DOI:
10.1039/C5RA13252E
(Communication)
RSC Adv., 2015,
5, 75263-75267
3,6-Di(pyridin-2-yl)-1,2,4,5-tetrazine capped Pd(0) nanoparticles: a catalyst for copper-free Sonogashira coupling of aryl halides in aqueous medium†
Received
7th July 2015
, Accepted 27th August 2015
First published on 27th August 2015
Abstract
3,6-Di(pyridin-2-yl)-1,2,4,5-tetrazine (pytz) capped Pd(0) nanoparticles (TzPdNPs) as a catalyst in the Sonogashira coupling of aryl halides in aqueous medium with diminished homocoupling is reported. The methodology provides a facile route to obtain polyfunctional alkynes under ligand- and copper-free conditions. The procedure is also efficient for aryl chlorides.
Introduction
The synthesis of new donor–acceptor terminal alkynes or π-conjugated aromatic alkynes has always required demanding starting materials in organic synthesis including natural products.1 Moreover, their recent application in OLEDs as nonlinear optical materials2 and in molecular electronics3 has enhanced the importance of the synthetic route. Sonogashira cross-coupling4 proves to be a most reliable practical tool for this challenging objective in the construction of these alkynes.
Traditionally this cross-coupling of aryl halides and alkynes proceeded in the presence of palladium and copper but it suffered from several limitations like homogeneous catalytic conditions, a higher activity of the palladium-based catalyst5 and the formation of undesired Glaser–Hay homocoupling products.6 A lot of modifications are under process, using other metal co-catalysts,1a,b,h,7 or copper free8 and co-catalyst free7 conditions to diminish self-coupling. Another current challenge associated with the Sonogashira coupling reaction is its inefficiency when “unreactive” aryl chlorides are used. The application of aryl chlorides is advantageous due to their low cost relative to aryl bromides or iodides and increased availability. To date, few methods have been reported involving aryl chlorides in Sonogashira cross-coupling.9 It is also important to remove the expensive ligand choices which are generally used to activate the alkyl halides especially for reactions with chlorides as a coupling partner. Consequently, the search for a new protocol, using a green solvent, which diminishes other side products and activates aryl chlorides without using ligands is quintessential. Recently, a ligand- and copper salt-free Sonogashira reaction in aqueous medium using heterogeneous 10% Pd/C as the catalyst has also been developed but the catalyst is only active with aryl iodides.10 Herein we report the synthesis of 3,6-di(pyridin-2-yl)-1,2,4,5-tetrazine (pytz) capped Pd(0) nanoparticles (TzPdNPs) which are a potential catalyst in the cross-coupling of aryl halides including aryl chloride and aryl acetylenes with diminished homocoupling in aqueous medium using tetrabutyl ammonium bromide (TBAB) as the phase transfer catalyst (PTC).
Results and discussion
The synthesis of TzPdNPs was carried out according to the method reported earlier by us.11 An aqueous solution of Na2PdCl4 was reacted with 3,6-di(pyridin-2-yl)-1,4-dihydro-1,2,4,5-tetrazine (H2pytz) in ethanol under sonication (details in ESI†). In this synthesis 3,6-di(pyridin-2-yl)-1,4-dihydro-1,2,4,5-tetrazine (H2pytz) acts as a reducing agent for Na2PdCl4. On the other hand, H2pytz oxidizes to 3,6-di(pyridin-2-yl)-1,2,4,5-s-tetrazine (pytz) which finally caps the Pd(0) nanoparticles to produce TzPdNPs. The TzPdNPs were characterized using FT-IR, powder X-ray diffraction (PXRD) and transmission electron microscopy (TEM). The crystallinity and purity of the TzPdNPs were examined (Fig. 1a) by the powder X-ray diffraction (XRD) technique. The XRD pattern of the TzPdNPs shows the inter planar d-spacing of XRD peaks corresponding to the (111), (200), (220) and (311) planes of Pd(0) with fcc structure (JCPDS no. 01-1310), without any impurity phase. The XRD pattern of TzPdNPs is similar to that of pure PdNPs, indicating that the nanoparticles have not been affected by the capping with pytz. The fairly intense peaks observed are indicative of crystallinity, whereas, the peak broadening indicates the presence of smaller materials (particle size). The morphology and size distribution of the prepared nanoparticles were elucidated from the transmission electron micrographs. Fig. 1b exhibits a morphological image of the prepared palladium nanoparticles. The size of the particles varies from 1.5 to 3 nm and the average size observed was 2.2 ± 0.4 nm (Fig. S1†). The shape of the particles is almost spherical, showing no faceted surfaces. It can also be noted that, despite the small size, the particles are separated from one another and no significant aggregation is observed. The SAED pattern of the TzPdNPs (Fig. 1c) shows spots and the rings indexed to the (111) and (200) planes of palladium with an fcc structure. This is in agreement with the X-ray diffraction studies. The HRTEM image, depicted in Fig. 1d shows lattice fringes corresponding to the (111) plane of Pd metal. The FT-IR spectrum of the TzPdNPs exhibits all the characteristic bands observed for pytz (Fig. S2†).
