DOI:
10.1039/C5RA17503H
(Paper)
RSC Adv., 2015,
5, 88133-88140
Stereocontrolled construction of 3H-furo[3,4-b]chromen-1(9H)-one scaffolds via organocatalyzed Michael addition and the following intramolecular dehydration†
Received
29th August 2015
, Accepted 9th October 2015
First published on 12th October 2015
Abstract
An efficient approach for the stereocontrolled construction of 3H-furo[3,4-b]chromen-1(9H)-one skeleton has been successfully developed through a sequential Michael addition/intramolecular dehydration strategy. The Michael addition of tetronic acid to 2-((E)-2-nitrovinyl)phenols catalyzed by a bifunctional squaramide derived from L-tert-leucine, and the subsequent intramolecular dehydration promoted by concentrated sulfuric acid, proceed smoothly to give the corresponding pharmaceutically valuable 3H-furo[3,4-b]chromen-1(9H)-ones in acceptable yields with 79–97% ee.
Introduction
The benzopyran scaffold ranks among the most commonly found heterocycles in known bioactive molecules. Functionalized benzopyran and fused cyclic molecular frameworks with benzopyran units have attracted much attention due to their considerable biological and pharmacological activities, such as use as antibacterial,1 antitumor,2 anti-anaphylactic,3 antisecretory agents,4 apoptosis inducers,5 and Chk1-inhibitors.6 Among them, 3H-furo[3,4-b]chromen-1(9H)-ones (benzopyran lactones) represent an important class of polycyclic benzopyrans, as they are the privileged structural motif of a number of synthetic 4-oxa-podophyllotoxin derivatives displaying an extensive array of biological activities (Fig. 1).7 Despite their high relevance as biologically active compounds, however, nonracemic 3H-furo[3,4-b]chromen-1(9H)-ones have been entirely unknown. Since the preparation of enantiomerically pure compounds has become a stringent requirement for pharmaceutical synthesis,8 the development of efficient synthesis of chiral benzopyran lactones in highly enantioselective manner will be of great importance and remains a challenge task. As a part of our continued interest in the stereoselective synthesis of biologically relevant heterocycles,9 herein we describe the tertiary amine-squaramide catalyzed Michael addition of tetronic acid (4-hydroxyfuran-2-one) to 2-((E)-2-nitrovinyl)phenols and the subsequent acid promoted intramolecular dehydration reaction, which provide a straightforward access to a variety of functionalized chiral benzopyran lactones in a highly enantioselective manner (Scheme 1).10
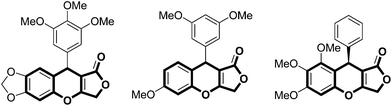 |
| Fig. 1 Examples of bioactive compounds bearing a 3H-furo[3,4-b]chromen-1(9H)-one motif. | |
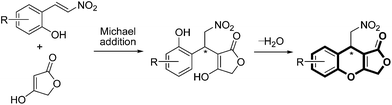 |
| Scheme 1 Construction of 3H-furo[3,4-b]chromen-1(9H)-one skeleton. | |
Results and discussion
Initially, 2-((E)-2-nitrovinyl)phenol (1a) and 4-hydroxyfuran-2-one (2a) were chosen as the model substrates, and the initial Michael addition reaction was investigated at 20 °C in methylene chloride, the following intramolecular dehydration was performed at 100 °C in glacial acetic acid in the presence of 2 equivalents of concentrated sulfuric acid. Various thiourea-11 and squaramide-based12 hydrogen-bonding catalysts I–V (Fig. 2) were screened for the Michael addition reaction (Table 1, entries 1–8). In the presence of (1R,2R)-1,2-diphenylethane-1,2-diamine derived thiourea catalyst I, the desired polycyclic product 3aa was obtained in 75% yield with 30% ee (Entry 1). Under the same condition, sharply increased enantioselectivity (63% ee) was observed for the squaramide catalyst IIa bearing the same chiral diamine scaffold (entries 2 vs. entry 1), which indicate that the squaramide catalysts are superior to thiourea catalysts in this transformation. We then turn our attention to other chiral squaramide catalysts (entries 3–8). The effect of the tertiary amino group on reactivity and enantioselectivity was firstly investigated in more detail (entries 3 and 4). Of the tertiary amino groups surveyed, piperidinyl group resulted in an obviously improved enantioselectivity of 79% ee (entry 4). Further changing the chiral diamine skeleton of the squaramide catalyst revealed that squaramide Va, derived from L-tert-leucine, was the optimal catalyst for this sequential process and afforded the desired product 3aa with the highest ee value (entry 7, 81%).
Table 1 Screening of catalysta

|
Entry |
Catalyst |
Time (h) |
Yieldb (%) |
eec (%) |
Michael addition reactions were carried out with 2-((E)-2-nitrovinyl)phenol (1a, 0.24 mmol), tetronic acid (2a, 0.2 mmol) and catalyst (20 mol%) in methylene chloride (1 mL) at 20 °C. Intramolecular dehydration reactions were performed in glacial acetic acid (1.5 mL) at 100 °C in the presence of 2 equivalent of concentrated sulfuric acid. Isolated yield after two steps. Determined by HPLC analysis with a chiral stationary phase. |
1 |
I |
52 |
74 |
−30 |
2 |
IIa |
28 |
94 |
−63 |
3 |
IIb |
68 |
97 |
−67 |
4 |
IIc |
48 |
87 |
−79 |
5 |
III |
92 |
77 |
−38 |
6 |
IV |
48 |
95 |
31 |
7 |
Va |
72 |
87 |
81 |
8 |
Vb |
92 |
90 |
57 |
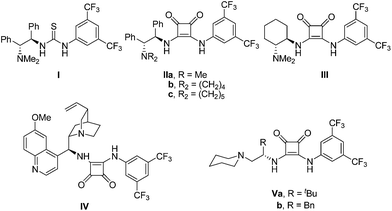 |
| Fig. 2 Screened thiourea- and squaramide-based organocatalyst. | |
Having confirmed squaramide Va as the optimum catalyst for the reaction, other parameters, such as solvent, reaction temperature, and catalyst loading, influencing the reaction were further investigated employing 2-((E)-2-nitrovinyl)phenol (1a) and 4-hydroxyfuran-2-one (2a) as the model substrates. The results are listed in Table 2.
