DOI:
10.1039/C5RA17907F
(Paper)
RSC Adv., 2015,
5, 97102-97112
Design, synthesis and biological evaluation of coumarin coupled nitroimidazoles as potential imaging agents
Received
3rd September 2015
, Accepted 12th October 2015
First published on 12th October 2015
Abstract
Solid tumors contain regions of hypoxia in comparison to normal tissues. The nitroimidazoles have shown great promise for targeting different types of cancers. The present work involves the design, syntheses, and in vitro and in vivo investigations of hypoxia targeted nitroimidazole radioconjugates (2NIHC and 4NIHC). Flow cytometry analysis showed that the normoxic–hypoxic mean fluorescence of 2NIHC is 10 fold greater than that of 4NIHC and much higher than that of the well-known pimonidazole hypoxia marker. Furthermore, molecular modeling studies of these ligands with CK2α revealed that 2NIHC is a more potential CK2α inhibitor due to π–π interactions and H-bonding with Val116, Glu114, Asp175, Asn161 and His160 in the active pockets of the target. Radio labeling yields for both the complexes with 99mTc were >98%. Biodistribution in EAT tumor bearing mice demonstrated rapid clearance of 99mTc-2NIHC and high T/M ratio (3.57% ID/g) as compared to 99mTc-4NIHC (2.05% ID/g) at 4 h. IC50 values of both ligands were estimated by MTS in different cell lines in addition to tumor regression studies.
1. Introduction
Adaptation of tumor cells to hypoxia is a critical driving force in tumor progression and metastasis.1 Such hypoxic zones have been postulated to have a reduced response to radiotherapy due to a decrease in oxygen free radicals that are required to produce enough DNA damage to give cell death.2 In addition, cells of these regions are considered to be chemo-therapy resistant due to limited delivery of drugs via circulation.3,4 Therefore, identification of hypoxic zones becomes crucial for effective treatment of tumors.
A decade before assessment of hypoxia in tumor was difficult, as it involves the use of polarographic electrodes.5 It was invasive, expensive and limited for usage in few tumors. It led to the exploration of noninvasive method of diagnosing tumor hypoxia. In this regard, several probes have been developed to image hypoxia using PET and SPECT in nuclear medicine. Appropriately labeled nitroimidazoles (NI) are particularly attractive for imaging tumor hypoxia as nitro group undergoes one electron reduction by intracellular reductases, selectively in hypoxic conditions to generate reactive intermediates, which bind to cellular components and get trapped inside the cell.6 The PET radiotracer includes, [18F] EF5,7 [18F] FETNIM,8 [18F] FAZA9 but the use of above markers get limited due to the choice of radioisotope and it has led to great interest in the development of 99mTc labeled compounds like BMS1813-21,10 BRV59-21
11 and HL91.12–14
A constraint in the development of radiopharmaceuticals is the inability to image at cellular and sub cellular level. Dual modality imaging is emerging as a method for improved visual quality as well as increase the qualitative accuracy of radio imaging for diagnosis of a variety of diseases. For mechanistic studies of the uptake and bio-distribution in cells, the coupling of radio imaging with optical imaging is desirable. Chromophores are responsible for cell auto fluorescence predominantly in the blue region of the spectrum allowing the visualization at the cellular level. One of the used example is the nitro group which quenches the fluorescence of the ring system. Nitroimidazoles with fluorescent groups on side chain were well reported as hypoxic cell markers.15,16 We have selected substituted coumarins as fluorescent label for imaging hypoxic cells because substituted coumarins with electron donating group (–OH, –OCH3) at 7th position resulted in the increase of fluorescence.17,18
Protein kinase CK2 often presents as a heterotetramer composed of two catalytic α subunits and two regulatory β subunits. Overexpressed CK2α is a key oncogenic force for tumorigenesis, and pharmacological inhibitors of this therapeutic target have been considered as promising drug candidates.19,20 Besides that many hydroxycoumarins display IC50 (and Ki) values in micro to nano level.17,18 Therefore here we report synthesis and biological evaluation of 7-hydroxycoumarin coupled nitroimidazole derivatives i.e. 2NIHC and 4NIHC.
2. Experimental section
All the chemicals were purchased from Sigma-Aldrich and Merck. All solvents used were of analytical grades. TLC was run on silica gel coated aluminium sheets (Silica gel 60 F254, Merck, Germany) and visualized in UV light 254 nm. Radiocomplexation and radiochemical purity were checked by thin layer chromatography.
2.1 Instrumentation
1H and 13CNMR spectra were recorded on a Bruker Avance II 400 MHz system (Ultra shield). Mass spectra (ESI-MS in positive and negative ion mode) were performed on Agilent 6310 system ion trap. Radioimaging was performed using HAWKEYE gamma camera and single well type capintec γ scintillation counter respectively. Molecular modeling investigations were carried out using an advanced molecular docking program GLIDE, version 9.3 (Schrodinger, Inc, USA).
2.2 Cell culture
Cells were maintained in minimal essential medium supplemented with 10% fetal bovine serum, 4 mM glutamine, 1 mM sodium pyruvate, and 50 μg ml−1 gentamicin. Cells were grown in a humidified incubator at 37 °C/5% CO2 and removed from flasks for passage or for transfer to 12-well assay plates by incubating them with 0.25% trypsin/EDTA.
2.3 Evaluation of in vitro specificity
Completion ability of the CA-IX inhibitor with 99mTc-2NIHC for binding to hypoxic HeLa cells was examined. HeLa cells were plated in 12-well plates at approximately 2.5 × 105 cells per well and allowed to adhere to the plate for 24 h. Cells were then incubated under hypoxic conditions (0.1% O2/5% CO2 at 37 °C) for an additional 24 h. The cells were then removed from hypoxia and incubated for 1 h in HBS Solution along with 0.5% BSA and 5 nM 999mTc-2NIHC in the presence of 10 μM actinomycin D. The assay media was then removed and the cells were washed 2× with cold HBS/0.5% BSA, collected by adding 0.25 mL of 1% SDS, and transferred to a 1.5 mL tube for counting the amount of radioactivity by gamma counter.
2.3.1 Flow cytometric studies. Cells of human lung carcinoma A549 × 106 cells in 3 ml medium per petridish were cultured similarly as discussed above and maintained under normoxic condition (5% CO2, 95% air) at 37 °C in a humidified CO2 incubator. Cells were routinely subcultured twice a week using 0.05% of trypsin (Sigma, USA) in 0.02% EDTA.
2.3.2 Animal model. All animal experiments were done in accordance with the guidelines of Institutional Animal Ethics Committee. EAT tumor bearing BALB/c mice were used for tumor regression study, biodistribution and gamma imaging. Mice were housed on normal diet of sterile food pellets and water at a controlled temperature of 22 ± 2 °C.BALB/c mice (aged 2 months) weighing between 20 and 25 g were selected for the study. EAT was maintained in the peritoneum of the mice in the ascites form by serial weekly passage. Exponentially growing EAT (Ehlrisch ascites tumor) cells were harvested, washed, and resuspended in PBS and 107 cells per mice were injected intramuscularly in the thigh of the left hind leg of the mice. Animal models were ready for hypoxic studies when tumor grew to a volume of 500–800 cm3 in 12–14 days.
