DOI:
10.1039/C5RA13441B
(Communication)
RSC Adv., 2015,
5, 66718-66722
Iodine catalysed intramolecular C(sp3)–H functionalization: synthesis of 2,5-disubstituted oxazoles from N-arylethylamides†
Received
9th July 2015
, Accepted 28th July 2015
First published on 28th July 2015
Abstract
Iodine catalyzed synthesis of 2,5-disubstituted oxazoles from N-arylethylamides through intramolecular C(sp3)–H functionalization under metal-free conditions is described. The method is tolerable to a wide range of substrates having a variety of functional groups with moderate to good yields of the products.
In addition to biologically active molecules for drug discovery, oxazoles are also key intermediates for the synthesis of natural products, pharmaceutical and agricultural products.1–6 Additionally, oxazole moieties are used as fluorescent dyes,7 in polymer industries,8 and also serve as ligands in various metal-catalysed organic transformations.9 As a result, the development of more facile synthetic methods to access oxazole derivatives has become of great interest to chemists.
Considering the importance of oxazole moieties, many groups have developed important methods for the synthesis of substituted oxazoles.10–15 Among the reported methods the conversion of acyclic precursors to oxazole is the common strategy.11–13 For example Robinson–Gabriel condensation is a versatile cyclisation strategy to synthesise a range of substituted oxazoles (Scheme 1A).11 On the other hand enamides bearing β-vinylic carbon heteroatom (Br, and S) were subjected for the synthesis of oxazoles12 (Scheme 1B). A step forward to the aforementioned strategies, several examples of transition metal catalyzed direct vinylic C–H functionalization of enamides have also been described (Scheme 1C).13 Recently, Stahl and Buchwald groups were independently reported copper mediated/catalysed vinylic C–H functionalization of enamides to obtain 2,5-disubstituted oxazoles.13h,i Although these approaches are effective, the cyclisation of N-arylethylamides to oxazoles through C(sp3)–H functionalization under transition metal-free conditions would be a convenient method to access the substituted oxazoles (Scheme 2). The separation of metal catalyst from the products is of particular importance for the synthesis of pharmaceutical fine chemicals because of their residual toxicity is a central issue to consider. Moreover, transition metal-catalyzed reactions also generate hazardous waste which is environmentally problematic and hence, should be avoided wherever possible. Very recent reports from Ghosh et al., and Bathula et al. described the synthesis of substituted oxazoles from N-arylethylamides with more than stoichiometric use of N-bromosuccinimide (NBS),14 these methods also suffers drawbacks such as generation of organic waste (succinimide) in the effluent and is not applicable to obtain 2,5-disubstituted oxazoles. At the outset of our interest towards the development of new strategies for the synthesis of various heterocyclic compounds,16a–e we wish to report herewith a metal-free synthesis of oxazoles through intramolecular C(sp3)–H functionalization (Scheme 2). To the best of our knowledge, no such reports exist for the synthesis of 2,5-disubstituted oxazoles from N-arylethylamides.
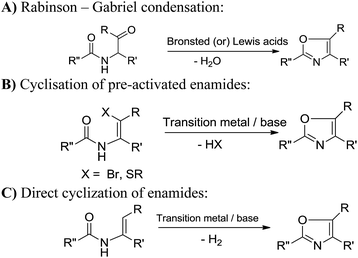 |
| Scheme 1 Reported strategies for oxazole synthesis. | |
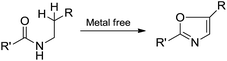 |
| Scheme 2 Oxazole synthesis from N-arylethylamides. | |
We initiated our studies with N-phenethylbenzamide 1a as starting substrate, which has been subjected to oxidative intramolecular C–O bond formation to obtain 2,5-diphenyloxazole 2a with catalytic amount of iodine source and TBHP as the oxidant based on our recent reports on such reactions16f,g and the results are illustrated in Table 1. Initially we tested the reaction with 20 mol% of KI as an iodine source, TBHP (5.0 equiv.) as an oxidant and acetonitrile as the solvent at 100 °C for 36 h, 15% of desired product 2a was observed (Table 1, entry 1). Then we have screened out other iodine sources under these reaction conditions up to 35% yield of desired product 2a could be obtained (Table 1, entries 2–5). When the reaction was performed under the same conditions with 20 mol% of elemental iodine, 68% of 2a was isolated (Table 1, entry 6). By decreasing the reaction time to 24 h or reaction temperature to 80 °C the yield of 2a was dropped to 55% and 28% respectively (Table 1, entries 7 and 8). Further, its yield was decreased by decreasing the amount of oxidant (TBHP) or I2 (Table 1, entries 9–11) and no reaction or traces amount of 2a was observed in the absence of I2 or TBHP (Table 1, entries 12 and 13) or with other oxidants such as K2S2O8, KHSO5, H2O2 and DTBP (Table 1, entries 14–17). Then we focused on the effect of other solvents for the present transformation by fixing the temperature at 100 °C, reaction time 36 h, I2 (20 mol%) and the oxidant TBHP (5.0 equivalents). With the several solvents tested for the reaction, the yield of 2a was not improved (Table 1, entries 18–23). However, the best result was obtained in acetonitrile as the solvent (entry 6) for the present transformation.