Table 2 Optimization of reaction conditionsa

|
Entry |
Solvent |
Time (h) |
Yieldb (%) |
eec (%) |
Unless otherwise specified, the Michael addition reactions were carried out with 1a (0.24 mmol), 2a (0.20 mmol) and squaramide Va (20 mol%) in solvent (1 mL) at 20. The intramolecular dehydration reactions were performed in glacial acetic acid (1.5 mL) at 100 °C in the presence of 2 equivalent of concentrated sulfuric acid. Isolated yield after two steps. Determined by HPLC analysis using a chiral stationary phase. Reaction was performed at 0 °C. The catalyst loading is 10 mol%. |
1 |
CH2Cl2 |
72 |
87 |
81 |
2 |
CHCl3 |
21 |
92 |
58 |
3 |
ClCH2CH2Cl |
40 |
90 |
65 |
4 |
PhCH3 |
40 |
58 |
47 |
5 |
CH3CN |
72 |
87 |
78 |
6 |
Et2O |
144 |
79 |
54 |
7 |
EA |
72 |
85 |
80 |
8 |
THF |
40 |
81 |
91 |
9d |
THF |
144 |
75 |
92 |
10e |
THF |
59 |
82 |
97 |
With 20 mol% of Va as the catalyst at 20 °C, various solvents have been examined for the initial Michael addition reaction (entries 1–8). The asymmetric Michael addition could be carried out smoothly in several conventional solvents with ee values ranged from 47–91%. THF was found to be superior to any other solvents screened, delivering the tricyclic product 3aa in 81% yield with the highest enantioselectivity of 91% ee (entry 8). Although a slightly increased enantioselectivity was observed, conducting the reaction at 0 °C resulted in a quite slower reaction (entry 9 vs. entry 8). Finally, it was gratifying that the enantioselectivity could be further improved to 97% ee by adjusting the catalyst loading to 10 mol% (entry 10). Under this condition, the Michael addition product of ortho-nitrovinylphenol 1a and tetronic acid 2a was isolated in 93% yield with a comparable enantioselectivity of 96% ee.
Having established the optimal reaction conditions (Table 2, entry 10), we next examined the scope and limitations of the above sequential process with variants of 2-((E)-2-nitrovinyl)phenols 1 (Table 3). Although the reactions of these substituted 2-((E)-2-nitrovinyl)phenols proceeded more slowly, both the electron-withdrawing and electron-donating substituents were tolerated well in this sequential Michael addition/dehydration transformation, delivering the corresponding products in good to excellent enantioselectivities irrespective of the substitution pattern (entries 1–13). The effect of electronic property of the substituent on the intramolecular dehydration process is obvious. Generally, the existence of electron-withdrawing group decreases the nucleophilicity of the phenolic hydroxy group and slows down the reaction. Especially for the dihalo-substituted substrate 1d and 1f, quite sluggish reactions were observed and provided the cyclization product 3da and 3fa in low yield even after a prolonged reaction time (entries 4 and 6). In addition, 2-hydroxynaphthalene-1-carbaldehyde derived nitroolefin 1n was also applicable, albeit the Michael addition reaction proceeded more slowly with a partial conversion of 1n and afforded the corresponding product with decreased yield (entry 14). Because all of the products were solids with high melting points, the optical purity of the product could be dramatically improved by a single recrystallization from methylene chloride/hexane (v/v, 2/1) (entry 8). Chen,13a Sohtome and Nagasawa13b–d have reported the formation of dimer of that structures containing both nitro and phenol group. However, the generation of the dimer was not observed in our system, in some cases, the observed low yields may be attributed to the formation of appreciable amounts of tarry residue upon treatment with concentrated sulfuric acid.
Table 3 Substrate scope for the newly developed sequential processa
In addition, tolerance to substitution on the tetronic acid derivative 2 was also preliminarily investigated (Scheme 2). The reaction of 4-hydroxy-5,5-dimethylfuran-2(5H)-one (2b) to nitrostyrene 1a also ran smoothly to provide the desired product 3ab in acceptable yield with high enantioselectivity. Racemic, (R)- and (S)-4-hydroxy-5-methylfuran-2(5H)-ones (2c–e) were also proved to be suitable reaction partners with 2-((E)-2-nitrovinyl)phenol (1a), furnishing the desired products 3ac–3ae with 92%, 98% and >99% ee, respectively.
|
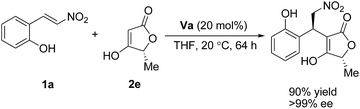 | (1) |
|
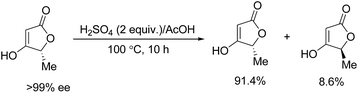 | (2) |
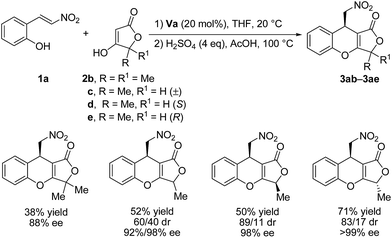 |
| Scheme 2 Reaction of other tetronic acid derivatives. | |
In case of the reaction of optically pure tetronic acids 2d and 2e, partial racemization was observed at the C4 position of the lactone ring. To determine which step is responsible for the racemization, two additional experiments were performed: (1) Va-catalyzed simple Michael addition of 2e to 1a (eqn (1) and (2)) treatment of optically active 2e under the dehydration condition (eqn (2)). These results clearly indicate that the partial racemization was occurred in the subsequent acid promoted dehydration step rather than in the first Michael addition step.
The absolute configuration and molecular structure of 3aa was unambiguously determined by X-ray crystallography and the remaining configurations are assumed by analogy (Fig. 3).
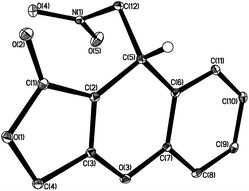 |
| Fig. 3 X-ray crystal structure of (R)-3aa. Most of the hydrogen atoms have been omitted for clarity. | |
To explanation of the observed stereochemistry outcome of the reaction, a plausible transition state for the initial Michael addition reaction is shown in Fig. 4. ortho-Nitrovinylphenol 1a is activated and fixed through double hydrogen bonding interaction with the squaramide moiety of catalyst Va. Meanwhile, tetronic acid is deprotonated by the basic tertiary amino group in the catalyst, and the resulting enolate is assumed to interact with the protonated amine by hydrogen bonding. Thereafter, nucleophilic addition of the enolate to the nitroolefin from the si-face preferentially leads to the formation of (R)-adduct.