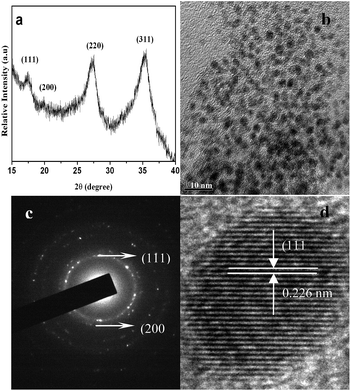 |
| Fig. 1 (a) Powder XRD pattern, (b) TEM image, (c) SAED pattern and (d) HRTEM image of TzPdNPs. | |
We began our investigation with the coupling of phenylacetylene and iodobenzene at room temperature in the presence of 2 mg of catalyst. Initial results revealed that the presence of 2 equiv. of K2CO3 in water afforded the desired coupled product at room temperature with a 94% yield in 2 h (Table 1, entry 1). This preliminary catalytic system was also capable of coupling both bromobenzene and chlorobenzene (Table 1, entries 4 and 5) in high yield. The formation of the desired product was observed with an 86% yield within 3 h for chlorobenzene, although the reaction proceeded at 100 °C. The coupling of bromobenzene was also furnished with an excellent yield of 90% after 3 h at 60 °C.

|
Entry |
X |
Catalyst (mg) |
Time (h) |
Yieldb (%) |
Reaction conditions (unless otherwise specified): 1 (2 mmol), 2 (2 mmol), catalyst (2 mg), TBAB (20 mg), water (10 mL), K2CO3 (2 mmol), room temperature. Yield of the isolated product. Temperature: 60 °C, time: 3 h. Temperature: 100 °C, time: 3 h. |
1 |
I |
2 |
2 |
94 |
2 |
I |
1 |
2 |
72 |
3 |
I |
3 |
2 |
95 |
4 |
Brc |
2 |
3 |
90 |
5 |
Cld |
2 |
3 |
86 |
With a preliminary idea of the limitations of the protocol, we chose the coupling of chlorobenzene and phenylacetylene to further optimize our catalytic system. Various common organic solvents like DMF, DMSO, THF, 1,4-dioxane and toluene were also screened, but they were not as effective as water (Table 2, entries 2–6) and also produced a substantial amount of the undesired homo-coupled product. Utilizing water as the solvent, we next examined the effect of the base in the model coupling of phenylacetylene and chlorobenzene.