2.4 Synthesis
2.4.1 Synthesis of tert-butyl (2-amino-ethyl)-carbamate (2). To a stirred solution of ethylenediamine 1 (3.0 ml, 45 mmol) in ethanol at 0 °C triethylamine (1.2 ml, 9.1 mmol) was added. The solution of boc-anhydride (2.0 g, 9.1 mmol) dissolved in 10 ml of ethanol was added dropwise after the reaction mixture was cooled down for 20 minutes. The reaction mixture was allowed to stir for 12 h and then solvent was evaporated to dryness under reduced pressure. The reaction mixture was extracted with chloroform/water and combined organic extracts were dried over anhydrous sodium sulphate, evaporated under reduced pressure to obtain the transparent oily product (5.32 g, 79%). 1H NMR (400 MHz, CDCl3) 1H: −1.45 (s, 9H, CH3), 2.81 (t, 2H, J = 6.0 and 5.6 Hz, CH2), 3.19 (t, 2H, J = 5.6 and 5.2 Hz, CH2). 13C NMR (100 MHZ, CDCl3) 13C: −28.37 (CH3), 41.81, 43.29 (CH2), 79.40, 156.2 (C
O). MS (ESI): found 161.2 [M + H]+, 105.5 [M − (C(CH3)3)]+.
2.4.2 Synthesis of tert-butyl{2-[7-hydroxy-2-oxo-2H-chromen-4-yl]-acetamido}-ethyl-carbamate (4). A solution of 7-hydroxy coumarinyl-4-acetic acid 3 (0.9 g, 4.5 mmol) and HOBT (0.61 g, 4.5 mmol) in dry DMF (25 ml) was stirred at 0 °C and a solution of DCC (1.0 g, 4.9 mmol) in dry DMF (5 ml) was added. Compound 2 (0.73 g, 4.5 mmol) was added at 0 °C to the reaction mixture and stirred for 2 h and then reaction mixture was stirred at rt for 24 h. The DCU (dicyclohexylurea) was filtered off and solvent was concentrated under reduced pressure. The concentrate was dissolved in 1 ml of MeOH followed by addition of 30 ml of cold chloroform dropwise. The compound gets precipitated out as pale white solid and purified by silica gel chromatography (20% methanol in chloroform) with a yield of 1.35 g, 70%. 1H NMR (400 MHz, DMSO) 1H: −1.39 (s, 9H, CH3), 2.83 (t, 2H, J = 6.0 and 6.0 Hz, CH2), 3.07 (t, 2H, J = 6.4 and 5.6 Hz, CH2). 3.76 (s, 2H, CH2), 6.18 (s, 1H, CH), 6.72 (d, 1H, J = 2.4 Hz, CH), 6.83 (dd, 1H, J1 = 6.8 Hz, J2 = 2.0 Hz, CH), 7.60 (d, 1H, J = 8.4 Hz, CH). 13C NMR (100 MHz, DMSO) 13C: −27.34, 38.77, 39.44, 78.81, 102.24, 111.95, 112.28, 113.30, 127.20, 151.56, 155.45, 156.10, 160.73, 161.62, 168.21, 168.30. MS (ESI): found 362.1 [M − H]−.
2.4.3 Synthesis of N-(2-amino-ethyl)-2-(7-hydroxy-2-oxo-2H-chromen-4-yl)-acetamide (5). The tert-butyl group of compound 4 (1.0 g, 2.7 mmol) was deprotected by the solution of trifluoroacetic acid (5 ml) in anhydrous dichloromethane at 0 °C and followed by stirring at room temperature for 6 h. The solvent was evaporated to dryness under reduced pressure to obtain the crude product. The product was washed with methanol (3 × 20 ml). Addition of cold diethyl ether led to the precipitation of pure product as white solid (0.66 g, 69% yield). 1H NMR (400 MHz, D2O) 1H: −3.01 (t, 2H, J = 4.0 and 8.0 Hz, CH2), 3.40 (t, 2H, J = 4.0 and 8.0 Hz, CH2), 3.72 (s, 2H, CH2), 6.11 (s, 1H), 6.64 (s, H, CH), 6.74 (d, 1H, J = 8.0 Hz, CH), 7.40 (d, 1H, J = 8.0 Hz, CH). 13C NMR (100 MHz, D2O) 13C: −37.04, 38.47, 38.88, 102.80, 112.02, 112.05, 113.58, 126.22, 151.34, 154.51, 160.07, 164.03, 172.04. MS (ESI): found 261.1 [M − H]−; 175.1 [M − CH2CONH(CH2)2NH2]−.
2.4.4 Synthesis of 1-(4-bromo-butyl)-4(or 2)-nitro-1H-imidazole (8, 9). A solution of 4-nitroimidazole and 2-nitroimidazole (1.0 g, 8.9 mmol) and K2CO3 (9.8 g, 71.3 mmol) in MeCN (50 ml) was stirred at 70 °C for 0.5 h followed by the addition of 1,4-dibromobutane (4.2 ml, 35.7 mmol). After completion, reaction mixture was filtered, evaporated under reduced pressure and purified by silica gel chromatography (10% methanol in chloroform). The product was crystallized as pale yellow crystal of 4-nitroimidazole derivative (1.22 g, 76% yield) and yellow powder of 2-nitroimidazole derivative (1.31 g, 79% yield) in hexane.
4-Nitroimidazole derivative. 1H NMR (400 MHz, D2O) 1H: 1.83–1.96 (m, 2H), 1.99–2.10 (m, 2H), 3.42 (t, J = 6.0 and 6.0 Hz, 2H), 4.09 (t, 2H, J = 7.2 and 7.2 Hz). 7.53 (s, 1H), 7.85 (s, 1H). 13C NMR (100 MHz, D2O) ppm: 29.15, 32.29, 47.60, 119.35, 136.06, 148.11. MS (ESI): found 248.0 and 250.0 [M + H]+, 270.0 and 272.2 [M + Na]+ with isotopic pattern of Br.
2-Nitroimidazole derivative. 1H NMR (400 MHz, D2O) 1H: 1.89–1.96 (m, 2H), 2.01–2.14 (m, 2H), 3.41 (t, J = 6.4 and 6.4 Hz, 2H), 4.45 (t, 2H, J = 7.2 and 7.2 Hz). 7.13 (d, 2H, J = 6.0 Hz). 13C NMR (100 MHz, D2O, Me4Si): 13C = 29.29, 30.93, 32.38, 49.43, 126.02, 128.52. MS (ESI): found 248 and 250 [M + H]+ with isotopic pattern of Br.
2.4.5 2-(7-hydroxy-2-oxo-2H-chromen-4-yl)-N-{2-[(4 or 2-nitro-1H-imidazole-1-yl)-butyl amino]-ethyl}-acetamide. Triethylamine (0.15 ml, 1.14 mmol) was added to the stirred solution of 5 (0.2 g, 0.76 mmol) in water. It was allowed to stir for 15 min followed by the addition of 8 (0.18 g, 0.76 mmol) and heated to 80 °C. Reaction was continued for 10 h and the progress of the reaction was monitored by TLC. Reaction mixture was evaporated under reduced pressure, dissolved in minimum amount of methanol and get precipitated as light brown solid with the addition of cold diethyl ether. Yield of 2-nitroimidazole derivative (2NIHC) and of 4-nitroimidazole derivative (4NIHC) was (0.24 g, 68% yield), (0.19 g, 60% yield) respectively.
