatin A-4 (II), E-7010 (III) and (Z)-3-(arylamino)-1-(2-(arylamino)pyridin-3-yl)prop-2-en-1-one conjugates (9a–p). | |
A disodium phosphate derivative of CA-4 (CA-4P) has shown promising cytotoxicity in human cancer and it is in clinical trials.16 Structure activity relationship (SAR) studies of CA-4 reveals that 3,4,5-trimethoxy phenyl ring and cis-double bond are essential for activity.17–19 Similarly E-7010 (III) was designed and synthesized by Hiroshi Yoshino in 1992, it inhibits tubulin polymerization due to its reversible binding at colchicine binding site on tubulin20 and exhibits potent in vivo antitumor activity against tumor xenografts as well as various rodent tumors21 and is in phase II clinical studies.22 Therefore, a variety of synthetic small molecules that contain different heterocycle scaffolds like 2-anilinopyridine, thiazole, arylindoles, pyrazole, oxadiazoline, imidazoles and oxindoles23–26 have been reported as inhibitors of tubulin polymerization. Recently, we have reported a new family of 2-anilinonicotinyl arylsulfonyl hydrazide and imidazopyridine–oxindole conjugates that exhibit promising cytotoxic activity.27,28 However, several clinically useful tubulin depolymerizing drugs have huge drawbacks. To overcome these problems, we have designed and synthesised a new class of (Z)-3-(arylamino)-1-(2-(arylamino)pyridin-3-yl)prop-2-en-1-one derivatives (9a–p) by keeping structural aspects of combretastatin A-4 (II) and E-7010 (III). Further, evaluated them for their cytotoxicity against various selected human cancer cell lines. These compounds also showed significant inhibition of tubulin polymerization.
Results and discussion
Chemistry
Synthesis of the (Z)-3-(arylamino)-1-(2-(arylamino)pyridin-3-yl)prop-2-en-1-one conjugates (9a–p) is shown in Scheme 1. The esterification of 2-chloronicotinic acid in presence of sulphuric acid in ethanol gave ethyl 2-chloronicotinate (2) followed by nucleophilic substitution with substituted aromatic amines 3a–d provided ethyl 2-(arylamino)nicotinates (4a–d) in quantitative yield. This upon reduction with LAH in THF afforded (2-(arylamino)pyridin-3-yl)methanol intermediates (5a–d), followed by oxidation with IBX produces aldehydes 6a–d. These aldehydes 6a–d were treated with ethynylmagnesium bromide in THF to obtain the intermediates 7a–d followed by once again oxidation with IBX in DMSO provided the corresponding precursors 8a–d. Consequently, the target conjugates 9a–p were formed by reaction between corresponding precursors 8a–d and arylamines in ethanol (Table 1).
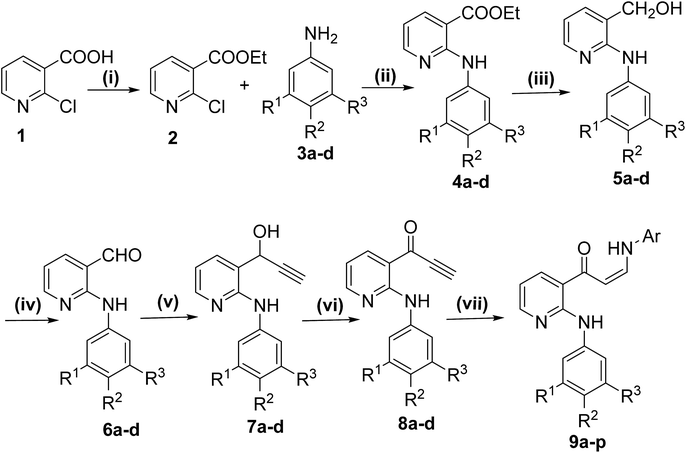 |
| Scheme 1 Reagents & conditions: (i) conc. H2SO4, ethanol, rt, 75–85%; (ii) ethylene glycol, 120 °C, 5–6 h, 70–80%; (iii) LAH, THF, rt, 3–4 h, 70–75%; (iv) IBX, DMSO, 0 °C, 2 h, 65%; (v) ethynylmagnesium bromide, THF, 0 °C, rt, 3 h, 55–65%; (vi) IBX, DMSO, 0 °C, 2 h, 75%; (vii) arylamines, EtOH, 4 h, rt, 55–60%. | |
Table 1 Structures of compounds 9a–p melting points and their yields
Entry |
Ar |
R1 |
R2 |
R3 |
Melting point (°C) |
Yield% (mg) |
9a |
5-Indolyl |
H |
OCH3 |
H |
162–164 |
68% (93) |
9b |
6-Indolyl |
H |
OCH3 |
H |
227–229 |
72% (99) |
9c |
3,5-Dimethoxyphenyl |
H |
OCH3 |
H |
238–240 |
80% (116) |
9d |
4-Methoxyphenyl |
H |
OCH3 |
H |
260–262 |
79% (106) |
9e |
5-Indolyl |
OCH3 |
OCH3 |
OCH3 |
139–141 |
81% (127) |
9f |
6-Indolyl |
OCH3 |
OCH3 |
OCH3 |
156–158 |
69% (108) |
9g |
4-Methoxyphenyl |
OCH3 |
OCH3 |
OCH3 |
197–199 |
70% (107) |
9h |
Trimethoxyphenyl |
OCH3 |
OCH3 |
OCH3 |
242–244 |
79% (138) |
9i |
5-Indolyl |
H |
F |
H |
134–136 |
76% (106) |
9j |
3,5-Dimethoxyphenyl |
H |
F |
H |
196–198 |
65% (95) |
9k |
4-Methoxyphenyl |
H |
F |
H |
290–292 |
81% (110) |
9l |
Trimethoxyphenyl |
H |
F |
H |
291–293 |
80% (127) |
9m |
4-Trifluoromethylphenyl |
H |
F |
H |
279–281 |
78% (117) |
9n |
5-Indolyl |
H |
H |
H |
278–280 |
75% (119) |
9o |
6-Indolyl |
H |
H |
H |
152–154 |
79% (126) |
9p |
Trimethoxyphenyl |
H |
H |
H |
165–167 |
71% (129) |
Biological studies
Antiproliferative activity. Compounds 9a–p have been evaluated for their cytotoxicity in selected human cancer cell lines of MCF-7 (breast), A549 (lung), HCT116 (colon) and Hela (cervix) by using MTT assay method. Some of these (Z)-3-(aryl amino)-1-(2-(aryl amino)pyridin-3-yl)prop-2-en-1-ones (9a, 9c, 9g and 9j) exhibited significant cytotoxic activity against various cancer cell line (IC50 range 0.51–2.23 μM). From the structure activity relationship (SAR) studies, some interesting trends have been observed in these compounds. With respect to substitution 4-methoxy on the B-ring derivatives, with 5-indolyl aryl group containing compound (9a) showed promising cytotoxic activity against most of the cancer cell lines compared to 9b, which contain 6-indolyl aryl group and similar results were appeared in compounds 9e, 9f, 9n and 9o and these results confirms that 5-indolyl aryl group is essential for activity. Compounds 9c and 9j which includes 3,5-dimethoxyphenyl group at aryl (Ar) position showed potent anticancer activity with IC50 ranging from 0.90 to 2.23 μM. 3,4,5-Trimethoxy substituted on B-ring with 4-methoxy phenyl group at aryl (Ar) position compound 9g showed potent anticancer activity against tested cancer cell lines with IC50 ranging from 0.62–1.39 μM. Particularly, compounds 9a, 9g and 9j were shown promising anticancer activity than positive control, nocodazole (III) against all tested cell lines (Table 2). These encouraging cytotoxicity results of compounds 9a, 9g and 9j promoted us to understand their effect on cell cycle and tubulin polymerization.
Table 2 IC50 valuesa (in μM) for compounds 9a–p in selected human cancer cell lines
Comp. |
IC50 valuesa (μM) |
A549b |
Helac |
MCF-7d |
HCT116e |
50% growth inhibition and the values are mean of three determinations. Lung cancer cell line. Cervical cancer cell line. Breast cancer cell line. Colon cancer cell line. Not tested. |
9a |
0.51 ± 0.11 |
0.65 ± 0.08 |
0.71 ± 0.23 |
0.99 ± 0.20 |
9b |
1.47 ± 0.51 |
1.33 ± 0.31 |
1.37 ± 0.06 |
3.31 ± 0.29 |
9c |
1.52 ± 0.13 |
1.39 ± 0.05 |
2.23 ± 0.28 |
2.05 ± 0.11 |
9d |
4.36 ± 0.21 |
4.58 ± 0.09 |
2.85 ± 0.11 |
4.14 ± 0.22 |
9e |
1.94 ± 0.06 |
2.27 ± 0.37 |
2.42 ± 0.09 |
2.49 ± 0.32 |
9f |
3.17 ± 0.61 |
3.32 ± 0.29 |
2.81 ± 0.37 |
5.06 ± 0.40 |
9g |
1.12 ± 0.12 |
1.29 ± 0.18 |
0.62 ± 0.21 |
1.39 ± 0.43 |
9h |
4.61 ± 0.31 |
4.10 ± 0.36 |
3.38 ± 0.08 |
5.35 ± 0.39 |
9i |
2.81 ± 0.28 |
2.69 ± 0.21 |
2.33 ± 0.13 |
3.01 ± 0.26 |
9j |
0.91 ± 0.05 |
0.90 ± 0.27 |
1.21 ± 0.19 |
1.16 ± 0.19 |
9k |
5.57 ± 0.71 |
2.56 ± 0.16 |
2.78 ± 0.28 |
6.01 ± 0.38 |
9l |
5.73 ± 0.39 |
4.86 ± 0.39 |
2.21 ± 0.65 |
3.78 ± 0.08 |
9m |
3.37 ± 0.25 |
5.16 ± 0.17 |
2.09 ± 0.31 |
3.86 ± 0.16 |
9n |
2.67 ± 0.17 |
2.38 ± 0.17 |
2.17 ± 0.29 |
3.02 ± 0.21 |
9o |
4.79 ± 0.18 |
4.01 ± 0.08 |
3.18 ± 0.07 |
3.81 ± 0.38 |
9p |
5.61 ± 0.09 |
6.75 ± 0.16 |
4.47 ± 0.05 |
7.53 ± 0.71 |
Noc (III) |
1.50 ± 0.0.6 |
0.92 ± 0.37 |
1.80 ± 0.19 |
—f |
Effect on cell cycle arrest. To examine the mechanism underlying the antiproliferative activities of these (Z)-3-(aryl amino)-1-(2-(aryl amino)pyridin-3-yl)prop-2-en-1-ones (9a–p), we analyzed the cell cycle alterations by treating A549 cells with some of the potential compounds like 9a, 9g and 9j at 3 μM concentration for 24 h to know the phase distribution by flow cytometry. They caused G2/M cell-cycle arrest with a large accumulation of cells 76.42%, 75.24% and 75.56% respectively in the G2/M phase (Fig. 2).
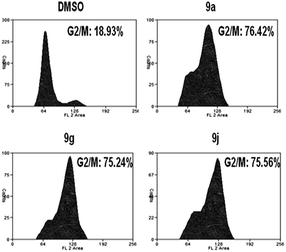 |
| Fig. 2 Anti-mitotic effect of 9a, 9g and 9j by FACS analysis. Induction of cell cycle G2/M arrest by compounds 9a, 9g and 9j. A549 cells were harvested after treatment at 3 μM for 24 h. Untreated cells and DMSO treated cells served as controls. The percentage of cells in each phase of cell cycle was quantified by flow cytometry. | |
Effect on microtubule network. Compounds that alter cell-cycle factors with preferential G2/M phase are known to exhibit effects on tubulin assembly. Furthermore, inhibition of tubulin polymerization is strongly correlated with G2/M cell-cycle arrest.29 Therefore as these compounds 9a, 9g and 9j exhibited G2/M cell-cycle arrest, it was considered of interest to understand the microtubule inhibitory function. Thus, A549 cells were treated with 9a, 9g and 9j at 3 μM for 24 h and the results indicate that there is dispersed microtubule organization relative to the control cells, as observed by immunofluorescence and microscopy studies using anti-α-tubulin antibody (Fig. 3).