Table 1 Optimisation of reaction conditionsa

|
Entry |
Catalyst (mol%) |
Oxidant (equiv.) |
Solvent |
Temp. (°C) |
Time (h) |
2a yields (%) |
Reaction conditions otherwise stated: 0.2 mmol of 1a, 1.0 mmol of TBHP (decane), 20 mol% catalyst in 1.0 mL of CH3CN at 100 °C for 36 h. 10 mol% I2. DTBP = di-tert-butyl peroxide. |
1 |
KI |
TBHP (5) |
CH3CN |
100 |
36 |
15 |
2 |
nBu4NI |
TBHP (5) |
CH3CN |
100 |
36 |
10 |
3 |
NIS |
TBHP (5) |
CH3CN |
100 |
36 |
35 |
4 |
NaI |
TBHP (5) |
CH3CN |
100 |
36 |
23 |
5 |
PIDA |
TBHP (5) |
CH3CN |
100 |
36 |
0 |
6 |
I2 |
TBHP (5) |
CH3CN |
100 |
36 |
68 |
7 |
I2 |
TBHP (5) |
CH3CN |
100 |
24 |
55 |
8 |
I2 |
TBHP (5) |
CH3CN |
80 |
36 |
28 |
9 |
I2 |
TBHP (4) |
CH3CN |
100 |
36 |
60 |
10 |
I2 |
TBHP (2) |
CH3CN |
100 |
36 |
33 |
11b |
I2 |
TBHP (5) |
CH3CN |
100 |
36 |
44 |
12 |
— |
TBHP (5) |
CH3CN |
100 |
36 |
Trace |
13 |
I2 |
— |
CH3CN |
100 |
36 |
0 |
14 |
I2 |
K2S2O8 (2) |
CH3CN |
100 |
36 |
0 |
15 |
I2 |
KHSO5 (2) |
CH3CN |
100 |
36 |
0 |
16 |
I2 |
H2O2 (5) |
CH3CN |
100 |
36 |
0 |
17 |
I2 |
DTBP (5) |
CH3CN |
100 |
36 |
Trace |
18 |
I2 |
TBHP (5) |
DMF |
100 |
36 |
0 |
19 |
I2 |
TBHP (5) |
DMSO |
100 |
36 |
0 |
20 |
I2 |
TBHP (5) |
THF |
100 |
36 |
10 |
21 |
I2 |
TBHP (5) |
DCE |
100 |
36 |
51 |
22 |
I2 |
TBHP (5) |
Toluene |
100 |
36 |
10 |
23 |
I2 |
TBHP (5) |
Dioxane |
100 |
36 |
36 |
24 |
I2 |
O2 |
CH3CN |
100 |
36 |
0 |
In order to generalize the present transformation, we have applied this strategy to various N-arylethylamide derivatives (Table 2). The halogens (Br, Cl & F) present on the para-position of aryl ring attached to the ethylene chain of N-phenethylbenzamide 1a do not affect the yield of the corresponding products (2b–2d). In the case of ortho-methoxy phenyl and pyridyl substituted amides, moderate yields of corresponding products observed (2e and 2f). To extend the scope of the present transformation, we focused on the various groups attached to the carbonyl carbon of N-arylethylamides. The halogen (Br, Cl & F) substituents on the aryl ring irrespective of their position (either o/m/p) provided the corresponding products in good yields (2g–2m). Notably, the strong electron withdrawing groups such as nitro and fluoromethyl substituents at para-position of aryl ring afforded corresponding products in 74% and 76% of yield respectively (2n and 2o). However, the electron donating substituents (Me and OMe) gave comparatively low yields (2p and 2q). Hetero aromatic amides, such as