DOI:
10.1039/C5RA16447H
(Paper)
RSC Adv., 2015,
5, 83295-83303
Bis(phenolate) N-heterocyclic carbene rare earth metal complexes: synthesis, characterization and applications in the polymerization of n-hexyl isocyanate†
Received
15th August 2015
, Accepted 24th September 2015
First published on 25th September 2015
Abstract
The rare earth complexes [Ln(L)2K(thf)] bearing a bis(phenolate) N-heterocyclic carbene (NHC) ligand (L = 1,3-bis[O-4,6-di-tBu-C6H2-2-CH2][C(NCH2CH2CH2N)], Ln = Nd (1), Y (2)) were synthesized in situ by the reaction of L1 with KN(SiMe3)2 and Ln[N(SiMe3)2]. The bis(phenolate) NHC precursor L1 also coordinated with rare earth metal forming bis(phenolate) pyrimidinium-bridged rare earth metal complexes [ Ln2(L1)3] (L1 = 1,3-bis[O-4,6-di-tBu-C6H2-2-CH2][CH(NCH2CH2CH2N)]+Cl−, Ln = Sm (3), Y (4)). Very high molecular weight (up to 106) and narrow molecular weight distribution (Mw/Mn = 1.7–2.3) polyhexyl isocyanate could be obtained by using the pyrimidinium based NHC rare earth metal complexes 1, 2 as well as imidazolinium based NHC rare earth complexes 5–7. The NHC complexes were found to be highly active towards the polymerization of n-hexyl isocyanate whereas non-NHC rare earth metal complexes 3, 4, 8 were inactive. The radius of rare earth metal, solvent, polymerization temperature and the structure of the ligand greatly affected the catalytic activity of polymerization. The mechanism of the initiation of the polymerization was studied and the NHC moiety played an important role in the initiation step which was evidenced via NMR analysis.
Introduction
N-Heterocyclic carbene (NHC) ligands have been successfully applied in transition-metal complexes.1 Recently, transition-metal complexes supported by bidentate or tridentate phenolate NHC ligands have attracted attention.2 Bis(phenolate) ligands as dianionic ligands have the advantages of avoiding ligand distribution reactions and helping to reduce the tendency for NHC ligand dissociation by chelating effects.2f,2p,3 Backbone fully saturated NHCs have the effect of increasing the donor ability since they do not allow delocalization of the charge beyond the NCN region and the pyrimidinium based NHC would be more basic with high donor capability.4 Despite the fact that a few articles about NHC rare earth metal complexes have been reported,1h,5 backbone saturated NHCs have rarely been applied in rare earth metal chemistry. To our best knowledge, rare earth metal complexes bearing the backbone saturated pyrimidinium based NHCs have not been reported. Lanthanide phenolate complexes have also received considerable attention for their chemical stability, easy availability, etc., and have been applied in various reactions and polymerizations.6 Rare earth metal complexes supported by this tridentate pincer ligand of bis(phenolate) NHC may be interesting.
Polyisocyanates which form dynamic helical conformations have been much concerned due to their liquid crystalline properties, stiff-chain solution characteristics and induced optical activities, and the polymer-based materials are good candidates for optical switches and recognition devices.7 Polyisocyanates could be synthesized through anionic polymerizations which proceeded at the optimum temperature between −50 and −70 °C using NaCN as the initiator.8 The living anionic polymerization of n-hexyl isocyanate was achieved using Na-Naph initiators in the presence of additives such as crown ethers or NaBPh4 and performed at −98 °C.9 Na-BH as an initiator and an additive could also achieve the living anionic polymerization of n-hexyl isocyanate at −98 °C.10 Besides anionic polymerizations, transition metal complexes and rare earth metal complexes could also initiate the polymerization of isocyanates. Alkoxytitanium initiators TiCl3(OCH2CF3) and TiCl2(C5H5)(OCH2CF3) created by Novak group could polymerize alkyl isocyanates at room temperature in a “living” feature and gave polymers with narrow molecular weight distribution.7d,11 The polymerization of n-hexyl isocyanate initiated by La(C5Me5)2[CH(SiMe3)]2 at −20 °C led to high molecular weight (up to 5.9 × 105) poly(hexyl isocyanate) in moderate yields.12 Lanthanum isopropoxide as anionic initiator for the polymerization of hexyl isocyanate afforded very high molecular weight (Mn > 106) and narrow molecular weight distribution (Mw/Mn = 2.08–3.16) at low temperature (−78 °C).7a Rare earth metal complexes have exhibited good activity and relatively mild polymerization conditions toward the polymerization of isocyanates in contrast to highly sensitive, low-temperature, anionic isocyanate polymerizations.7a,13
Here we report the synthesis and characterization of pyrimidinium based N-heterocyclic carbene rare earth metal complexes as well as the comparison of activities with imidazolinium based NHC counterparts toward the polymerization of n-hexyl isocyanate. The mechanism of the initiation step of the polymerization was also studied by NMR analysis.
Results and discussion
Synthesis and characterization of complexes 1–4
The ligand used in this work was designed and synthesized easily. N,N′-bis(3,5-di-tert-2-hydroxyphenylmethyl)propylenediimine was obtained by the reaction of 3,5-di-tert-butylsalicylaldehyde with 1,2-propanediamine in ethanol at reflux temperature, and then reduced by NaBH4 to give the corresponding diamine.14 Finally, the bis(phenolate) NHC precursor, N,N′-bis(3,5-di-tert-butyl-2-hydroxyphenylmethyl)pyrimidinium chloride (L1) was synthesized by treatment of diamine with HCl and CH(OEt)3 at reflux temperature (Scheme 1).
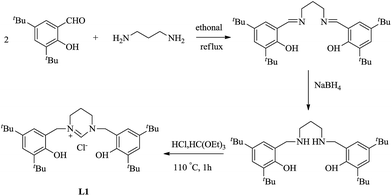 |
| Scheme 1 Synthesis of L1. | |
Complex 1 was synthesized by treatment of L1 with 1 equiv. of KN(SiMe3)2 and half equiv. of Nd[N(SiMe3)2] in THF at −30 °C (Scheme 2). The ORTEP diagram is depicted in Fig. 1 with the selected bond lengths and angles. The ligand coordinates to Nd in a tridentate mode, with two oxygens on the aryloxo groups and Ccarbene on the six-membered ring.