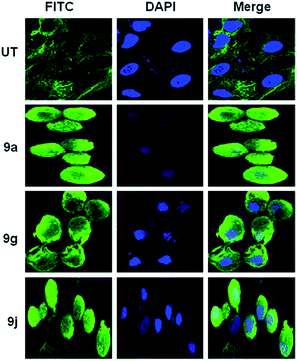 |
| Fig. 3 Effect of 9a, 9g and 9j on microtubules and nuclear condensation. A549 cells were treated with 9a, 9g and 9j concentrations of 3 μM for 24 h, followed by fixation, permeabilization and indirect immune fluorescent analysis with an anti-α-tubulin–FITC. Nuclei were stained with DAPI. The merged images of cells stained for tubulin and DAPI are represented. | |
Effect on inhibition of tubulin polymerization. To investigate whether the antiproliferative activity of these synthesized conjugates (9a, 9g and 9j) has any relationship to the interaction with tubulin, we evaluated their effect on the inhibition of tubulin polymerization. Among these compounds, 9a exhibited potent inhibition of microtubule assembly with IC50 value 1.34 μM. However, compounds 9g and 9j also appreciably inhibited tubulin polymerization with IC50 values 5.68 and 5.34 μM respectively (Table 3). Thus, these results suggest that 9a is a potent inhibitor of tubulin polymerization than nocodazole, a known tubulin polymerization inhibitor and similar to combretastatin A-4.23
Table 3 Effect of compounds 9a, 9g and 9j on tubulin polymerization
Compound |
Inhibition of tubulin polymerizationa, IC50 (μM) |
Compounds (final concentration: 3 μM and final volume: 10 μL) were pre-incubated with tubulin at a final conc. of 10 μm. |
9a |
1.34 ± 0.3 |
9g |
5.68 ± 1.0 |
9j |
5.34 ± 1.0 |
CA-4 |
1.30 ± 0.12 |
Noc (III) |
2.64 ± 0.4 |
Analysis of free versus polymerized tubulin by western blotting. The microtubules continuously undergo polymerization and depolymerization which are mediated by α and β-tubulin, and changing this dynamic balance is considered as a potential target for cancer drug development (e.g.; paclitaxel or colchicines).30,31 In order to extend the in vitro effects of these conjugates on tubulin polymerization to the cellular effects i.e., ratio of free vs. polymerized tubulin. Western blot analysis of α-tubulin in A549 cells was performed by treating the cells with nocodazole, paclitaxel, 9a, 9g and 9j at 2 μM for 24 h. Immunoblot results show that the cells exposed to these compounds 9a contain more tubulin in the soluble fraction of the cells, when compared to insoluble fraction. Hence, increased tubulin in soluble fraction of cells treated by these conjugates also supports the inhibition of tubulin polymerization (Fig. 4).
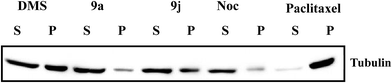 |
| Fig. 4 Distribution of tubulin in polymerized vs. soluble fractions as analyzed by immunoblotting in 9a and 9j treated A549 cells. A549 cells were treated with 2 μM of 9a, 9j, nocodazole (III) and paclitaxel for 24 h. The fractions containing soluble and polymerised tubulin were collected and separated by SDS-PAGE. Tubulin was detected by immunoblot analysis. | |
Hoechst staining. Apoptosis is one of the major pathways that leads to the process of cell death. Chromatin condensation and fragmented nuclei are known as the classic characteristics of apoptosis.32 It was considered of interest to investigate the apoptotic inducing effect of the potent compounds 9a, 9g and 9j by Hoechst staining (H 33258) method in A549 cancer cell line. Therefore cells were treated with 9a, 9g and 9j at 2 μM concentrations for 48 h. Manual field quantification of apoptotic cells based on cytoplasmic condensation, presence of apoptotic bodies, nuclear fragmentation and relative fluorescence of the test compounds (9a, 9g and 9j) revealed that there was significant increase in the percentage of apoptotic cells as shown in Fig. 5.
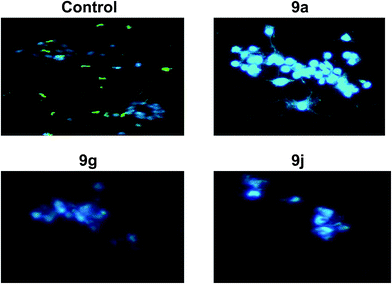 |
| Fig. 5 Hoechst staining of 9a, 9g and 9j in A549 cells at 2 μM concentration. | |
Caspase-3 activation. It is well known that the cell cycle arrest at G2/M phase is shown to induce cellular apoptosis, hence it was considered of interest to examine whether the cytotoxicity of 9a, 9g and 9j is by virtue of apoptotic cell death.33 Cysteine aspartase group namely, caspases play a crucial role in the induction of apoptosis and amongst them caspase-3 happens to be one of the effector caspase. This prompted us to treat A549 cells with compounds 9a, 9g and 9j to examine the activation of caspase-3. The results indicate that there is nearly 3 to 4-fold induction in caspase-3 activity in cells treated with 2 μM concentration by these compounds when compared to control, thus suggesting the activation of caspase-3 by 9a, 9g and 9j indicate that they have the ability to induce apoptosis in A549 cells (Fig. 6).
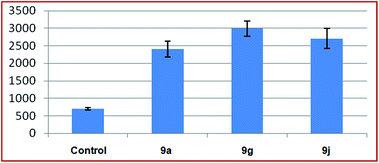 |
| Fig. 6 Effect of compounds 9a, 9g and 9j on caspase-3 activity: A549 cells were treated for 48 h with 2 μM concentrations of compounds 9a, 9g and 9j. Values indicated are the mean_SD of two different experiments performed in triplicates. | |
Competitive tubulin-binding assay. To confirm the above observations, we investigated the effect of 9a, 9g and 9j on tubulin polymerization using an in vitro tubulin polymerization assay. Compounds 9a, 9g and 9j inhibited polymerization of tubulin similar to that of nocodazole (I). Therefore, we further assessed the ability of compounds 9a, 9g and 9j to compete with the colchicine for binding to tubulin via competitive binding assays. Nocodazole (I) was used as a positive control and paclitaxel as a negative control. Because the intrinsic fluorescence of colchicine increases upon binding to tubulin,34 it was used as an index for 9a, 9g and 9j competition with colchicine in tubulin binding. As shown in Fig. 7, paclitaxel did not affect the binding to tubulin. However, the fluorescence of colchicine–tubulin complex was reduced in the presence of compounds 9a, 9g, 9j and nocodazole (III) inhibit the binding of colchicine to tubulin, there by suggesting that the compounds 9a, 9g and 9j binds at the colchicine binding site.
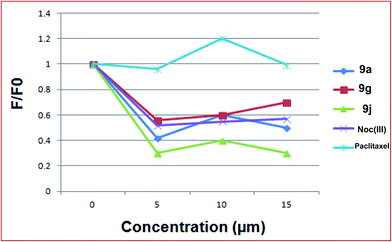 |
| Fig. 7 Fluorescence based colchicine competitive binding assay of conjugates 9a, 9g and 9j were carried out at various concentrations containing 3 μM of tubulin and colchicine for 60 min at 37 °C. Nocodazole (I) was used as a positive control where as taxol was used as negative control which binds at taxane site. Fluorescence values are normalized to control. | |
Docking studies. In order to understand the binding interactions, we have performed the molecular docking study of active compounds obtained from competitive tubulin-binding assay by using Glide 5.8 module in maestro 9.3. These compounds 9a, 9g have been docked against the tubulin protein (PDB code: 3UT5).35 The molecular docking study suggest that these compounds bind in the colchicine binding pocket with hydrogen bonds with the α-chain, β-chain and extensive hydrophobic contacts within the binding pocket of the β-chain. In case of 9a, the methoxy benzene is directed towards Cysβ241, the amino acid with which methoxy groups (ring A) of colchicine form hydrogen bond interactions. The carbonyl group showed hydrogen bonding interaction with Valα181 (2.0 Å) and the –NH group of indole nucleus showed hydrogen bonding interaction with Gluβ247 (1.8 Å). Further, the protein ligand complex was stabilized by hydrophobic interactions like Leuβ242, Alaβ250, Asnβ258, Valβ315, Alaβ316, Ileβ318 and Ileβ378. The trimethoxy benzene ring of 9g showed key hydrogen bond interactions with the side chain –SH of Cysβ241 (hydrogen bond distance 2.2 Å and 2.3 Å). In addition to this –NH group of 9g showed hydrogen bonding interaction with Lysβ254 (2.3 Å). Other residues like Leuβ242, Asnβ258, Valβ315, Alaβ316, Ileβ318 and Ileβ378 were found to be involved in hydrophobic interactions. So, the docking studies revealed that the conjugates 9a and 9g binds to the colchicine binding site of tubulin by forming favourable interactions (Fig. 8).
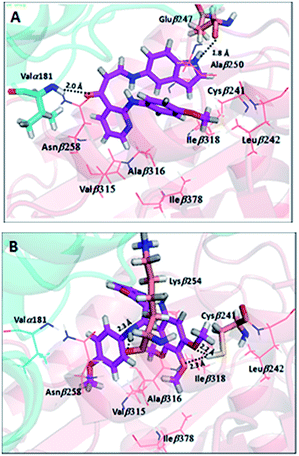 |
| Fig. 8 Binding model of ligands at the interface of α,β-tubulin heterodimer. Ligands are shown in stick models (magenta colour). Hydrogen bonding interactions are shown as black dashes, and residues involved are represented also in stick models, while residues involved in hydrophobic interactions are represented as lines. Cyan and salmon color represents α- and β-tubulin, respectively. (A) Represent the binding mode of 9a and (B) represent the binding mode of 9g. | |
Conclusions
In conclusions, we have designed and synthesized new analogues of E-7010 and tested for their in vitro anticancer activities against variety of different human cancer cell lines. All the compounds showed moderate to good anticancer potency against most of the tested cancer cell lines, however some of the compounds 9a, 9c, 9g and 9j exhibited significant anticancer potency IC50 values in the range of 0.51 to 2.23 μM. This investigation reveals that compounds 9a, 9g and 9j have shown promising anticancer activity in comparison with nocodazole (I). FACS results reveals that compounds 9a, 9g and 9j caused cell cycle arrest and accumulated cells in the G2/M phase. These compounds inhibited tubulin assembly as shown by the tubulin polymerization assay. Docking studies also supported the fact that these compounds binds to colchicines binding site of the tubulin. Interestingly, it was observed that IC50 of compound 9a was less than nocodazole (I), a well known tubulin polymerization inhibitor. Hoechst 33258 staining and DNA fragmentation assay revealed that these compounds induce cell death by apoptosis. Further, activation of caspase-3 also suggested that these compounds produce apoptotic cell death. Based on the results from the biological studies it is evident that compounds 9a, 9g and 9j have the potential to be taken up for further detailed studies either alone or in combination with existing therapies as potential tubulin polymerization inhibitors.
Experimental section
Chemistry
All chemicals were purchased from Spectrochem Pvt. Ltd (Mumbai, India), Aldrich (Sigma-Aldrich, St. Louis, MO, USA), Lancaster (Alfa Aesar, Johnson Matthey Company, Ward Hill, MA, USA) and were used without further purification. Reactions were monitored by TLC, performed on silica gel glass plates containing 60 GF-254, and visualization on TLC was attained by UV light or iodine indicator. Column chromatography was performed with Merck 60–120 mesh silica gel. 1H NMR and 13C NMR spectra were recorded on Bruker UXNMR/XWIN-NMR (300 MHz) instruments. Chemical shifts are reported in parts per million (δ in ppm) relative to the peak for TMS (tetramethylsilane) as an internal standard, coupling constants are reported in hertz (Hz). ESI spectra were recorded on Micro mass, Quattro LC using ESI+ software with capillary voltage 3.98 kV and ESI mode positive ion trap detector. High-resolution mass spectra (HRMS) were recorded on a QSTAR XL Hybrid MS-MS mass spectrometer. Melting points were determined with an electro thermal melting point apparatus and are uncorrected.
Preparation of ethyl 2-chloronicotinate (2)
A solution of 2-chloro nicotinic acid (10 g, 64 mmol) and ethanol (100 mL) was stirred at 0 °C for 15 minutes. To this solution concentrated sulfuric acid (3 mL) was added drop wise and refluxed for 4 h. After completion of reaction (checked by TLC), ethanol was removed by vacuum followed by neutralization with sodium bicarbonate solution and ethyl acetate was added. The organic layer was extracted, washed with water, dried over anhydrous sodium sulphate and evaporated in vacuum to obtain pure ethyl 2-chloronicotinate (2) and it was used directly to next step without further purification.
Preparation of ethyl 2-(aryl amino)nicotinate (4a–d)
A solution of ethyl 2-chloronicotinate and substituted anilines were dissolved in ethylene glycol and heated at 120 °C for 5–6 h. After completion of reaction, the reaction mixture was cooled to room temperature followed by water and ethyl acetate was added. The organic layer was separated, dried over anhydrous Na2SO4, evaporated in vacuum and it was purified by column chromatography (20% ethyl acetate/hexane) to get pure compounds 4a–d.
Ethyl 2-(3,4,5-trimethoxyphenylamino)nicotinate (4a). Ethyl 2-(3,4,5-trimethoxyphenylamino)nicotinate 4a was obtained by using above described method by dissolving ethyl 2-chloronicotinate (3 g, 16.2 mmol) and 3,4,5-trimethoxyaniline (2.97 g, 16.2 mmol) in ethylene glycol (20 mL) as yellow coloured solid (4.2 g, yield 78%): 1H NMR (300 MHz, CDCl3); δ 1.39 (t, 3H, J = 7.1 Hz, CH3), 3.83 (s, 3H, –OCH3), 3.91 (s, 6H, –OCH3), 4.21–4.29 (q, 2H, J = 7.1 Hz, CH2), 6.62–6.66 (dd, 1H, J = 2.8 Hz, J = 5.7 Hz, ArH), 7.01 (s, 2H, ArH), 8.20 (dd, 1H, J = 5.7 Hz, J = 2.2 Hz, ArH), 8.31 (dd, 1H, J = 2.8 Hz, J = 1.9 Hz, ArH), 10.01 (s, 1H, NH) ppm; MS (ESI) m/z 333 [M + H].