2-nitroimidazole Derivative. 1H NMR (400 MHz, DMSO) 1H: 1.73–1.79 (m, 4H, CH2), 3.0 (brs, 4H, CH2), 3.44 (brs, 4H, CH2), 3.63 (s, 2H, CH2), 4.27–4.34 (m, 2H, CH2), 6.01 (s, 1H, CH), 6.35 (brs, 1H, CH), 6.60 (brs, 1H, CH), 7.00 (brs, 1H, CH), 7.29 (d, 2H, J = 16.4 Hz, CH). 13C NMR (100 MHz, DMSO) 13C: 26.22, 28.21, 37.13, 39.01, 49.90, 52.69, 60.99, 102.66, 111.81, 113.68, 126.30, 127.76, 127.94, 144.18, 151.31, 154.18, 160.35, 161.66, 163.69, 171.70. HRMS: 429.1782.
4-Nitroimidazole derivative. 1H NMR (400 MHz, DMSO) 1H: 1.69–1.76 (m, 2H, CH2), 1.90–1.97 (m, 2H, CH2), 2.39 (s, 2H, CH2), 2.50–2.51 (m, 4H, CH2), 4.10 (t, J = 6.4 and 6.4 Hz, 2H, CH2), 4.15 (t, J = 7.2 and 7.2 Hz, 2H, CH2), 6.20 (s, 1H, J = 0.8 Hz, CH), 6.71 (br, s, NH), 6.93- 6.96 (m, 2H, CH), 7.35 (br, s, 1H, NH), 7.67 (d, 1H, J = 8.8 Hz, CH), 7.91 (dd, 1H, J = 10.8 and 10.8 Hz, CH), 8.46 (dd, 1H, 10.4 and 10.4 Hz, CH). 13C NMR (100 MHz, DMSO) 13C: 18.59, 22.91, 25.68, 27.13, 47.50, 68.02, 101.62, 111.53, 112.95, 113.55, 122.03, 126.94, 137.90, 147.41, 154.01, 155.16, 162.02, 172.24. HRMS: 429.1846.
2.5 In-vitro study
2.5.1 Cell uptake by flow cytometry. To evaluate cell uptake, exposure of hypoxia was given to A549 cell line (<1% O2) in a hypoxic chamber. Hypoxic and normoxic A549 cells were incubated with 2NIHC and 4NIHC for 4 h at different concentration of 10 mM, 10 μM and 10 nm. After incubation cells were washed with PBS twice, detached using a treatment of 0.5 ml of trypsin for 5 minutes and resuspended in PBS. 6 μl of 1 μg ml−1 of propidium iodide in PBS was added, incubated for 30 min in the dark at −4 °C and then samples were analyzed by flow cytometry with a flow cytometer FACS calibur.
2.6 Computational analysis
2.6.1 Receptor for docking. Over activation of receptor protein kinase CK2α is a key factor in tumor genesis. Thus there is increasing interest on the deactivation of CK2α to inhibit the growth of cancerous cells. The human CK2 catalytic subunit was built using a homology modeling approach with α isoform as template (PDB code ID: 2ZJW). In order to gain insight into the putative binding mode of the 2NIHC with CK2α it is docked with a crystallographic structure of CK2α.
2.6.2 Ligand-receptor docking. X-ray crystal structure of human protein kinase CK2α complexed with ellagic acid [PDB ID: 2ZJW] with resolution of 2.40 Å was taken for molecular docking studies. The following criteria were kept in mind before choosing the protein–ligand complexes: non-covalent binding between protein and ligand, crystallographic resolution less than or equal to 3 Å and known experimental binding data. During the docking process, initially GLIDE performs a complete systematic search of the conformational, orientation and positional space of the docked ligand and eliminating unwanted conformations using scoring and followed by energy optimization. Preparation of the protein for docking included removal of unnecessary heteroatom and solvent coupled with addition of hydrogen atoms, bond order for crystal ligand and protein were adjusted and minimized up to 0.30 Å RMSD. Using extra precision (XP) mode of GLIDE application, docking studies was performed on coumarin derivative. Using ‘Glide grid generation’ the binding region was defined by a 36.75 Å, 4.62 Å, and 9.61 Å box centred on the centroid of the crystallographic ligand to confine the centroid of the docked ligand. No scaling factors were applied to the van der Waals radii. Best pose was selected on the basis of glide score and the interactions formed between the ligands and active site amino acids.Docking experiments were carried out by using GLIDE (grid based ligand docking with energetic) and ligand docking programme from calculations in extra precision (XP) mode. The docking was initiated with putting specified receptor grid and prepared ligand molecule together. GLIDE was run in the flexible docking mode which automatically generates conformations for each input ligand. The combination of position and orientation of a ligand relative to the receptor, along with its conformations in flexible docking is referred to as a ligand pose. The ligand poses that GLIDE generates pass through a series of hierarchial filters that evaluate the ligand's interaction with the receptor. The initial filters test the spatial fit of the ligand to the defined active site, and examine the complementarity of ligand–receptor interactions using a grid based method pattern after the empirical chemscore function. Poses that pass their initial screens enter the final stage of the algorithm, which involves evaluation and minimization of a grid approximation to the OPLS-AA non-bonded ligand receptor interaction energy. Final scoring was then carried out on the energy minimized poses. By default, Schrodinger's proprietary glide score multi-ligand scoring function was used to score the poses and analyze the result of binding affinity on the basis of the score. The time to dock one ligand was approximately 2–5 min.
2.7 Radiochemistry
2.7.1 99mTc radiocomplexation of 2-NIHC and 4-NIHC. The ligand i.e. 2-NIHC and 4NIHC (4.6 mmol) was dissolved in 1 ml of double distilled water. 100 μL of this solution was taken in sealed glass vial and stannous chloride (1 μmol; 1 mg) dissolved in 1 ml of 10% acetic acid (purged with N2) was added to it followed by the addition of freshly eluted 99mTechnecium pertechnetate (2 mCi; 200 μl) saline solution. The pH of the reaction mixture was adjusted to 7 using 0.1 M sodium carbonate solution. All contents were thoroughly mixed and vial was kept at room temperature for 0.5 h.
2.7.2 Radiochemical purity of 99mTc-2NIHC and 4-NIHC complex. The radiolabeling efficiency of above complexes was determined by using ascending instant thin layer chromatography on ITLC-SG (Paul Gelman, USA) using solvents 100% acetone, pyridine/acetic acid/water (PAW) (3
:
5
:
1.5) and saline. TLC strips were cut into 0.5 cm fragments and counts of each fragment were taken. The complexed 99mTc remained at the origin whereas free 99mTc moved with the solvent front.
2.7.3 Serum stability of complex. To measure the stability of the labeled complex, human serum was incubated with 99mTc radiolabeled complex at 37 °C for 1 h in a humidified incubator maintained at 5% CO2 and 95% air. Samples were centrifuged and serum was filtered through 0.22 μm syringe filter into a sterile plastic culture tubes. The radiolabeled conjugate was immediately placed in a CO2 chamber incubated at 37 °C and then analyzed for any dissociation of the complex by ITLC strips with 100% acetone and pyridine, acetic acid and water (PAW) (3
:
5
:
1.5) as a mobile phase. Percentage of free pertechnetate at different time intervals was estimated from it, which gives the percentage labeling efficiency or dissociation of the complex.
2.8 In vivo study
2.8.1 Biodistribution study in mice bearing hypoxic tumor. This study was done after 12 days of inoculation of EAT cells into BALB/c mice, so that hypoxic conditions were generated in tumor for its assessment. Approximately 2 mCi of 99mTc-2NIHC and 99mTc-4NIHC was injected intravenously into tumor bearing BALB/c mice through tail veins. At 1 h, 4 h and 24 h post injection, mice were sacrificed by cervical dislocation. Biodistribution measurements were evaluated in tumor and in various organs including lung, liver, kidney, stomach, blood and muscle. The selected tissues and organs were harvested and weighed. Uptake of 99mTc radioactivity in each sample was measured as % ID/g of the tissue with an automated gamma scintillation counter and was normalized with 99mTc-decay correction.