Table 2 Optimization of the reaction parametersa

|
Entry |
Catalyst |
Solvent |
Base |
Yieldb (%) |
Reaction conditions (unless otherwise specified): 1 (2 mmol), 2 (2 mmol), catalyst (2 mg), TBAB (20 mg), solvent (10 mL), base (2 mmol), temperature: 100 °C, time: 3 h. Yield of the isolated product. PVP stabilized Pd(0) nanoparticles. |
1 |
TzPdNPs |
H2O |
K2CO3 |
92 |
2 |
TzPdNPs |
DMF |
K2CO3 |
10 |
3 |
TzPdNPs |
DMSO |
K2CO3 |
15 |
4 |
TzPdNPs |
THFc |
K2CO3 |
5 |
5 |
TzPdNPs |
Dioxane |
K2CO3 |
7 |
6 |
TzPdNPs |
Toluene |
K2CO3 |
12 |
7 |
TzPdNPs |
H2O |
EtNH2 |
5 |
8 |
TzPdNPs |
H2O |
Et2NH |
12 |
9 |
TzPdNPs |
H2O |
Et3N |
20 |
10 |
TzPdNPs |
H2O |
Cs2CO3 |
82 |
11 |
PdCl2 |
H2O |
K2CO3 |
Trace |
12 |
Pd(PPh3)2Cl2 |
H2O |
K2CO3 |
Trace |
13 |
Pd(0) NPsc |
H2O |
K2CO3 |
11 |
Several organic bases ranging from primary to tertiary amines afforded poor yields (Table 2, entries 7–9) with a considerable quantity of homo-coupled products. On the other hand, inorganic bases like K2CO3 or Cs2CO3 resulted in good yields (Table 2, entries 1 and 10). We chose K2CO3 as it is a low cost material and also as the product yield is relatively higher. Then we screened different Pd-catalysts like PdCl2, Pd(PPh3)2Cl2 and PVP stabilized Pd(0) nanoparticles.12 However, the reaction did not proceed at all in the presence of PdCl2 and Pd(PPh3)2Cl2 (Table 2, entries 11 and 12). The PVP stabilized Pd(0) (for PXRD, see Fig. S3†) nanoparticles furnished very poor yields (Table 2, entry 13).
With the optimized conditions in hand, at first a variety of aryl chlorides were coupled with aryl alkynes (Scheme 1).
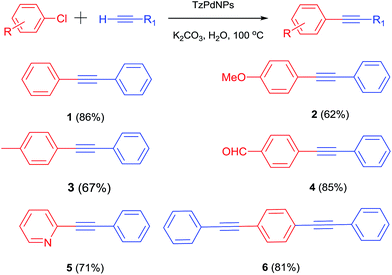 |
| Scheme 1 Sonogashira reactions of aryl chlorides and aryl acetylenes catalyzed by TzPdNPs. Reaction conditions: (1–5) aryl chloride (2 mmol), aryl acetylene (2 mmol), catalyst (2 mg), TBAB (20 mg), water (10 mL), temperature: 100 °C, time: 3 h; (6) aryl chloride (2 mmol), aryl acetylenes (4 mmol). The isolated yields are shown. | |
Phenyl acetylene was coupled with a series of neutral (Scheme 1, 1, 6), electron-rich (Scheme 1, 2, 3), electron-poor (Scheme 1, 4) and heterocyclic chlorides (Scheme 1, 5), in yields ranging from 62% to 95%. With respect to the aryl chlorides, both the electron-neutral and electron-poor aryl chlorides reacted with phenyl acetylene to afford the products in excellent yields. More remarkable was that even the extremely electron-rich aryl chloride 4-chloroanisole (Scheme 1, 2) coupled efficiently with alkynes in the presence of this catalyst. When 1,4-dicholorobenzene was reacted with phenyl acetylene, 1,4-bis(phenylethynyl)benzene (Scheme 1, 6) was obtained almost exclusively irrespective of the ratio of the reactants. Application of trimethylsilyl acetylene under the same conditions predominantly affords the corresponding diaryl acetylenes. These results indicate that the present catalyst system also exhibited high catalytic activity to catalyze the cross-coupling reaction of aryl chlorides with 1-aryl-2-(trimethylsilyl) acetylene via activation of the C–Si bond at high temperature.13
In further experiments to expand the scope of this method, several heterocyclic iodo compounds were coupled with trimethylsilyl acetylene to afford the corresponding 1-aryl-2-(trimethylsilyl) acetylenes (Scheme 2, 1–7) at ambient temperature in aqueous medium. The C–Si bonds in the 1-aryl-2-(trimethylsilyl) acetylenes can be easily hydrolyzed in the presence of KOH in aqueous medium at ambient temperature to produce pyridine, pyrazine, quinoline and quinoxaline based terminal alkynes (Scheme 3, 1–8) in one pot. Pyridine, pyrazine, quinoline, quinoxaline and their derivatives have been widely used as functional building blocks in the fabrication of the π-conjugated host materials for organic light-emitting diodes (OLEDs),14 saccharide recognition,15 nonlinear optical materials,16 solar cells17 and columnar mesophases18 due to their specific optical and electrochemical properties.