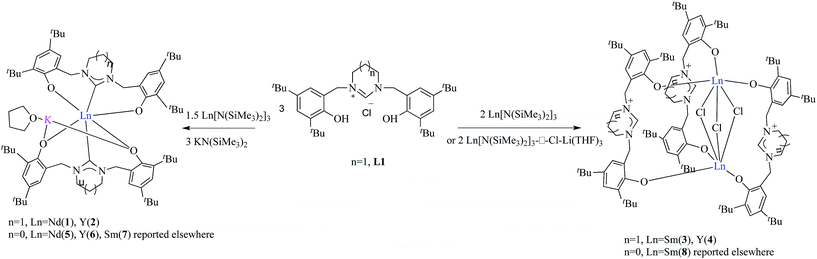 |
| Scheme 2 Synthesis of NHC rare earth metal complexes 1–4. | |
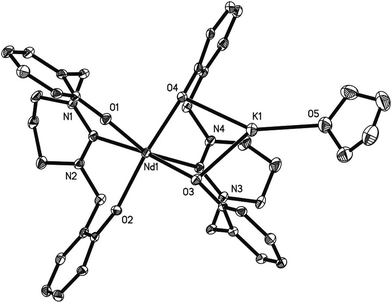 |
| Fig. 1 X-ray structure of complex 1, with 30% probability thermal ellipsoids. Hydrogen atoms and tert-butyl groups are omitted for clarity. Selected bond distances (Å) and angles (°) of 1: Nd1–O1 2.226(2), Nd1–O2 2.267(2), Nd1–O3 2.315(2), Nd1–O4 2.339(2), Nd1–C11 2.750(4), Nd1–C45 2.767(3), K1–O3 2.727(2), K1–O4 2.766(2), K1–O5 2.696(3), N1–C11 1.346(4), N2–C11 1.335(4); O1–Nd1–O2 102.87(8), O3–Nd1–O4 89.20(8), C11–Nd1–C45 88.99(10), O1–Nd1–C11 82.06(9), O2–Nd1–C11 80.13(9), O3–Nd1–C45 77.43(9), O4–Nd1–C45 73.23(9), O3–K1–O4 73.01(7), O3–K1–O5 128.47(8), O4–K1–O5 151.82(8), N1–C11–N2 116.3(3). | |
The geometry of the potassium atom is planar with two oxygens on one ligand and one oxygen on THF molecule. The central metal Nd is six-coordinated by the geometry of distorted octahedron, with O1, O2, O4 and C45 (linking to N3 and N4) occupying the equatorial positions and O3, C11 occupying the axial positions of the octahedron. The similar structure of imidazolinium based NHC complexes has been reported by us and here we employ the complexes for comparison (Scheme 2, complexes 5–8).5j The average bond length of Nd–Ccarbene 2.759 Å falls in the range of the published literatures,5g,5h,5j,15 slightly longer than that of the imidazolinium based NHC Nd complex (2.716 Å). The bond angle of N–Ccarbene–N increases from 107.2° of imidazolinium based NHC to 116.3° of pyrimidinium based NHC complex 1.
The average bond length of the Nd–O on the ligand which the oxygens link to the potassium atom is 2.326 Å, longer than the Nd–O distance (2.246 Å) on the other ligand. The average bond length of K–O is 2.729 Å. The tridentate ligand bends to half a circle when coordinated to the metal center and the ligand without the binding force of potassium opens wider with the bond angle of O1–Nd1–O2 at 102.9° in contrast with the 89.2° of O3–Nd–O4.
Complex 2 was synthesized in a similar way. Suitable crystals for X-ray diffraction were not obtained. NMR analysis could not be performed on Nd complex 1 due to the paramagnetic properties and complex 2 was characterized by 1H(13C) NMR analysis. The chemical shift of the 1H NMR spectrum moved downfield towards the ligand with potassium ion due to the electron-withdrawing effect of the alkali metals. The methene hydrogen [CH2-Ar] linking the phenyl ring on the ligand with potassium ion showed high chemical shift at δ 5.4–6.3 in comparison with that of δ 2.8–3.8 the counterpart at the other ligand. The formation of Y–Ccarbene bond could be observed obviously by 1H(13C) NMR spectra. The resonance of the hydrogen on the six-membered ring (C2) was at δ 8.72 in CDCl3 and the resonance peak diminished after deprotonation by KN(SiMe3)2 and coordination with yttrium. The high Ccarbene resonance appeared at δ 211.37 and 211.99. Complexes 1–2 were soluble in THF and toluene and partially soluble in hexane.
Complex 3 was synthesized by treatment of a stirring solution of L1 with Sm[N(SiMe3)2]3 or Sm[N(SiMe3)2]3-μ-Cl-Li(THF)3 in THF at room temperature for 1 day. Complex 3 was soluble in THF and had a poor solubility in toluene and hexane. Without the addition of strong base KN(SiMe3)2, the hydrogen on the NHC ring of the NHC precursor could not be abstracted. The two Sm metal centers were also bridged by the three chloride ions from the ligand and forming the pyrimidinium-bridged rare earth metal complex. The solid structures of complex 3 were detected by single-crystal X-ray diffraction analysis. ORTEP drawing, bond lengths and angles are compiled in Fig. 2. Three ligands coordinate to two metal centers in a bidentate mode. The geometry at the metal center Sm is best described as a trigonal antiprism, with three oxygens from the three ligands on one plane and three chlorides on the other plane. On the bridged six-membered ring, the bond angle of N1–C11–N2 is 124.7°, larger than the 116.3° of complex 1, and larger than the 114.7° of imidazolinium based NHC complex 5. In complex 3, the bond angles of Sm1–Cl–Sm2 are between 88.8–89.8°, slightly smaller than the 90.9–91.6° in imidazolinium based NHC Sm complex 8;5j the sum of Cl–Sm1–Cl is 227.9°, larger than that of 223.9° in complex and shows a more contact structure in complex 3. The hydrogens (NCHN, NCHH-Ar) have some interactions with chlorides. C–H⋯Cl hydrogen bond lengths ranges from 2.64 to 2.83 Å with the bond angles of C–H⋯Cl ranges from 137.6 to 158.1° in the solid structure, showing some characteristics of the C–H⋯Cl interactions.16 Complex 4 was synthesized by the same method with complex 3, using Y[N(SiMe3)2]3 instead. 1H(13C) NMR analysis was performed on complex 4 in THF-d8. The 1H NMR spectrum shows that the resonances of a half hydrogens on the methene (NCHH-Ar) occur at δ 6.47, 6.50 due to the C–H⋯Cl interaction, and the methine H on the ring (NCHN) occurs at δ 9.63. The resonance of the other half hydrogens on the methene (NCHH-Ar) which do not participate in the C–H⋯Cl interaction occur at δ 3.57, 3.59. The 13C NMR spectrum shows that the resonance of the methine carbon (NCHN) occurs at δ 153.41.