Ethyl 2-(4-methoxyphenylamino)nicotinate (4b). Ethyl 2-(4-methoxyphenylamino)nicotinate 4b was obtained by using above described method by dissolving ethyl 2-chloronicotinate (3 g, 16.2 mmol) and 4-methoxyaniline (1.99 g, 16.2 mmol) in ethylene glycol (20 mL) as yellow coloured solid (3.57 g, yield 81%): 1H NMR (300 MHz, CDCl3); δ 1.41 (t, 3H, J = 7.1 Hz, CH3), 3.81 (s, 3H, –OCH3), 4.35–4.41 (q, 2H, J = 7.1 Hz, CH2), 6.64–6.67 (dd, 1H, J = 7.8 Hz, J = 4.7 Hz, ArH), 6.90 (d, 2H, J = 9.0 Hz, ArH), 7.53 (d, 2H, J = 9.0 Hz, ArH), 8.22 (dd, 1H, J = 7.8 Hz, J = 2.1 Hz, ArH), 8.32 (dd, 1H, J = 2.0 Hz, J = 4.7 Hz, ArH), 9.96 (s, 1H, NH) ppm; MS (ESI) m/z 273 [M + H].
Ethyl 2-(4-fluorophenylamino)nicotinate (4c). Ethyl 2-(4-fluorophenylamino)nicotinate 4c was obtained by using above described method by dissolving ethyl 2-chloronicotinate (3 g, 16.2 mmol) and 4-fluoroaniline (1.80 g, 16.2 mmol) in ethylene glycol (20 mL) as yellow coloured solid (3.08 g, 73%): 1H NMR (300 MHz, CDCl3); δ 1.42 (t, 3H, J = 7.2 Hz, CH3), 4.36–4.41 (q, 2H, J = 7.2 Hz, CH2), 6.69–6.72 (dd, 1H, J = 5.6 Hz, J = 1.9 Hz, ArH), 7.00–7.06 (m, 2H, ArH), 7.62 (dd, 2H, J = 4.3 Hz, J = 4.7 Hz, ArH), 8.24 (dd, 1H, J = 5.6 Hz, J = 2.1 Hz, ArH), 8.34 (dd, 1H, J = 2.7 Hz, J = 1.9 Hz, ArH), 10.12 (s, 1H, NH) ppm; MS (ESI) m/z 261 [M + H].
Ethyl 2-(phenylamino)nicotinate (4d). Ethyl 2-(phenylamino)nicotinate 4d was obtained by using above described method by dissolving ethyl 2-chloronicotinate (2.5 g, 13.5 mmol) and aniline (1.26 g, 13.5 mmol) in ethylene glycol (20 mL) as yellow coloured solid (2.49 g, yield 76%): 1H NMR (300 MHz, CDCl3); δ 1.41 (t, 3H, J = 7.2 Hz, CH3), 4.34–4.43 (q, 2H, J = 7.2 Hz, CH2), 6.68–6.73 (dd, 1H, J = 5.8 Hz, J = 2.8 Hz, ArH), 7.05 (t, 1H, J = 7.4 Hz, ArH), 7.34 (t, 2H, J = 7.5 Hz, ArH), 7.70 (d, 2H, J = 7.7 Hz, ArH), 8.24 (dd, 1H, J = 5.8 Hz, J = 2.1 Hz, ArH), 8.37 (dd, 1H, J = 2.8 Hz, J = 1.9 Hz, ArH), 10.20 (s, 1H, NH) ppm; MS (ESI) m/z 243 [M + H].
General method for synthesis of (2-(aryl amino)pyridin-3-yl)methanol (5a–d)
To the ethyl 2-(aryl amino)nicotinate 4a–d, obtained in the above step was added LiAH4 (0.5 mol) in dry THF at 0 °C and stirred for 2 h at room temperature. Added saturated NH4Cl solution drop wise to quench the unreacted LiAlH4 and removed the THF under vacuum then extracted with ethyl acetate. The organic layer was dried on anhydrous NaSO4 and evaporated ethyl acetate to obtain brown solid products of (2-(aryl amino)pyridin-3-yl)methanol 5a–d (yield 73–81%). The alcohols produced in this step were pure, and no further purification was required. These compounds were taken as such for the next step.
(2-(3,4,5-Trimethoxyphenylamino)pyridin-3-yl)methanol (5a). (2-(3,4,5-Trimethoxyphenylamino)pyridin-3-yl)methanol 5a was obtained according to above described method by adding ethyl 2-(3,4,5-trimethoxyphenylamino)nicotinate 4a (4.1 g, 12 mmol) to LiAlH4 (234 mg, 6 mmol) in THF (30 mL) as brown coloured solid, (2.65 g, yield 74%): 1H NMR (300 MHz, CDCl3); δ 3.83 (s, 3H, OCH3), 3.89 (s, 6H, OCH3), 4.62 (s, 2H, –CH2OH), 6.61–6.65 (dd, 1H, J = 3.1 Hz, J = 6.6 Hz, ArH), 6.92 (s, 2H, ArH), 7.21–7.26 (m, 1H, ArH), 7.80 (brs, 1H, NH), 8.16 (dd, 1H, J = 3.1 Hz, J = 1.9 Hz, ArH); ppm; MS (ESI) m/z 291 [M + H].
(2-(4-Methoxyphenylamino)pyridin-3-yl)methanol (5b). (2-(4-Methoxyphenylamino)pyridin-3-yl)methanol 5b was obtained according to above described method by adding ethyl 2-(4-methoxyphenylamino)nicotinate 4b (3.4 g, 12.5 mmol) to LiAlH4 (238 mg, 6 mmol) in THF (30 mL) as brown coloured solid, (2.01 g, yield 70%); 1H NMR (300 MHz, DMSO-d6); δ 3.78 (s, 3H, OCH3), 4.60 (s, 2H, –CH2OH), 6.58–6.66 (dd, 1H, J = 2.1 Hz, J = 5.1 Hz, ArH), 6.87 (d, 2H, J = 9.0 Hz, ArH), 7.24–7.29 (dd, 1H, J = 1.7 Hz, J = 5.1 Hz, ArH), 7.40 (d, 2H, J = 9.0 Hz, ArH), 7.46 (brs, 1H, NH), 8.09 (dd, 1H, J = 3.2 Hz, J = 1.9 Hz, ArH); ppm; MS (ESI) m/z 231 [M + H].
2-(4-Fluorophenylamino)pyridin-3-ylmethanol (5c). 2-(4-Fluorophenylamino)pyridin-3-ylmethanol 5c was obtained according to above described method by adding ethyl 2-(4-fluorophenylamino)nicotinate 4c (3.0 g, 11.5 mmol) to LiAlH4 (219 mg, 6 mmol) in THF (30 mL) as brown coloured solid, (1.99 g, yield 79%); 1H NMR (300 MHz, DMSO-d6); δ 4.59 (s, 2H, –CH2OH), 6.62–6.69 (dd, 1H, J = 4.5 Hz, J = 2.3 Hz, ArH), 6.94–7.04 (m, 2H, ArH), 7.24–7.29 (dd, 1H, J = 5.3 Hz, J = 1.5 Hz, ArH), 7.39–7.48 (dd, 2H, J = 9.0 Hz, J = 4.5 Hz, ArH), 7.61 (brs, 1H, NH), 8.10 (dd, 1H, J = 3.0 Hz, J = 2.3 Hz, ArH); ppm; MS (ESI) m/z 219 [M + H].
(2-(Phenyl amino) pyridin-3-yl)methanol (5d). (2-(Phenyl amino)pyridin-3-yl)methanol 5d was obtained according to above described method by adding ethyl 2-(phenyl amino)nicotinate 4d (2.4 g, 10 mmol) to LiAlH4 (188 mg, 5 mmol) in THF (30 mL) as brown coloured solid, (1.45 g, yield 73%); 1H NMR (300 MHz, CDCl3); δ 4.66 (s, 2H, –CH2OH), 6.67–6.71 (dd, 1H, J = 5.0 Hz, J = 2.1 Hz, ArH), 6.99 (t, 1H, J = 7.4 Hz, ArH), 7.28–7.34 (m, 3H, ArH), 7.54 (d, 2H, J = 8.1 Hz, ArH), 7.68 (brs, 1H, NH), 8.15–8.18 (dd, 1H, J = 1.7 Hz, J = 5.0 Hz, ArH); ppm; MS (ESI) m/z 201 [M + H].
General method for synthesis of 2-(aryl amino)nicotinaldehyde (6a–d)
To the (2-(aryl amino) pyridin-3-yl)methanol 5a–d produced in the above step was added IBX (1.2 mol) in DMSO and stirred for 1 h at room temperature. Added ice cold water to the reaction mixture and extracted with ethyl acetate. The organic layer was dried on anhydrous NaSO4 and evaporated the ethyl acetate to obtain pure corresponding 2-(aryl amino)nicotinaldehyde 6a–d in good yields (72–81%).
2-(3,4,5-Trimethoxyphenylamino)nicotinaldehyde (6a). 2-(3,4,5-Trimethoxyphenylamino)nicotinaldehyde 6a was prepared according to above explained method by the addition of (2-(3,4,5-trimethoxyphenylamino)pyridin-3-yl)methanol 5a (2.4 g, 8.3 mmol) to IBX (3.47 g 12.4 mmol) in DMSO solution. Yellow coloured solid; (1.88 g, yield 79%); 1H NMR (300 MHz, CDCl3); δ 3.84 (s, 3H, OCH3), 3.89 (s, 6H, OCH3), 6.83–6.87 (dd, 1H, J = 4.9 Hz, J = 2.7 Hz, ArH), 7.04 (s, 2H, ArH), 7.86–7.89 (dd, 1H, J = 5.6 Hz, J = 2.0 Hz, ArH), 8.41–8.44 (dd, 1H, J = 2.9 Hz, J = 2.0 Hz, ArH), 9.89 (s, 1H, CHO), 10.38 (brs, 1H, NH); ppm; MS (ESI) m/z 289 [M + H].
2-(4-Methoxyphenylamino)nicotinaldehyde (6b). 2-(4-Methoxyphenylamino)nicotinaldehyde 6b was prepared according to above explained method by the addition of (2-(4-methoxyphenylamino)pyridin-3-yl)methanol 5b (1.9 g, 8.3 mmol) to IBX (3.47 g 12.4 mmol) in DMSO solution. Yellow colored solid; (1.36 g, yield 72%): 1H NMR (300 MHz, CDCl3); δ 3.82 (s, 3H, OCH3), 6.75–6.81 (dd, 1H, J = 5.3 Hz, J = 2.3 Hz, ArH), 6.92 (d, 2H, J = 9.0 Hz, ArH), 7.58 (d, 2H, J = 9.0 Hz, ArH), 7.82–7.87 (dd, 1H, J = 6.0 Hz, J = 1.5 Hz, ArH), 8.36–8.39 (dd, 1H, J = 3.0 Hz, J = 1.5 Hz, ArH), 9.87 (s, 1H, CHO), 10.27 (brs, 1H, NH); ppm; MS (ESI) m/z 229 [M + H].
2-(4-Fluorophenylamino)nicotinaldehyde (6c). 2-(4-Fluorophenylamino)nicotinaldehyde 6c was prepared according to above explained method by the addition of (2-(4-fluorophenylamino)pyridin-3-yl)methanol 5c (1.9 g, 8.7 mmol) to IBX (3.66 g 13 mmol) in DMSO solution. Yellow colored solid; (1.50 g, yield 81%): 1H NMR (300 MHz, CDCl3); δ 6.64–6.70 (dd, 1H, J = 6.1 Hz, J = 1.8 Hz, ArH), 6.91 (d, 2H, J = 7.6 Hz, ArH), 7.21–7.29 (m, 2H, ArH), 7.67 (dd, 1H, J = 6.1 Hz, J = 2.0 Hz, ArH), 8.39 (dd, 1H, J = 3.1 Hz, J = 1.8 Hz, ArH), 9.81 (s, 1H, CHO), 10.35 (brs, 1H, NH); ppm; MS (ESI) m/z 217 [M + H].
2-(Phenyl amino)nicotinaldehyde (6d). 2-(Phenyl amino)nicotinaldehyde 6d was prepared according to above explained method by the addition of (2-(phenyl amino)pyridin-3-yl)methanol 5d (1.4 g, 7 mmol) to IBX (2.93 g 10.5 mmol) in DMSO solution. Yellow coloured solid; (1.00 g, yield 72%): 1H NMR (300 MHz, CDCl3); δ 6.82–6.86 (dd, 1H, J = 4.9 Hz, J = 2.7 Hz, ArH), 7.10 (t, 1H, J = 7.5 Hz, ArH), 7.36 (t, 2H, J = 7.5 Hz, ArH), 7.74 (d, 2H, J = 7.6 Hz, ArH), 7.85–7.88 (dd, 1H, J = 5.5 Hz, J = 2.0 Hz, ArH), 8.40–8.43 (dd, 1H, J = 2.9 Hz, J = 2.0 Hz, ArH), 9.88 (s, 1H, CHO), 10.44 (brs, 1H, NH); ppm; MS (ESI) m/z 199 [M + H].