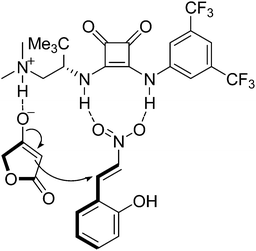 |
| Fig. 4 Proposed transition state for the Michael addition step. | |
Conclusions
We have developed a new methodology to access 3H-furo[3,4-b]chromen-1(9H)-one derivatives enantioselectively through sequential Michael addition and intramolecular dehydration reactions. Bifunctional chiral squaramide Va derived from L-tert-leucine was found to be the efficient catalyst for the conjugate addition of tetronic acid to 2-((E)-2-nitrovinyl)phenols. The intramolecular dehydration of the Michael addition products was achieved in the presence of concentrated sulfuric acid. A number of 3H-furo[3,4-b]chromen-1(9H)-one derivatives were synthesized in acceptable to good yields and with good to excellent enantioselectivities.
Experimental
General methods
All reagents and solvents were commercial grade and purified prior to use when necessary. NMR spectra were acquired on Varian 400 MHz instrumental. Chemical shifts are measured relative to residual solvent peaks as an internal standard set to δ 7.26 and δ 77.0 (CDCl3), 2.50 and 38.45 (DMSO-d6). Specific rotations were measured on a Perkin-Elmer 341 MC polarimeter. Enantiomeric excesses were determined on a Shimadzu LC-20A instrument (chiral column; mobile phase: hexane/i-PrOH). HRMS was performed on a Varian QFT-ESI instrumental. Melting points were determined on a Taike X-4 melting point apparatus. All temperatures were uncorrected.
General procedure for sequential Michael addition–intramolecular dehydration reaction of tetronic acids and 2-((E)-2-nitrovinyl)phenols
A solution of squaramide catalyst Va (10 mol%), 2-((E)-2-nitrovinyl)phenols (1, 0.24 mmol) and tetronic acids (2, 0.2 mmol) in tetrahydrofuran (1 mL) was stirred at 20 °C. After the reaction was complete (monitored by TLC), the reaction mixture was concentrated under reduced pressure and the crude product was used directly in the next step without further purification. The Michael addition product of 1a and 2a was purified by column chromatography on silica gel (200–300 mesh, PE/EtOAc/AcOH = 80/20/1) and fully characterized by 1H NMR, 13C NMR, HRMS and specific rotation data.
4-Hydroxy-3-((R)-1-(2-hydroxyphenyl)-2-nitroethyl)furan-2(5H)-one
Pale yellow oil, 93% yield, [α]25D − 5.45 (c 1.47, ethyal acetate), 96% ee. 1H NMR (400 MHz, DMSO-d6): δ 10.95 (br. s, 2H), 7.13 (d, J = 7.6 Hz, 1H), 7.06 (t, J = 7.6 Hz, 1H), 6.82 (d, J = 8.0 Hz, 1H), 6.73 (t, J = 7.6 Hz, 1H), 5.07 (dd, J = 12.8, 10.0 Hz, 1H), 4.80–4.89 (m, 2H), 4.66 (s, 2H). 13C NMR (100.6 MHz, DMSO-d6): δ 175.9, 173.9, 154.6, 128.3, 128.2, 123.8, 118.9, 115.2, 96.6, 75.0, 66.5, 32.1. HRMS (ESI) m/z calcd for C12H12NO6 [M + H]+: 266.0659, found 266.0660. HPLC analysis (Chiralpak OJ-H column, hexane
:
2-propanol
:
TFA = 20
:
80
:
0.1, flow rate = 1.0 mL min−1, wavelength = 220 nm): Rt = 13.76 (major) and 20.31 min (minor).
To a solution of the crude Michael addition product in glacial acetic acid (1.5 mL) was added two equivalent of concentrated sulfuric acid, and the resulting mixture was heated to 100 °C and maintained this temperature for completion of the reaction (monitored by TLC). Upon completion of the reaction, the product was directly purified by column chromatography on silica gel (200–300 mesh, PE/EtOAc = 2/1) to afford the desired 3H-furo[3,4-b]chromen-1(9H)-ones 3. The title compounds were fully characterized by 1H NMR, 13C NMR, HRMS and specific rotation data. The enantiomeric excess of the pure products was determined by HPLC analysis using a chiral stationary phase.
(R)-9-Nitromethyl-3H-furo[3,4-b]chromen-1(9H)-one (3aa)
White solid, mp 138–140 °C, 80% yield, [α]25D + 12.96 (c 1.17, CHCl3), 97% ee. 1H NMR (400 MHz, DMSO-d6): δ 7.58 (d, J = 7.6 Hz, 1H), 7.39 (t, J = 7.6 Hz, 1H), 7.29 (t, J = 7.6 Hz, 1H), 7.24 (d, J = 8.0 Hz, 1H), 5.07–5.15 (m, 3H), 4.97 (dd, J = 12.8, 4.0 Hz, 1H), 4.59 (s, 1H). 13C NMR (100.6 MHz, DMSO-d6): δ 171.0, 170.6, 150.3, 129.6 (2C), 126.0, 118.8, 117.4, 98.6, 77.8, 66.1, 30.7. HRMS (ESI) m/z calcd for C12H10NO5 [M + H]+: 248.0553, found 248.0552. HPLC analysis (Chiralpak OJ-H column, hexane
:
2-propanol = 70
:
30, flow rate = 1.0 mL min−1, wavelength = 220 nm): Rt = 39.17 (major) and 44.54 min (minor).
