DOI:
10.1039/C5RA15548G
(Paper)
RSC Adv., 2015,
5, 92387-92393
MCM-41 bound dibenzo-18-crown-6 ether: a recoverable phase-transfer nano catalyst for smooth and regioselective conversion of oxiranes to β-azidohydrins and β-cyanohydrins in water†
Received
4th August 2015
, Accepted 15th October 2015
First published on 15th October 2015
Abstract
A new organic–inorganic hybrid nanocomposite was prepared by immobilizing dibenzo-18-crown-6 onto covalently linked amine functionalized mesoporous MCM-41 via a simple post-synthesis method. The heterogeneous hybrid nanocomposite was characterized by TEM, TGA, BET and FT-IR. The potential applications of this covalently linked crown ether functionalized MCM-41 catalyst were also investigated as an efficient, heterogeneous and recyclable host material for the regioselective ring opening of epoxides by cyanide and azide anions in water. As expected, the cavity of immobilized dibenzo-18-crown-6 demonstrated excellent selectivity of metal ion adsorption and showed better complexation with K+ than Na+ and so the reaction time for the ring opening of epoxides by KCN reduced. Low catalyst loading, high efficiency and reusability are among the advantages of this environmentally friendly method.
1. Introduction
In the past few decades increasing attention has been paid to the design and development of economically feasible materials and processes to eliminate the use of harmful substances and the generation of toxic waste materials. In response to this challenge, recently, research has been focused on the synthesis and functionalization of mesoporous materials with novel structures.1 Among these, MCM-41 has attracted a great deal of attention due to its interesting advantages such as tunable pore size, controlled size and morphology, and dual-functional surface (external and internal).2 The one-dimensional pores in MCM-41 are of uniform size and shape distribution over the micrometer length scale, which allows much faster diffusion of large organic molecules.3 These features make the material of considerable interest to heterogeneous catalysis.4,5
Crown ethers, a class of macrocyclic ligands with high affinity and selectivity for alkali and alkaline earth metal ions,6 have proven to be effective as phase transfer catalyst in organic transformations. Due to their hydrophobic exteriors and hydrophilic cores, they are typically proper segments to make a junction with larger globular organic molecules. This can facilitate the dissolution in solvents with different polarities.7
On the other hands, β-azido and β-cyanohydrins have found widespread applications as pharmaceuticals and industrial compounds and shown a broad spectrum of biological activities.8 Owing to the widespread interest in β-azidohydrins and β-cyanohydrins, and in conjunction with ongoing work in our laboratory on the synthesis of new phase-transfer catalysts,9,10 we would like to describe the preparation of a new organic–inorganic hybrid nanocomposite, dibenzo-18-crown-6 functionalized MCM-41, as a green, efficient, and heterogeneous catalyst for the regioselective ring opening of epoxides in water. We found that the presence of crown ether as functional groups on the MCM-41 has a great prospective to accelerate the ring opening reaction rate. As described here, a variety of azidohydrins and cyanohydrins can be synthesized in atom economical manner with high regioselectivity under mild and non-toxic conditions.
2. Experimental
2.1. General
3,4-Dihydroxy benzaldehyde, cetyltrimethyl ammonium bromide (CTAB), epoxides and other chemicals were purchased from Fluka and Merck companies. The purity determination of the products and reaction monitoring were accomplished by TLC on silica gel. Products were characterized by comparison of their physical data, IR, NMR spectra with known samples. NMR spectra were recorded on a Bruker Advance DPX 400 MHz instrument spectrometer using TMS as internal standard. IR spectra were recorded on a BOMEM MB-Series 1998 FT-IR spectrometer. The surface area and pore size distribution of the support was measured by the nitrogen adsorption–desorption method (ASAP 2000, Micromeritics). Transmission electron microscope (TEM) images were obtained using Zeiss – EM10C – 80 kV instrument. The mass spectra were scanned on Agilent Technology ((HP) 5973 Network Mass Selective Detector) instrument at 70 eV.