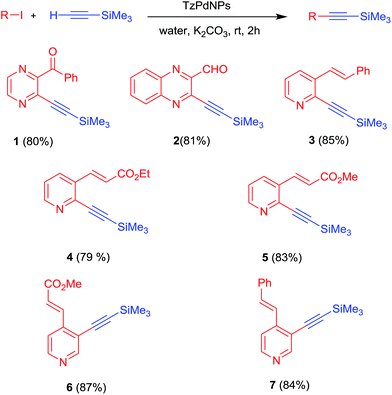 |
| Scheme 2 Sonogashira reactions of heterocyclic iodides and trimethylsilyl acetylene catalyzed by TzPdNPs. Reaction conditions for Sonogashira coupling: heterocyclic iodide (2 mmol), trimethylsilyl acetylene (2 mmol), catalyst (2 mg), TBAB (20 mg), water (10 mL), room temperature, time: 2 h. The isolated yields are shown. | |
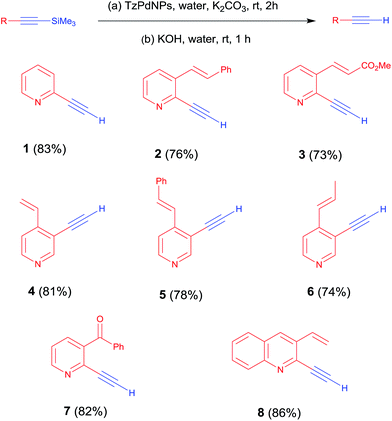 |
| Scheme 3 One pot synthesis of pyridine, pyrazine, quinoline and quinoxaline based terminal alkynes in the presence of KF. Reaction conditions for Sonogashira coupling: heterocyclic iodide (2 mmol), trimethylsilyl acetylene (2 mmol), catalyst (2 mg), TBAB (20 mg), water (10 mL), room temperature, time: 2 h. Reaction conditions for hydrolysis: 1-aryl-2-(trimethylsilyl) acetylenes (2 mmol), KOH (1.0 mmol), water (10 mL), room temperature, time: 1 h. The isolated yields are shown. | |
A plausible mechanism for the reaction is illustrated in Scheme 4, based on the classical description of the Sonogashira reaction.19 The catalytic cycle goes through Pd0/PdII/Pd0 oxidative addition/transmetalation/reductive elimination processes. Initially oxidative addition of the organohalide R–X to the TzPdNPs gives rise to the intermediate I. During the formation of the intermediate I, capping tetrazine (Tz) molecules act as ligands for Pd(II). The subsequent steps are the attachment of the alkyne to the complex I, followed by deprotonation which results in the cis-configured complex (III). Then the acetylenic derivative is formed through reductive elimination, and the active, low oxidation state palladium catalyst is regenerated.
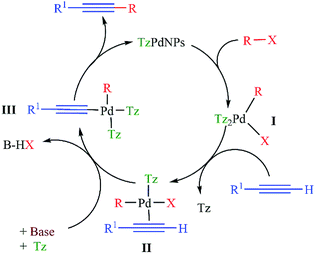 |
| Scheme 4 Proposed mechanism for TzPdNP catalysed Sonogashira reaction. | |
Conclusion
In summary we have developed a 3,6-di(pyridin-2-yl)-1,2,4,5-tetrazine (pytz) capped Pd(0) nanoparticle catalysed efficient procedure for the Sonogashira cross coupling reaction of aryl chlorides with aryl acetylenes in aqueous medium under copper free conditions. Another advantage of the protocol is that the reaction operates at mild conditions for aryl iodides. This protocol is also suitable for the synthesis of terminal alkynes from the reaction of aryl iodides with trimethylsilyl acetylene. In addition, an inert atmosphere is not required to carry out the reaction, offering a simple methodology for the Sonogashira reaction.
Acknowledgements
S. D. and S. S. are indebted to CSIR, India for their Senior Research Fellowship (SRF) [08/003(0072)/2010-EMR-I] and [08/003(0083)/2011-EMR-1], respectively. P. B. acknowledges CSIR-India for the Project (Sanction letter no. 01(2459)/11/EMR-II dated 16/05/2011). The authors also acknowledge the Sophisticated Analytical Instruments Facility (SAIF) at North Eastern Hill University (SAIF-NEHU) for 1H NMR and TEM analysis.