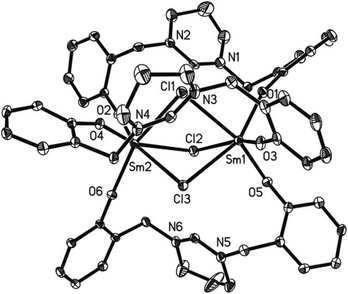 |
| Fig. 2 X-ray structure of complex 3, with 30% probability thermal ellipsoids. Hydrogen atoms and tert-butyl groups are omitted for clarity. Selected bond distances (Å) and angles (°) of 3: Sm1–O3 2.183(3), Sm1–O5 2.199(3), Sm1–O1 2.199(3), Sm1–Cl1 2.851(11), Sm1–Cl2 2.858(11), Sm1–Cl3 2.872(11), N1–C11 1.312(6), N2–C11 1.306(6), O3–Sm1–O5 102.86(11), O3–Sm1–O1 101.60(11), O5–Sm1–O1 104.59(11), O3–Sm1–Cl1 96.70(8), O5–Sm1–Cl1 157.89(8), O1–Sm1–Cl1 81.13(8), O3–Sm1–Cl2 158.49(9), O5–Sm1–Cl2 81.38(8), O1–Sm1–Cl2 97.58(8), Cl1–Sm1–Cl2 76.66(3), O3–Sm1–Cl3 83.02(9), O5–Sm1–Cl3 96.38(8), O1–Sm1–Cl3 156.76(8), Cl1–Sm1–Cl3 75.70(3), Cl2–Sm1–Cl3 75.53(3), Sm2–Cl1–Sm1 89.80(3), Sm2–Cl2–Sm1 89.52(3), Sm1–Cl3–Sm2 88.80(3), N2–C11–N1 124.7(4). | |
All the complexes are air and moisture sensitive.
Polymerization of n-hexyl isocyanate
The relationship of structure and catalytic activity of these rare earth metal complexes were studied and the polymerization data were listed in Table 1. All of the polyisocyanates obtained showed very high molecular weight (up to 106) with narrow molecular weight distribution (Mw/Mn = 1.7–2.3) when using toluene as the solvent and the yield could exceed 60%. The pyrimidinium based NHC rare earth complexes 1–2 and imidazolinium based NHC rare earth complexes 5–7 exhibited high activities toward the polymerization of n-hexyl isocyanate in comparatively mild conditions compared with that of the anionic polymerizations. However, non-NHC rare earth complexes 3, 4, 8 showed no activity, which probably suggested the NHC moiety played an important role in the polymerization. The polymerization rate was very fast with considerable viscosity of the solution upon the addition of the catalyst. Thus, adequate toluene solvent was considered to slow down the polymerization process for the sake of a well dispersion of the complexes in the polymerization system as well as a better control of the polymerization and an easier comparison of the structure and activity among the complexes. Complex 5 was employed to optimize the polymerization condition of n-hexyl isocyanate. Polymerization temperature exerted great influence on the activity and lower temperature facilitated the polymerization with −20 °C giving the highest polymerization yield (entry 11). Polymerization of n-hexyl isocyanate could be performed at 0 °C with relatively low yield as was observed in entry 9.
Table 1 Polymerization of n-hexyl isocyanate catalyzed by complex 1–2, 5–7a
Entry |
Complex |
[I]0/[M]0 |
Temp. (°C) |
t (min) |
Yieldb |
10−4 Mnc |
Mw/Mnc |
General polymerization conditions: toluene as the solvent, [n-hexyl isocyanate] = 1 M. Yield: (weight of polymer obtained)/(weight of monomer used). Measured by GPC relative to polystyrene standards. [n-Hexyl isocyanate] = 10 M, toluene as the solvent. Polymerized in THF. |
1 |
1 |
1 : 700 |
−20 |
1 |
28 |
58 |
2.21 |
2 |
1 |
1 : 700 |
−20 |
5 |
57 |
59 |
2.20 |
3 |
1 |
1 : 700 |
−20 |
10 |
60 |
62 |
2.21 |
4 |
1 |
1 : 700 |
−20 |
30 |
64 |
69 |
2.23 |
5d |
1 |
1 : 700 |
−20 |
30 |
63 |
49 |
2.32 |
6e |
1 |
1 : 700 |
−20 |
15 |
9 |
35 |
2.50 |
7 |
2 |
1 : 700 |
−20 |
360 |
8 |
97 |
1.91 |
8d |
2 |
1 : 700 |
−20 |
5 |
15 |
83 |
1.85 |
9 |
5 |
1 : 700 |
0 |
30 |
18 |
80 |
1.80 |
10 |
5 |
1 : 700 |
−10 |
30 |
45 |
92 |
1.76 |
11 |
5 |
1 : 700 |
−20 |
30 |
64 |
99 |
1.72 |
12 |
5 |
1 : 700 |
−30 |
30 |
59 |
97 |
1.71 |
13 |
5 |
1 : 700 |
−20 |
5 |
33 |
74 |
1.84 |
14 |
5 |
1 : 700 |
−20 |
15 |
48 |
98 |
1.71 |
15 |
5 |
1 : 700 |
−20 |
120 |
60 |
98 |
1.77 |
16 |
5 |
1 : 500 |
−20 |
30 |
63 |
78 |
1.89 |
17 |
5 |
1 : 1000 |
−20 |
30 |
41 |
106 |
1.84 |
18 |
6 |
1 : 700 |
−20 |
120 |
10 |
93 |
1.84 |
19 |
6 |
1 : 700 |
−20 |
360 |
18 |
92 |
1.90 |
20 |
7 |
1 : 700 |
−20 |
30 |
34 |
102 |
1.80 |
21 |
7 |
1 : 700 |
−20 |
60 |
59 |
101 |
1.78 |
22 |
7 |
1 : 700 |
−20 |
120 |
62 |
97 |
1.83 |
The polymerization of isocyanate displayed celling temperature effects and monomer to trimer transformation might exist which decreased the yield.7d The monomer/complex ratio could rise to 1000 with the yield exceeding 40% (entry 17). The polymerization yield could reach 57% in 5 minutes catalyzed by the pyrimidinium based NHC complex 1 (entry 2), exhibiting a higher catalytic activity than the imidazolinium based one which might indicate the higher activity of the pyrimidinium based NHC and the easier breakage of the rare earth metal carbene bond. However, the polymers obtained by complex 1 gave lower molecular weights and comparatively wider molecular weight distributions. The activity of the polymerization decreased greatly using the polar solvent THF instead which could be reasonably explained by the completion of the THF molecule with monomer for coordination at the high oxophilic affinitive metal center (entry 6).17
The catalytic activities of the yttrium complexes were obviously decreased due to the steric effect caused by the much smaller radius of the metal and the pyrimidinium based NHC showed much steric hindrance and reduced the activity. However, the yield could reach 15% in 5 minutes in a higher monomer concentration catalyzed by the yttrium complex 2 (entry 8). For the imidazolinium based NHC Sm complex, the catalytic activity was high but lower than that of complex 5. The yield could exceed 60% within 2 hours by both Nd complexes and small radius Sm complex. The magnitude of the activity of different rare earth metal complexes was in line with the radius of the rare earth metal (Nd > Sm > Y). Complexes with suitable metal radius provided enough room for the coordination of the monomers at the metal center.