General procedure for the synthesis of 1-(2-(arylphenylamino)pyridin-3-yl)prop-2-yn-1-ol (7a–d)
A solution of aldehydes 6a–d in dry tetrahydrofuran (THF) was added to stirred solution of ethynylmagnesium bromide in tetrahydrofuran (0.5 M) at 0 °C for 2 h and then stirred at room temperature for another 5–6 h. After completion of reaction saturated aqueous ammonium chloride solution 5–10 mL was added, and the THF was removed in vacuum followed by ethyl acetate was added. The organic layer was extracted and washed with brine solution, dried over anhydrous Na2SO4 and evaporated in vacuum to obtain pure compounds and these were used for next step without purification.
1-(2-(3,4,5-Trimethoxyphenylamino)pyridin-3-yl)prop-2-yn-1-ol (7a). 1-(2-(3,4,5-Trimethoxyphenylamino)pyridin-3-yl)prop-2-yn-1-ol 7a was obtained using above described method by adding 2-(3,4,5-trimethoxyphenylamino)nicotinaldehyde 6a (1.75 g, 6 mmol) to the ethynylmagnesium bromide (18.2 mL, 9 mmol) in dry tetrahydrofuran solution as brown solid (1.32 g, 69%): 1H NMR (300 MHz, CDCl3); δ 2.79 (d, 1H, J = 2.1 Hz,
H), 3.81 (s, 3H, OCH3), 3.84 (s, 6H, OCH3), 5.47 (d, 1H, J = 2.0 Hz, H–C–OH), 6.73–6.77 (dd, 1H, J = 4.9 Hz, J = 2.4 Hz, ArH), 6.78 (s, 2H, ArH), 7.57 (brs, 1H, NH), 7.71–7.75 (dd, 1H, J = 5.8 Hz, J = 1.5 Hz, ArH), 8.17–8.20 (dd, 1H, J = 4.9 Hz, J = 1.6 Hz, ArH) ppm; MS (ESI) m/z 315 [M + H].
1-(2-(4-Methoxyphenylamino)pyridin-3-yl)prop-2-yn-1-ol (7b). 1-(2-(4-Methoxyphenylamino)pyridin-3-yl)prop-2-yn-1-ol 7b was obtained using above described method by adding 2-(4-methoxyphenylamino)nicotinaldehyde 6b (1.25 g, 5.5 mmol) to the ethynylmagnesium bromide (16.4 mL, 8 mmol) in dry tetrahydrofuran solution as brown solid (920 mg, yield 66%): 1H NMR (300 MHz, CDCl3); δ 2.70 (d, 1H, J = 2.2 Hz,
H), 3.87 (s, 3H, OCH3), 6.03–6.11 (m, 1H, H–C–OH), 6.88–6.97 (dd, 1H, J = 6.8 Hz, J = 6.0 Hz, ArH), 6.99–7.10 (m, 2H, ArH), 7.27–7.40 (m, 1H, ArH), 7.55–7.68 (m, 3H, ArH, NH), 8.71–8.82 (dd, 1H, J = 6.8 Hz, J = 6.6 Hz, ArH); ppm; MS (ESI) m/z 255 [M + H].
1-(2-(4-Fluorophenylamino)pyridin-3-yl)prop-2-yn-1-ol (7c). 1-(2-(4-Fluorophenylamino)pyridin-3-yl)prop-2-yn-1-ol 7c was obtained using above described method by adding 2-(4-fluorophenylamino)nicotinaldehyde 6c (1.40 g, 6.5 mmol) to the ethynylmagnesium bromide (19.4 mL, 9.3 mmol) in dry tetrahydrofuran solution as brown solid (960 mg, yield 61%): 1H NMR (300 MHz, CDCl3); δ 2.77 (d, 1H, J = 2.3 Hz,
H), 5.43 (d, 1H, J = 1.5 Hz, H–C–OH), 6.71–6.78 (dd, 1H, J = 5.3 Hz, J = 2.3 Hz, ArH), 6.95–7.04 (m, 2H, ArH), 7.38–7.46 (dd, 2H, J = 4.5 Hz, J = 9.0 Hz, ArH), 7.54 (brs, 1H, NH), 7.67–7.73 (dd, 1H, J = 6.0 Hz, J = 1.5 Hz, ArH), 8.13–8.18 (dd, 1H, J = 3.0 Hz, J = 1.5 Hz, ArH); ppm; MS (ESI) m/z 243 [M + H].
1-(2-(Phenylamino)pyridin-3-yl)prop-2-yn-1-ol (7d). 1-(2-(Phenylamino)pyridin-3-yl)prop-2-yn-1-ol 7d was obtained using above described method by adding 2-(phenylamino)nicotinaldehyde 6d (900 mg, 4.5 mmol) to the ethynylmagnesium bromide (13.6 mL, 7 mmol) in dry tetrahydrofuran solution as brown solid (710 mg, yield 70%): 1H NMR (300 MHz, CDCl3); δ 2.79 (d, 1H, J = 2.3 Hz,
H), 5.48 (d, 1H, J = 2.1 Hz, H–C–OH), 6.73–6.79 (dd, 1H, J = 5.1 Hz, J = 2.5 Hz, ArH), 7.00 (t, 1H, J = 7.4 Hz, ArH), 7.31 (t, 2H, J = 7.6 Hz, ArH), 7.51 (d, 2H, J = 7.6 Hz, ArH), 7.61 (brs, 1H, NH), 7.72–7.78 (dd, 1H, J = 5.8 Hz, J = 1.7 Hz, ArH), 8.18–8.28 (dd, 1H, J = 3.2 Hz, J = 1.7 Hz, ArH); ppm; MS (ESI) m/z 225 [M + H].
General method for synthesis of 1-(2-(arylamino)pyridin-3-yl)prop-2-yn-1-one (8a–d)
A solution of 2-iodoxy benzoic acid (quantity) and dimethyl sulfoxide (DMSO) (quantity) was stirred for 10 min at room temperature until homogeneous solution. A solution of alcohols 7a–d in dimethyl sulfoxide was added slowly, and it was stirred for 4–5 h. After completion of reaction, ice water was added to reaction mixture and the mixture was stirred for another 10 min. To this mixture ethyl acetate was added and filtered through celite. The organic layer was separated and washed with water subsequently saturated Na2CO3 solution and brine, after that dried over anhydrous Na2SO4 and evaporated in vacuum to attain the pure compound and was used directly for next step.
1-(2-(3,4,5-Trimethoxyphenylamino)pyridin-3-yl)prop-2-yn-1-one (8a). 1-(2-(3,4,5-Trimethoxyphenylamino)pyridin-3-yl)prop-2-yn-1-one 8a was obtained using above described method by adding 1-(2-(3,4,5-trimethoxyphenylamino)pyridin-3-yl)prop-2-yn-1-ol 7a (1.20 g, 3.8 mmol) to the 2-iodoxy benzoic acid (1.60 g, 5.7 mmol) in dimethyl sulfoxide solution as yellow solid (970 mg, yield 81%): 1H NMR (300 MHz, CDCl3); δ 3.55 (s, 1H,
H), 3.84 (s, 3H, –OCH3), 3.89 (s, 6H, –OCH3), 6.78–6.84 (dd, 1H, J = 5.3 Hz, J = 3.0 Hz, ArH), 6.99 (s, 2H, ArH), 8.42–8.46 (dd, 1H J = 2.2 Hz, J = 2.3 Hz, ArH), 8.47–8.51 (dd, 1H, J = 6.0 Hz, J = 2.3 Hz, ArH), 10.74 (brs, 1H, NH) ppm; MS (ESI) m/z 313 [M + H].
1-(2-(4-Methoxyphenylamino)pyridin-3-yl)prop-2-yn-1-one (8b). 1-(2-(4-Methoxyphenylamino)pyridin-3-yl)prop-2-yn-1-one 8b was obtained using above described method by adding 1-(2-(4-methoxyphenylamino)pyridin-3-yl)prop-2-yn-1-ol 7b (800 mg, 3.2 mmol) to the 2-iodoxy benzoic acid (1.32 g, 4.7 mmol) in dimethyl sulfoxide solution as yellow solid (600 mg, yield 76%): 1H NMR (300 MHz, CDCl3); δ 3.51 (s, 1H,
H), 3.81 (s, 3H, –OCH3), 6.71–6.78 (dd, 1H, J = 4.5 Hz, J = 3.2 Hz, ArH), 6.92 (d, 2H, J = 8.9 Hz, ArH), 7.55 (d, 2H, J = 8.9 Hz, ArH), 8.36–8.41 (dd, 1H, J = 4.5 Hz, J = 1.9 Hz, ArH), 8.42–8.48 (dd, 1H, J = 5.8 Hz, J = 1.9 Hz, ArH), 10.63 (brs, 1H, NH) ppm; MS (ESI) m/z 253 [M + H].
1-(2-(4-Fluorophenylamino)pyridin-3-yl)prop-2-yn-1-one (8c). 1-(2-(4-Fluorophenylamino)pyridin-3-yl)prop-2-yn-1-one 8c was obtained using above described method by adding 1-(2-(4-fluorophenylamino)pyridin-3-yl)prop-2-yn-1-ol 7c (900 mg, 3.7 mmol) to the 2-iodoxy benzoic acid (1.56 g, 5.6 mmol) in dimethyl sulfoxide solution as yellow solid (640 mg, yield 72%): 1H NMR (300 MHz, CDCl3); δ 3.54 (s, 1H,
H), 6.76–6.84 (dd, 1H, J = 4.5 Hz, J = 3.8 Hz, ArH), 7.00–7.11 (m, 2H, ArH), 7.59–7.68 (dd, 2H, J = 3.7 Hz, J = 9.0 Hz, ArH), 8.38–8.42 (dd, 1H, J = 3.0 Hz, J = 1.5 Hz, ArH), 8.45–8.50 (dd, 1H, J = 6.0 Hz, J = 1.5 Hz, ArH), 10.72 (brs, 1H, NH) ppm; MS (ESI) m/z 241 [M + H].
1-(2-(Phenylamino)pyridin-3-yl)prop-2-yn-1-one (8d). 1-(2-(Phenylamino)pyridin-3-yl)prop-2-yn-1-one 8d was obtained using above described method by adding 1-(2-(phenylamino)pyridin-3-yl)prop-2-yn-1-ol 7d (600 mg, 2.7 mmol) to the 2-iodoxy benzoic acid (1.12 g, 4 mmol) in dimethyl sulfoxide solution as yellow solid (420 mg, yield 70%): 1H NMR (300 MHz, CDCl3); δ 3.50 (s, 1H,
H), 6.71–6.76 (m, 1H, ArH), 7.05 (t, 1H, J = 7.3 Hz, ArH), 7.39 (t, 2H, J = 7.2 Hz, ArH), 7.45 (d, 2H, J = 7.2 Hz, ArH), 8.40–8.46 (dd, 1H, J = 5.6 Hz, J = 1.5 Hz, ArH), 8.48–8.56 (dd, 1H, J = 4.5 Hz, J = 1.5 Hz, ArH) ppm; MS (ESI) m/z 223 [M + H].
General method for synthesis of (Z)-3-(aryl amino)-1-(2-(aryl amino)pyridin-3-yl)prop-2-en-1-one (9a–p)
The ethynyl ketone (8a–d) and aryl amine were dissolved in absolute ethanol and the mixture was stirred for 4–5 h at room temperature. After the completion of the reaction (confirm by TLC), water was added to the reaction mixture and the crude product filtered. It was recrystallized from methanol to afford pure compound 9a–p.
(Z)-3-(1H-Indol-5-ylamino)-1-(2-(4-methoxyphenylamino)pyridin-3-yl)prop-2-en-1-one (9a). (Z)-3-(1H-Indol-5-ylamino)-1-(2-(4-methoxyphenylamino)pyridin-3-yl)prop-2-en-1-one 9a was obtained according to above described method by using starting materials 1-(2-(4-methoxyphenylamino)pyridin-3-yl)prop-2-yn-1-one 8b (90 mg, 0.4 mmol) and 5-amino indole (47 mg, 0.4 mmol) as yellow solid. 1H NMR (300 MHz, CDCl3); δ 3.81 (s, 3H, OCH3), 5.94 (d, 1H, J = 7.9 Hz, COCH
), 6.54 (s, 1H, ArH), 6.62–6.68 (dd, 1H, J = 3.0 Hz, J = 4.7 Hz, ArH), 6.91 (d, 2H, J = 8.8 Hz, ArH), 7.03 (dd, 1H, J = 6.4 Hz, J = 2.2 Hz, ArH), 7.23–7.27 (m, 1H, ArH), 7.35–7.42 (m, 2H, ArH), 7.50–7.58 (dd, 1H, J = 7.9 Hz, J = 12.6 Hz,
CH–NH), 7.61 (d, 2H, J = 8.8 Hz, ArH), 7.98–8.04 (dd, 1H, J = 1.7 Hz, J = 6.2 Hz, ArH), 8.22 (brs, 1H, NH), 8.25–8.29 (dd, 1H, J = 1.7 Hz, J = 3.0 Hz, ArH), 11.14 (brs, 1H, NH), 12.06 (d, 1H, J = 12.6 Hz, NH–CH
) ppm; 13C NMR (300 MHz, CDCl3): δ 190.2, 154.8, 154.3, 150.5, 136.8, 132.9, 132.0, 127.8, 125.6, 121.7, 114.7, 113.2, 111.7, 106.9, 100.7, 91.82, 54.7; IR (KBr) (νmax/cm−1): 3346, 2923, 2851, 1630, 1576, 1491, 1460, 1282, 1236, 1125, 1005, 769; MS (ESI): 385 [M + H]+; HRMS (ESI): calcd for C23H21N4O2 (M + H)+ 385.1659; found: 385.1658.