References
-
(a) U. H. F. Bunz, Chem. Rev., 2000, 100, 1605 CrossRef CAS PubMed;
(b) K. C. Nicolaou, P. G. Bulger and D. Sarlah, Angew. Chem., Int. Ed., 2005, 44, 4442 CrossRef CAS PubMed;
(c) T. J. J. Müller and D. M. D’Souza, Pure Appl. Chem., 2008, 80, 609 CrossRef;
(d) M. S. Shiedel, C. A. Briehn and P. J. Baüerle, Organomet. Chem., 2002, 653, 200 CrossRef;
(e) C. Hortholary and C. Coudret, J. Org. Chem., 2003, 68, 2167 CrossRef CAS PubMed;
(f) C. Torborg and M. Beller, Adv. Synth. Catal., 2009, 351, 3027 CrossRef CAS;
(g) R. Chinchilla and C. Najera, Chem. Rev., 2007, 107, 874 CrossRef CAS PubMed;
(h) Z. Novák, A. Szabó, J. Répási and A. Kotschy, J. Org. Chem., 2003, 68, 3327 CrossRef PubMed.
-
(a) H. S. Nalwa and S. Miyata, Nonlinear Optics of Organic Molecules and Polymers, CRC Press, Boca Raton, FL, 1997 Search PubMed;
(b) C.-H. Lee and T. Yamamoto, Tetrahedron Lett., 2001, 42, 3993 CrossRef CAS;
(c) N. Matsumi, K. Naka and Y. Chujo, J. Am. Chem. Soc., 1998, 120, 5112 CrossRef CAS;
(d) G. Wegner and K. Müllen, Electronic Materials, The Oligomer Approach, Wiley-VCH, Weinheim, 1998 Search PubMed;
(e) R. E. Martin and F. Diederich, Angew. Chem., Int. Ed., 1999, 38, 1350 CrossRef;
(f) M. Inouye, K. Takahashi and H. Nakazumi, J. Am. Chem. Soc., 1999, 121, 341–345 CrossRef CAS.
-
(a) J. Suffert and R. Ziessel, Tetrahedron Lett., 1991, 32, 757 CrossRef CAS;
(b) S. H. Chanteau and J. M. Tour, Tetrahedron Lett., 2001, 42, 3057 CrossRef CAS.
-
(a) K. Sonogashira, Y. Tohda and N. Hagihara, Tetrahedron Lett., 1975, 16, 4467 CrossRef;
(b) K. Takahashi, Y. Kuroyama, K. Sonogashira and N. Hagihara, Synthesis, 1980, 627 CrossRef;
(c) J. A. Marsden and M. M. Haley, in Metal-Catalyzed Cross-Coupling Reactions, ed. A. de Meijre and F. Diederich, Wiley-VCH, Weinheim, Germany, 2nd edn, 2004, pp. 317–394 Search PubMed;
(d) K. Sonogashira, in Handbook of Organopalladium Chemistry for Organic Synthesis, ed. E.-I. Negishi, John Wiley & Sons, Inc., Hoboken, N. J., 2002, vol. 1, pp. 493–529 Search PubMed.
- J. Liu, X. Peng, W. Sun, Y. Zhao and C. Xia, Org. Lett., 2008, 18, 3933 CrossRef PubMed.
-
(a) A. S. Hay, J. Org. Chem., 1962, 27, 3320 CrossRef CAS;
(b) C. Glaser, Ann. Chem. Pharm., 1870, 154, 137 CrossRef;
(c) C. Glaser, Ber. Dtsch. Chem. Ges., 1869, 2, 422 CrossRef.
-
(a) A. Molnar, Chem. Rev., 2011, 111, 2251 CrossRef CAS PubMed;
(b) H. Doucet and J.-C. Hierso, Angew. Chem., Int. Ed., 2007, 46, 834 CrossRef CAS PubMed;
(c) R. L. Duncan and J. Macquarrie, Org. Biomol. Chem., 2009, 7, 1627 RSC;
(d) A. Komaromi and Z. Novak, Chem. Commun., 2008, 4968 RSC;
(e) K. Sonogashira, J. Organomet. Chem., 2002, 653, 46 CrossRef CAS;
(f) A. L. K. Shi Shun and R. R. Tykwinski, Angew. Chem., Int. Ed., 2006, 45, 1034 CrossRef PubMed;
(g) V. Farina, Adv. Synth. Catal., 2004, 346, 1553 CrossRef CAS.