2.2. Synthesis of diethylene glycol ditosylate
Diethylene glycol ditosylate was synthesized by a previously reported method.11 Briefly, 14.86 g of diethylene glycol (140 mmol) was dissolved in 80 mL of THF. Meanwhile, an aqueous solution of 16 g sodium hydroxide (400 mmol) in 80 mL of water was prepared. Two solutions were mixed and cooled on ice bath with magnetic stirring. To the mixture was added drop wise 48.6 g of p-toluenesulfonyl chloride (260 mmol) in THF (80 mL) over 2 h with continuous stirring and cooling of mixture below 5 °C. The solution was stirred at 0–5 °C for additional 2 h, after this, the mixture was poured into ice-water (250 mL). The product was filtered, washed with distilled water, dried and recrystallized twice from methanol to give a pure product. Mp: 85–88 °C (Lit11 (86–89)).
2.3. Synthesis of 4′,4′′-diformyl dibenzo-18-crown-6 ether
The benzocrown ether has been synthesized according Pederson's method with suitable adaptation.12 A mixture of 8.28 g of 3.4-dihydroxy benzaldehyde (60 mmol), 40 mL of 1-butanol and 2.44 g of sodium hydroxide pellets (60 mmol) was refluxed under argon for 30 min to ensure complete dissolution of sodium hydroxide. A solution of 12.36 g of diethylene glycol ditosylate (30 mmol) diluted with 20 mL of 1-butanol was added slowly over 1 h, and the mixture was refluxed for 1 h. The temperature was lowered to 90 °C, and 2.44 g of sodium hydroxide pellets was added. Refluxing was continued for 30 min, and 12.36 g of diethylene glycol ditosylate diluted with 20 mL of l-butanol was added slowly over 1 h. Refluxing was continued for 16 h. Concentrated hydrochloric acid (1 mL) was slowly added, and then 1/3 of solvent was rapidly removed by distillation. The distillation was continued but the volume in the flask was kept constant by the steady addition of water until the vapour temperature reached 100 °C. The mixture was filtered, washed with 200 mL of water, and sucked dry. The solids were dispersed in 200 mL of acetone, stirred for 30 min, filtered, washed with 50 mL of acetone, and dried in an oven at 100 °C.
2.4. Synthesis of aminopropyl-grafted mesoporous MCM-41
The aminopropyl-grafted mesoporous MCM-41 has been synthesized using an earlier procedure with suitable adaptation.13 In a typical synthesis, cetyltrimethylammonium bromide (CTAB) (2 mmol, 0.8 g) was dissolved in 175 mL of de-ionized water containing 0.204 g of sodium hydroxide (5.1 mmol) heated at 80 °C for 30 min. 0.362 g of 3-aminopropyltrimethoxysilane (APTMS) (12 mmol) and 3.82 g of tetraorthosilicate (TEOS) (18.3 mmol) was added to the homogenous solution with constant stirring at 80 °C for 2 h. The reaction was quenched by cooling the solution to the room temperature. The white solid product was filtered off, washed with sufficient amount of water followed by ethanol and dried under reduced pressure. The surfactant (CTAB) was removed by acid extraction with concentrated HCl (0.5 mL) in methanol (50 mL) at 60 °C for 6 h. The surfactant removed aminopropyl-grafted mesoporous MCM-41 was filtered and washed with ethanol, and then dried under vacuum at 60 °C.
2.5. Synthesis of MCM-41 bound dibenzo-18-crown-6 ether
0.624 g of 4′,4′′diformyl dibenzo-18-C-6 (1.5 mmol) was dissolved in 50 mL of CHCl3 and refluxed to complete dissolving of crown ether. 1 g of aminopropyl-grafted mesoporous MCM-41 was added to the mixture and refluxed for 24 h. The participate was filtered, washed with dry CHCl3 (3 × 20 mL) and dried in oven at 50 °C for 4 h.