2.8.2 Scintigraphy in mice bearing normal and hypoxic tumor. As it was cleared from the biodistribution studies that 2NIHC accumulates more in the hypoxic tumor than 4NIHC along with fast clearance from the body. Therefore scintigraphic imaging of mice bearing hypoxic tumor was done with only 2NIHC. After intravenous injection of 2 mCi of 99mTc-2NIHC in mice bearing hypoxic tumor, animals were subjected to SPECT imaging at different time intervals of 1, 4 and 24 h post injection with an acquisition time of 10 min per view. The effect of 99mTc-2NIHC and 99mTc-4NIHC was also observed in normal tumor bearing mice where tumor was ∼1 week old. Image acquired at 4 h post injection shown the maximum drug uptake in hypoxic tumor site and at same time also taken for normal tumor bearing mice. The regions of interest (ROI) were drawn on the right thigh at tumor site and to the contralateral muscle indicating specific and non specific binding of drug. The tumor to muscle ratio was calculated from the counts per pixel of the ROI.
2.8.3 Antitumor screening. 7-Hydroxy coumarin and its derivatives were selective inhibitor of tumor growth, to evaluate the effect of pharmacological inhibition of tumor growth in vivo, we treated mice bearing EAT tumor only with 2NIHC. As molecular docking studies have shown that 2NIHC is more potent anticancerous drug than 4NIHC. Animals were randomly divided into three groups (control, 2NIHC dose 1 and dose 2) with each group having three mice. After initial implantation of cells, tumors were allowed to grow for 3 weeks till they become hypoxic and then treatment was initiated. In one set of group (n = 3), 2NIHC (1 mg ml−1) was administered on the 15th day of implantation of tumor by intravenous injection via a lateral tail vein at a dose of 5 mg kg−1 to one group and other group as 30 mg kg−1. Another set was taken as control which was without any vehicle. The dosing schedule involved injections every alternate day for doses 2 and 3 i.e. on 17th and 19th, followed by injection every third day i.e. 23rd and 27th day for remaining doses. Tumor diameters were measured on days 17, 19, 23, 27 and 31 with digital calipers and tumor volume was measured using the modified ellipsoid formula (L × W2/2). Throughout the experiments, all animals were accommodated in a pathogen-free laboratory environment. The study was terminated at 30 days post treatments.
3. Results and discussion
3.1 Chemistry: synthesis of 7-hydroxycoumarin coupled 2(4)-nitroimidazole derivatives
The mono tert-butyl ester of ethylenediamine was achieved by the controlling the stoichiometric amount of boc-anhydride. The (2-amino-ethyl)-carbamic acid tert-butyl ester 2 was prepared by dropwise addition of boc-anhydride to ethylene diamine in the presence of triethylamine at 0 °C followed by stirring at room temperature for 12 h in good yield (79%) (Scheme 1). The oily product was obtained used as such without further purification. The COOH group of 7-hydroxy coumarinyl-4-acetic acid 3 was in situ converted into to the active ester by using HOBT and DCC in dried DMF. The active ester was coupled to the NH2 group of synthesized oily compound for 2 h at 0 °C. The stirring was continued for 24 h at room temperature. The dicyclohexyl urea was filtered off and solvent was concentrated to afford the {2-(2-hydroxy-2-oxo-2H-chromen-4-yl)-acetylaminoethyl}-carbamic acid tert-butyl ester. The compound was purified by column chromatography to give 4 in 70% yield. Deprotection of tert-butyl ester 4 with trifluoroacetic acid in dried DCM furnished N-(2-amino-ethyl)-2-(7-hydroxy-2-oxo-2-2H-chromen-4-yl)-acetamide 5 (69%) in 6 h. Additionally 2-nitroimidazole and 4-nitroimidazole were refluxed with 1,4-dibromobutane in the presence of potassium carbonate at 70 °C for 0.5 h. The reaction mixture was cooled down to the room temperature and K2CO3 was removed by simple filteration by Whatman filter paper. The compound was purified by column chromatography and finally crystallized to yielded 1-(4-bromo-butyl)-4-nitro-1H-imidazole 8 as yellow powder and 1-(4-bromo-butyl)-2-nitro-1H-imidazole 9 as yellow crystal respectively in 76–79% overall yields. The bromo group of nitroimidazole derivative 8 and 9 were finally reacted with substituted acetamide 5 at 80 °C for 10 h in presence of triethylamine to give the desired products i.e. 2-(7-hydroxy-2-oxo-2H-chromen-4-yl)-N-{2-[4-nitro-imidazole-1-yl)-pentylamino]-ethyl}-acetamide (4-NIHC) 10 and 2-(7-hydroxy-2-oxo-2H-chromen-4-yl)-N-{2-[5-(2-nitro-imidazol-1-yl)-pentylamino]-ethyl}-acetamide (2-NIHC) 11 in 60–68% yields.
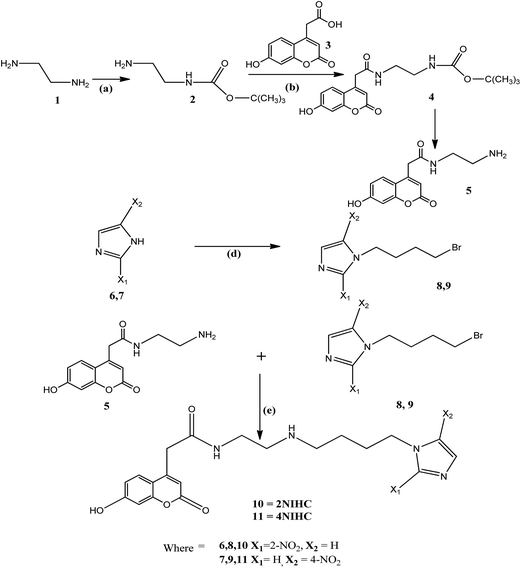 |
| Scheme 1 Synthesis of 2-(7-hydroxy-2-oxo-2H-chromen-4-yl)-N-{2-(4(or 2)-nitro-imidazole-1-yl)-pentylaminoethyl}-acetamide. (a) (boc)2O, TEA, 0 °C – rt, 79%; (b) 7-hydroxycoumarinyl-4-acetic acid, HOBT, DCC, 70%; (c) TFA, 0 °C, 69%; (d) 1,4-dibromobutane, K2CO3, 70 °C, 76–79%; (e) TEA, 70 °C, N-(2-amino-ethyl)-2-(7-hydroxy-2-oxo-2-2H-chromen-4-yl)-acetamide, 60–68%. | |
3.2 Biological characterization
3.2.1 In vitro binding. Before proceeding for their evaluation as a hypoxia marker, fluorescent spectra of 2NIHC and 4NIHC determined from a spectrofluorometer are illustrated in Fig. 1 which indicates that the both fluorescent coumarins have peak of emission wavelengths at 460 nm but the fluorescent intensity of 2NIHC is more than 4NIHC which makes 4NIHC more suitable for flow cytometric studies.