-
(a) C. Torborg, J. Huang, T. Schulz, B. Schäffner, A. Zapf, A. Spannenberg, A. Börner and M. Beller, Chem.–Eur. J., 2009, 15, 1329 CrossRef CAS PubMed;
(b) L. Yang, P. Guan, P. He, Q. Chen, C. Cao, Y. Peng, Z. Shi, G. Pang and Y. Shi, Dalton Trans., 2012, 41, 5020 RSC;
(c) V. P. W. Böhm and W. A. Herrmann, Eur. J. Org. Chem., 2000, 3679 CrossRef;
(d) X. Pu, H. Li and T. J. J. Colacot, Org. Chem., 2013, 78, 568 CrossRef CAS PubMed;
(e) C. Yi and R. Hua, J. Org. Chem., 2006, 71, 2535 CrossRef CAS PubMed;
(f) M. K. Samantaray, M. M. Shaikh and P. Ghosh, J. Organomet. Chem., 2009, 694, 3477 CrossRef CAS;
(g) B. H. Lipshutz, D. W. Chung and B. Rich, Org. Lett., 2008, 10, 3793 CrossRef CAS PubMed;
(h) A. Soheili, J. Albaneze-Walker, J. A. Murry, P. G. Dormer and D. L. Hughes, Org. Lett., 2003, 5, 4191 CrossRef CAS PubMed;
(i) Y. Ma, C. Song, W. Jiang, Q. Wu, Y. Wang, X. Liu and M. B. Andrus, Org. Lett., 2003, 5, 3317 CrossRef CAS PubMed;
(j) L. Yang, Y. Li, Q. Chen, Y. Du, C. Cao, Y. Shi and G. Pang, Tetrahedron, 2013, 69, 5178 CrossRef CAS;
(k) A. Gogoi, A. Dewana and U. Bora, RSC Adv., 2015, 5, 16 RSC;
(l) S. Urgaonkar and J. G. Verkade, J. Org. Chem., 2004, 69, 5428 CrossRef PubMed.
-
(a) M.-J. Jin and D.-H. Lee, Angew. Chem., Int. Ed., 2010, 6, 1137 CrossRef;
(b) H. Huang, H. Liu, H. Jiang and K. Chen, J. Org. Chem., 2008, 15, 6037 CrossRef PubMed;
(c) D. Gelman and S. L. Buchwald, Angew. Chem., Int. Ed., 2003, 42, 5993 CrossRef CAS PubMed;
(d) M. R. Eberhard, Z. H. Wang and C. M. Jensen, Chem. Commun., 2002, 8, 818 RSC;
(e) A. Köllhofer, T. Pullmann and H. Plenio, Angew. Chem., Int. Ed., 2003, 42, 1056 CrossRef PubMed;
(f) H. Remmele, A. Köllhofer and H. Plenio, Organometallics, 2003, 22, 4098 CrossRef CAS;
(g) C. A. Fleckenstein and H. Plenio, Organometallics, 2007, 26, 2758 CrossRef CAS;
(h) C. Y. Yi and R. M. Hua, J. Org. Chem., 2006, 71, 2535 CrossRef CAS PubMed;
(i) Y. Liang, Y.-X. Xie and J.-H. Li, J. Org. Chem., 2006, 71, 379 CrossRef CAS PubMed;
(j) S. Protti, M. Fagnoni and A. Albini, Angew. Chem., Int. Ed., 2005, 44, 5675 CrossRef CAS PubMed;
(k) K. Heuzé, D. Méry, D. Gauss, J.-C. Blais and D. Astruc, Chem.–Eur. J., 2004, 10, 3936 CrossRef PubMed;
(l) B. M. Choudary, S. Madhi, N. S. Chowdari, M. L. Kantam and B. Sreedhar, J. Am. Chem. Soc., 2002, 124, 14127 CrossRef CAS PubMed;
(m) W.-B. Yi, C. Cai and X. Wang, Eur. J. Org. Chem., 2007, 3445 CrossRef CAS.