2.6. Typical procedure for the preparation of β-azidohydrins and β-cyanohydrins in water catalyzed by MCM-41 bound dibenzo-18-crown-6 ether
To a magnetically stirred mixture of the epoxide (1.0 mmol) and nucleophilic reagents (NaN3, NaCN) (3 mmol) in H2O (3 mL), MCM-41 bound dibenzo-18-crown-6 ether (0.01 g in reaction with azide and 0.03 g in reaction with cyanide nucleophile) was added. The resulting mixture was stirred at 80 °C for the appropriate time (10–45 min). After completion of the reaction as indicated by TLC [using n-hexane/ethyl acetate (3
:
1)], the insoluble catalyst was filtered off and the filtrate extracted with diethyl ether (2 × 10 mL). The organic phase was dried over calcium chloride, and evaporated in vacuo to give the product in 75–90% isolated yields.
3. Results and discussion
The systematic steps of MCM-41 bound dibenzo-18-crown-6 ether, MCM-crown, preparation was shown in Scheme 1.
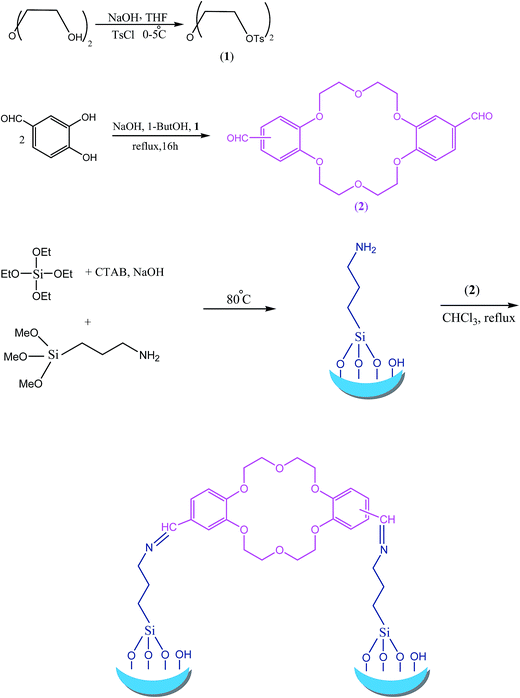 |
| Scheme 1 Synthetic procedure of MCM-crown. | |
First, diethylene glycol ditosylate was synthesized by nucleophilic substitution of p-toluenesulfonyl chloride with diethylene glycol, with molar ratio 2
:
1, in basic aqueous conditions and verified by FT-IR spectra. The lake of OH peak in diethylene glycol ditosylate spectra shows that this reaction is well done and so a new peak at 1579 cm−1 correspond to stretch vibrating of aromatic ring and the presence of peaks at 1364 and 1170 cm−1 due to the asymmetric and symmetric stretching vibrations of sulfonyl groups shows that OH groups are converted to the tosylate (ESI†).
In the next step, 4′,4′′-diformyl dibenzo-18-crown-6 was synthesized according to Pedersen's procedure by the reaction of 3,4-dihydroxy benzaldehyde and diethylene glycol ditosylate under controlled conditions. This reaction had a good yield (76%) as compared to similar reaction with catechol (40–50%). The FT-IR, mass spectra, C, H, N technique and melting point (226 °C (Lit:14 228–230)) successfully confirmed the corresponding crown ether formation.
Well-ordered mesoporous aminopropyl-grafted, mesoporous MCM-41, MCM-NH2, was synthesized by the direct incorporation of aminopropyl groups through co-condensation of TEOS and APTMS precursors in the present of CTAB.
CTAB as cationic surfactant micelle act as templates as well as structure directing agents in mesoporous material synthesis through an electrostatic interaction with the polymerizing silica components. For the preparation of target nanocomposite, MCM-41 bound dibenzo-18-crown-6 ether (MCM-crown), 4′,4′′-diformyl dibenzo-18-crown-6 was covalently grafted onto the surface of the mesoporous silica by simple condensation reaction (Scheme 1).