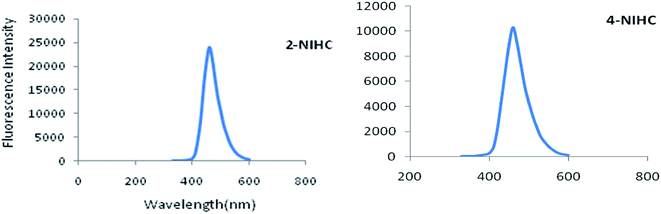 |
| Fig. 1 Fluorescence spectra measured by spectrofluorometer for 2NIHC and 4NIHC. | |
In vitro potency of 2NIHC and 4-NIHC was determined by flow cytometric analysis of cells incubated with both compounds under normoxic and hypoxic conditions as shown in Fig. 2.
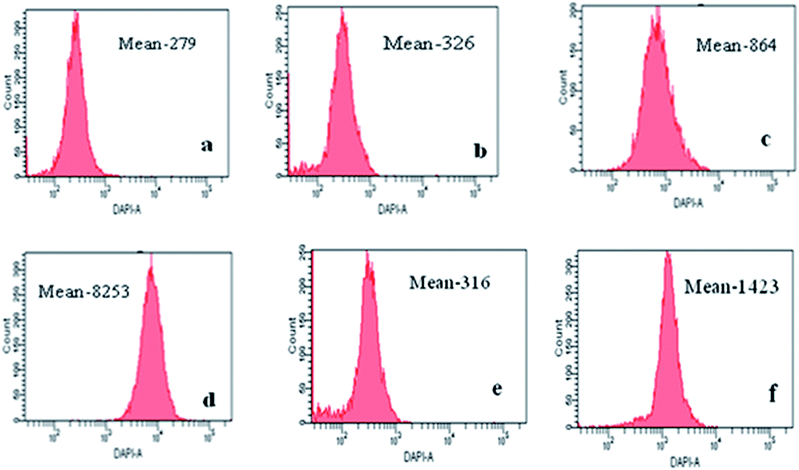 |
| Fig. 2 Flow cytometric analysis of A549 cells incubated at 37 °C with 10 mM of 2NIHC and 4NIHC for 4 h (a) control-hypoxic (b) control-oxic (c) 2NIHC-normoxic (d) 2NIHC-hypoxic (e) 4NIHC-normoxic (f) 4NIHC-hypoxic. | |
There was no significant difference in the controls of hypoxic and normoxic conditions and histograms of 2NIHC and 4NIHC can be compared to them. There was approximately 10 fold increase in mean fluorescence in hypoxic cells when A549 (ref. 21 and 22) cells were incubated with 2NIHC as compared to normoxic condition i.e. from 864 to 8253. However in 4NIHC, only five time increase in uptake was noticed in hypoxic condition i.e. 316 to 1423. The negligible amount of overlap between the fluorescence of hypoxic and well oxygenated cells incubated with 2NIHC would be a desirable property of a compound to measure a low proportion of hypoxic cells in a well oxygenated tumor. Mean fluorescence of pimonidazole23 in normoxic as well as in hypoxic cell is 56.8 and 286 with cells in the range of 101 to 103 i.e. mean fluorescence increases only five fold in hypoxic cells. Comparative studies showed that mean fluorescence is much higher for 2NIHC with cells in the range of more than 104, which makes it a highly selective hypoxia marker.
The concentration dependent development of fluorescence in cells incubated with 2NIHC is shown in Fig. 3. A large differential can be seen in fluorescence of cells incubated under normoxic and hypoxic conditions at different concentrations. Thus maximum fluorescence intensity was observed with 10 mM concentration of 2NIHC which indicates that drug binds firmly to the cellular macromolecules.
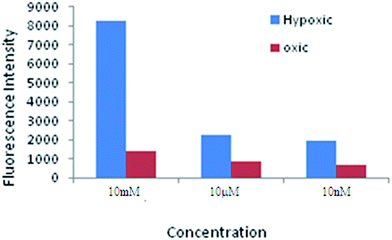 |
| Fig. 3 Mean fluorescence intensity from hypoxic and normoxic cells incubated for 4 h at 37 °C with 2NIHC. | |
3.2.2 Cytotoxicity assays. The cytotoxicity of compounds 2NIHC and 4NIHC was evaluated in vitro against human cervical carcinoma (HeLa) and human lung cancer (A-549) cell lines (Table 1). As a screening assay, the cytotoxicity was tested using an MTS tetrazolium reduction assay, for HeLa cell lines, and expressed as IC50 values. IC50 is the concentration (μM) required to reduce the absorbance values, i.e., the capability of the cells to reduce MTS, by 50% after 24 h of treatment. In vitro growth inhibitory effect of these compounds on EAT cells was also determined by measuring the absorbance by same assay for living cells. Briefly, EAT cells were seeded in 96-well microtiter plates. After incubation for 24 h in a dose-dependent way for various time periods (0–24 h), 50 μL of solution (2 mg mL−1 in PBS) was added to each well, and plates were incubated for an additional 4 h at 37 °C and finally measuring the absorbance at 490 nm.
Table 1 IC50 of nitroimidazoles
Compound |
IC50 ± SD (μM) |
HeLaa,b |
A549a,b |
EATa,b |
CK2αa,b |
Cells were exposed in optimal culture conditions in 96-well plates to seven concentrations of the compounds (1, 5, 10, 15, 20, 25 and 100 μM) or control medium for 24 h before determining cellular metabolic activity by an MTS tetrazolium compound bioreduction assay. Each value is determined from five samples using a non-linear regression analysis. |
2NIHC |
13.22 ± 0.95 |
9.58 ± 0.76 |
16.24 ± 0.42 |
11.28 ± 3.86 |
4NIHC |
19.02 ± 1.02 |
15.78 ± 0.58 |
19.98 ± 0.17 |
27.18 ± 5.39 |
Actinomycin D |
1.85 ± 0.46 |
1.16 ± 0.82 |
2.92 ± 0.66 |
4.86 ± 1.15 |
Doxorubicin |
9.21 ± 0.32 |
6.08 ± 0.14 |
7.83 ± 0.82 |
6.32 ± 0.20 |
Determination of CK2α activity. The final reaction mixture (final volume of 40 μL) for the determination of CK2 activity (apoenzyme CK2α or holoenzyme CK2α/2β2) contained: casein substrate (75 μg) or peptide substrate (RRRDDDSDDD, 20 μM), Tris–HCl pH 7.5 (20 mM), MgCl2 (20 mM) and appropriate concentrations of inhibitor in 1 μL DMSO. After 30 min of incubation at 30 °C, 40 μL of the assay mixture was spotted onto a square (2 cm × 2 cm) of Whatman 3 MM (for casein), which was immediately immersed in cold 5% (w/v) trichloroacetic acid containing 0.3% o-phosphoric acid (10 mL per square), and washed with H2O three times for 10 min. Then the requisite squares were washed in 96% ethanol and dried. The radioactivity was quantified by liquid scintillation counter.
3.2.3 In vitro binding and selectivity. To ensure that the binding of 99mTc-2NIHC on HeLa cells was specific, cells were incubated with 5 nM 99mTc-2NIHC alone or in the presence of 10 μM actinomycin D, after exposure of the cells to hypoxic or normoxic conditions for 24 h. As shown in Fig. 4, 99mTc-2NIHC bound only to hypoxic HeLa cells and not to HeLa cells that remained under normoxic conditions. Further, the binding of 99mTc-2NIHC to the hypoxic HeLa cells was homogenised by coincubation with 10 μM actinomycin D.