Previously, lanthanum isopropoxide7a and phenolate rare earth metal complexes18 were found active for the polymerization of n-hexyl isocyanate. Surprised by the inactivity of the bis(phenolate) non-NHC rare earth metal complexes toward the polymerization of n-hexyl isocyanate which suggested the Ln–O bond could not catalyze the polymerization, we further researched the mechanism of the initiation of polymerization by the NHC complexes. The initiation step of the polymerization by the NHC complex 2 was monitored by NMR analysis. The 1H NMR spectrum was shown in Fig. 3 and the ligand with potassium ion was omitted for clarity. The electron-withdrawing effect of potassium ion could be seen by chemical shifts of hydrogen on the ligands, so the potassium ion linked to the second ligand all the time. The function of NHC moiety in the initiation was observed by the in situ 1H NMR analysis in abstracting the one hydrogen after the bond breakage of the yttrium–NHC. The most striking evidence of the hydrogen abstraction of NHC was the hydrogen Ha found at δ 8.78 on the 1H NMR spectrum. Meanwhile, the high resonance of the Ccarbene that was supposed to appear at δ 211.68 and 216.89 in toluene-d8 disappeared on the 13C NMR spectrum (Fig. S11†). This pattern of initiation was rare, but can also be found in the initiation of L-lactide polymerization by the imidazolinium based NHC complexes 5–7 in which the free NHC abstracted the methine hydrogen of L-lactide.5j For the convenience of judgment of the signals of the hydrogen and carbon on the spectra, two online 1H(13C) NMR spectra were performed with one of complex nearly all participated in the initiation and the other of complex a small amount participated. The two-dimensional NMR spectra 1H-1H COSY and 1H-13C HSQC were also performed on the polymerization system with a small amount of complex participated in the initiation (Fig. 4 and see ESI, Fig. S12–14†).
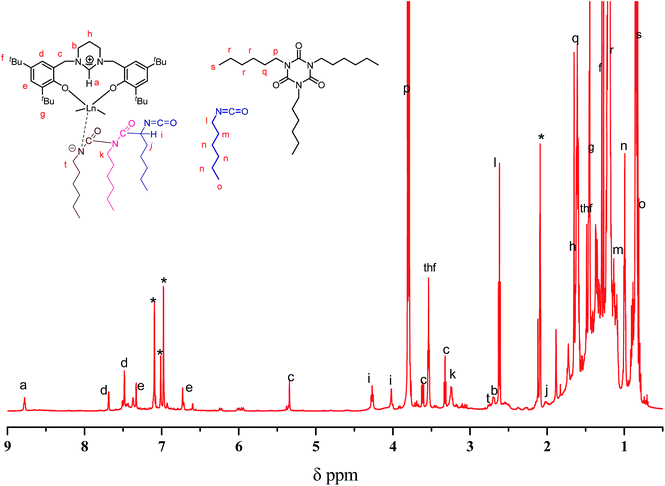 |
| Fig. 3 1H NMR spectrum of in situ polymerization of n-hexyl isocyanate initiated by complex 2 in toluene-d8 (*toluene, the ligand with K was omitted for clarity). | |
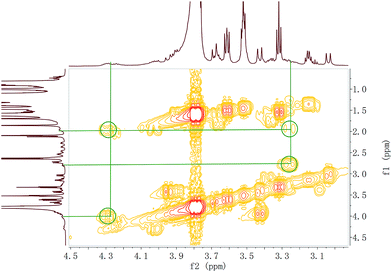 |
| Fig. 4 1H-1H COSY spectrum of in situ polymerization of n-hexyl isocyanate initiated by complex 2 in toluene-d8. | |
N-Hexyl isocyanate was thought to provide the hydrogen resource. After hydrogen-abstraction, the hydrogen left Hi on the chiral carbon occurred at δ 4.27, 4.02 and the methene signals were coupled with protons Hj (δ 1.97) on adjacent carbon (Fig. 4). The signals of the chiral carbon on the 13C NMR spectrum were at δ 45.62, 44.87 (see details of comparison in ESI, Fig. S10 and 11† and 1H–13C HSQC Fig. S14†). The signal at δ 3.25 was assigned to Hk, which was coupled with Ht and Hj on the 1H-1H COSY spectrum. The proposed mechanism was shown in Scheme 3, and the second ligand with potassium ion of the complex was omitted for clarity. The integration of abstracted hydrogen is in proportion (1
:
4) to that of the hydrogen on the phenyl, so both the ligands participated in the initiation. After abstraction, the carbanion was thought to be the initiating species in the polymerization. The polymerization was terminated by adding excess methanol.
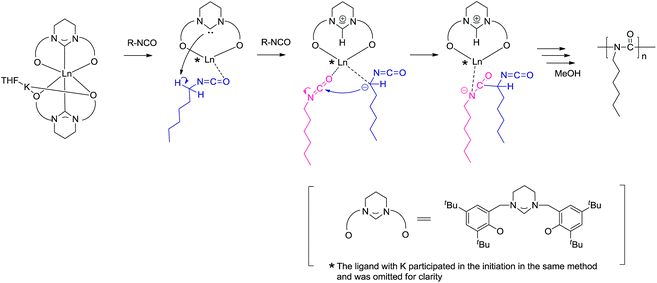 |
| Scheme 3 The proposed mechanism of the initiation by NHC rare earth metal complexes. | |
Small amount of n-hexyl isocyanate was found compared with the 1H NMR spectrum of the n-hexyl isocyanate in toluene-d8 (see ESI, Fig. S7†). The conversion of n-hexyl isocyanate to cyclic trimer as a result of back-biting reaction was thought to decrease the yield of polymerization. A large amount of trimers existed in this system.13h,19 Some remaining unreacted NHC yttrium complex existed in this system and could be identified.
Conclusions
The bis(phenolate) pyrimidinium based NHC rare earth metal complexes and bis(phenolate) pyrimidinium-bridged rare earth metal complexes were successfully synthesized and characterized by X-ray diffraction analysis, 1H(13C) NMR analysis and elemental analysis. The imidazolinium and pyrimidinium based NHC rare earth metal complexes had been applied in the polymerization of n-hexyl isocyanate. The polymer obtained showed very high molecular weight and narrow molecular weight distribution. The catalytic activity was much affected by the radius of rare earth metal, solvent, polymerization temperature and the structure of the ligand. The pyrimidinium-bridged rare earth metal complexes were inactivity for the polymerization. The mechanism of the initiation by NHC rare earth metal complexes was studied, and NHC moiety played an important role in the initiation step, which was evidenced by NMR analysis.