The structures of MCM-41, MCM-NH2 and MCM-crown were further confirmed by FT-IR spectra. As shown in Fig. 1, in MCM-41, MCM-NH2 and MCM-crown, the typical Si–O–Si bands around 1220, 1070, 801 and 473 cm−1 associated with the formation of a condensed silica network are present, but weak peaks associated with noncondensed Si–OH groups in the range of 940–960 cm−1 were also present. The strong peak around 1630 cm−1 is mainly from the bending vibration of adsorbed H2O and the new peak at 1686 cm−1 in (b) spectra assigned to the imine band due to the reaction of aldehyde group (bisformyl dibenzo-18-crown-6) and MCM-NH2. The band at 2987 cm−1 corresponded to stretch vibrating mode of C–H in (b) and (c).
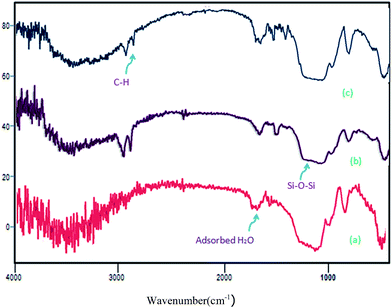 |
| Fig. 1 The FT-IR spectra of (a) MCM-41, (b) MCM-NH2 and (c) MCM-crown. | |
TEM images of MCM-crown are shown in Fig. 2. Periodic structure was observed indicating the high quality and long range order of the modified MCM-41 material. Some dark spots were observed in the TEM images of MCM-crown, which was attributed to the fact that some crown ethers were dispersed within the pores and/or on the surface of the MCM-41.
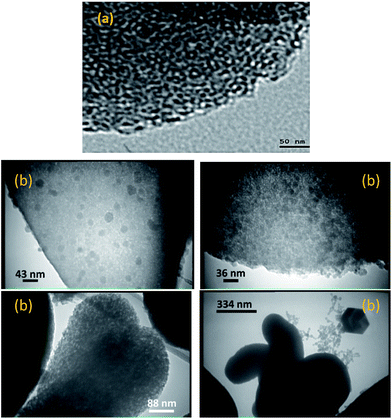 |
| Fig. 2 TEM images the (a) MCM-41, (b) MCM-crown. | |
Fig. 3 and 4 represent the low temperature nitrogen adsorption–desorption isotherms and pore size distributions of MCM-crown. Sample exhibited type-IV isotherms with sharp capillary condensation steps at relative pressure (P/Po) range and an H1 type narrow hysteresis loop, which is typical of mesoporous solids.
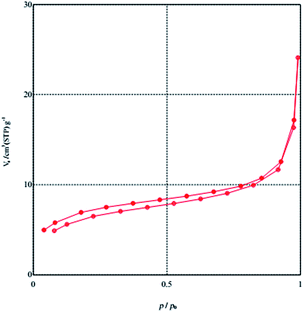 |
| Fig. 3 Nitrogen adsorption isotherms of MCM-crown. | |
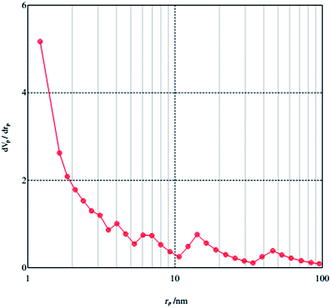 |
| Fig. 4 Pore size distribution curves of MCM-crown. | |
The hysteresis loops are very narrow for material, which clearly points out the narrow channels of MCM-41 materials. The BET surface area and the pore volume of MCM-crown were determined to be 22.53 m2 g−1 and 0.0366 cm3 g−1. According to the previous report about BET surface area and pore volume of MCM-41 (BET: 836 m2 g−1 and pore volume: 0.725 cm3 g−1),15 decrease in these values after loading crown ethers on MCM-41 is due to this fact that the crown ethers unites were deposited inside the mesochannels and dispersed on the surface of MCM-41.