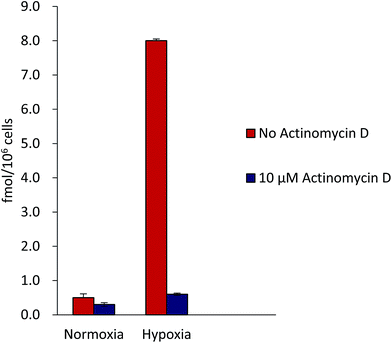 |
| Fig. 4 Binding of 2NIHC to HeLa cells. | |
2NIHC was incubated with hypoxic or normoxic HeLa cells in the presence or absence of 10 μM actinomycin D.
3.3 Computational analysis
3.3.1 Linear interaction energy model. Molecular modeling is a key tool in drug designing to predict the predominant binding modes of a ligand with protein of known three-dimensional structures. There are two aims of docking studies: accurate structural modeling and correct prediction of activity. The choice of best docked structure is made using a model energy score. Thus linear interaction energy (LIE) approach has been used to evaluate the binding free energy of this class of CK2α inhibitors. Several important structural features like glide score, glide energy, interaction energy of H-bond, Coulomb and van der Waals energy score of coumarin derivatives (Fig. 5) are identified from a qualitative analysis of their activity on CK2α (Table 2). The ranking of ligands is based on glide score. As summarized in Table 2 2NIHC proved totally refractory to all compounds with higher glide score of −9.5 against protein kinase CK2α. It means, it can fit well in the receptor cavity and forming energetically most stable drug receptor complex. More the glide score, higher the inhibitory activity of ligand to proliferating tumor.
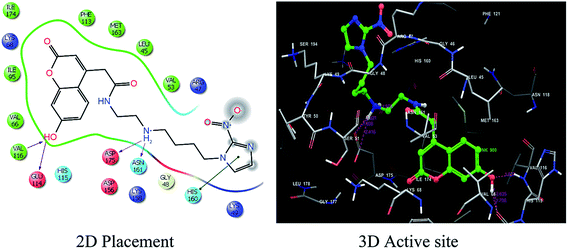 |
| Fig. 5 2D interaction diagram of cocrystal structure of CK2α (PDB code) with 10 hydrogen bonds with side chains of Val 116 and Glu 114, to the backbone of Asp 175 and Asn 161, by purple arrows and interaction of imidazole group with His 160 by green stick. | |
Table 2 Molecular docking results of 2NIHC, 4NIHC compared with well known anticancer coumarin derivative against crystal structure of protein kinase CK2α [PDB ID: 2ZJW]
Compound |
G* score |
EvdW |
Ecoul |
Emodel |
Glide Hbond |
Lipo |
10 |
−9.5 |
−40.1 |
−19.4 |
−75.5 |
−2.1 |
−4.4 |
11 |
−3.6 |
−46.6 |
−41.4 |
−58.1 |
−1.4 |
−2.7 |
1 (ref. 24) |
−7.3 |
−38.5 |
−5.6 |
−67.1 |
−1.0 |
−4.1 |
2 (ref. 25) |
−6.6 |
−50.6 |
−8.9 |
−38.5 |
−1.3 |
−3.5 |
Primarily hydroxyl group at the 7 position of coumarin moiety is considered as essential feature to increase the inhibitory potency. Analysis of the lipophilic contact term (−4.4) clearly reveals that the binding site is very hydrophilic and hydrophilic interactions dominate (Table 2). The van der Waals energy is considered to be the main energy term favoring binding. Even with less favorable van der Waals energy for 10 (−40.1 kcal mol−1) in comparison to 11 i.e. −50.6 kcal mol−1, ligand 4NIHC achieves the highest glide score. The Emodel energy term of −75.1 kcal mol−1 for the highest ranked ligand 10 shows that this is the dominant interaction controlling the ranking of a ligand. For instance, in 4NIHC substitution of nitro group at position 2 results in loss of activity with glide score decrease from −9.5 to −3.6. Although the EvdW and Ecoul energy terms for 11 is higher than 10 but possess low glide score due to low Emodel and Hbond values. However the contribution of Hbond for 10 is −2.1 which is highest among the other coumarin derivatives and revealed that there is good hydrogen bonding interaction between ligand 10 and the crystal structure of protein complex CK2α.
3.3.2 Ligand-receptor docking. 2NIHC was analysed in the CK2α binding pocket, which provide not only the relationship between the molecular structure and their activity but also provide the valuable clues for drug designing. As determined from the LIE model that 4NIHC possess the highest glide score, so it is docked with CK2α. The CK2α inhibitor is small and largely planar, which makes it feasible to lay out the nearby residues in a manner that is qualitatively representative of the actual 3D environment. A total of 19 residues in proximity of ligand along with hydrogen bonding interaction are shown in Fig. 5. The ligand is anchored into the active site by four hydrogen bonds, including a donor and an acceptor interaction with the sidechain of Glu114 and Val116 and a donor interaction with backbone of Asp175 and Asn161 shown by purple arrows. Imidazole ring oriented parallel to protein structure for a possible π–π interaction with the His160. Apart from an imidazole ring at one end of the ligand which projects out slightly from the active site, the ligand fit well within the cavity, which is shown by the continuity of the substitution contour line. Most of the ligand is deeply buried and shows a negligible solvent exposure, except for the exposed corner of imidazole ring.
3.4 Radiochemistry
3.4.1 Quality control of labeled conjugate. The labeled complex remained at the origin while 99mTcO4− moved toward the solvent front in acetone with Rf = 1.0. The optimum pH concentration for radiolabeling was 6.0–7.5 and labeling efficiency for 99mTc-DO3A-BT was calculated to be >99%. In vitro stability of 99mTc-DO3A-BT in PBS buffer at pH 7.0 was checked at different time intervals: 4, 6, 24 and 48 h and percentage labeling efficiency at 48 h was found to be 98%, implying that labeled conjugate was stable up to 48 h post labeling.
3.5 In vivo study
3.5.1 Biodistribution study in mice bearing hypoxic tumor. In order to assess the potentiality of our approach for the design of potential radiopharmaceuticals for nuclear oncology, evaluation in mice bearing hypoxic tumor was performed. In vivo biodistribution studies of 99mTc-2NIHC and 99mTc-4NIHC were performed in BALB/c mice xenografted in their right leg with EAT tumors. The time course tissue uptake of 99mTc-2NIHC at 1, 4 and 24 h was investigated and compared with 99mTc-4NIHC at 4 h after tracer injection, where maximum uptake takes place. Results obtained at 4 h post injection (p.i) are summarized in Table 3 and 4. Clearance from background tissues at 1 h and 4 h through blood (4.89% ID/g and 1.39% ID/g) and muscle (0.32% ID/g and 0.23% ID/g) was seems to be more rapid, so that tumor/muscle ratio of 99mTc-2NIHC increased with time and peak uptake value of 3.57(% ID/g) was observed at 4 h while for 99mTc-4NIHC it was 2.05 (% ID/g) at 1 h p.i. The 99mTc-2NIHC was delivered to the tumor more efficiently as compared to 99mTc-4NIHC with significant washout over time i.e. 0.24% ID/g was remained after 24 h. In general, hydrophilic character of both radioconjugates increases its clearance via renal-urinary pathway, which is preferable for the rapid clearance of background signals in images. Their kidney uptakes were high i.e. 22.41 ± 1.04% ID/g at 1 h for 99mTc-4NIHC and 37.99 ± % ID/g at 4 h for 99mTc-4NIHC indicative of extensive metabolism in the kidney. High uptake values of 99mTc-4NIHC and 99mTc-2NIHC in liver (15.53 ± 1.04% and 13.27 ± 1.04%) indicates that they are eliminated from the body via renal as well as by hepatobiliary pathways. No significant uptake in stomach suggests the in vivo stability of both compounds.