(R)-7-Fluoro-9-nitromethyl-3H-furo[3,4-b]chromen-1(9H)-one (3ba)
White solid, mp 189–191 °C, 81% yield, [α]25D + 5.14 (c 1.40, CHCl3), 87% ee. 1H NMR (400 MHz, DMSO-d6): δ 7.54 (dd, J = 9.2, 2.8 Hz, 1H), 7.32 (dd, J = 9.2, 4.8 Hz, 1H), 7.26 (dt, J = 9.2, 2.8 Hz, 1H), 5.19 (dd, J = 12.8, 4.0 Hz, 1H), 5.03–5.15 (m, 2H), 4.99 (dd, J = 12.8, 3.6 Hz, 1H), 4.60 (s, 1H). 13C NMR (100.6 MHz, DMSO-d6): δ 171.1, 170.4, 159.1 (d, J = 242.0 Hz), 146.7 (d, J = 2.3 Hz), 121.0 (d, J = 8.2 Hz), 119.2 (d, J = 8.8 Hz), 116.5 (d, J = 23.7 Hz), 115.8 (d, J = 24.7 Hz), 97.9, 77.3, 66.0, 31.0. HRMS (ESI) m/z calcd for C12H9FNO5 [M + H]+: 266.0459, found 266.0453. HPLC analysis (Chiralpak AD-H column, hexane
:
2-propanol = 80
:
20, flow rate = 1.0 mL min−1, wavelength = 220 nm): Rt = 13.22 (minor) and 14.55 min (major).
(R)-7-Chloro-9-nitromethyl-3H-furo[3,4-b]chromen-1(9H)-one (3ca)
White solid, mp 203–205 °C, 66% yield, [α]25D − 14.83 (c 1.20, CHCl3), 87% ee. 1H NMR (400 MHz, DMSO-d6): δ 7.75 (d, J = 2.0 Hz, 1H), 7.45 (dd, J = 8.8, 2.4 Hz, 1H), 7.30 (d, J = 8.8 Hz, 1H), 5.20 (dd, J = 12.8, 4.0 Hz, 1H), 5.04–5.16 (m, 2H), 4.98 (dd, J = 12.8, 3.6 Hz, 1H), 4.60 (s, 1H). 13C NMR (100.6 MHz, DMSO-d6) δ 170.9, 170.3, 149.2, 129.64, 129.5, 129.2, 121.1, 119.2, 98.4, 77.4, 66.0, 30.7. HRMS (ESI) m/z calcd for C12H9ClNO6 [M + H]+: 282.0162, found 282.0160. HPLC analysis (Chiralpak AD-H column, hexane
:
2-propanol = 90
:
10, flow rate = 1.0 mL min−1, wavelength = 220 nm): Rt = 27.36 (minor), and 29.23 min (major).
(R)-5,7-Dichloro-9-nitromethyl-3H-furo[3,4-b]chromen-1(9H)-one (3da)
Red solid, mp 218–220 °C, 29% yield, [α]25D + 37.73 (c 0.32, CHCl3), 87% ee. 1H NMR (400 MHz, CDCl3): δ 7.43 (d, J = 2.4 Hz, 1H), 7.20 (d, J = 2.0 Hz, 1H), 4.91–5.03 (m, 3H), 4.82 (dd, J = 13.2, 3.6 Hz, 1H), 4.48 (s, 1H). 13C NMR (100.6 MHz, CDCl3): δ 170.2, 169.8, 145.7, 131.4, 130.7, 126.9, 124.5, 121.5, 99.5, 76.3, 66.2, 31.6. HRMS (ESI) m/z calcd for C12H11Cl2N2O5 [M + NH4]+: 333.0040, found 333.0040. HPLC analysis (Chiralpak AD-H column, hexane
:
2-propanol = 90
:
10, flow rate = 1.0 mL min−1, wavelength = 220 nm): Rt = 20.42 (minor) and 23.33 min (major).
(R)-7-Bromo-9-nitromethyl-3H-furo[3,4-b]chromen-1(9H)-one (3ea)
Pale red solid, mp 215–217 °C, 61% yield, [α]25D + 56.80 (c 1.00, CHCl3), 86% ee. 1H NMR (400 MHz, DMSO-d6): δ 7.80 (s, 1H), 7.58 (d, J = 8.8 Hz, 1H), 7.23 (d, J = 8.8 Hz, 1H), 5.20 (dd, J = 12.8, 3.6 Hz, 1H), 5.04–5.15 (m, 2H), 4.97 (dd, J = 12.8, 3.6 Hz, 1H), 4.60 (s, 1H). 13C NMR (100.6 MHz, DMSO-d6): δ 170.8, 170.2, 149.7, 132.4, 132.1, 121.5, 119.5, 117.6, 98.5, 77.4, 66.1, 30.6. HRMS (ESI) m/z calcd for C12H9BrNO6 [M + H]+: 325.9659, found 325.9666. HPLC analysis (Chiralpak AD-H column, hexane
:
2-propanol = 95
:
5, flow rate = 1.0 mL min−1, wavelength = 220 nm): Rt = 57.42 (minor) and 60.13 min (major).
(R)-5,7-Dibromo-9-nitromethyl-3H-furo[3,4-b]chromen-1(9H)-one (3fa)
Red solid, mp 254–256 °C, 25% yield, [α]25D + 43.50 (c 0.27, CHCl3), 94% ee. 1H NMR (400 MHz, CDCl3): δ 7.73 (d, J = 2.0 Hz, 1H), 7.39 (d, J = 2.0 Hz, 1H), 4.91–5.03 (m, 3H), 4.82 (dd, J = 13.2, 3.6 Hz, 1H), 4.48 (s, 1H). 13C NMR (100.6 MHz, CDCl3): δ 170.4, 169.8, 147.1, 136.4, 130.6, 121.8, 118.9, 113.3, 99.7, 76.4, 66.2, 31.6. HRMS (ESI) m/z calcd for C12H11Br2N2O5 [M + NH4]+: 420.9029, found 420.9013. HPLC analysis (Chiralpak AD-H column, hexane
:
2-propanol = 90
:
10, flow rate = 1.0 mL min−1, wavelength = 220 nm): Rt = 20.15 (minor) and 23.63 min (major).
(R)-7-Methyl-9-nitromethyl-3H-furo[3,4-b]chromen-1(9H)-one (3ga)
White solid, mp 212–214 °C, 80% yield, [α]25D + 29.00 (c 1.40, CHCl3), 86% ee. 1H NMR (400 MHz, DMSO-d6): δ 7.38 (s, 1H), 7.19 (d, J = 8.4 Hz, 1H), 7.13 (d, J = 8.4 Hz, 1H), 5.02–5.12 (m, 3H), 4.95 (dd, J = 12.8, 3.6 Hz, 1H), 4.53 (s, 1H), 2.30 (s, 3H). 13C NMR (100.6 MHz, DMSO-d6): δ 171.1, 170.6, 148.4, 135.2, 130.1, 129.6, 118.4, 117.1, 98.4, 77.7, 66.0, 30.7, 20.3. HRMS (ESI) m/z calcd for C13H12NO5 [M + H]+: 262.0710, found 262.0714. HPLC analysis (Chiralpak AD-H column, hexane
:
2-propanol = 85
:
15, flow rate = 1.0 mL min−1, wavelength = 220 nm): Rt = 12.97 (minor) and 14.18 min (major).