Experimental section
General procedures
The syntheses of rare earth metal complexes and polymerization were carried out under purified argon atmosphere using Schlenk techniques. Hexane, THF and toluene were distilled from sodium benzophenone ketyl before use. All other reagents and solvents were commercially available. HN(TMS)2 was dried over CaH2 and distilled under vacuum. n-Hexyl isocyanate was dried over CaH2 and distilled under vacuum over three times. Metal analyses were performed by complexometric titration. NMR spectra were recorded on an Agilent 600 MHz Direct Drive 2 spectrometer. Elemental analyses were performed by direct combustion with a Flash EA-1112 instrument. Molecular weight and molecular weight distribution were measured by GPC equipped with a RI (Waters 2414) detector and a Waters 1525 isocratic high performance liquid chromatography pump at 40 °C with THF as the eluent at a flow rate of 1.0 mL min−1. The starting materials of Ln[N(SiMe3)2]3-μ-Cl-Li(THF)3 (ref. 20) and Ln[N(SiMe3)2]3 (ref. 21) were synthesized according to literature procedures. The yttrium complexes samples for NMR analysis were prepared in a glovebox using dry and oxygen-free NMR tubes. The in situ reaction of complex 2 and n-hexyl isocyanate were performed at −20 °C for several minutes in toluene-d8 sealed in dry and oxygen-free NMR tubes and measured on Agilent 600 MHz Direct Drive 2 spectrometer at room temperature.
Synthesis of L1
1,2-Propanediamine (1.65 ml) in ethanol was dropped in the ethanol solution of 3,5-di-tert-butylsalicylaldehyde (9 g) at reflux temperature for 2 h, then reduced by NaBH4 (5.8 g), adding 50 ml THF and reflux overnight. The solution was evaporated under vacuum and washed by water and extracted by CH2Cl2.after evaporation, the resultant recrystallized in ethanol/ethyl acetate mixture for twice. The white solid (4.95 g, 10 mmol) was dissolved in ethanol/EtOAc and was treated with concentrate HCl (2.2 ml, 22 mol) for half an hour. After evaporation, the white solid was reacted with HC(OEt)3 (50 ml) and HCOOH (9 drops) at 120 °C for 1 h. White precipitation (3 g) was formed and filtrated. The precipitation was washed with ethyl ether three times. 1H NMR (600 MHz, CDCl3) δ 8.86 (1H, s, C2–H(imid)), 7.32 (2H, d, Ar–H), 6.93 (2H, d, Ar–H), 4.85 (4H, s, CH2), 3.26 (4H, t, NCH2CH3CH2N), 1.99 (2H, q, NCH3CH2CH3N), 1.42 (18H, s, tBu), 1.26 (18H, s, tBu); 13C NMR (600 MHz, CDCl3) δ 19.02 (NCH2CH2CH2N); 30.35 (–C(CH3)3); 31.71 (–C(CH3)3); 34.38 (–C(CH3)3); 35.27 (–C(CH3)3); 42.25 (NCH2CH2CH2N); 56.83 (NCH2-Ar); 122.01 (tBu-Ar); 125.33 (tBu-Ar); 125.77 (CH2-Ar); 140.72 (H-Ar); 143.29 (H-Ar); 152.76 (NCHN); 153.59 (O-Ar).
Synthesis of L12NdK(THF) (1)
In a THF solution, L1 (0.37 mmol) was reacted with Nd[N(SiMe3)2]3 (0.18 mmol) and KN(SiMe3)2 (1.11 mol) and stirred for 2 h at −30 °C and overnight at room temperature. Then solvent was removed under vacuum and extracted by hexane/THF solvent mixture. Blue block crystals were obtained at room temperature in 1–2 days. Anal. calcd for C72H108N4O5NdK: C, 66.90; H, 8.26; N, 4.59. Found: C, 67.18; H, 8.59; N, 4.47.
Synthesis of L12YK(thf) (2)
Following a similar procedure described for complex 1. Treatment of a stirring solution of L3 (0.20 g, 0.37 mmol) in THF with Y[N(SiMe3)2]3 (0.18 mmol) and KN(SiMe3)2 (1.11 mol). The reaction mixture was stirred for 2 h at −30 °C and overnight at room temperature, and then the solvent was removed under vacuum and extracted by hexane/THF solvent mixture. Colorless crystals were obtained at room temperature in 1–2 days. 1H NMR (600M, C6D6) δ 1.37, 1.38, 1.50 (36H, s, tBu); 1.52, 1.55, 1.78, 1.97 (36H, s, tBu); 1.40 (4H, t, THF); 1.74 (4H, s, NCH2CH2CH2N); 2.28, 2.35, 2.42, 2.53, 2.70 (8H, m, NCH2CH2CH2N); 2.84, 3.14, 3.47, 3.75, 5.46, 6.05, 6.07, 6.26 (8H, b, NCH2-Ar); 3.52 (4H, m, THF); 6.70, 6.84, 6.95, 7.17 (4H, b, Ar–H); 7.44, 7.60 (4H, m, Ar–H); 13C NMR (600M, C6D6) δ 25.81 (THF); 19.86, 21.44 (NCH2CH2CH2N); 31.06, 31.15, 31.48, 31.56 (–C(CH3)3); 32.14, 32.20, 32.50, 32.52 (–C(CH3)3); 34.05, 34.09, 34.16, 34.23 (–C(CH3)3); 35.71, 35.73, 36.20, 36.24 (–C(CH3)3); 39.87, 40.03, 40.54, 40.65 (NCH2CH2CH2N); 60.14, 61.01, 61.69, 62.01 (NCH2-Ar); 67.79 (THF); 123.06, 123.74, 123.93, 124.43 (tBu-Ar); 124.93, 125.13, 125.30, 125.46 (tBu-Ar); 126.18, 126.44 (CH2-Ar); 134.38, 134.59, 135.51, 136.20 (H-Ar); 136.86, 137.86, 138.44, 138.82 (H-Ar); 162.45, 162.57, 163.53, 164.01 (O-Ar); 211.14, 216.81 (carbene C). Anal. calcd for C72H108N4O5YK: C, 70.07; H, 8.65; N, 4.81. Found: C, 69.90; H, 8.82; N, 4.48.
Synthesis of L13Sm2Cl3 (3)
In a THF solution, L1 (0.20 g, 0.37 mmol) was reacted with Sm[N(SiMe3)2]3 (0.24 mmol) or Sm[N(SiMe3)2]3-μ-Cl-Li(THF)3 (0.24 mmol) and stirred overnight at room temperature, and then the solvent was removed under vacuum and extracted by hexane/THF solvent mixture. Colorless block crystals were obtained at room temperature in 1–2 days. Anal. calcd for C102H153N6Cl13O6Sm2: C, 62.30; H, 7.84; N, 4.27. Found: C, 62.42; H, 7.80; N, 4.24.