N-phenethylthiophene-2-carboxamide, N-phenethylpicolinamide, N-phenethylisonicotinamide phenethylnicotinamide and N-phenethylisonicotinamide were also reactive under the optimised conditions and provided moderate yields of corresponding oxazoles (2r–2u). Amides like N-phenethylcyclohexanecarboxamide and N-phenethylpivolamide underwent to this procedure smoothly and afford the corresponding oxazoles in good yield (2v and 2w). Finally the present procedure has been successfully applied for a variety of substituted aryl rings containing N-phenethylbenzamides and obtained respective oxazoles in good yields (2x–2z and 2aa–2ae). Aliphatic amides such as N-octylbenzamide and N-decylbenzamide afforded low yield of the desired products 2af, 2ag. As can be seen from the yields and broad range of substituted products of Table 2, including heteroaromatic amides and aliphatic amides, the present protocol indicates its versatile nature.
Table 2 Substrate scope for 2,5-disubstituted oxazolesa
Reaction conditions otherwise stated: 0.2 mmol of 1a, 1.0 mmol of TBHP (5–6 M in decane solution), 20 mol% I2 in 1.0 mL of CH3CN at 100 °C for 36 h. |
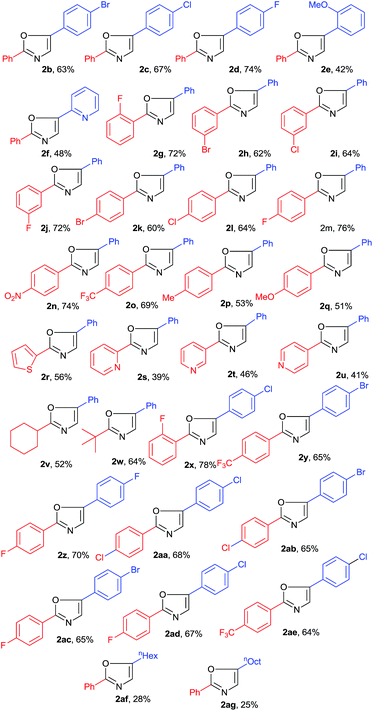 |
To understand the mechanistic path of present transformation we performed some control experiments, in presences of TEMPO as a radical scavenger under the optimised conditions, no desired product was observed and starting material 80% recovered. But the expected intermediate 3 was detected in the reaction mixture by HRMS (ESI S52†). It indicates that, the reaction may proceed through a radical pathway. In the case of N-methyl-N-phenethylbenzamide, 75% of starting substrate was recovered (Scheme 3 (eqn (2))). Further it represents that, free N–H is essential for this transformation.
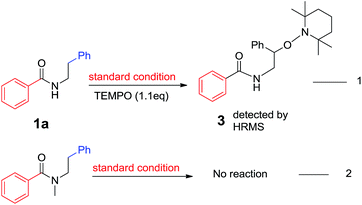 |
| Scheme 3 Mechanistic experiments. | |
On the basis of the above observations and the literature reports,14b,17 a plausible mechanism has been proposed (Scheme 4). Initially the reaction of 1a with iodine generates corresponding N-iodo intermediate A, it undergoes homolytic cleavage in the presence of peroxide and subsequently it generates another intermediate B. Through its 1, 5 proton shift forms C, which in the presence of iodine radical, eliminates the hydroiodic acid and converts to intermediate D. Further, D undergoes addition, substitution and elimination processes (through E and F) in the presence of iodine and provides the final product 2a.