Table 3 Biodistribution of 99mTc-4NIHC in BALB/c mice bearing EAT hypoxic tumor at 4 h p.ia
Organ |
% ID/g |
1 h |
4 h |
24 h |
Values are expressed as the mean ± SD; n = 3. |
Blood |
4.82 ± 0.12 |
2.67 ± 0.14 |
2.75 ± 0.07 |
Heart |
1.88 ± 0.2 |
0.83 ± 0.11 |
0.82 ± 0.08 |
Kidney |
22.41 ± 1.04 |
18.37 ± 1.09 |
12.60 ± 0.95 |
Liver |
15.53 ± 1.04 |
11.21 ± 0.68 |
10.05 ± 0.22 |
Lungs |
1.65 ± 0.14 |
1.78 ± 0.21 |
2.89 ± 0.08 |
Stomach |
0.97 ± 0.14 |
0.89 ± 0.12 |
0.75 ± 0.08 |
Muscle |
0.81 ± 0.02 |
0.44 ± 0.01 |
0.45 ± 0.002 |
Tumor |
1.66 ± 0.02 |
0.86 ± 0.01 |
0.32 ± 0.02 |
T/M ratio |
2.05 ± 0.34 |
1.99 ± 0.27 |
1.02 ± 0.29 |
Table 4 Biodistribution of 99mTc-2NIHC in BALB/c mice bearing EAT hypoxic tumor at 4 h p.ia
Organ |
% ID/g |
1 h |
4 h |
24 h |
Values are expressed as the mean ± SD; n = 3. |
Blood |
4.89 ± 0.12 |
1.39 ± 0.14 |
0.24 ± 0.07 |
Heart |
0.55 ± 0.2 |
1.35 ± 0.11 |
0.13 ± 0.08 |
Kidney |
29.60 ± 1.04 |
37.99 ± 1.09 |
17.73 ± 0.95 |
Liver |
13.27 ± 1.04 |
9.05 ± 0.68 |
1.47 ± 0.22 |
Lungs |
9.23 ± 0.14 |
5.69 ± 0.21 |
2.46 ± 0.08 |
Stomach |
0.85 ± 0.14 |
0.52 ± 0.12 |
0.18 ± 0.08 |
Muscle |
0.32 ± 0.02 |
0.23 ± 0.01 |
0.07 ± 0.002 |
Tumor |
0.59 ± 0.02 |
0.83 ± 0.01 |
0.20 ± 0.02 |
T/M ratio |
1.82 ± 0.34 |
3.57 ± 0.27 |
2.97 ± 0.29 |
3.5.2 Scintigraphy in mice bearing normal and hypoxic tumor. Based on literature reports the effect of hypoxia on drug sensitivity had suggested that there was consistently more cytotoxicity under hypoxic conditions for the drugs. Both the ligands were studied for scintigraphic imaging at 1 h p.i shown in Fig. 6. The 1 h p.i. whole body planar image of the unblocked mouse demonstrated increase uptake in the tumor sites as well as in kidneys, liver, intestine and bladder in descending level of intensity relative to the background soft tissues. Tumor grafted in BALB/c mice clearly identifiable in the γ camera images (Fig. 6a) and showed high target to non target ratio of 99mTc-2NIHC. Blocking study was also performed which confirmed the selectivity of these radioligands. Due to high abdominal uptake, as seen with 99mTc-2NIHC, the potential application of radiotracers could be envisaged for imaging primary tumors that are outside the abdomen.
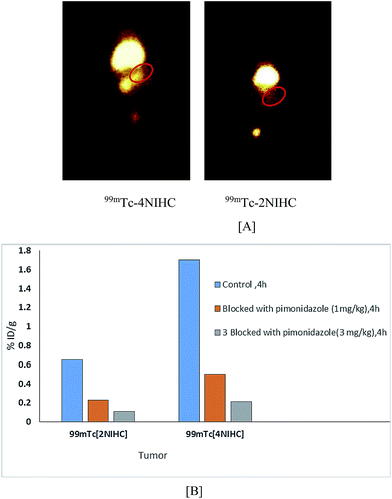 |
| Fig. 6 Whole-body γ image of BALB/c mice with tumor in right thigh (red circle indicate tumor site) at 1 h p.i. (A) Effect of blocking studies with pimonidazole (B). | |
3.5.3 Antitumor screening. In vivo animal studies were carried out to examine targeting and antitumor effects of 2NIHC using an EAT bearing BALB/c mice model. The growth of tumor volume was observed for 30 days after treatment with 2NIHC and control group which was without any vehicle at two dose rate 5 mg kg−1 and 30 mg kg−1 body weight (Fig. 7). 2NIHC dominantly controlled tumor growth compared to control group as demonstrated by calculating tumor volume (mm3), thus constitute it as an interesting candidate for the development of conceptually novel anticancer drug. The inhibitor concentrations were well tolerated and there was no significant reduction in weight of any of the mice during the tumor regression study. The result indicated that 2NIHC reduced the tumor growth as compared to control mainly at dose of 30 mg kg−1 and increased the survival time, constituting it as interesting candidates for the development of anticancer drugs.
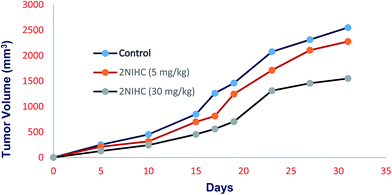 |
| Fig. 7 Tumor growth inhibition in EAT-bearing BALB/c mice. Data represented are mean of three animals experiment. 2NIHC was given at a dose of 5 mg kg−1 and 30 mg kg−1 on 15th, 17th, 19th and on 23rd day. Control was considered without any vehicle. | |
4. Conclusion
In conclusion, we have synthesized and evaluated two nitroimidazole conjugated fluorophore systems, 2NIHC and 4NIHC for the purpose of enhancing retention in hypoxic cells. Computational docking study showed that the 2NIHC is potent CK2 inhibitor. The flow cytometry demonstrated that only 2NIHC showed improved retention in hypoxic relative to normoxic cells. In vitro studies confirm its potential and selectivity for hypoxic cells. Further longitudinal animal studies and optimization may give a lead for new small molecule based agent which may be useful for clinical application.
Conflict of interest
This is also certified that there is no interest of conflict between the authors of this manuscript.
Abbreviations
NIHC | Nitroimidazoles hydroxyl coumarin |
NI | Nitroimidazoles |
CK2 | Casein kinase 2 |
EAT | Ehlrisch ascites tumor |
GLIDE | Grid based ligand docking with energetic |
MBq | Megabecquerel |
PET | Positron emission tomography |
XP | Extra precision |
ITLC | Instant thin layer chromatography |
PAW | Pyridine, acetic acid and water |
Acknowledgements
We are highly thankful to Dr R. P. Tripathi, Director INMAS, for providing necessary facilities. The work was supported by Council of Scientific and Industrial research (CSIR) and Defence Research and Development Organization, under R&D project INM-311(3.1).