- S. Mori, T. Yanase, S. Aoyagi, Y. Monguchi, T. Maegawa and H. Sajiki, Chem.–Eur. J., 2008, 14, 6994 CrossRef CAS PubMed.
- S. Samanta, S. Das and P. Biswas, Sens. Actuators, B, 2014, 202, 23 CrossRef CAS.
- Y. Li, E. Boone and M. A. El-Sayed, Langmuir, 2002, 18, 4921 CrossRef CAS.
-
(a) U. S. Sorense and E. Pombo-Villar, Tetrahedron, 2005, 61, 2697 CrossRef;
(b) C. Yi and R. Hua, J. Org. Chem., 2006, 71, 2535 CrossRef CAS PubMed.
-
(a) H. Sasabe and J. Kido, Chem. Mater., 2011, 23, 621 CrossRef CAS;
(b) S.-J. Su, H. Sasabe, T. Takeda and J. Kido, Chem. Mater., 2008, 20, 1691 CrossRef CAS;
(c) P. K. Koech, E. Polikarpov, J. E. Rainbolt, L. Cosimbescu, J. S. Swensen, A. L. V. Ruden and A. B. Padmaperuma, Org. Lett., 2010, 12, 5534 CrossRef CAS PubMed;
(d) S. V. Rocha and N. S. Finney, Org. Lett., 2010, 12, 2598 CrossRef CAS PubMed;
(e) C. Huang, C.-G. Zhen, S. P. Su, K. P. Loh and Z.-K. Chen, Org. Lett., 2005, 7, 391 CrossRef CAS PubMed;
(f) T. Lifka, A. Oehlhof and H. Meier, J. Heterocycl. Chem., 2008, 45, 935 CrossRef CAS.
- H. Abe, H. Machiguchi, S. Matsumoto and M. Inouye, J. Org. Chem., 2008, 73, 4650 CrossRef CAS PubMed.
-
(a) Y.-X. Yan, X.-T. Tao, Y.-H. Sun, C.-K. Wang, G.-B. Xu, J.-X. Yang, Y. Ren, X. Zhao, Y.-Z. Wu, X.-Q. Yua and M.-H. Jiang, J. Mater. Chem., 2004, 14, 2995 RSC;
(b) H. Wang, Z. Li, P. Shao, Y. Liang, H. Wang, J. Qin and Q. Gong, New J. Chem., 2005, 29, 792 RSC;
(c) A. Abbotto, L. Beverina, R. Bozio, A. Facchetti, C. Ferrante, G. A. Pagani, G. Pedron and R. Signorini, Chem. Commun., 2003, 2144 RSC;
(d) P. Poornesh, P. Hegde, M. Manjunatha, G. Umesh and A. Adhikari, Polym. Eng. Sci., 2009, 49, 875 CrossRef CAS.
-
(a) Y. Ooyama, T. Nagano, S. Inoue, I. Imae, K. Komaguchi, J. Ohshita and Y. Harima, Chem.–Eur. J., 2011, 17, 14837 CrossRef CAS PubMed;
(b) Y. Ooyama, S. Inoue, T. Nagano, K. Kushimoto, J. Ohshita, I. Imae, K. Komaguchi and Y. Harima, Angew. Chem., Int. Ed., 2011, 50, 7429 CrossRef CAS PubMed;
(c) Y. Ooyama, S. Inoue, R. Asada, G. Ito, K. Kushimoto, K. Komaguchi, I. Imae and Y. Harima, Eur. J. Org. Chem., 2010, 92 CrossRef CAS.
-
(a) A.-J. Attias, C. Cavalli, B. Donnio, D. Guillon, P. Hapiot and J. Malthete, Chem. Mater., 2002, 14, 375 CrossRef CAS;
(b) A.-J. Attias, C. Cavalli, B. Donnio, D. Guillon, P. Hapiot and J. Malthete, Mol. Cryst. Liq. Cryst., 2004, 415, 169 CrossRef CAS.
- M. García-Melchor, M. C. Pacheco, C. Nájera, A. Lledós and G. Ujaque, ACS Catal., 2012, 2, 135 CrossRef and references therein.
Footnote |
† Electronic supplementary information (ESI) available: Experimental procedures, 1H NMR data and spectra. See DOI: 10.1039/c5ra13252e |
|
This journal is © The Royal Society of Chemistry 2015 |