Synthesis of L13Y2Cl3(4)
In a THF solution, L1 (0.20 g, 0.37 mmol) was reacted with Y[N(SiMe3)2]3 (0.24 mmol) and stirred overnight at room temperature, and then the solvent was removed under vacuum and extracted by hexane/THF solvent mixture. Colorless crystals were obtained at room temperature in 1–2 days. 1H NMR (600M, THF-d8) δ 0.97 (54H, s, tBu); 1.27 (54H, s, tBu); 1.76 (6H, m, NCH2CH2CH2N); 3.60, 3.23 (12H, m, NCH2CH2CH2N); 3.57, 3.59 (6H, s, NCH2-Ar); 6.47, 6.50 (6H, s, NCH2-Ar); 6.95 (6H, b, Ar–H); 7.03 (6H, b, Ar–H); 9.63 (3H, s, C2–H(ring)); 13C NMR (600M, THF-d8) δ 20.10 (NCH2CH2CH2N); 30.60 (–C(CH3)3); 32.34(–C(CH3)3); 34.41(–C(CH3)3); 35.71(–C(CH3)3); 42.91 (NCH2CH2CH2N); 56.62 (NCH2-Ar); 123.96 (tBu-Ar); 123.98 (tBu-Ar); 125.70 (CH2-Ar); 135.59 (H-Ar); 138.89 (H-Ar); 153.41 (NCHN); 162.29, 162.32 (O-Ar). Anal. calcd for C102H153N6Cl13O6Y2: C, 66.46; H, 8.37; N, 4.56. Found: C, 67.09; H, 8.45; N, 4.40.
Typical procedure for the polymerization of n-hexyl isocyanate
All the polymerizations were carried out in purified argon atmosphere using Schlenk technique and the polymerizations procedure catalyzed by complexes 1–2, 5–7 were similar. In a dry and oxygen-free 20 ml flask, n-hexyl isocyanate (about 0.4 g) and desired toluene was added. The flask was placed in a −20 °C atmosphere, and then desired amount of complex 1 was added and the system became viscous very quickly. The reaction was quenched by adding excess methanol after the prescribed time. The polymer was precipitated and dried under vacuum and weighed.
X-ray crystallographic study
Single crystals of complexes 1 and 3 were sealed in liquid paraffin oil for determination of the single-crystal structures. Data were collected at 170 K on a CrysAlisPro, Oxford Diffraction Ltd. using graphite-monochromatic Mo Kα radiation (λ = 0.71073 Å). The data sets were corrected by empirical absorption correction using spherical harmonics, implemented in SCALE3 ABSPACK scaling algorithm. All structures were solved by direct methods, and refined by full-matrix least-square methods based on |F|2. The structures were solved and refined using SHELX-97 programs. All non-hydrogen atoms were located successfully from Fourier maps and were refined anisotropically. Crystal and refinement data for complexes 1 and 3 are given in Table S1.†
Acknowledgements
The authors gratefully acknowledge the financial support of the National Natural Science Foundation of China (21174121), the Special Funds for Major Basic Research Projects (G2011CB606001), and Zhejiang Provincial Natural Science Foundation of China (LY13B040001). We thank Dr Jun Ling and Mr Fangyu Hu (Zhejiang University) for helpful discussions.
Notes and references
-
(a) S. Bellemin-Laponnaz and S. Dagorne, Chem. Rev., 2014, 114, 8747–8774 CrossRef CAS PubMed
;
(b) L. Palacios, A. Di Giuseppe, A. Opalinska, R. Castarlenas, J. J. Pérez-Torrente, F. J. Lahoz and L. A. Oro, Organometallics, 2013, 32, 2768–2774 CrossRef CAS
;
(c) P. Horeglad, O. Ablialimov, G. Szczepaniak, A. M. Dąbrowska, M. Dranka and J. Zachara, Organometallics, 2014, 33, 100–111 CrossRef CAS
;
(d) D. Zhang and G. Zi, Chem. Soc. Rev., 2015, 44, 1898–1921 RSC
;
(e) G. J. Barbante, E. H. Doeven, P. S. Francis, B. D. Stringer, C. F. Hogan, P. R. Kheradmand, D. J. D. Wilson and P. J. Barnard, Dalton Trans., 2015, 44, 8564–8576 RSC
;
(f) P. L. Arnold, I. J. Casely, Z. R. Turner, R. Bellabarba and R. B. Tooze, Dalton Trans., 2009, 7236–7247 RSC
;
(g) S. T. Liddle, I. S. Edworthy and P. L. Arnold, Chem. Soc. Rev., 2007, 36, 1732–1744 RSC
;
(h) P. L. Arnold and S. T. Liddle, Chem. Commun., 2006, 38, 3959–3971 RSC
;
(i) D. Zhang, S. Zhou, Z. Li, Q. Wang and L. Weng, Dalton Trans., 2013, 42, 12020–12030 RSC
;
(j) Z. Wang, L. Jiang, D. K. B. Mohamed, J. Zhao and T. S. A. Hor, Coord. Chem. Rev., 2015, 293–294, 292–326 CrossRef CAS
. -
(a) C. Romain, S. Choua, J. P. Collin, M. Heinrich, C. Bailly, L. Karmazin-Brellt, S. Bellemin-Laponnaz and S. Dagorne, Inorg. Chem., 2014, 53, 7371–7376 CrossRef CAS PubMed
;
(b) D. Zhang and H. Kawaguchi, Organometallics, 2006, 25, 5506–5509 CrossRef CAS
;
(c) D. Zhang and N. Liu, Organometallics, 2009, 28, 499–505 CrossRef CAS
;
(d) Y. Kong, H. Ren, S. Xu, H. Song, B. Liu and B. Wang, Organometallics, 2009, 28, 5934–5940 CrossRef CAS
;
(e) D. R. Weinberg, N. Hazari, J. A. Labinger and J. E. Bercaw, Organometallics, 2010, 29, 89–100 CrossRef CAS
;
(f) C. Romain, L. Brelot, S. Bellemin-Laponnaz and S. Dagorne, Organometallics, 2010, 29, 1191–1198 CrossRef CAS
;
(g) Y. Kong, M. Cheng, H. Ren, S. Xu, H. Song, M. Yang, B. Liu and B. Wang, Organometallics, 2011, 30, 1677–1681 CrossRef CAS
;
(h) S. Dagorne, S. Bellemin-Laponnaz and C. Romain, Organometallics, 2013, 32, 2736–2743 CrossRef CAS