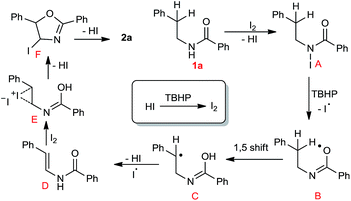 |
| Scheme 4 Proposed mechanism. | |
Conclusions
In conclusion, we have developed a new approach for the synthesis of 2,5-disubstituted oxazoles employing N-arylethylamides with the catalytic amount of iodine and TBHP as an oxidant. Present method is appreciable as it is applicable for wide range of substrates with variety of functional group tolerance under metal-free conditions.
Acknowledgements
CSIR–CSMCRI Communication No. 063/2015. S.S., D. R. R. and M. D. are thankful to AcSIR for their Ph. D. enrolment and the “Analytical Discipline and Centralized Instrumental Facilities” for providing instrumentation facilities. S. S. and M. D. and D. R. R. are also thankful to CSIR and UGC New Delhi, India for their fellowships. We thank DST, Government of India (SR/S1/OC-13/2011), for financial support and CSIR–CSMCRI (OLP–0076) for partial assistance.
Notes and references
-
(a) Oxazoles: Synthesis, Reactions and Spectroscopy, Part B, ed. D. C. Palmer, John Wiley and Sons, Hoboken, NJ, 2004, vol. 60 Search PubMed;
(b) E. Riego, D. Hernandez, F. Albericio and M. Alvarez, Synthesis, 2005, 1907 CrossRef CAS;
(c) D. W. Knight, Oxazole and its Derivatives, in Heterocycles in Natural Product Synthesis, ed. K. C. Majumdar and S. K. Chattopadhyay, Wiley-VCH, Weinheim, 2011 Search PubMed.
- For reviews, see:
(a) Z. Jin, Nat. Prod. Rep., 2011, 28, 1143 RSC;
(b) Z. Jin, Nat. Prod. Rep., 2013, 30, 869 RSC;
(c) M. V. Metelev and D. A. Ghilarov, Mol. Biol., 2014, 48, 29 CrossRef CAS;
(d) P. Comba, N. Dovalil, L. R. Ghan, G. R. Hanson and M. Westphal, Dalton Trans., 2014, 1935 RSC;
(e) B. Axelrod and J. R. Belzile, J. Org. Chem., 1958, 23, 919 CrossRef CAS.
-
(a) J. Linder, T. P. Garner, H. E. L. Williams, M. S. Searle and C. J. Moody, J. Am. Chem. Soc., 1044, 2011, 133 Search PubMed;
(b) T. Doi, M. Yoshida, K. Shin-ya and T. Takahashi, Org. Lett., 2006, 8, 4165 CrossRef CAS PubMed.
- M. Lachia and C. J. Moody, Nat. Prod. Rep., 2008, 25, 227 RSC.
-
(a) Y. Momose, T. Maekawa, T. Yamano, M. Kawada, H. Odaka, H. Ikeda and T. Sohda, J. Med. Chem., 2002, 45, 1518 CrossRef CAS PubMed;
(b) I. E. Andersson, T. Batsalova, B. Dzhambazov, L. Edvinsson, R. Holmdahl, J. Kihlberg and A. Linusson, Org. Biomol. Chem., 2010, 8, 2931 RSC;
(c) P. Raval, M. Jain, A. Goswami, S. Basu, A. Gite, A. Godha, H. Pingali, S. Raval, S. Giri, D. Suthar, M. Shah and P. Patel, Bioorg. Med. Chem. Lett., 2011, 21, 3103 CrossRef CAS PubMed;
(d) W. S. Yang, K. Shimada, D. Delva, M. Patel, E. Ode, R. Skouta and B. R. Stockwell, ACS Med. Chem. Lett., 2012, 3, 35 CrossRef CAS PubMed;
(e) M. Rusch, S. Zahov, I. R. Vetter, M. Lehr and C. Hedberg, Bioorg. Med. Chem., 2012, 20, 1100 CrossRef CAS PubMed;
(f) X. Lu, X. Liu, B. Wan, S. G. Franzblau, L. Chen, C. Zhou and Q. You, Eur. J. Med. Chem., 2012, 49, 164 CrossRef CAS PubMed;
(g) J. Zhou, J. Jin, Y. Zhang, Y. Yin, X. Chen and B. Xu, Eur. J. Med. Chem., 2013, 68, 222 CrossRef CAS PubMed;
(h) M. J. Choi, E. S. No, D. A. Thorat, J. W. Jang, H. Yang, J. Lee, H. Choo, S. J. Kim, J. Lee, H. Choo, S. J. Kim, C. S. Lee, S. Y. Ko, J. Lee, G. Nam and A. N. Pae, J. Med. Chem., 2013, 56, 9008 CrossRef CAS PubMed;
(i) R. E. Thompson, F. Collin, A. Maxwell, K. A. Jolliffe and R. J. Payne, Org. Biomol. Chem., 2014, 12, 1570 RSC.