(Z)-3-(1H-Indol-6-ylamino)-1-(2-(4-methoxyphenylamino)pyridin-3-yl)prop-2-en-1-one (9b). (Z)-3-(1H-Indol-6-ylamino)-1-(2-(4-methoxyphenylamino)pyridin-3-yl)prop-2-en-1-one 9b was obtained according to above described method by using starting materials 1-(2-(4-methoxyphenylamino)pyridin-3-yl)prop-2-yn-1-one 8b (90 mg, 0.4 mmol) and 6-amino indole (47 mg, 0.4 mmol) as yellow solid. 1H NMR (300 MHz, CDCl3); δ 3.81 (s, 3H, OCH3), 5.96 (d, 1H, J = 7.4 Hz, COCH
), 6.53 (s, 1H, ArH), 6.63–6.67 (m, 1H, ArH), 6.91 (d, 2H, J = 8.9 Hz, ArH), 6.96 (d, 1H, J = 6.7 Hz, ArH), 7.13 (brs, 1H, ArH), 7.18 (t, 1H, J = 6.7 Hz, ArH), 7.51–7.56 (dd, 1H, J = 7.4 Hz, J = 12.1 Hz,
CH–NH), 7.59–7.63 (m, 3H, ArH), 7.99–8.02 (dd, 1H, J = 1.2 Hz, J = 6.7 Hz, ArH), 8.18 (brs, 1H, NH), 8.26–8.28 (dd, 1H, J = 1.5 Hz, J = 3.1 Hz, ArH), 11.13 (brs, 1H, NH), 12.06 (d, 1H, J = 12.1 Hz, NH–CH
) ppm; 13C NMR (300 MHz, CDCl3): δ 192.0, 161.9, 155.6, 151.9, 150.5, 145.0, 144.5, 141.8, 138.0, 136.1, 122.7, 115.4, 115.1, 113.0, 95.8, 95.2, 93.9, 55.5 ppm; IR (KBr) (νmax/cm−1): 3074, 2929, 2835, 1633, 1609, 1589, 1514, 1288, 1236, 1140, 1068, 1012, 824; MS (ESI): 385 [M + H]+; HRMS (ESI): calcd for C23H21N4O2 (M + H)+ 385.1659; found: 385.1657.
(Z)-3-(3,5-Dimethoxyphenylamino)-1-(2-(4-methoxyphenylamino)pyridin-3-yl)prop-2-en-1-one (9c). (Z)-3-(3,5-Dimethoxyphenylamino)-1-(2-(4-methoxyphenylamino)pyridin-3-yl)prop-2-en-1-one 9c was obtained according to above described method by using starting materials 1-(2-(4-methoxyphenylamino)pyridin-3-yl)prop-2-yn-1-one 8b (90 mg, 0.4 mmol) and 3,5-dimethoxyaniline (55 mg, 0.4 mmol) as yellow solid. 1H NMR (300 MHz, CDCl3); δ 3.81 (s, 3H, OCH3), 3.83 (s, 6H, OCH3), 5.98 (d, 1H, J = 7.9 Hz, COCH
), 6.21 (s, 1H, ArH), 6.27 (s, 2H, ArH), 6.64–6.68 (dd, 1H, J = 3.0 Hz, J = 6.4 Hz, ArH), 6.91 (d, 2H, J = 9.0 Hz, ArH), 7.41–7.46 (dd, 1H, J = 7.9 Hz, J = 12.5 Hz,
CH–NH), 7.60 (d, 2H, J = 9.0 Hz, ArH), 8.00–8.04 (dd, 1H, J = 1.1 Hz, J = 6.4 Hz, ArH), 8.26–8.29 (dd, 1H, J = 1.8 Hz, J = 3.0 Hz, ArH), 11.07 (brs, 1H, NH), 11.76 (d, 1H, J = 12.5 Hz, NH–CH
) ppm; 13C NMR (300 MHz, CDCl3): δ 192.0, 161.9, 156.0, 155.5, 152.1, 144.1, 141.9, 137.9, 133.1, 128.5, 123.1, 115.0, 114.7, 114.1, 112.4, 95.7, 95.1, 94.0, 55.45 ppm; IR (KBr) (νmax/cm−1): 3213, 2922, 1621, 1549, 1510, 1460, 1291, 1235, 1107, 1014, 827; MS (ESI): 406 [M + H]+; HRMS (ESI): calcd for C23H24N3O4 (M + H)+ 406.1761; found: 406.1752.
(Z)-3-(4-Methoxyphenylamino)-1-(2-(4-methoxyphenylamino)pyridin-3-yl)prop-2-en-1-one (9d). (Z)-3-(4-Methoxyphenylamino)-1-(2-(4-methoxyphenylamino)pyridin-3-yl)prop-2-en-1-one 9d was obtained according to above described method by using starting materials 1-(2-(4-methoxyphenylamino)pyridin-3-yl)prop-2-yn-1-one 8b (90 mg, 0.4 mmol) and 4-methoxyaniline (44 mg, 0.4 mmol) as yellow solid. 1H NMR (300 MHz, CDCl3); δ 3.82 (s, 6H, OCH3), 5.93 (d, 1H, J = 7.9 Hz, COCH
), 6.63–6.67 (dd, 1H, J = 4.1 Hz, J = 7.5 Hz, ArH), 6.88–6.94 (m, 4H, ArH), 7.08 (d, 2H, J = 9.0 Hz, ArH), 7.37–7.44 (m, 1H,
CH–NH), 7.60 (d, 2H, J = 8.7 Hz, ArH), 8.00 (d, 1H, J = 7.5 Hz, ArH), 8.27 (d, 1H, J = 6.4 Hz, ArH), 11.09 (brs, 1H, NH), 11.89 (d, 1H, J = 12.1 Hz, NH–CH
) ppm; 13C NMR (300 MHz, CDCl3): δ 190.7, 154.7, 150.9, 146.1, 137.2, 133.2, 132.5, 132.5, 128.2, 125.8, 122.3, 115.1, 113.6, 112.2, 112.0, 107.4, 92.2, 55.1; IR (KBr) (νmax/cm−1): 3238, 3002, 2844, 1636, 1498, 1341, 1157, 1140, 1068, 1014, 925, 871; MS (ESI): 376 [M + H]+; HRMS (ESI) calcd for C22H22N3O3 [M + H]+ 376.1655, found: 376.1852.
(Z)-3-(1H-Indol-5-ylamino)-1-(2-(3,4,5-trimethoxyphenylamino)pyridin-3-yl)prop-2-en-1-one (9e). (Z)-3-(1H-Indol-5-ylamino)-1-(2-(3,4,5-trimethoxyphenylamino)pyridin-3-yl)prop-2-en-1-one 9e was obtained according to above described method by using starting materials 1-(2-(3,4,5-trimethoxyphenylamino)pyridin-3-yl)prop-2-yn-1-one 8a (110 mg, 0.35 mmol) and 5-amino indole (47 mg, 0.35 mmol) as yellow solid. 1H NMR (300 MHz, CDCl3); δ 3.84 (s, 3H, OCH3), 3.91 (s, 6H, OCH3), 5.95 (d, 1H, J = 7.9 Hz, COCH
), 6.54 (s, 1H, ArH), 6.67–6.74 (dd, 1H, J = 2.6 Hz, J = 4.9 Hz, ArH), 7.01–7.11 (m, 4H, ArH), 7.36–7.45 (m, 2H, ArH), 7.51–7.61 (dd, 1H, J = 8.1 Hz, J = 12.3 Hz,
CH–NH), 8.04 (d, 1H, J = 6.9 Hz, ArH), 8.23 (brs, 1H, NH), 8.31 (d, 1H, J = 5.8 Hz, ArH), 11.27 (brs, 1H, NH), 12.07 (d, 1H, J = 13.7 Hz, NH–CH
) ppm; 13C NMR (300 MHz, CDCl3): δ 192.1, 155.6, 153.1, 152.1, 144.3, 138.7, 138.0, 136.0, 122.7, 117.3, 115.1, 112.9, 98.7, 94.4, 60.9, 56.0 ppm; IR (KBr) (νmax/cm−1): 3109, 2920, 2837, 1631, 1581, 1512, 1481, 1292, 1210, 1028, 833, 765; MS (ESI): 445 [M + H]+; HRMS (ESI): calcd for C25H25N4O4 (M + H)+ 445.1870; found: 445.1863.
(Z)-3-(1H-Indol-6-ylamino)-1-(2-(3,4,5-trimethoxyphenylamino)pyridin-3-yl)prop-2-en-1-one (9f). (Z)-3-(1H-Indol-6-ylamino)-1-(2-(3,4,5-trimethoxyphenylamino)pyridin-3-yl)prop-2-en-1-one 9f was obtained according to above described method by using starting materials 1-(2-(3,4,5-trimethoxyphenylamino) pyridine-3-yl)prop-2-yn-1-one 8a (110 mg, 0.35 mmol) and 6-amino indole (47 mg, 0.35 mmol) as yellow solid. 1H NMR (300 MHz, CDCl3); δ 3.83 (s, 3H, OCH3), 3.89 (s, 6H, OCH3), 5.92 (d, 1H, J = 7.9 Hz, COCH
), 6.52 (brs, 1H, ArH), 6.66–6.73 (m, 1H, ArH), 6.91–6.98 (dd, 1H, J = 6.9 Hz, J = 1.5 Hz, ArH), 7.02–7.10 (m, 3H, ArH), 7.17 (brs, 1H, ArH), 7.46–7.56 (dd, 1H, J = 7.9 Hz, J = 12.5 Hz,
CH–NH), 7.59 (d, 1H, J = 8.5 Hz, ArH), 7.97–8.03 (dd, 1H, J = 1.3 Hz, J = 6.4 Hz, ArH), 8.28–8.33 (dd, 1H, J = 3.1 Hz, J = 1.5 Hz, ArH), 8.49 (brs, 1H, NH), 11.28 (brs, 1H, NH), 12.06 (d, 1H, J = 12.6 Hz, NH–CH
) ppm; 13C NMR (300 MHz, CDCl3): δ 191.2, 155.5, 153.1, 151.4, 145.8, 137.7, 136.4, 135.2, 125.1, 124.7, 115.8, 112.9, 110.7, 102.5, 98.9, 98.5, 93.1, 60.9, 56.0 ppm; IR (KBr) (νmax/cm−1): 3233, 2922, 1633, 1581, 1496, 1414, 1296, 1244, 1144, 1092, 1018, 845; MS (ESI): 445 [M + H]+; HRMS (ESI): calcd for C25H25N4O4 (M + H)+ 445.1870; found: 445.1866.
(Z)-3-(4-Methoxyphenylamino)-1-(2-(3,4,5-trimethoxyphenylamino)pyridin-3-yl) prop-2-en-1-one (9g). (Z)-3-(4-Methoxyphenylamino)-1-(2-(3,4,5-trimethoxyphenylamino)pyridin-3-yl)prop-2-en-1-one 9g was obtained according to above described method by using starting materials 1-(2-(3,4,5-trimethoxyphenylamino)pyridin-3-yl)prop-2-yn-1-one 8a (110 mg, 0.35 mmol) and 4-methoxyaniline (43 mg, 0.35 mmol) as yellow solid. 1H NMR (300 MHz, CDCl3); δ 3.82 (s, 3H, OCH3), 3.83 (s, 3H, OCH3), 3.90 (s, 6H, OCH3), 5.94 (d, 1H, J = 7.9 Hz, COCH
), 6.67–6.75 (dd, 1H, J = 2.6 Hz, J = 4.9 Hz, ArH), 6.92 (d, 2H, J = 8.4 Hz, ArH), 7.03–7.13 (m, 4H, ArH), 7.37–7.47 (dd, 1H, J = 7.9 Hz, J = 12.1 Hz,
CH–NH), 8.02 (d, 1H, J = 7.7 Hz, ArH), 8.31 (d, 1H, J = 7.6 Hz, ArH), 11.22 (brs, 1H, NH), 11.89 (d, 1H, J = 12.6 Hz, NH–CH
) ppm; 13C NMR (300 MHz, CDCl3): δ 192.3, 153.3, 152.3, 145.2, 144.5, 139.0, 138.3, 136.2, 122.9, 117.5, 115.4, 113.1, 98.9, 61.1, 56.2 ppm; IR (KBr) (νmax/cm−1): 3337, 2925, 1614, 1588, 1549, 1504, 1479, 1414, 1279, 1241, 1126, 1014, 897; MS (ESI): 436 [M + H]+; HRMS (ESI): calcd for C24H26N3O5 (M + H)+ 436.1867; found: 445.1852.