;
(i) E. Despagnet-Ayoub, L. M. Henling, J. A. Labinger and J. E. Bercaw, Organometallics, 2013, 32, 2934–2938 CrossRef CAS
;
(j) E. Borré, G. Dahm, A. Aliprandi, M. Mauro, S. Dagorne and S. Bellemin-Laponnaz, Organometallics, 2014, 33, 4374–4384 CrossRef
;
(k) C. Romain, C. Fliedel, S. Bellemin-Laponnaz and S. Dagorne, Organometallics, 2014, 33, 5730–5739 CrossRef CAS
;
(l) L. Dang, H. Song and B. Wang, Organometallics, 2014, 33, 6812–6818 CrossRef CAS
;
(m) E. Despagnet-Ayoub, K. Miqueu, J. M. Sotiropoulos, L. M. Henling, M. W. Day, J. A. Labinger and J. E. Bercaw, Chem. Sci., 2013, 4, 2117–2121 RSC
;
(n) H. Aihara, T. Matsuo and H. Kawaguchi, Chem. Commun., 2003, 17, 2204–2205 RSC
;
(o) S. Bellemin-Laponnaz, R. Welter, L. Brelot and S. Dagorne, J. Organomet. Chem., 2009, 694, 604–606 CrossRef CAS
;
(p) S. Barroso, S. R. M. M. de Aguiar, R. F. Munhá and A. M. Martins, J. Organomet. Chem., 2014, 760, 60–66 CrossRef CAS
;
(q) M. Nirmala, G. Prakash, P. Viswanathamurthi and J. G. Malecki, J. Mol. Catal. A: Chem., 2015, 403, 15–26 CrossRef CAS
;
(r) A. El-Batta, A. W. Waltman and R. H. Grubbs, J. Organomet. Chem., 2011, 696, 2477–2481 CrossRef CAS
. - D. Zhang, H. Aihara, T. Watanabe, T. Matsuo and H. Kawaguchi, J. Organomet. Chem., 2007, 692, 234–242 CrossRef CAS
. - D. Bourissou, O. Guerret, F. P. Gabbaï and G. Bertrand, Chem. Rev., 2000, 100, 39–92 CrossRef CAS PubMed
. -
(a) C. Yao, C. Wu, B. Wang and D. Cui, Organometallics, 2013, 32, 2204–2209 CrossRef CAS
;
(b) K. Lv and D. Cui, Organometallics, 2008, 27, 5438–5440 CrossRef CAS
;
(c) Z. Wang, H. Sun, H. Yao, Y. Yao, Q. Shen and Y. Zhang, J. Organomet. Chem., 2006, 691, 3383–3390 CrossRef CAS
;
(d) P. L. Arnold, Z. R. Turner, A. I. Germeroth, I. J. Casely, R. Bellabarba and R. P. Tooze, Dalton Trans., 2010, 39, 6808–6814 RSC
;
(e) L. Maron and D. Bourissou, Organometallics, 2007, 26, 1100–1103 CrossRef CAS
;
(f) Z. Li, M. Xue, H. Yao, H. Sun, Y. Zhang and Q. Shen, J. Organomet. Chem., 2012, 713, 27–34 CrossRef CAS
;
(g) J. Zhang, H. Yao, Y. Zhang, H. Sun and Q. Shen, Organometallics, 2008, 27, 2672–2675 CrossRef CAS
;
(h) P. L. Arnold and S. T. Liddle, Chem. Commun., 2006, 38, 3959–3971 RSC
;
(i) P. L. Arnold, S. A. Mungur, A. J. Blake and C. Wilson, Angew. Chem., 2003, 115, 6163–6166 CrossRef
;
(j) M. Zhang, X. Ni and Z. Shen, Organometallics, 2014, 33, 6861–6867 CrossRef CAS
;
(k) W. Xie, H. Hu and C. Cui, Angew. Chem., 2012, 124, 11303–11306 CrossRef
;
(l) W. Xie, H. Hu and C. Cui, Angew. Chem., Int. Ed., 2012, 51, 11141–11144 CrossRef CAS PubMed
;
(m) Y. Hu, G. M. Miyake, B. Wang, D. Cui and E. Y. X. Chen, Chem.–Eur. J., 2012, 18, 3345–3354 CrossRef CAS PubMed
;
(n) H. Yao, Y. Zhang, H. Sun and Q. Shen, Eur. J. Inorg. Chem., 2009, 13, 1920–1925 CrossRef
;
(o) C. Yao, F. Lin, M. Wang, D. Liu, B. Liu, N. Liu, Z. Wang, S. Long, C. Wu and D. Cui, Macromolecules, 2015, 48, 1999–2005 CrossRef CAS
;
(p) P. L. Arnold and S. T. Liddle, Organometallics, 2006, 25, 1485–1491 CrossRef CAS
;
(q) B. Wang, D. Wang, D. Cui, W. Gao, T. Tang, X. Chen and X. Jing, Organometallics, 2007, 26, 3167–3172 CrossRef CAS
;
(r) H. Yao, J. Zhang, Y. Zhang, H. Sun and Q. Shen, Organometallics, 2010, 29, 5841–5846 CrossRef CAS
;
(s) X. Gu, X. Zhu, Y. Wei, S. Wang, S. Zhou, G. Zhang and X. Mu, Organometallics, 2014, 33, 2372–2379 CrossRef CAS
;
(t) P. L. Arnold, J. McMaster and S. T. Liddle, Chem. Commun., 2009, 7, 818–820 RSC
;
(u) D. Patel, S. T. Liddle, S. A. Mungur, M. Rodden, A. J. Blake and P. L. Arnold, Chem. Commun., 2006, 10, 1124–1126 RSC
;
(v) B. Wang, T. Tang, Y. Li and D. Cui, Dalton Trans., 2009, 8963–8969 RSC
;
(w) T. Mehdoui, J.-C. Berthet, P. Thuery and M. Ephritikhine, Chem. Commun., 2005, 22, 2860–2862 RSC
;
(x) P. L. Arnold, T. Cadenbach, I. H. Marr, A. A. Fyfe, N. L. Bell, R. Bellabarba, R. P. Tooze and J. B. Love, Dalton Trans., 2014, 43, 14346–14358 RSC
;
(y) W. Fegler, T. P. Spaniol and J. Okuda, Dalton Trans., 2010, 39, 6774–6779 RSC
;
(z) P. L. Arnold, Z. R. Turner, A. I. Germeroth, I. J. Casely, G. S. Nichol, R. Bellabarba and R. P. Tooze, Dalton Trans., 2013, 42, 1333–1337 RSC
;
(a
a) P. L. Arnold, I. A. Marr, S. Zlatogorsky, R. Bellabarba and R. P. Tooze, Dalton Trans., 2014, 43, 34–37 RSC
;
(a
b) W. Fegler, T. Saito, K. Mashima, T. P. Spaniol, J. Okuda, J. Organomet. Chem., 695, 2794–2797 Search PubMed;
(a
c) Y. Pan, T. Xu, Y.-S. Ge and X.-B. Lu, Organometallics, 2011, 30, 5687–5694 CrossRef CAS
. -
(a) M. Zhang, Z. Liang, J. Ling, X. Ni and Z. Shen, Dalton Trans., 2015, 44, 11182–11190 RSC
;
(b) Z. Liang, X. Ni, X. Li and Z. Shen, Dalton Trans., 2012, 41, 2812–2819 RSC
;
(c) K. Nie, X. Gu, Y. Yao, Y. Zhang and Q. Shen, Dalton Trans., 2010, 39, 6832–6840 RSC