-
(a) Q. Zhao, S. Liu, Y. Li and Q. Wang, J. Agric. Food Chem., 2009, 57, 2849 CrossRef CAS PubMed;
(b) P. Guo, J.-H. Huang, Q.-C. Huang and X.-H. Qian, Chin. Chem. Lett., 2013, 24, 957 CrossRef CAS PubMed.
-
(a) R. Y. Iliashenko, N. Y. Gorobets and A. O. Doroshenko, Tetrahedron Lett., 2011, 52, 5086 CrossRef CAS PubMed;
(b) F. Mahuteau-Betzer and S. Piguel, Tetrahedron Lett., 2013, 54, 3188 CrossRef CAS PubMed;
(c) J. Min, J. W. Lee, Y.-H. Ahn and Y.-T. Chang, J. Comb. Chem., 2007, 9, 1079 CrossRef CAS PubMed.
-
(a) R. L. Beyer, Y. Singh and D. P. Fairlie, Org. Lett., 2008, 10, 3481 CrossRef CAS PubMed;
(b) J. J. Intemann, E. S. Hellerich, B. C. Tlach, M. D. Ewan, C. A. Barnes, A. Bhuwalka, M. Cai, J. Shinar, R. Shinar and M. Jeffries-El, Macromolecules, 2012, 45, 6888 CrossRef CAS;
(c) O. Grotkopp, A. Ahmad, W. Frank and T. J. Muller, Org. Biomol. Chem., 2011, 9, 8130 RSC.
-
(a) J. Mazuela, A. Paptchikhine, O. Pamies, P. G. Andersson and M. Diéguez, Chem.–Eur. J., 2010, 16, 4567 CrossRef CAS PubMed;
(b) J. Mazuela, P. Tolstoy, O. Pàmies, P. G. Andersson and M. Diéguez, Org. Biomol. Chem., 2011, 9, 941 RSC.
- See for two component reactions for the synthesis of oxazoles:
(a) W. He, C. Li and L. Zhang, J. Am. Chem. Soc., 2011, 133, 8482 CrossRef CAS PubMed;
(b) I. Cano, E. Álvarez, M. C. Nicasio and P. J. Perez, J. Am. Chem. Soc., 2011, 133, 191 CrossRef CAS PubMed;
(c) J. Xie, H. Jiang, Y. Cheng and C. Zhu, Chem. Commun., 2012, 48, 979 RSC;
(d) W.-J. Xue, Q. Li, Y.-P. Zhu, J.-G. Wang and A.-X. Wu, Chem. Commun., 2012, 48, 3485 RSC;
(e) M. Zheng, L. Huang, H. Huang, X. Li, W. Wu and H. Jian, Org. Lett., 2014, 16, 5906 CrossRef CAS PubMed;
(f) Y. Bai, W. Chen, Y. Chen, H. Huang, F. Xiao and G.-J. Deng, RSC Adv., 2015, 5, 8002 RSC;
(g) J. L. Bailey and R. R. Sudini, Tetrahedron Lett., 2014, 55, 367 Search PubMed;
(h) P. Hu, Q. Wang, Y. Yan, S. Zhang, B. Zhang and Z. Wang, Org. Biomol. Chem., 2013, 11, 4304 RSC;
(i) C. Wan, J. Zhang, S. Wang, J. Fan and Z. Wang, Org. Lett., 2010, 12, 2338 CrossRef CAS PubMed;
(j) C. Wan, L. Gao, Q. Wang, J. Zhang and Z. Wang, Org. Lett., 2010, 12, 3902 CrossRef CAS PubMed.