(R)-6-Methyl-9-nitromethyl-3H-furo[3,4-b]chromen-1(9H)-one (3ha)
White solid, mp 144–146 °C, 77% yield (46% yield after a single recrystallization), [α]25D + 131.23 (c 1.30, CHCl3), 88% ee (98% ee after a single recrystallization). 1H NMR (400 MHz, DMSO-d6): δ 7.44 (d, J = 8.0 Hz, 1H), 7.11 (d, J = 8.0 Hz, 1H), 7.06 (s, 1H), 5.02–5.13 (m, 3H), 4.93 (dd, J = 12.4, 3.6 Hz, 1H), 4.52 (s, 1H), 2.31 (s, 3H). 13C NMR (100.6 MHz, DMSO-d6): δ 171.1, 170.6, 150.1, 139.6, 129.2, 126.8, 117.5, 115.6, 98.6, 77.7, 66.0, 30.5, 20.4. HRMS (ESI) m/z calcd for C13H12NO5 [M + H]+: 262.0710, found 262.0712. HPLC analysis (Chiralpak AD-H column, hexane
:
2-propanol = 90
:
10, flow rate = 1.0 mL min−1, wavelength = 220 nm): Rt = 21.87 (major) and 23.62 min (minor).
(R)-5-Methyl-9-nitromethyl-3H-furo[3,4-b]chromen-1(9H)-one (3ia)
Pale yellow solid, mp 201–203 °C, 80% yield, [α]25D + 12.60 (c 1.00, CHCl3), 90% ee. 1H NMR (400 MHz, DMSO-d6): δ 7.38 (d, J = 7.6 Hz, 1H), 7.26 (d, J = 7.2 Hz, 1H), 7.18 (t, J = 7.6 Hz, 1H), 5.05–5.16 (m, 3H), 4.95 (dd, J = 12.8, 4.0 Hz, 1H), 4.58 (s, 1H), 2.28 (s, 3H). 13C NMR (100.6 MHz, DMSO-d6): δ 170.9, 170.6, 148.6, 130.9, 127.1, 126.2, 125.3, 118.4, 98.5, 77.9, 66.2, 30.9, 15.5. HRMS (ESI) m/z calcd for C13H12NO5 [M + H]+: 262.0710, found 262.0715. HPLC analysis (Chiralpak OJ-H column, hexane
:
2-propanol = 80
:
20, flow rate = 1.0 mL min−1, wavelength = 220 nm): Rt = 50.05 (major) and 57.38 min (minor).
(R)-7-Methoxy-9-nitromethyl-3H-furo[3,4-b]chromen-1(9H)-one (3ja)
White solid, mp 168–170 °C, 61% yield, [α]25D + 47.23 (c 1.03, CHCl3), 88% ee. 1H NMR (400 MHz, CDCl3): δ 7.07 (d, J = 8.8 Hz, 1H), 6.87 (dd, J = 9.2, 2.8 Hz, 1H), 6.76 (d, J = 2.8 Hz, 1H), 4.81–4.92 (m, 4H), 4.50 (br. s, 1H), 3.80 (s, 3H). 13C NMR (100.6 MHz, CDCl3): δ 171.1, 170.8, 157.5, 144.6, 119.0, 118.8, 115.4, 113.0, 98.1, 76.9, 66.1, 55.7, 31.6. HRMS (ESI) m/z calcd for C13H12NO6 [M + H]+: 278.0659, found 278.0664. HPLC analysis (Chiralpak AD-H column, hexane
:
2-propanol = 70
:
30, flow rate = 1.0 mL min−1, wavelength = 220 nm): Rt = 9.57 (minor) and 10.78 min (major).
(R)-6-Methoxy-9-nitromethyl-3H-furo[3,4-b]chromen-1(9H)-one (3ka)
White solid, mp 158–160 °C, 60% yield, [α]25D − 12.46 (c 0.87, CHCl3), 94% ee. 1H NMR (400 MHz, DMSO-d6): δ 7.47 (d, J = 8.8 Hz, 1H), 6.89 (dd, J = 8.8, 2.4 Hz, 1H), 6.81 (d, J = 2.4 Hz, 1H), 5.03–5.13 (m, 3H), 4.92 (dd, J = 12.4, 3.6 Hz, 1H), 4.50 (s, 1H), 3.78 (s, 3H). 13C NMR (100.6 MHz, DMSO-d6): δ 171.0, 170.5, 159.9, 151.1, 130.2, 112.4, 110.2, 102.5, 99.0, 77.8, 66.0, 55.6, 30.3. HRMS (ESI) m/z calcd for C13H12NO6 [M + H]+: 278.0659, found 278.0660. HPLC analysis (Chiralpak AD-H column, hexane
:
2-propanol = 70
:
30, flow rate = 1.0 mL min−1, wavelength = 220 nm): Rt = 11.13 (major) and 12.31 min (minor).
(R)-5-Methoxy-9-nitromethyl-3H-furo[3,4-b]chromen-1(9H)-one (3la)
White solid, mp 182–184 °C, 63% yield, [α]25D + 56.8 (c 1.10, CHCl3), 91% ee. 1H NMR (400 MHz, DMSO-d6): δ 7.22 (t, J = 8.0 Hz, 1H), 7.10 (d, J = 6.4 Hz, 1H), 7.08 (d, J = 6.4 Hz, 1H), 5.03–5.15 (m, 3H), 4.94 (dd, J = 12.8, 3.6 Hz, 1H), 4.56 (s, 1H), 3.84 (s, 3H). 13C NMR (100.6 MHz, DMSO-d6): δ 170.7, 170.5, 147.9, 139.9, 125.8, 120.2, 119.6, 112.3, 98.5, 77.8, 66.2, 55.8, 30.8. HRMS (ESI) m/z calcd for C13H12NO6 [M + H]+: 278.0659, found 278.0665. HPLC analysis (Chiralpak AD-H column, hexane
:
2-propanol = 70
:
30, flow rate = 1.0 mL min−1, wavelength = 220 nm): Rt = 11.77 (minor) and 13.52 min (major).