(Z)-3-(3,4,5-Trimethoxyphenylamino)-1-(2-(3,4,5-trimethoxyphenylamino)pyridin-3-yl)prop-2-en-1-one (9h). (Z)-3-(3,4,5-Trimethoxyphenylamino)-1-(2-(3,4,5-trimethoxyphenylamino)pyridin-3-yl)prop-2-en-1-one 9h was obtained according to above described method by using starting materials 1-(2-(3,4,5-trimethoxyphenylamino)pyridin-3-yl)prop-2-yn-1-one 8a (110 mg, 0.35 mmol) and 3,4,5-trimethoxyaniline (64 mg, 0.35 mmol) as yellow solid. 1H NMR (300 MHz, CDCl3); δ 3.84 (s, 6H, OCH3), 3.91 (s, 12H, OCH3), 5.98 (d, 1H, J = 7.8 Hz, COCH
), 6.35 (s, 2H, ArH), 6.71–6.74 (dd, 1H, J = 3.0 Hz, J = 4.9 Hz, ArH), 7.05 (s, 2H, ArH), 7.41–7.46 (dd, 1H, J = 7.8 Hz, J = 12.4 Hz,
CH–NH), 8.02–8.05 (dd, 1H, J = 1.1 Hz, J = 6.8 Hz, ArH), 8.32–8.34 (dd, 1H, J = 1.5 Hz, J = 3.3 Hz, ArH), 11.16 (brs, 1H, NH), 11.83 (d, 1H, J = 12.1 Hz, NH–CH
) ppm; 13C NMR (300 MHz, CDCl3): δ 191.8, 155.6, 154.2, 153.2, 151.8, 144.9, 137.9, 136.2, 115.5, 113.9, 112.9, 99.0, 94.4, 93.7, 61.0, 56.2 ppm; IR (KBr) (νmax/cm−1): 3419, 3072, 2941, 1642, 1504, 1448, 1417, 1384, 1236, 1124, 1009, 837; MS (ESI): 496 [M + H]+; HRMS (ESI) calcd for C26H30N3O7 [M + H]+ 496.2078, found: 496.2083.
(Z)-3-(1H-Indol-5-ylamino)-1-(2-(4-fluorophenylamino)pyridin-3-yl)prop-2-en-1-one (9i). (Z)-3-(1H-Indol-5-ylamino)-1-(2-(4-fluorophenylamino)pyridin-3-yl)prop-2-en-1-one 9i was obtained according to above described method by using starting materials 1-(2-(4-fluorophenylamino)pyridin-3-yl)prop-2-yn-1-one 8c (90 mg, 0.37 mmol) and 6-amino indole (50 mg, 0.37 mmol) as yellow solid: 1H NMR (300 MHz, CDCl3); δ 5.97 (d, 1H, J = 7.9 Hz, COCH
), 6.54 (s, 1H, ArH), 6.69–6.73 (dd, 1H, J = 4.8 Hz, J = 1.8 Hz, ArH), 6.96–7.00 (dd, 1H, J = 1.8 Hz, J = 6.7 Hz, ArH), 7.01–7.06 (m, 2H, ArH), 7.15–7.21 (m, 2H, ArH), 7.54–7.59 (dd, 1H, J = 7.9 Hz, J = 12.4 Hz,
CH–NH), 7.62 (d, 1H, J = 8.6 Hz, ArH), 7.68–7.71 (m, 2H, ArH), 8.01–8.05 (dd, 1H, J = 1.8 Hz, J = 6.1 Hz, ArH), 8.16 (brs, 1H, NH), 8.28–8.31 (dd, 1H, J = 1.8 Hz, J = 4.8 Hz, ArH), 11.27 (brs, 1H, NH), 12.08 (d, 1H, J = 12.7 Hz, NH–CH
) ppm; 13C NMR (300 MHz, CDCl3 + DMSO-d6): δ 188.9, 157.6, 153.5, 153.3, 149.4, 144.9, 136.3, 134.9, 132.7, 123.8, 123.5, 120.1, 119.6, 113.8, 113.5, 111.8, 108.3, 99.8, 97.5, 91.2 ppm; IR (KBr) (νmax/cm−1): 3064, 2967, 2838, 1630, 1581, 1481, 1415, 1284, 1247, 1168, 1023, 809; MS (ESI): 373 [M + H]+; HRMS (ESI) calcd for C22H18FN4O [M + H]+ 373.1459, found: 373.1456.
(Z)-3-(3,5-Dimethoxyphenylamino)-1-(2-(4-fluorophenylamino)pyridin-3-yl)prop-2-en-1-one (9j). (Z)-3-(3,5-Dimethoxyphenylamino)-1-(2-(4-fluorophenylamino)pyridin-3-yl)prop-2-en-1-one 9j was obtained according to above described method by using starting materials 1-(2-(4-fluorophenylamino)pyridin-3-yl)prop-2-yn-1-one 8c (90 mg, 0.37 mmol) and 3,5-dimethoxyaniline (57 mg, 0.37 mmol) as yellow solid: 1H NMR (300 MHz, CDCl3); δ 3.82 (s, 6H, OCH3), 5.98 (d, 1H, J = 8.1 Hz, COCH
), 6.19–6.33 (m, 3H, ArH), 6.67–6.76 (dd, 1H, J = 3.0 Hz, J = 4.7 Hz, ArH), 6.98–7.10 (m, 2H, ArH), 7.40–7.51 (dd, 1H, J = 7.9 Hz, J = 12.1 Hz,
CH–NH), 7.64–7.73 (m, 2H, ArH), 8.04 (d, 1H, J = 7.8 Hz, ArH), 8.30 (dd, 1H, J = 1.7 Hz, J = 4.5 Hz, ArH), 11.21 (brs, 1H, NH), 11.77 (d, 1H, J = 12.1 Hz, NH–CH
) ppm; 13C NMR (300 MHz, CDCl3): δ 192.0, 161.9, 155.6, 151.9, 144.5, 141.8, 137.9, 136.1, 129.5, 122.6, 115.3, 115.0, 113.0, 95.8, 95.1, 93.9, 55.5 ppm; IR (KBr) (νmax/cm−1): 3427, 2928, 2833, 2356, 1628, 1576, 1506, 1484, 1414, 1236, 1124, 1012, 822; MS (ESI): 394 [M + H]+; HRMS (ESI) calcd for C22H21FN3O3 [M + H]+ 394.1561, found: 394.1559.
(Z)-1-(2-(4-Fluorophenylamino)pyridin-3-yl)-3-(4-methoxyphenylamino)prop-2-en-1-one (9k). (Z)-1-(2-(4-Fluorophenylamino)pyridin-3-yl)-3-(4-methoxyphenylamino)prop-2-en-1-one 9k was obtained according to above described method by using starting materials 1-(2-(4-fluorophenylamino)pyridin-3-yl)prop-2-yn-1-one 8c (90 mg, 0.37 mmol) and 4-methoxyaniline (46 mg, 0.37 mmol) as yellow solid: 1H NMR (300 MHz, CDCl3); δ 3.82 (s, 3H, OCH3), 5.94 (d, 1H, J = 7.9 Hz, COCH
), 6.69–6.72 (dd, 1H, J = 3.0 Hz, J = 4.8 Hz, ArH), 6.92 (d, 2H, J = 9.0 Hz, ArH), 7.00–7.06 (m, 2H, ArH), 7.08 (d, 2H, J = 9.0 Hz, ArH), 7.39–7.45 (dd, 1H, J = 7.9 Hz, J = 12.4 Hz,
CH–NH), 7.65–7.71 (m, 2H, ArH), 8.02 (d, 1H, J = 4.8 Hz, ArH), 8.29 (d, 1H, J = 6.3 Hz, ArH), 11.23 (brs, 1H, NH), 11.92 (d, 1H, J = 12.7 Hz, NH–CH
) ppm; IR (KBr) (νmax/cm−1): 3241, 2922, 2851, 1634, 1573, 1498, 1439, 1324, 1240, 1074, 1017, 976, 889, 817; MS (ESI): 364 [M + H]+; HRMS (ESI) calcd for C21H19FN3O2 [M + H]+ 364.1455, found: 364.1452.
(Z)-1-(2-(4-Fluorophenylamino)pyridin-3-yl)-3-(3,4,5-trimethoxyphenylamino)prop-2-en-1-one (9l). (Z)-1-(2-(4-Fluorophenylamino)pyridin-3-yl)-3-(3,4,5-trimethoxyphenylamino)prop-2-en-1-one 9l was obtained according to above described method by using starting materials 1-(2-(4-fluorophenylamino)pyridin-3-yl)prop-2-yn-1-one 8c (90 mg, 0.37 mmol) and 3,4,5-trimethoxyaniline (69 mg, 0.37 mmol) as yellow solid: 1H NMR (300 MHz, CDCl3); δ 3.83 (s, 3H, OCH3), 3.91 (s, 6H, OCH3), 5.98 (d, 1H, J = 8.1 Hz, COCH
), 6.34 (s, 2H, ArH), 6.68–6.76 (dd, 1H, J = 3.0 Hz, J = 4.7 Hz, ArH), 7.01–7.07 (m, 2H, ArH), 7.39–7.47 (dd, 1H, J = 8.1 Hz, J = 12.4 Hz,
CH–NH), 7.65–7.71 (m, 2H, ArH), 8.01–8.06 (dd, 1H, J = 1.5 Hz, J = 6.2 Hz, ArH), 8.28–8.32 (dd, 1H, J = 1.8 Hz, J = 2.8 Hz, ArH), 11.21 (brs, 1H, NH), 11.85 (d, 1H, J = 12.6 Hz, NH–CH
) ppm; 13C NMR (300 MHz, CDCl3): δ 191.8, 155.6, 154.3, 151.8, 144.9, 137.9, 136.2, 122.6, 115.4, 115.1, 113.1, 94.4, 93.6, 61.1, 56.2 ppm; IR (KBr) (νmax/cm−1): 3417, 3001, 2923, 2851, 1635, 1578, 1488, 1415, 1285, 1233, 1125, 1011, 815; MS (ESI): 424 [M + H]+; HRMS (ESI) calcd for C23H23FN3O4 [M + H]+ 424.1667, found: 424.1657.
(Z)-1-(2-(4-Fluorophenylamino)pyridin-3-yl)-3-(4-(trifluoromethyl)phenylamino)prop-2-en-1-one (9m). (Z)-1-(2-(4-Fluorophenylamino)pyridin-3-yl)-3-(4-(trifluoromethyl)phenylamino)prop-2-en-1-one 9m was obtained according to above described method by using starting materials 1-(2-(4-fluorophenylamino)pyridin-3-yl)prop-2-yn-1-one 8c (90 mg, 0.37 mmol) and 4-trifluoromethylaniline (60 mg, 0.37 mmol) as yellow solid: 1H NMR (300 MHz, CDCl3); δ 6.09 (d, 1H, J = 7.3 Hz, COCH
), 6.74 (t, 1H, J = 6.7 Hz, ArH), 7.06 (d, 2H, J = 6.9 Hz, ArH), 7.14–7.35 (m, 2H, ArH), 7.42–7.52 (dd, 1H, J = 7.3 Hz, J = 12.3 Hz,
CH–NH), 7.57–7.81 (m, 4H, ArH), 8.06 (d, 1H, J = 6.7 Hz, ArH), 8.34 (d, 1H, J = 6.4 Hz, ArH), 11.21 (brs, 1H, NH), 11.91 (d, 1H, J = 12.3 Hz, NH–CH
) ppm; 13C NMR (300 MHz, CDCl3): δ 192.4, 160.2, 157.0, 155.7, 152.4, 143.2, 142.9, 138.2, 136.0, 127.1, 122.8, 122.7, 115.8, 115.2, 114.9, 113.0, 95.3 ppm; IR (KBr) (νmax/cm−1): 3419, 3089, 2928, 1635, 1573, 1514, 1483, 1280, 1237, 1198, 1125, 1017, 849; MS (ESI): 402 [M + H]+; HRMS (ESI) calcd for C21H15F4N3O [M + H]+ 402.1224, found: 402.1218.