References
- J. Zhou, T. Schmid, S. Schnitze and B. Brune, Cancer hypoxia and cancer progression, Cancer Lett., 2006, 237(1), 10–21 CrossRef CAS PubMed
. - P. Vaupel and L. Harrison, Tumor hypoxia: causative factors, compensatory mechanisms and cellular response, Oncologist, 2004, 9(5), 4–9 CrossRef PubMed
. - J. M. Browm, Tumor hypoxia in cancer therapy, Methods Enzymol., 2007, 435(10), 297–321 Search PubMed
. - A. M. Shannon, D. J. Bouchier-Hates, C. M. Condron and D. Toomey, Tumor hypoxia, chemotherapeutic resistance and hypoxia related therapies, Cancer Treat. Rev., 2003, 29(4), 297–307 CrossRef CAS PubMed
. - H. Lyng, K. Sundfor and E. K. Rofstad, Oxygen tension in human tumors measured with polarographic needle electrodes and its relationship to vascular density, necrosis and hypoxia, Radiother. Oncol., 1997, 44(2), 163–169 CrossRef CAS PubMed
. - A. Nunn, K. Linder and H. W. Strauss, Nitroimidazoles and imaging hypoxia, Eur. J. Nucl. Med. Mol. Imaging, 1995, 22(3), 265–280 CrossRef CAS PubMed
. - G. Mees, R. Diercks, C. Vangestel and C. V. Wiele, Molecular imaging of hypoxia with radiolabeled agents, Eur. J. Nucl. Med. Mol. Imaging, 2009, 36(10), 1674–1886 CrossRef CAS PubMed
. - L. S. Ziemer, S. M. Evans, A. V. Kachur, A. L. Shuman, C. A. Cardi, W. T. Jenkins, J. S. Karp, A. Alavi, W. R. Dolbier and C. J. Koch, Non invasive imaging of hypoxia in rats using the 2-nitroimidazole 18F-EF5, Eur. J. Nucl. Med. Mol. Imaging, 2003, 30(2), 259–266 CrossRef CAS PubMed
. - L. Kaisa, O. Vesa, N. Samuel, G. Tove, R. Anne, E. Olli and M. Heikki, Quantifying tumor hypoxia with 18F-fluroerythronitroimidazole (18F-FETNM) using tumor to plasma ratio, Eur. J. Nucl. Med., 2003, 30(3), 101–108 Search PubMed
. - K. E. Linder, Y. W. Chan, J. E. Cyr, M. F. Malley, D. P. Nowotnik and A. D. Nunn, TcO(PnAO-1-(2-nitroimidazole)) [BMS-181321], a new technetium-containing nitroimidazole complex for imaging hypoxia: synthesis, characterization, and xanthine oxidase-catalyzed reduction, J. Med. Chem., 1994, 37(1), 9–17 CrossRef CAS PubMed
. - T. Melo, J. Duncan, J. R. Ballinger and A. M. Rauth, BRU59–21, a second generation 99mTc-labeled 2-nitroimidazole for imaging hypoxia in tumors, J. Nucl. Med., 2000, 41(1), 169–176 CAS
. - S. J. Wang, J. M. Lo, L. H. Shen and S. P. Wey, Tumor Uptake of 99mTc-HL91 and 99mTc-PnAO-Nitroimidazole in an Animal Model of Non-small Cell Lung Cancer, Ann. Nucl. Med., 2004, 17, 139–146 Search PubMed
. - R. D. Okada, G. Johnson, K. N. Nguyen, B. Edwards, C. M. Archer and J. D. Kelly, 99mTc-HL91 effects of low flow and hypoxia on a new ischemia-avid myocardial imaging agent, Circulation, 1997, 95, 1892–1899 CrossRef CAS PubMed
. - G. J. R. Cook, S. Houston, S. F. Barrington and I. Fogelman, Technetium-99m-labeled HL91 to identify tumor hypoxia: correlation with fluorine-18-FDG, J. Nucl. Med., 1998, 39, 99–103 CAS
. - P. Wardman, E. D. Clarke, R. J. Hodgkiss, R. W. Middleton, J. Parrick and M. R. L. Stratford, Nitroaryl compounds as potential fluorescent probes for hypoxia. II. Identification and properties of reductive metabolites, Int. J. Radiat. Oncol., Biol., Phys, 1984, 10(8), 1353–1356 CrossRef
. - R. J. Hodgkiss, G. W. Jones, A. Long, R. W. Middleton, J. Parrick, M. R. L. Stratford, R. Wardman and G. D. Wilson, Fluorescent markers for hypoxic cells: a study of nitroaromatic compounds, with fluorescent heterocyclic side chains, that undergo bioreductive binding, J. Med. Chem., 1991, 34(7), 2268–2274 CrossRef CAS PubMed
. - Q. Zhu, M. Uttamchandani, D. Li, M. L. Lesaicherre and S. Q. Yao, Enzymatic profiling system in a small molecule array, Org. Lett., 2003, 5(8), 1257–1260 CrossRef CAS PubMed
. - I. Kostova, Synthetic and natural coumarins as cytotoxic agents, Curr. Med. Chem.: Anti-Cancer Agents, 2005, 5(1), 29–46 CrossRef CAS PubMed
. - Z. Dan, H. Jennifer, H. Robert, A. Mahan, P. Owen, D. Gordafaried, H. H. Xueqyn and C. Sarah, Inhibition of protein kinase CK2 expression and activity blocks tumor growth, Mol. Cell. Biochem., 2010, 333(4), 159–167 Search PubMed
. - C. Adriana, B. Roberto, B. Andrea, C. Giorgio, Z. Samuele, P. Giorgia, M. Marco, Z. Giuseppe, U. Eugenio, G. Adriano, A. P. Lorenzo, M. Flavio and M. Stefano, Coumarin as Attractive Casein Kinase 2 (CK2) Inhibitor Scaffold: An Integrate Approach to elucidate the Putative Binding Motif and Explain Structure-Activity Relationships, J. Med. Chem., 2008, 51(4), 752–759 CrossRef PubMed
. - T. Wang, T. Nik, A. Goto, S. Ota, T. Morikawa, Y. Nakamura, E. Ohara, S. Ishikawa, H. Aburatani, J. Nakajima and M. Fukayama, Hypoxia increases the motility of lung adenocarcinoma cell line A549 via activation of the epidermal growth factor receptor pathway, Cancer Sci., 2007, 98, 506–518 CrossRef CAS PubMed
. - S. E. Schnitzer, T. Schmid, J. Zhou and B. Brüne, Hypoxia and HIF-1protect A549 cells from drug-induced apoptosis, Cell Death Differ., 2006, 13, 1611–1613 CrossRef CAS PubMed
. - N. K. Wagh, Z. Zhou, S. M. Ogbomo, W. Shi, S. K. Brusnahan and J. C. Garrison, Development of hypoxia enhanced 111In-labeled bombesin conjugates: design, synthesis and in vitro evaluation in PC-3 human prostate cancer, Bioconjugate Chem., 2012, 23, 527–537 CrossRef CAS PubMed
. - T. Nadia, M. Alfonso, C. M. Paul, L. Yuanmei, S. Andrea, D. Shoukat, W. Jean-Yves and T. S. Claudiu, Glycosyl coumarin carbonic anhydrase IX and XII inhibitors strongly attenuate the growth of primary breast tumor, J. Med. Chem., 2011, 54(24), 8271–8277 CrossRef PubMed
. - V. S. Koneni, K. Abdhesh, K. Manoj, S. Jayanta and S. Sudhir, Synthesis and in vitro evaluation of novel coumarin–chalcone hybrids as potential anticancer agents, Bioorg. Med. Chem. Lett., 2010, 20(24), 7205–7211 CrossRef PubMed
.
|
This journal is © The Royal Society of Chemistry 2015 |