-
(a) I. J. Turchi and M. J. S. Dewar, Chem. Rev., 1975, 75, 389 CrossRef CAS;
(b) I. J. Turchi, Ind. Eng. Chem. Prod. Res. Dev., 1981, 20, 32 CrossRef CAS;
(c) J. Revuelta, F. Machetti and S. Cicchi, in Modern Heterocyclic Chemistry, ed. J. Alvarez-Builla, J. J. Vaquero and J. Barluenga, Wiley-VCH Verlag & Co., Weinheim, Germany, 2011, vol. 2 Search PubMed. For the recent examples of synthetic methods to oxazoles, see:
(d) B. Shi, A. J. Blake, W. Lewis, I. B. Campbell, B. D. Judkins and C. J. Moody, J. Org. Chem., 2010, 75, 152 CrossRef CAS PubMed;
(e) J. P. Weyrauch, A. S. K. Hashmi, A. Schuster, T. Hengst, S. Schetter, A. Littmann, M. Rudolph, M. Hamzic, J. Visus, F. Rominger, W. Frey and J. W. Bats, Chem.–Eur. J., 2010, 16, 956 CrossRef CAS PubMed.
- (i) C–Br bond:
(a) C. Shin, Y. Sato, H. Sugiyama, K. Nanjo and J. Yoshimura, Bull. Chem. Soc. Jpn., 1977, 50, 1788 CrossRef CAS;
(b) J. Das, J. A. Reid, D. R. Kronenthal, J. Singh, P. D. Pansegrau and R. H. Mueller, Tetrahedron Lett., 1992, 33, 7835 CrossRef CAS;
(c) S. K. Chattopadhyay, J. Kempson, A. McNeil, G. Pattenden, M. Reader, D. E. Rippon and D. Waite, J. Chem. Soc., Perkin Trans. 1, 2000, 2415 RSC;
(d) K. Schuh and F. Glorius, Synthesis, 2007, 15, 2297 Search PubMed (ii) C–S bond: N. C. Misra and H. Ila, J. Org. Chem., 2010, 75, 5195 CrossRef CAS PubMed; S. Vijay Kumar, B. Saraiah, N. C. Misra and H. Ila, J. Org. Chem., 2012, 77, 10752 CrossRef PubMed.
-
(a) R. Martin, A. Cuenca and S. L. Buchwald, Org. Lett., 2007, 9, 5521 CrossRef CAS PubMed;
(b) H. Zhou, W.-J. Chung, Y.-H. Xu and T.-P. Loh, Chem. Commun., 2009, 3472 RSC;
(c) L. Yang, C.-H. Lei, D.-X. Wang, Z.-T. Huang and M.-X. Wang, Org. Lett., 2010, 12, 3918 CrossRef CAS PubMed;
(d) Y.-H. Xu, Y. K. Chok and T.-P. Loh, Chem. Sci., 2011, 2, 1822 RSC;
(e) T. Besset, N. Kuhl, F. W. Patureau and F. Glorius, Chem.–Eur. J., 2011, 17, 7167 CrossRef CAS PubMed;
(f) H.-J. Zhang and C. Bolm, Org. Lett., 2011, 13, 3900 CrossRef CAS PubMed;
(g) K. D. Hesp, R. G. Bergman and J. A. Ellman, J. Am. Chem. Soc., 2011, 133, 11430 CrossRef CAS PubMed;
(h) A. E. Wendlandt and S. S. Stahl, Org. Biomol. Chem., 2012, 10, 3866 RSC;
(i) C. W. Cheung and S. L. Buchwald, J. Org. Chem., 2012, 77, 7526 CrossRef CAS PubMed.
-
(a) M. Dinda, S. Samanta, S. Eringathodi and P. K. Ghosh, RSC Adv., 2014, 4, 12252 RSC;
(b) S. R. Bathula, M. P. Reddy, K. K. Durga Rao Viswanadham, P. Sathyanarayana and M. S. Reddy, Eur. J. Org. Chem., 2013, 4552 CrossRef CAS PubMed.