(Z)-3-(1H-Indol-5-ylamino)-1-(2-(phenylamino)pyridin-3-yl)prop-2-en-1-one (9n). (Z)-3-(1H-Indol-5-ylamino)-1-(2-(phenylamino)pyridin-3-yl)prop-2-en-1-one 9n was obtained according to above described method by using starting materials 1-(2-(phenylamino)pyridin-3-yl)prop-2-yn-1-one 8d (100 mg, 0.45 mmol) and 5-amino indole (59 mg, 0.45 mmol) as yellow solid: 1H NMR (300 MHz, CDCl3); δ 5.94 (d, 1H, J = 7.8 Hz, COCH
), 6.54 (brs, 1H, ArH), 6.69–6.73 (m, 1H, ArH), 6.99–7.06 (m, 3H, ArH), 7.34 (t, 2H, J = 8.2 Hz, ArH), 7.39 (d, 1H, J = 8.6 Hz, ArH), 7.42 (brs, 1H, ArH), 7.52–7.58 (dd, 1H, J = 7.8 Hz, J = 12.7 Hz,
CH–NH), 7.77 (d, 2H, J = 8.2 Hz, ArH), 8.02–8.05 (dd, 1H, J = 1.5 Hz, J = 6.4 Hz, ArH), 8.19 (brs, 1H, NH), 8.30–8.33 (dd, 1H, J = 1.8 Hz, J = 3.0 Hz, ArH), 11.34 (brs, 1H, NH), 12.08 (d, 1H, J = 13.5 Hz, NH–CH
) ppm; IR (KBr) (νmax/cm−1): 3180, 2923, 1626, 1574, 1491, 1287, 1217, 1132, 1014, 887, 863; MS (ESI): 355 [M + H]+; HRMS (ESI) calcd for C22H19N4O [M + H]+ 355.1553, found: 355.1551.
(Z)-3-(1H-Indol-6-ylamino)-1-(2-(phenylamino)pyridin-3-yl)prop-2-en-1-one (9o). (Z)-3-(1H-Indol-6-ylamino)-1-(2-(phenylamino)pyridin-3-yl)prop-2-en-1-one 9o was obtained according to above described method by using starting materials 1-(2-(phenylamino)pyridin-3-yl)prop-2-yn-1-one 8d (100 mg, 0.45 mmol) and 6-amino indole (59 mg, 0.45 mmol) as yellow solid: 1H NMR (300 MHz, CDCl3); δ 5.97 (d, 1H, J = 8.1 Hz, COCH
), 6.54 (brs, 1H, ArH), 6.70–6.74 (m, 1H, ArH), 6.97–7.00 (dd, 1H, J = 1.8 Hz, J = 6.5 Hz, ArH), 7.03 (t, 1H, J = 7.5 Hz, ArH), 7.12 (brs, 1H, ArH), 7.15–7.23 (m, 2H, ArH), 7.34 (t, 2H, J = 7.5 Hz, ArH), 7.53–7.58 (dd, 1H, J = 7.9 Hz, J = 12.4 Hz,
CH–NH), 7.62 (d, 1H, J = 8.3 Hz, ArH), 7.77 (d, 2H, J = 7.8 Hz, ArH), 8.02–8.05 (dd, 1H, J = 1.5 Hz, J = 6.5 Hz, ArH), 8.17 (brs, 1H, NH), 8.30–8.33 (dd, 1H, J = 1.8 Hz, J = 3.3 Hz, ArH), 11.32 (brs, 1H, NH), 12.09 (d, 1H, J = 12.4 Hz, NH–CH
) ppm; 13C NMR (300 MHz, CDCl3): δ 189.2, 149.6, 144.9, 138.7, 136.6, 132.8, 127.1, 123.7, 120.3, 119.7, 118.4, 112.0, 108.4, 99.9, 91.3 ppm; IR (KBr) (νmax/cm−1): 3194, 2922, 1619, 1575, 1511, 1468, 1279, 1218, 1135, 1018, 866, 814; MS (ESI): 355 [M + H]+, HRMS (ESI) calcd for C22H19N4O [M + H]+ 355.1553, found: 355.1554.
(Z)-1-(2-(Phenylamino)pyridin-3-yl)-3-(3,4,5-trimethoxyphenylamino)prop-2-en-1-one (9p). (Z)-1-(2-(Phenylamino)pyridin-3-yl)-3-(3,4,5-trimethoxyphenylamino)prop-2-en-1-one 9p was obtained according to above described method by using starting materials 1-(2-(phenylamino)pyridin-3-yl)prop-2-yn-1-one 8d (100 mg, 0.45 mmol) and 3,4,5-trimethoxyaniline (82 mg, 0.45 mmol) as yellow solid: 1H NMR (300 MHz, CDCl3); δ 3.40 (s, 9H, OCH3), 5.93 (d, 1H, J = 7.9 Hz, COCH
), 6.51 (s, 2H, ArH), 6.70–6.76 (dd, 1H, J = 2.8 Hz, J = 4.9 Hz, ArH), 7.04 (t, 2H, J = 8.1 Hz, ArH), 7.30–7.45 (m, 2H, ArH,
CH–NH), 7.72 (d, 2H, J = 8.1 Hz, ArH), 8.02–8.06 (dd, 1H, J = 1.3 Hz, J = 6.4 Hz, ArH), 8.24–829 (dd, 1H, J = 1.3 Hz, J = 3.2 Hz, ArH), 11.28 (brs, 1H, NH), 12.09 (d, 1H, J = 13.7 Hz, NH–CH
) ppm; 13C NMR (300 MHz, CDCl3): δ 191.2, 155.4, 153.1, 151.3, 145.8, 137.8, 136.3, 135.2, 125.1, 124.7, 121.7, 115.8, 113.0, 110.7, 102.6, 98.5, 93.1, 60.9, 56.0 ppm; IR (KBr) (νmax/cm−1): 3429, 2937, 1633, 1577, 1506, 1485, 1415, 1231, 1123, 1013, 918, 768; MS (ESI): 406 [M + H]+. HRMS (ESI) calcd for C23H24N3O4 [M + H]+ 406.1761, found: 406.1756.
Biology
Cell cultures, maintenance and antiproliferative evaluation. All cell lines used in this study were purchased from the American Type Culture Collection (ATCC, United States). A-549, Hela, MCF-7 and HCT-116 were grown in Dulbecco's modified Eagle's medium (containing 10% FBS in a humidified atmosphere of 5% CO2 at 37 °C). A-549 cells were cultured in Eagle's minimal essential medium (MEM) containing non-essential amino acids, 1 mM sodium pyruvate, 10 mg mL−1 bovine insulin, and 10% FBS. Cells were trypsinized when sub-confluent from T25 flasks/60 mm dishes and seeded in 96-well plates. The synthesized test compounds were evaluated for their in vitro antiproliferative in four different human cancer cell lines. A protocol of 48 h continuous drug exposure was used, and a MTT cell proliferation assay was used to estimate cell viability or growth. The cell lines were grown in their respective media containing 10% fetal bovine serum and were seeded into 96-well microtiter plates in 200 μL aliquots at plating densities depending on the doubling time of individual cell lines. The microtiter plates were incubated at 37 °C, 5% CO2, 95% air, and 100% relative humidity for 24 h prior to addition of experimental drugs. Aliquots of 2 μL of the test compounds were added to the wells already containing 198 μL of cells, resulting in the required final drug concentrations. For each compound, four concentrations (0.1, 1, 2, 10 and 50 μM) were evaluated, and each was done in triplicate wells. Plates were incubated further for 48 h, and the assay was terminated by the addition of 10 μL of 5% MTT and incubated for 60 min at 37 °C. Later, the plates were air-dried. Bound stain was subsequently eluted with 100 μL of DMSO, and the absorbance was read on multimode plate reader (Tecan M200) at a wavelength of 560 nm. Percent growth was calculated on a plate by plate basis for test wells relative to control wells. The above determinations were repeated thrice. The growth inhibitory effects of the compounds were analyzed by generating dose response curves as a plot of the percentage surviving cells versus compound concentration. The sensitivity of the cancer cells to the test compound was expressed in terms of IC50, a value defined as the concentration of compound that produced 50% reduction as compared to the control absorbance. IC50 values are indicated as means ± SD of three independent experiments.
Cell cycle analysis. A-549 cells in 60 mm dishes were incubated for 24 h in the presence or absence of test compounds 9a, 9g and 9j at 3 μM concentrations. Cells were harvested with trypsin–EDTA, fixed with ice-cold 70% ethanol at 4 °C for 30 min, ethanol was removed by centrifugation and cells were stained with 1 mL of DNA staining solution [0.2 mg of propidium iodide (PI), and 2 mg RNase A] for 30 min as described earlier. The DNA contents of 20
000 events were measured by flow cytometer (BD FACS Canto II). Histograms were analyzed using FCS express 4 plus.
Tubulin polymerization assay. An in vitro assay for monitoring the time-dependent polymerization of tubulin to microtubules was performed employing a fluorescence-based tubulin polymerization assay kit (BK011, Cytoskeleton, Inc.) according to the manufacturer's protocol. The reaction mixture in a final volume of 10 μL in PEM buffer (80 mM PIPES, 0.5 mM EGTA, 2 mM MgCl2, pH 6.9) in 384 well plates contained 2 mg mL−1 bovine brain tubulin, 10 μM fluorescent reporter, 1 mM GTP in the presence or absence of test compounds at 37 °C. Tubulin polymerization was followed by monitoring the fluorescence enhancement due to the incorporation of a fluorescence reporter into microtubules as polymerization proceeds. Fluorescence emission at 420 nm (excitation wavelength is 360 nm) was measured for 1 h at 1 min intervals in a multimode plate reader (Tecan M200). To determine the IC50 values of the compounds against tubulin polymerization, the compounds were pre-incubated with tubulin at varying concentrations (0.1, 1, 2, 5 and 10 μM). Assays were performed under similar conditions as employed for polymerization assays as described above.
Immunohistochemistry of tubulin and analysis of nuclear morphology. A549 cells were seeded on glass cover slip, incubated for 24 h in the presence or absence of test compounds 9a, 9g and 9j at concentrations of 3 μM. Cells grown on cover slips were fixed in 3.5% formaldehyde in phosphate-buffered saline (PBS) pH 7.4 for 10 minutes at room temperature. Cells were permeabilized for 6 minutes in PBS containing 0.5% Triton X-100 (Sigma) and 0.05% Tween-20 (Sigma). The permeabilized cells were blocked with 2% BSA (Sigma) in PBS for 1 h. Later, the cells were incubated with primary antibody for tubulin from (Sigma) at (1
:
200) diluted in blocking solution for 4 h at room temperature. Subsequently the antibodies were removed and the cells were washed thrice with PBS. Cells were then incubated with FITC labeled anti-mouse secondary antibody (1
:
500) for 1 h at room temperature. Cells were washed thrice with PBS and mounted in medium containing DAPI. Images were captured using the Olympus confocal microscope and analyzed with Provision software.
Morphological analysis for apoptosis with Hoechst staining. A549 cells were seeded at a density of 10
000 cells over 18 mm cover slips and incubated for 24 h. Then, the medium was replaced, and cells were treated with compounds 9a, 9g and 9j at 2 μM for 24 h. Cells treated with vehicle (0.001% DMSO) were included as controls for all experiments. An overnight treatment, Hoechst 33342 (Sigma-Aldrich) were added to medium at a concentration of 0.5 mg mL−1 containing 4% paraformaldehyde. After incubation for 30 min at 37 °C, cells from each dish were captured from randomly selected fields under fluorescent microscope (Leica, Germany) to qualitatively determine the proportion of viable and apoptotic cells based on their relative fluorescence and nuclear fragmentation.
Caspase-3 activity assay. AFC conjugated Ac-DEVD substrate was used to determine the caspase-3 activity of the potent compounds 9a, 9g and 9j at 2 μM. A549 cells were seeded in six well plates with the confluence of per (2.5 × 10−6) well and are treated with the compounds at 2 μM concentration. The cells were washed with PBS after incubation for 48 h and then scraped in to the PBS and centrifuged at 2000 rpm for 10 min at 4 °C. Pellet was resuspended in 80 mL of lysis buffer. Then the pellet was passed through insulin syringe followed by incubation of suspension on ice for 20–30 min centrifuged the lysate at 13
200 rpm for 20 min at 4 °C and transferred the supernatant to fresh tubes. In a 96 well black polystyrene plate, 50 mL cell lysate, 50 mL of 2× assay buffer and 2 mL of caspase-3 substrate were taken. The reaction was allowed to take place for 1 h. The fluorescence generated by the release of the fluorogenic group AFC on cleavage by caspase-3 was measured by excitation at 400 nm and emission at 505 nm for every 5 min over 1 h. Protein was estimated by Bradford's method and normalized consequently.
Competitive tubulin binding assay. The various concentrations (0 μM, 5 μM, 10 μM and 15 μM) of conjugates 9a, 9g and 9j were coincubated with 3 μM colchicines in 30 mM Tris buffer containing 3 μM tubulin at 37 °C for 60 min. The standard nocodazole (I) was employed as a positive control whereas peclitaxel was used as the negative control. After incubation, the fluorescence of tubulin–colchicine complex was measured by using Tecan multimode reader at excitation wavelength of 380 nm and emission wavelength of 435 nm; whereas 30 mM Tris buffer was used as a blank. The raw fluorescence values were normalized first by subtracting the fluorescence of the buffer and then setting the fluorescence of 3 μM tubulin with 3 μM colchicine to 100%. Values represented were ±SD of at least three independent experiments.