(R)-5,7-Di-tert-butyl-9-nitromethyl-3H-furo[3,4-b]chromen-1(9H)-one (3ma)
White solid, mp 213–215 °C, 40% yield, [α]25D + 35.14 (c 0.70, CHCl3), 79% ee. 1H NMR (400 MHz, CDCl3): δ 7.35 (dd, J = 2.4 Hz, 1H), 7.08 (d, J = 2.4 Hz, 1H), 4.96 (d, J = 16.0 Hz, 1H), 4.86 (dd, J = 16.4, 1.6 Hz, 1H), 4.83 (d, J = 4.8 Hz, 2H), 4.54 (t, J = 4.4 Hz, 1H), 1.40 (s, 9H), 1.30 (s, 9H). 13C NMR (100.6 MHz, CDCl3): δ 170.8, 170.36, 148.5, 147.3, 138.1, 124.7, 123.5, 117.6, 98.6, 77.8, 66.1, 35.4, 34.7, 32.0, 31.2, 30.2. HRMS (ESI) m/z calcd for C20H26NO5 [M + H]+: 360.1805, found 360.1808. HPLC analysis (Chiralpak AS-H column, hexane
:
2-propanol = 70
:
30, flow rate = 1.0 mL min−1, wavelength = 220 nm): Rt = 5.58 (minor) and 8.75 min (minor).
(R)-11-Nitromethyl-8H-benzo[f]furo[3,4-b]chromene-10(11H)-one (3na)
Yellow solid, mp 246–248 °C, 27% yield, [α]25D + 70.80 (c 0.57, CHCl3), 88% ee. 1H NMR (400 MHz, CDCl3): δ 7.35 (dd, J = 2.4 Hz, 1H), 7.08 (d, J = 2.4 Hz, 1H), 4.96 (d, J = 16.0 Hz, 1H), 4.86 (dd, J = 16.4, 1.6 Hz, 1H), 4.83 (d, J = 4.8 Hz, 2H), 4.54 (t, J = 4.4 Hz, 1H), 1.40 (s, 9H), 1.30 (s, 9H). 13C NMR (100.6 MHz, CDCl3): δ 171.2, 170.4, 149.1, 131.4, 130.8, 130.4, 129.0, 127.9, 125.8, 123.0, 117.4, 111.5, 99.5, 78.0, 66.1, 29.1. HRMS (ESI) m/z calcd for C16H12NO5 [M + H]+: 298.0710, found 298.0713. HPLC analysis (Chiralpak AD-H column, hexane
:
2-propanol = 92
:
8, flow rate = 1.0 mL min−1, wavelength = 220 nm): Rt = 33.85 (minor) and 36.57 min (major).
(R)-3,3-Dimethyl-9-nitromethyl-3H-furo[3,4-b]chromen-1(9H)-one (3ab)
White solid, mp 150–152 °C, 38% yield, [α]25D + 12.24 (c 0.83, CHCl3), 88% ee. 1H NMR (400 MHz, DMSO-d6): δ 7.59 (d, J = 7.2 Hz, 1H), 7.40 (t, J = 7.6 Hz, 1H), 7.30 (t, J = 7.6 Hz, 1H), 7.25 (d, J = 8.0 Hz, 1H), 5.10 (dd, J = 12.8, 3.6 Hz, 1H), 4.96 (dd, J = 12.8, 3.6 Hz, 1H), 4.58 (t, J = 3.6 Hz, 1H), 1.54 (s, 3H), 1.53 (s, 3H). 13C NMR (100.6 MHz, DMSO-d6): δ 175.3, 168.5, 150.5, 129.6 (2C), 126.0, 118.6, 117.5, 97.2, 80.8, 78.0, 30.7, 23.8, 23.3. HRMS (ESI) m/z calcd for C14H14NO5 [M + H]+: 276.0866, found 276.0869. HPLC analysis (Chiralpak AD-H column, hexane
:
2-propanol = 90
:
10, flow rate = 1.0 mL min−1, wavelength = 220 nm): Rt = 11.81 (minor) and 13.37 min (major).
(9R)-3-Methyl-9-nitromethyl-3H-furo[3,4-b]chromen-1(9H)-one (3ac)
White solid, mp 140–142 °C, 52% yield, [α]25D + 14.10 (c 0.67, CHCl3), 60/40 dr, 92% ee for the major isomer, 98% ee for the minor isomer. 1H NMR (400 MHz, CDCl3): δ 7.29–7.37 (m, 2H), 7.22–7.26 (m, 1H), 7.14–7.17 (m, 1H), 5.04–5.15 (m, 1H), 4.90–4.98 (m, 1H), 4.83–4.88 (m, 1H), 4.49–4.54 (m, 1H), 1.62 (d, J = 6.8 Hz, 1.80H), 1.59 (d, J = 6.8 Hz, 1.20H). 13C NMR (100.6 MHz, CDCl3): δ major isomer: 174.1, 170.0, 150.8, 129.9, 128.8, 126.3, 118.0, 117.9, 98.3, 77.2, 74.1, 31.4, 17.4. Minor isomer: 173.9, 169.9, 150.7, 129.9, 128.7, 126.4, 118.1, 118.0, 98.2, 77.1, 74.0, 31.3, 17.3. HRMS (ESI) m/z calcd for C13H12NO5 [M + H]+: 262.0710, found 262.0713. HPLC analysis (Chiralpak AD-H column, hexane
:
2-propanol = 98
:
2, flow rate = 1.0 mL min−1, wavelength = 220 nm): Rt = 56.55 (minor for the major diastereomer), 61.70 (minor for the minor diastereomer), 65.10 (major for the minor diastereomer) and 69.38 min (major for the major diastereomer).