Thermal stability of samples was investigated by Thermal Gravimetric Analysis-Derivative Thermo gravimetric Analysis (TGA-DTG) in which the observed weight loss was associated with the loss of the organic components attached to the MCM-41. TGA-DTG curves for MCM-crown were shown in Fig. 5.
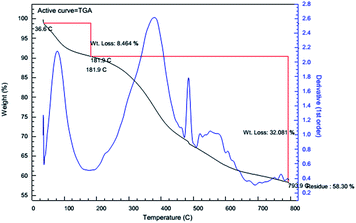 |
| Fig. 5 Thermal Gravimetric Analysis-Derivative Thermogravimetric Analysis (TGA-DTGA). | |
It shows three distinct steps of weight loss in the combined TG-DTG curves. The curves show that the first weight loss occurs before 200 °C, which can be attributed completely to the loss of adsorbed water molecules. The secondary weight losses at about 380 °C and 480 °C come from the decomposition of organic substances in MCM-crown composites. The decomposition is complete at about 650–700 °C to form the constituent inorganic oxides. TGA of the samples demonstrated high thermal stability, with decomposition starting at around 300 °C under a nitrogen atmosphere.
We examined the catalytic ability of MCM-crown for nucleophilic ring opening of oxirans in water with different nucleophiles. At first, ring opening of phenyl glycidyl ether was investigated with NaN3 in the presence of nanocomposite. TLC analysis of the reaction mixture interestingly showed that this catalyst acted very efficiently in H2O, and that 0.01 g of the catalyst was enough to convert 1 mmol of different epoxides, carrying electron-donating or withdrawing groups, to their corresponding β-azidohydrins in high yields ((Scheme 2) Table 1). It is noteworthy that no evidence for diols formation as reaction by-product was observed.
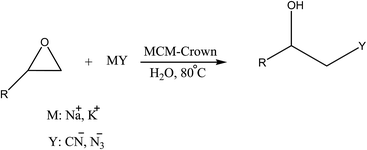 |
| Scheme 2 Preparation of β-azidohydrine and β-cyanohydrine in presence of MCM-crown catalyst. | |
Table 1 Ring opening of epoxide with sodium azide and sodium cyanide in water, catalyzed by MCM-crown
Entry |
Epoxides |
Products |
Nucleophile (Y) |
Time (min) |
Yield (%) |
The reaction performed at 60 °C. |
1 |
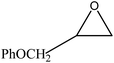 |
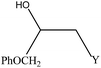 |
N3 (CN) |
20(45) |
89(85) |
2 |
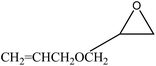 |
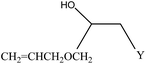 |
N3 (CN) |
10(40) |
93(90) |
3 |
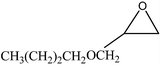 |
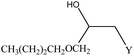 |
N3 (CN) |
15(45) |
87(82) |
4 |
 |
 |
N3 (CN) |
10(35) |
84(88) |
5 |
 |
 |
N3 (CN) |
15(45) |
88(86) |
6 |
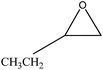 |
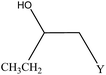 |
N3 (CN) |
15a(35) |
75(75) |
This reaction was also performed in similar manner with a weaker nucleophile, CN−, to obtain β-cyanohydrins. It is proved that for successfully conversion of epoxides to cyanohydrins, 0.03 g of nanocomposite is required (Table 1).
As shown in Table 1, the reaction time in the case of sodium cyanide increased (compared to azide nucleophile), but the changes of cation (K+ instead of Na+) reduced this time near the 40% (see Table 2). It is due to this fact that the cavity size of dibenzo-18-crown-6 conforming to the K+ size, therefore the nucleophilicity of CN− anion was improved and so the reaction time reduced (Scheme 3).