Docking. For the purpose of molecular docking studies, recently published X-ray crystal structure of α,β-tubulin heterodimer (PDB ID: 3UT5, resolution: 2.73 Å)35 was selected. This PDB ID is particularly chosen since it is available at a higher resolution without any missing structural components and overall good B-factor as compared to other tubulin structures. Both the chains were considered for molecular docking studies because the colchicine binds at the interface of αβ-tubulin subunits. Protein preparation was done using Maestro. Hydrogen atoms were added during protein preparation wizard. Receptor Grid Generation Panel within Glide suite was used to set up receptor grid for the prepared structures. The grid was defined by 10 Å around the centroid of the bound ligand. Then, this step is followed by restrained minimization using the OPLS 2005 force field to RMSD of 0.3 Å. Three-dimensional structures of these compounds were then prepared using LigPrep module of maestro implementing OPLS_2005 force field and ionic states for the ligands at pH values of 7.0 ± 2.0 were generated. Docking was performed using Glide 5.8 (Grid-based Ligand Docking with Energetics), with the standard precision (SP) mode to estimate protein–ligand binding affinities and static intermolecular interactions.36–38 Initially, to check the reliability of docking protocol, the bound ligand (colchicine) were redocked in the active sites of tubulin and RMSD value (0.23 Å) of the docked pose was compared to the X-ray crystallographic conformation of the colchicine. After the validation of docking protocol 9a and 9g were docked into the active site of tubulin. A maximum of 10 docking poses per ligand were generated in each case and analyzed further for the binding mode and intermolecular interactions.
Acknowledgements
V. S. R., A. B. S., G. B. K., M. K. R., I. B. S., S. V. and P. R. A. acknowledge CSIR-UGC, New Delhi and for the award of senior research fellowship. We also acknowledge CSIR for financial support under the 12th Five Year plan project “Affordable Cancer Therapeutics (ACT)” (CSC0301). The authors thank Prof. P. V. Bharatam, NIPER Mohali for discussion on molecular docking.
Notes and references
-
(a) L. Amos, Org. Biomol. Chem., 2004, 2, 2153 RSC;
(b) C. E. Walczak, Curr. Opin. Cell Biol., 2000, 12, 52 CrossRef CAS PubMed.
- P. K. Sarger, M. Dobles, R. Tournebize and A. A. Hyman, Curr. Opin. Cell Biol., 1997, 9, 807 CrossRef.
-
(a) S. Honore, E. Pasquier and D. Braguer, Cell. Mol. Life Sci., 2005, 62, 3039 CrossRef CAS PubMed;
(b) L. A. Amos, Org. Biomol. Chem., 2004, 2, 2153 RSC;
(c) K. H. Downing and E. Nogales, Curr. Opin. Struct. Biol., 1998, 8, 785 CrossRef CAS PubMed.
- M. A. Jordan and L. Wilson, Nat. Rev. Cancer, 2004, 4, 253 CrossRef CAS PubMed.
-
(a) M. N. Islam and M. N. Iskander, Mini-Rev. Med. Chem., 2004, 4, 1077 CrossRef CAS PubMed;
(b) J. A. Hadfield, S. Ducki, N. Hirst and A. T. McGown, Prog. Cell Cycle Res., 2003, 5, 309 Search PubMed;
(c) E. Hamel, Med. Res. Rev., 1996, 16, 207 CrossRef CAS PubMed.
- D. Hanahan and R. A. Weinberg, Cell, 2000, 100, 57 CrossRef CAS PubMed.
- R. O. Carlson, Expert Opin. Invest. Drugs, 2008, 17, 707 CrossRef CAS PubMed.
-
(a) R. Bharadwaj and H. Yu, Oncogene, 2004, 23, 2016 CrossRef CAS PubMed;
(b) D. A. Brito, Z. Yang and C. L. Rieder, J. Cell Biol., 2008, 182, 623 CrossRef CAS PubMed.
- F. Gueritte and J. Fahy, in Anticancer Agents from Natural Products, ed. G. M. Cragg, D. G. I. Kingston and D. J. Newman, CRC Press, Boca Raton, FL, 2005, pp. 123–135 Search PubMed.
- M. Rosner, L. H. G. Capraro, A. E. Jacobson, L. Atwell and A. Brossi, J. Med. Chem., 1981, 24, 257 CrossRef CAS PubMed.
- L. Wang, K. W. Woods, Q. Li, K. J. Barr, R. W. McCroskey, S. M. Hannick, L. Gherke, R. B. Credo, Y. H. Hui, K. Marsh, R. Warner, J. Y. Lee, N. Z. Mozng, D. Frost, S. H. Rosenberg and H. L. Sham, J. Med. Chem., 2002, 45, 1697 CrossRef CAS PubMed.
- H. Yoshino, N. Ueda, J. Niijima, H. Sugumi, Y. Kotake, N. Koyanagi, K. Yoshimatsu, M. Asada, T. Watanabe, T. Nagasu, K. Tsukahara, A. Iijima and K. Kitoh, J. Med. Chem., 1992, 35, 2496 CrossRef CAS PubMed.
- G. R. Pettit, S. B. Singh, E. Hamel, C. M. Lin, D. S. Alberts and D. Garcia-Kendall, Experentia, 1989, 45, 209 CrossRef CAS PubMed.
- C. M. Lin, H. H. Ho, G. R. Pettit and E. Hamel, Biochemistry, 1989, 28, 6984 CrossRef CAS PubMed.
- A. T. McGown and B. W. Fox, Cancer Chemother. Pharmacol., 1990, 26, 79 CrossRef CAS PubMed.
-
(a) L. Vincent, P. Kermani, L. M. Young, J. Cheng, F. Zhang, K. Shido, G. Lam, H. Bompais-Vincent, Z. Zhu, D. J. Hicklin, P. Bohlen, D. J. Chaplin, C. May and S. J. Rafii, Clin. Invest., 2005, 115, 2992 CAS;
(b) M. M. Cooney, J. Ortiz, R. M. Bukowski and S. C. Remick, Curr. Oncol. Rep., 2005, 7, 90 CrossRef CAS PubMed;
(c) S. L. Young and D. J. Chaplin, Expert Opin. Invest. Drugs, 2004, 13, 1171 CrossRef CAS PubMed;
(d) D. J. Chaplin and S. A. Hill, Int. J. Radiat. Oncol., Biol., Phys., 2002, 54, 1491 CrossRef CAS.
- G. R. Pettit, J. W. Lippert, M. R. Boyd, P. Verdier-Pinard and E. Hamel, Anti-Cancer Drug Des., 2000, 15, 361 CAS.
- G. R. Pettit and M. R. Rhodes, Anti-Cancer Drug Des., 1998, 13, 183 CAS.
- A. Jordan, J. A. Hadfield, N. J. Lawrence and A. T. McGown, Med. Res. Rev., 1998, 18, 259 CrossRef CAS PubMed.
- K. Yoshimatsu, A. Yamaguchi, H. Yoshino, N. Koyanagi and K. Kitoh, Cancer Res., 1997, 57, 3208 CAS.
- N. Koyanagi, T. Nagasu, F. Fujita, T. Watanabe, K. Tsukahara, Y. Funahashi, M. Fujita, T. Taguchi, H. Yoshino and K. Kitoh, Cancer Res., 1994, 54, 1702 CAS.
- A. M. Mauer, E. E. Cohen, P. C. Ma, M. F. Kozloff, L. Schwartzberg, A. I. Coates, J. Qian, A. E. Hagey and G. B. Gorden, J. Thorac. Oncol., 2008, 3, 631 CrossRef PubMed.
-
(a) A. Kamal, M. K. Reddy, T. B. Shaik, Rajender, Y. V. V. Srikanth, V. S. Reddy, G. B. Kumar and S. V. Kalivendi, Eur. J. Med. Chem., 2012, 17, 9 CrossRef PubMed;
(b) A. Kamal, G. B. Kumar, S. Polepalli, A. B. Shaik, V. S. Reddy, M. K. Reddy, C. R. Reddy, R. Mahesh, J. S. Kapure and N. Jain, ChemMedChem, 2014, 9, 2565 CrossRef CAS PubMed;
(c) A. Kamal, A. B. Shaik, S. Polepalli, V. S. Reddy, G. B. Kumar, S. Gupta, K. V. R. Krishna, A. Nagabhushana, R. K. Mishra and N. Jain, Org. Biomol. Chem., 2014, 12, 7993 RSC;
(d) A. Kamal, V. S. Reddy, S. Karnewar, S. S. Chourasiya, A. B. Shaik, G. B. Kumar, C. Kishor, M. K. Reddy, M. P. N. Rao, A. Nagabhushana, K. V. S. Ramakrishna, A. Addlagatta and S. Kotamraju, ChemMedChem, 2013, 8, 2015 CrossRef CAS PubMed;
(e) A. Kamal, V. S. Reddy, K. Santosh, G. B. Kumar, A. B. Shaik, R. Mahesh, S. S. Chourasiya, I. B. Sayeed and S. Kotamraju, Med. Chem. Commun., 2014, 5, 1718 RSC.
- Y. Niher, Y. Suga, Y. Morinaga, Y. Akiyama and T. Tsuji, Bioorg. Med. Chem. Lett., 1998, 8, 3153 CrossRef.
- J. P. Liou, N. Mahindroo, C. W. Chang, F. M. Guo, S. W. H. Lee, U. K. Tan, T. K. Yeh, C. C. Kuo, Y. W. Chang, P. H. Lu, Y. S. Tung, K. T. Lin, J. Y. Chang and H. P. Hsieh, ChemMedChem, 2006, 1, 1106 CrossRef CAS PubMed.
- B. G. Szczepankiewicz, G. Liu, H. S. Jae, A. S. Tasker, I. W. Gunawardana, T. W. von Geldern, S. L. Gwaltney II, J. R. Wu-Wong, L. Gehrke, W. J. Chiou, R. B. Credo, J. D. Alder, M. A. Nukkala, N. A. Zielinski, K. Jarvis, K. W. Mollison, D. J. Frost, J. L. Bauch, Y. H. Hui, A. K. Claiborne, Q. Li and S. H. Rosenberg, J. Med. Chem., 2001, 44, 4416 CrossRef CAS PubMed.
- A. Kamal, M. N. A. Khan, K. S. Reddy and K. Rohini, Bioorg. Med. Chem., 2007, 15, 1004 CrossRef CAS PubMed.
- A. Kamal, V. S. Reddy, S. Karnewar, S. S. Chourasiya, A. B. Shaik, G. B. Kumar, C. Kishor, M. K. Reddy, M. P. N. Rao, A. Nagabhushana, V. S. R. Kallaganti, A. Addlagatta and K. Srigiridhar, ChemMedChem, 2013, 8, 2015 CrossRef CAS PubMed.
- C. Kanthou, O. Greco, A. Stratford, I. Cook, R. Knight, O. Benzakour and G. Tozer, Am. J. Pathol., 2004, 165, 1401 CrossRef CAS PubMed.
- P. M. Checchi, J. H. Nettles, J. Zhou, J. P. Snyder and H. C. Joshi, Trends Pharmacol. Sci., 2003, 24, 361 CrossRef CAS PubMed.
- S. Honore, E. Pasquier and D. Braguer, Cell. Mol. Life Sci., 2005, 62, 3039 CrossRef CAS PubMed.
- Y. Zhang, X. Wang, W. Fang, X. Cai, F. Chu, X. Liao and J. Lu, Bioinorg. Chem. Appl., 2013, 43, 7134 Search PubMed.
- H. M. Alkahtani, A. Y. Abbas and S. Wang, Bioorg. Med. Chem. Lett., 2012, 22, 1317 CrossRef CAS PubMed.
- L. Risinger, C. D. Westbrook, A. Encinas, M. Mülbaier, C. M. Schultes, S. Wawro, J. D. Lewis, B. Janssen, F. J. Giles and S. L. Mooberry, J. Pharmacol. Exp. Ther., 2011, 336, 652 CrossRef PubMed.
- F. M. Ranaivoson, B. Gigant, S. Berritt, M. Joullie and M. Knossow, Acta Crystallogr., Sect. D: Biol. Crystallogr., 2012, 68, 927–934 CAS.
- R. A. Friesner, R. B. Murphy, M. P. Repasky, L. L. Frye, J. R. Greenwood, T. A. Halgren, P. C. Sanschagrin and D. T. Mainz, J. Med. Chem., 2006, 49, 6177–6196 CrossRef CAS PubMed.
- R. A. Friesner, J. L. Banks, R. B. Murphy, T. A. Halgren, J. J. Klicic, D. T. Mainz, M. P. Repasky, E. H. Knoll, M. Shelley, J. K. Perry, D. E. Shaw, P. Francis and P. S. Shenkin, J. Med. Chem., 2004, 47, 1739–1749 CrossRef CAS PubMed.
- N. Sanghai, V. Jain, R. Preet, S. Kandekar, S. Das, N. Trivedi, P. Mohapatra, G. Priyadarshani, M. Kashyap, D. Das, S. R. Satapathy, S. Siddharth, S. K. Guchhait, C. N. Kundu and P. V. Bharatam, Med. Chem. Commun., 2014, 5, 766–782 RSC.
Footnote |
† Electronic supplementary information (ESI) available: 1H NMR, 13C NMR and HRMS spectra of final compounds (9a–9p). See DOI: 10.1039/c5ra19468g |
|
This journal is © The Royal Society of Chemistry 2015 |