(3S,9R)-3-Methyl-9-nitromethyl-3H-furo[3,4-b]chromen-1(9H)-one (3ad)
White solid, mp 140–142 °C, 52% yield, [α]25D + 3.50 (c 0.80, CHCl3), 89/11 dr, 98% ee for the major isomer. 1H NMR (400 MHz, CDCl3): δ 7.35 (t, J = 7.6 Hz, 1H), 7.31 (d, J = 7.2 Hz, 1H), 7.24 (t, J = 7.6 Hz, 1H), 7.14 (d, J = 8.0 Hz, 1H), 5.12 (q, J = 6.8 Hz, 0.11H), 5.06 (q, J = 6.8 Hz, 0.89H), 4.96 (dd, J = 12.4, 4.4 Hz, 0.89H), 4.92 (dd, J = 12.4, 4.8 Hz, 0.11H), 4.85 (dd, J = 12.4, 3.6 Hz, 1H), 4.50 (s, 1H), 1.62 (d, J = 6.8 Hz, 2.67H), 1.58 (d, J = 6.8 Hz, 0.33H). 13C NMR (100.6 MHz, CDCl3): δ major isomer: 174.1, 170.0, 150.8, 129.9, 128.8, 126.3, 118.0, 117.9, 98.3, 77.2, 74.1, 31.4, 17.4. Minor isomer: 173.9, 169.9, 150.7, 129.9, 128.7, 126.4, 118.1, 118.0, 98.2, 77.1, 74.0, 31.3, 17.3. HPLC analysis (Chiralpak AD-H column, hexane
:
2-propanol = 98
:
2, flow rate = 1.0 mL min−1, wavelength = 220 nm): Rt = 55.32 (minor for the major diastereomer), 60.09 (major for the minor diastereomer), 63.49 (minor for the minor diastereomer) and 68.07 min (major for the major diastereomer).
(3R,9R)-3-Methyl-9-nitromethyl-3H-furo[3,4-b]chromen-1(9H)-one (3ae)
White solid, mp 140–142 °C, 52% yield, [α]25D + 28.11 (c 1.17, CHCl3), 83/17 dr, >99% ee for the major isomer. 1H NMR (400 MHz, CDCl3): δ 7.35 (t, J = 8.0 Hz, 1H), 7.30 (d, J = 7.2 Hz, 1H), 7.24 (t, J = 7.6 Hz, 1H), 7.14 (d, J = 8.0 Hz, 1H), 5.12 (q, J = 6.8 Hz, 0.83H), 5.06 (q, J = 6.8 Hz, 0.17H), 4.96 (dd, J = 12.4, 4.4 Hz, 0.17H), 4.92 (dd, J = 12.4, 4.8 Hz, 0.83H), 4.86 (dd, J = 12.4, 3.6 Hz, 1H), 4.53 (t, J = 4.4 Hz, 1H), 1.62 (d, J = 6.8 Hz, 0.51H), 1.58 (d, J = 6.8 Hz, 2.49H). 13C NMR (100.6 MHz, CDCl3): δ minor isomer: 174.1, 170.0, 150.8, 129.9, 128.8, 126.3, 118.0, 117.9, 98.3, 77.2, 74.1, 31.4, 17.4. Minor isomer: 173.9, 169.9, 150.7, 129.9, 128.7, 126.4, 118.1, 118.0, 98.2, 77.1, 74.0, 31.3, 17.3. HPLC analysis (Chiralpak AD-H column, hexane
:
2-propanol = 98
:
2, flow rate = 1.0 mL min−1, wavelength = 220 nm): Rt = 57.13 (minor for the minor diastereomer), 65.83 (major for the major diastereomer) and 69.38 min (major for the minor diastereomer).
Acknowledgements
We are grateful to the National Naturaaience Foundation of China (No. 21421062), the Key laboratory of Elemento-Organic Chemistry and Collaborative Innovation Center of Chemical Science and Engineering for generous financial support for our programs.
Notes and references
-
(a) M. Kidwai, S. Saxena, M. K. R. Khan and S. S. Thukral, Bioorg. Med. Chem. Lett., 2005, 15, 4295 CrossRef CAS PubMed;
(b) D. Kumar, V. B. Reddy, S. Sharad, U. Dube and S. Kapur, Eur. J. Med. Chem., 2009, 44, 3805 CrossRef CAS PubMed;
(c) S. Limsuwan, E. N. Trip, T. R. H. M. Kouwen, S. Piersma, A. Hiranrat, W. Mahabusarakam, S. P. Voravuthikunchai, J. M. van Dijl and O. Kayser, Phytomedicine, 2009, 16, 645 CrossRef CAS PubMed;
(d) K. C. Nicolaou, A. J. Roecker, S. Barluenga, J. A. Pfefferkorn and G. Q. Cao, ChemBioChem, 2001, 2, 460 CrossRef CAS.
-
(a) A. M. Shestopalov, Y. M. Litvinov, L. A. Rodinovskaya, O. R. Malyshev, M. N. Semenova and V. V. Semenov, ACS Comb. Sci., 2012, 14, 484 CrossRef CAS PubMed;
(b) J. M. Doshi, D. Tian and C. Xing, J. Med. Chem., 2006, 49, 7731 CrossRef CAS PubMed;
(c) J. Skommer, D. Wlodkowic, M. Mättö, M. Eray and J. Pelkonen, Leuk. Res., 2006, 30, 322 CrossRef CAS PubMed;
(d) J.-L. Wang, D. Liu, Z.-J. Zhang, S. Shan, X. Han, S. M. Srinivasula, C. M. Croce, E. S. Alnemri and Z. Huang, Proc. Natl. Acad. Sci. U. S. A., 2000, 97, 7124 CrossRef CAS;
(e) N. Yu, J. M. Aramini, M. W. Germann and Z. Huang, Tetrahedron Lett., 2000, 41, 6993 CrossRef CAS.
- L. Bonsignore, G. Loy, D. Secci and A. Calignano, Eur. J. Med. Chem., 1993, 28, 517 CrossRef CAS.
- J. A. Bristol, E. H. Gold, I. Gross and R. G. Lovey, J. Med. Chem., 1981, 24, 1010 CrossRef CAS.
-
(a) W. Kemnitzer, S. Jiang, Y. Wang, S. Kasibhatla, C. Crogan-Grundy, M. Bubenik, D. Labrecque, R. Denis, S. Lamothe, G. Attardo, H. Gourdeau, B. Tseng, J. Drewea and S. X. Cai, Bioorg. Med. Chem. Lett., 2008, 18, 603 CrossRef CAS PubMed;
(b) W. Kemnitzer, J. Drewe, S. Jiang, H. Zhang, C. Crogan-Grundy, D. Labreque, M. Bubenick, G. Attardo, R. Denis, S. Lamothe, H. Gourdeau, B. Tseng, S. Kasibhatla and S. X. Cai, J. Med. Chem., 2008, 51, 417 CrossRef CAS PubMed.