Table 2 Comparison of time/yield between NaCN and KCN
Entry |

|
NaCN |
KCN |
R |
Time (min) |
Yield (%) |
Time (min) |
Yield (%) |
1 |
PhOCH2 |
45 |
85 |
30 |
92 |
2 |
CH2 CHCH2OCH2 |
40 |
90 |
25 |
90 |
3 |
CH3(CH2)2CH2OCH2 |
45 |
82 |
30 |
85 |
4 |
Cyclohexenyl |
35 |
88 |
20 |
90 |
5 |
Ph |
45 |
86 |
35 |
88 |
6 |
CH3CH2 |
35 |
75 |
25 |
75 |
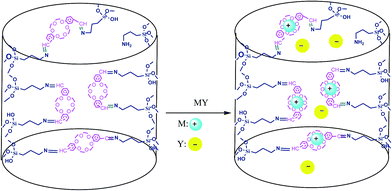 |
| Scheme 3 Operation of catalyst in presence of nucleophile salt. | |
The most ideal synthetic methodology could be defined as a system wherein 100% atom economy as preserved, the catalyst is recycled, and excess of reagent remains throughout in the medium and does not lose activity for several runs. As shown in Scheme 4, after completion of the reaction, the product was extracted by ether and so the remained mixture containing 2 mmol of NaN3 and catalyst was reused for another run by adding 1 mmol of NaN3 and epoxide (1 mmol). The catalyst can be reused for up to ten cycles without significant losing in its activity (Fig. 6).
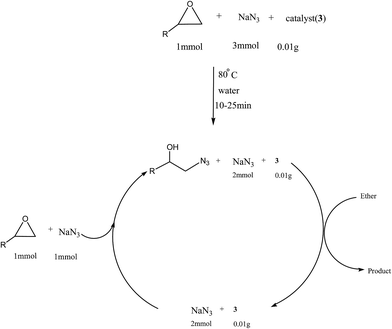 |
| Scheme 4 Facile nucleophilic ring opening of epoxides in the atom economical method. | |
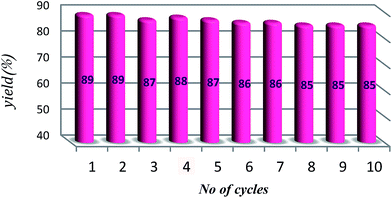 |
| Fig. 6 Recyclability of catalyst. | |
In order to show the merit of our procedure for the synthesis of 1,2-azidoalcohols, we have shown the advantages of present procedure by comparing our results with those previously reported in the literature (Table 3).
Table 3 Comparison of azidolysis of styrene oxide with different methods
Entry |
Catalyst/reagent/solvent |
Time (h) |
Yield (%) |
Ref. |
1 |
LiClO4/NaN3/CH3CN |
5 |
92 |
16 |
2 |
CeCl3/NaN3/CH3CN–H2O |
3 |
89 |
17 |
3 |
Polymeric PTC/NaN3/H2O |
6 |
95 |
18 |
4 |
NH4Cl/NaN3/MeOH–H2O |
5 |
90 |
16 |
5 |
PEG/NaN3 |
1.5 |
85 |
19 |
6 |
NaN3/H2O (pH = 9.5) |
13 |
90 |
20 |
7 |
(TBA)4PFeW11O39·3H2O/NaN3/CH3CN–H2O |
4.5 |
85 |
21 |
8 |
Co(salen)/HN3/TBME |
8 |
50 |
22 |
9 |
PEG-SiO2/NaN3/H2O |
0.5 |
86 |
9 |
10 |
Calix[4]resorcinarenes network polymer/NaN3/H2O |
1.5 |
89 |
10 |
11 |
MCM-crown/NaN3/H2O |
0.25 |
88 |
— |
4. Conclusion
In conclusion, MCM-crown was successfully synthesized and characterized by FT-IR, TEM, TGA-DTG, BET and BJH. The catalytic activity of this phase transfer catalyst has been applied for the ring opening of epoxides to the corresponding β-azidohydrins and β-cyanohydrins in water. Short reaction times, simplicity in operation, catalyst with high catalytic activity and good recycle exploitation are the advantages of this procedure.