;
(d) F. Bonnet, H. E. Dyer, Y. El Kinani, C. Dietz, P. Roussel, M. Bria, M. Visseaux, P. Zinck and P. Mountford, Dalton Trans., 2015, 44, 12312–12325 RSC
;
(e) F. Bonnet, A. R. Cowley and P. Mountford, Inorg. Chem., 2005, 44, 9046–9055 CrossRef CAS PubMed
;
(f) Y. Yao, M. Ma, X. Xu, Y. Zhang, Q. Shen and W. Wong, Organometallics, 2005, 24, 4014–4020 CrossRef CAS
;
(g) T. Zeng, Q. Qian, Y. Wang, Y. Yao and Q. Shen, J. Organomet. Chem., 2015, 779, 14–20 CrossRef CAS
;
(h) T. Zeng, Y. Wang, Q. Shen, Y. Yao, Y. Luo and D. Cui, Organometallics, 2014, 33, 6803–6811 CrossRef CAS
;
(i) T. Zeng, Q. Qian, B. Zhao, D. Yuan, Y. Yao and Q. Shen, RSC Adv., 2015, 5, 53161–53171 RSC
;
(j) J. Ling and Z. Shen, Macromol. Chem. Phys., 2002, 203, 735–738 CrossRef CAS
;
(k) Z. Liang, X. Ni, X. Li and Z. Shen, Inorg. Chem. Commun., 2011, 14, 1948–1951 CrossRef CAS
;
(l) L. Wang, Z. Liang, X. Ni and Z. Shen, Chin. Chem. Lett., 2011, 22, 249–252 CrossRef CAS
;
(m) X. Ni, Z. Liang, J. Ling, X. Li and Z. Shen, Polym. Int., 2011, 60, 1745–1752 CrossRef CAS
;
(n) J. Ling, W. Zhu and Z. Shen, Macromolecules, 2004, 37, 758–763 CrossRef CAS
. -
(a) N. Fukuwatari, H. Sugimoto and S. Inoue, Macromol. Rapid Commun., 1996, 17, 1–7 CrossRef CAS
;
(b) A. Touris, K. Kostakis, S. Mourmouris, V. Kotzabasakis, M. Pitsikalis and N. Hadjichristidis, Macromolecules, 2008, 41, 2426–2438 CrossRef CAS
;
(c) X. Liu, J. Deng, Y. Wu and L. Zhang, Polymer, 2012, 53, 5717–5722 CrossRef CAS
;
(d) T. E. Patten and B. M. Novak, J. Am. Chem. Soc., 1991, 113, 5065–5066 CrossRef CAS
;
(e) A. J. Bur and L. J. Fetters, Chem. Rev., 1976, 76, 727–746 CrossRef CAS
. -
(a) V. E. Shashoua, W. Sweeny and R. F. Tietz, J. Am. Chem. Soc., 1960, 82, 866–873 CrossRef CAS
;
(b) V. E. Shashoua, J. Am. Chem. Soc., 1959, 81, 3156–3156 CrossRef CAS
. -
(a) Y. D. Shin, S. Y. Kim, J. H. Ahn and J. S. Lee, Macromolecules, 2001, 34, 2408–2410 CrossRef CAS
;
(b) J. S. Lee and S. W. Ryu, Macromolecules, 1999, 32, 2085–2087 CrossRef CAS
;
(c) A. Vazaios, M. Pitsikalis and N. Hadjichristidis, J. Polym. Sci., Part A: Polym. Chem., 2003, 41, 3094–3102 CrossRef CAS
. - J. Min, P. N. Shah, J. H. Ahn and J. S. Lee, Macromolecules, 2011, 44, 3211–3216 CrossRef CAS
. - T. E. Patten and B. M. Novak, Makromol. Chem., Macromol. Symp., 1993, 67, 203–211 CrossRef CAS
. - K. Tanaka, M. Furo, E. Ihara and H. Yasuda, J. Polym. Sci., Part A: Polym. Chem., 2001, 39, 1382–1390 CrossRef CAS
. -
(a) X. Yang, X. Ni and Z. Shen, Sci. China, Ser. B: Chem., 2009, 52, 1843–1850 CrossRef CAS
;
(b) J. Jiao, X. Yang and X. Ni, Acta Polym. Sin., 2011, 5, 475–480 CrossRef
;
(c) X. Ni, X. Xu and Z. Shen, J. Appl. Polym. Sci., 2007, 103, 2135–2140 CrossRef CAS
;
(d) X. Xu, X. Ni and Z. Shen, Polym. Bull., 2005, 53, 81–88 CrossRef CAS
;
(e) E. Ihara, Y. Adachi, H. Yasuda, H. Hashimoto, N. Kanehisa and Y. Kai, J. Organomet. Chem., 1998, 569, 147–157 CrossRef CAS
;
(f) H. Wang, H. Li, X. Yu, Z. Ren and J. Lang, Tetrahedron, 2011, 67, 1530–1535 CrossRef CAS
;
(g) H. Yasuda, J. Organomet. Chem., 2002, 647, 128–138 CrossRef CAS
;
(h) T. Ikeda, H. Sugimoto and S. Inoue, J. Macromol. Sci., Part A: Pure Appl.Chem., 1997, 34, 1907–1920 CrossRef
. - V. T. Kasumov and F. Köksal, Spectrochim. Acta, Part A, 2005, 61, 225–231 CrossRef PubMed
. - P. L. Arnold and I. J. Casely, Chem. Rev., 2009, 109, 3599–3611 CrossRef CAS PubMed
. - P. Liu, M. Wesolek, A. A. Danopoulos and P. Braunstein, Organometallics, 2013, 32, 6286–6297 CrossRef CAS
. -
(a) A. Amgoune, C. M. Thomas, S. Ilinca, T. Roisnel and J. F. Carpentier, Angew. Chem., 2006, 118, 2848–2850 CrossRef
;
(b) N. Ajellal, D. M. Lyubov, M. A. Sinenkov, G. K. Fukin, A. V. Cherkasov, C. M. Thomas, J. F. Carpentier and A. A. Trifonov, Chem.–Eur. J., 2008, 14, 5440–5448 CrossRef CAS PubMed
. - X. Xu, X. Ni, X. Hong and Z. Shen, Chem. Res. Chin. Univ., 2005, 21, 224–226 CAS
. - S. Mourmouris, K. Kostakis, M. Pitsikalis and N. Hadjichristidis, J. Polym. Sci., Part A: Polym. Chem., 2005, 43, 6503–6514 CrossRef CAS
. - S. Zhou, S. Wang, G. Yang, X. Liu, E. Sheng, K. Zhang, L. Cheng and Z. Huang, Polyhedron, 2003, 22, 1019–1024 CrossRef CAS
. -
(a) F. T. Edelmann, A. Steiner, D. Stalke, J. W. Gilje, S. Jagner and M. H»kansson, Polyhedron, 1994, 13, 539–546 CrossRef CAS
;
(b) D. C. Bradley, J. S. Ghotra and F. A. Hart, J. Chem. Soc., Dalton Trans., 1973, 10, 1021–1023 RSC
.
Footnote |
† Electronic supplementary information (ESI) available: NMR spectra and cif files. CCDC 1407874 (1) and 994627 (3). For ESI and crystallographic data in CIF or other electronic format see DOI: 10.1039/c5ra16447h |
|
This journal is © The Royal Society of Chemistry 2015 |
Click here to see how this site uses Cookies. View our privacy policy here.