-
(a) C. Hempel and B. J. Nachtsheim, Synlett, 2013, 2119 CAS;
(b) H. A. Samimi and S. Mohammadi, Synlett, 2013, 223 CAS;
(c) Y. Zheng, X. Li, C. Ren, D. Zhang-Negrerie, Y. Du and K. Zhao, J. Org. Chem., 2012, 77, 10353 CrossRef CAS PubMed;
(d) H. Jiang, H. Huang, H. Cao and C. Qi, Org. Lett., 2010, 12, 5561 CrossRef CAS PubMed;
(e) N. Yasmin and J. K. Ray, Synlett, 2009, 2825 CAS;
(f) Y.-M. Pan, F.-J. Zheng, H.-X. Lin and Z.-P. Zhan, J. Org. Chem., 2009, 74, 3148 CrossRef CAS PubMed;
(g) K. Murai, Y. Takahara, T. Matsushita, H. Komatsu and H. Fujioka, Org. Lett., 2010, 12, 3456 CrossRef CAS PubMed;
(h) J. F. Sanz-Cervera, R. Blasco, J. Piera, M. Cynamon, I. Ibáñez, M. Murguía and S. Fustero, J. Org. Chem., 2009, 74, 8988 CrossRef CAS PubMed;
(i) Q.-H. Gao, Z. Fei, Y.-P. Zhu, M. Lian, F. –C. Jia, M.-C. Liu, N. –F. She and A. –X. Wu, Tetrahydron, 2013, 69, 22 CrossRef CAS PubMed.
-
(a) D. C. Mohan, R. R. Donthiri, S. N. Rao and S. Adimurthy, Adv. Synth. Catal., 2013, 355, 2217 CrossRef PubMed;
(b) R. R. Donthiri, V. Pappula, N. N. K. Reddy, D. Bairagi and S. Adimurthy, J. Org. Chem., 2014, 79, 11277 CrossRef CAS PubMed;
(c) D. C. Mohan, S. N. Rao and S. Adimurthy, J. Org. Chem., 2013, 78, 1266 CrossRef PubMed;
(d) D. C. Mohan, Ch. Ravi, S. N. Rao and S. Adimurthy, Org. Biomol. Chem., 2015, 13, 3556 RSC;
(e) D. C. Mohan, S. N. Rao, C. Ravi and S. Adimurthy, Org. Biomol. Chem., 2015, 13, 5602 Search PubMed;
(f) R. D. Patil, G. Joshi and S. Adimurthy, Ind. Eng. Chem. Res., 2010, 49, 8100 CrossRef CAS;
(g) S. Samanta, P. Venkatanarayana, D. Milan and S. Adimurthy, Org. Biomol. Chem., 2014, 12, 9453 RSC.
- See the references in support of mechanism:
(a) T. Dohi, N. Takenaga, A. Goto, A. Maruyama and Y. Kita, Org. Lett., 2007, 9, 3129 CrossRef CAS PubMed;
(b) S. Guyenne, E. I. León, A. Martín, I. Pérez-Martín and E. Suárez, J. Org. Chem., 2012, 77, 7371 CrossRef CAS PubMed;
(c) L. R. Reddy, B. V. S. Reddy and E. J. Corey, Org. Lett., 2006, 8, 2819 CrossRef CAS PubMed;
(d) E. J. Corey and W. R. Hertler, J. Am. Chem. Soc., 1960, 82, 1657 CrossRef CAS;
(e) S. L. Titouania, J. -P. Lavergne, P. Viallefonta and E. Jacquierb, Tetrahedron, 1980, 36, 2961 CrossRef;
(f) S. Tang, Y. Wu, W. Liao, R. Bai, C. Liu and A. Lei, Chem. Commun., 2014, 50, 4496 RSC.
Footnote |
† Electronic supplementary information (ESI) available: Detailed experimental procedures and spectroscopic data. See DOI: 10.1039/c5ra13441b |
|
This journal is © The Royal Society of Chemistry 2015 |
Click here to see how this site uses Cookies. View our privacy policy here.