Acknowledgements
We gratefully acknowledge the support of this work by Shahid Chamran University Research Council.
References
- N. Yoneda, T. Minami, J. Weiszmann, B. Spehlmann, H. Hattori and K. Otsuka, Stud. Surf. Sci. Catal., 1999, 121, 93–98 CrossRef CAS.
- K. Moller and T. Bein, Chem. Mater., 1998, 10, 2950 CrossRef CAS.
- M. D. Alba, Z. Luan and J. Klinowski, J. Phys. Chem., 1996, 100, 2178–2182 CrossRef CAS.
- S. Rostamnia and E. Doustkhah, RSC Adv., 2014, 4, 28238–28248 RSC.
- W. He, H. Zhao, R. Yao and M. Cai, RSC Adv., 2014, 4, 50285–50294 RSC.
- T. L. Yost Jr, B. C. Fagan, L. R. Allain, C. E. Barnes, Sh. Dai, M. J. Sepaniak and Z. Xue, Anal. Chem., 2000, 72, 5516–5519 CrossRef.
- A. Khazaei, M. Kianborazjani and K. Mansouri Moradian, J. Chem. Sci., 2012, 124, 1127–1135 CrossRef CAS.
- The Chemistry of the Azido Group, ed. S. Patai, Wiley, New York, 1971 Search PubMed.
- A. R. Kiasat, S. Nazari and J. Davarpanah, C. R. Chim., 2014, 17, 124–130 CrossRef CAS.
- A. Mouradzadegun, A. R. Kiasat and P. Kazemian Fard, Catal. Commun., 2012, 29, 1–5 CrossRef CAS.
- M. Ough, Y. Inoue, Y. Liu, S. Nagamune, S. Nakamur, K. Wada and T. Hakushi, Bull. Chem. Soc. Jpn., 1990, 63, 1260–1262 CrossRef.
- C. J. Pedersen, J. Am. Chem. Soc., 1967, 89, 7017–7036 CrossRef CAS.
- E. Vasile, F. Dumitru, A. Razvan, O. Oprea and C. Andronescu, Digest Journal of Nanomaterials and Biostructures, 2013, 8, 433–444 Search PubMed.
- F. Wada, H. Hirayama, H. Namiki, K. Kikukawa and T. Matsuda, Bull. Chem. Soc. Jpn., 1980, 53, 1473–1474 CrossRef CAS.
- G. Luo, L. Kang, M. Zhu and B. Dai, Fuel Process. Technol., 2014, 118, 20–27 CrossRef CAS.
- M. Chini, P. Crotti and F. Macchia, Tetrahedron Lett., 1990, 31, 5641 CrossRef CAS.
- G. Sabitha, R. S. Babu, M. Rajkumar and J. S. Yadav, Org. Lett., 2002, 4, 343 CrossRef CAS.
- B. Tamami and H. Mahdavi, Tetrahedron Lett., 2001, 42, 8721 CrossRef CAS.
- A. R. Kiasat and M. Fallah-Mehrjardi, J. Iran. Chem. Soc., 2009, 6, 542–546 CrossRef CAS.
- F. Fringuelli, O. Piermatti, F. Pizzo and L. J. Vaccaro, J. Org. Chem., 1999, 64, 6094 CrossRef CAS.
- B. Yadollahi and H. Danafar, Catal. Lett., 2007, 113, 120 CrossRef CAS.
- S. W. Chen, S. S. Thakur, W. Li, C. K. Shin, R. B. Kawthekar and G. J. Kim, J. Mol. Catal. A: Chem., 2006, 259, 116 CrossRef CAS.
Footnote |
† Electronic supplementary information (ESI) available. See DOI: 10.1039/c5ra15548g |
|
This journal is © The Royal Society of Chemistry 2015 |
Click here to see how this site uses Cookies. View our privacy policy here.