- N. Foloppe, L. M. Fisher, R. Howes, A. Potter, A. G. S. Robertson and A. E. Surgenor, Bioorg. Med. Chem., 2006, 14, 4792 CrossRef CAS PubMed.
-
(a) M. N. Semenova, D. V. Tsyganov, O. R. Malyshev, O. V. Ershov, I. N. Bardasov, R. V. Semenov, A. S. Kiselyov and V. V. Semenov, Bioorg. Med. Chem. Lett., 2014, 24, 3914 CrossRef CAS PubMed;
(b) C. Da, S. L. Mooberry, J. T. Gupton and G. E. Kellogg, J. Med. Chem., 2013, 56, 7382 CrossRef CAS PubMed;
(c) N. B. Chernysheva, D. V. Tsyganov, A. A. Philchenkov, M. P. Zavelevich, A. S. Kiselyov, R. V. Semenov, M. N. Semenova and V. V. Semenov, Bioorg. Med. Chem. Lett., 2012, 22, 2590 CrossRef CAS PubMed;
(d) J. Frackenpohl, I. Adelt, H. Antonicek, C. Arnold, P. Behrmann, N. Blaha, J. Böhmer, O. Gutbrod, R. Hanke, S. Hohmann, M. van Houtdreve, P. Lösel, O. Malsam, M. Melchers, V. Neufert, E. Peschel, U. Reckmann, T. Schenke, H.-P. Thiesen, R. Velten, K. Vogelsang and H.-C. Weiss, Bioorg. Med. Chem., 2009, 17, 4160 CrossRef CAS PubMed;
(e) L. Jurd, J. Heterocycl. Chem., 1997, 34, 601 CrossRef CAS PubMed;
(f) L. Jurd, J. Heterocycl. Chem., 1996, 33, 1227 CrossRef CAS PubMed.
- V. Farina, J. T. Reeves, C. H. Sesanayake and J. J. Song, Chem. Rev., 2006, 106, 2734 CrossRef CAS PubMed.
-
(a) H. Wang, L. Wu, Y. Wang and Z. Zhou, RSC Adv., 2015, 5, 42836 RSC;
(b) Y. Liu, Q. Wang, Y. Wang, H. Song and Z. Zhou, ChemCatChem, 2014, 6, 2298 CrossRef CAS PubMed;
(c) L. Wu, Y. Wang, H. Song, L. Tang, Z. Zhou and C. Tang, ChemCatChem, 2014, 6, 649 CrossRef CAS PubMed;
(d) S. Chen, J. Pan, Y. Wang and Z. Zhou, Eur. J. Org. Chem., 2014, 7940 CrossRef CAS PubMed;
(e) K. Hu, Y. Wang, Z. Zhou and C. Tang, Tetrahedron, 2014, 70, 181 CrossRef CAS PubMed;
(f) L. Wu, Y. Wang, H. Song, L. Tang, Z. Zhou and C. Tang, Chem.–Asian J., 2013, 8, 2204 CrossRef CAS PubMed;
(g) L. Wu, Y. Wang, H. Song, L. Tang, Z. Zhou and C. Tang, Adv. Synth. Catal., 2013, 355, 1053 CrossRef CAS PubMed;
(h) Y. Liu, A. Lu, K. Hu, Y. Wang, H. Song, Z. Zhou and C. Tang, Eur. J. Org. Chem., 2013, 4836 CrossRef CAS PubMed;
(i) A. Lu, K. Hu, Y. Wang, H. Song, Z. Zhou, J. Fang and C. Tang, J. Org. Chem., 2012, 77, 6208 CrossRef CAS PubMed.
- Synthesis of chiral tetronic acid derivatives via organocatalytic conjugate addition of ethyl 4-chloro-3-oxobutanoate to nitroalkenes, see, Y.-Y. Yan, R.-J. Lu, J.-J. Wang, Y.-N. Xuan and M. Yan, Tetrahedron, 2012, 68, 6123 CrossRef CAS PubMed Synthesis of functionalized 4H-chromenes via a one-pot domino
Michael-hemiacetalization and dehydration sequence, see: D. Enders, G. Urbanietz and G. Raabe, Synthesis, 2011, 1905 CrossRef Synthesis of functionalized 1,4-hydroquinolines via one-pot organocatalyzed Michael addition and aza-cyclization/dehydration cascade reaction strategy, see: H. Kim and S.-G. Kim, Tetrahedron Lett., 2015, 56, 4819 CrossRef PubMed. - For reviews on thiourea-based organocatalysis, see:
(a) W.-Y. Siau and J. Wang, Catal. Sci. Technol., 2011, 1, 1298 RSC;
(b) B. Han, J.-L. Li, Y.-C. Xiao, S.-L. Zhou and Y.-C. Chen, Curr. Org. Chem., 2011, 15, 4128 CrossRef CAS;
(c) Y. Takemoto, Chem. Pharm. Bull., 2010, 58, 593 CrossRef CAS;
(d) S. J. Connon, Chem.–Eur. J., 2006, 12, 5418 CrossRef PubMed.
- For a review of squaramide-based organocatalysis, see: J. Alemán, A. Parra, H. Jiang and K. A. Jørgensen, Chem.–Eur. J., 2011, 17, 6890 CrossRef PubMed.
-
(a) T.-Y. Liu, H.-L. Cui, Q. Chai, J. Long, B.-J. Li, Y. Wu, L.-S. Ding and Y.-C. Chen, Chem. Commun., 2007, 2228 RSC;
(b) Y. Sohtome, B. Shin, N. Horitsugi, R. Takagi, K. Noguchi and K. Nagasawa, Angew. Chem., Int. Ed., 2010, 49, 7299 CrossRef CAS PubMed;
(c) Y. Sohtome, B. Shin, N. Horitsugi, K. Noguchi and K. Nagasawa, Chem.–Asian J., 2011, 6, 2463 CrossRef CAS PubMed;
(d) Y. Sohtome, T. Yamaguchi, B. Shin and K. Nagasawa, Chem. Lett., 2011, 40, 843 CrossRef CAS.
Footnote |
† Electronic supplementary information (ESI) available. CCDC 1421230. For ESI and crystallographic data in CIF or other electronic format see DOI: 10.1039/c5ra17503h |
|
This journal is © The Royal Society of Chemistry 2015 |
Click here to see how this site uses Cookies. View our privacy policy here.