DOI:
10.1039/C5RA12925G
(Paper)
RSC Adv., 2015,
5, 80027-80038
Design, synthesis and biological evaluation of novel unsymmetrical azines as quorum sensing inhibitors†
Received
3rd July 2015
, Accepted 4th September 2015
First published on 4th September 2015
Abstract
Targeting quorum sensing signals using quorum sensing inhibitors has opened new avenues for the application of known antibiotics. In this context, twenty five unsymmetrical azines were synthesised and evaluated as quorum sensing inhibitors. An efficient one-pot procedure was adopted that directly links 3-methyl-2-(methylthio)benzo[d]thiazol-3-ium salt, hydrazine hydrate and substituted aldehyde to give the designed compounds. The synthesized compounds were preliminarily tested for their potential to inhibit CviR receptor based QS signals in Chromobacterium violaceum. The bioassay screening results suggested that two compounds exhibited potent QS inhibition activity against CviR receptor, showing violacein inhibition (>50%) at 200 μM. Further, the putative positive hits were checked for their potential to inhibit LasR receptor-based QS using the PlasB-gfp(ASV) biomonitor strain of Pseudomonas aeruginosa. These compounds were found to inhibit the QS-mediated GFP signals in a dose dependant manner. Two active compounds also exhibited biofilm clearance at 50 μM concentration. Docking studies were performed to examine their potential to bind to the LasR protein of Pseudomonas aeruginosa.
Introduction
The mortality rate due to bacterial infection is increasing annually, making it a major area of focus for researchers.1 In this direction, many antibacterial compounds have been studied but in most cases the bacteria develop resistance, either due to modification of a single amino acid at the target site, increased activity of enzymes that are capable of degrading antimicrobial compounds,2 or biofilm formation.3
Gram-negative bacteria communicate with each other by releasing chemical signals termed as autoinducers and this process of cell to cell communication is known as ‘quorum sensing’.4 This communication occurs by the release of various chemical signals, such as N-acyl-L-homoserine lactones (AHLs), to monitor and coordinate their genome expression in a cell density-dependent manner.5–7 These AHLs consist of homoserine lactone rings attached via amide bonds to alkyl/acyl chains containing 4 to 18 carbons (Fig. 1). The invading pathogen has to reach a critical cell population density, sufficient to overcome the host defences, for the development of infection. As the cell density increases, the concentration of these signal molecules increases and upon reaching a threshold level (when the population is “quorate”), the population activates a cellular response.8 Quorum sensing controls the expression of virulence factors, which includes the secretion of extracellular proteases, pectinase and biosurfactant, as well as the formation of a biofilm.9 The frequent occurrence of resistant pathogenic strains gradually decreases the effectiveness of traditional antibiotic treatment; hence, there is an urgent need to develop a new class of molecules to target quorum sensing.
 |
| Fig. 1 N-Acyl-L-homoserine lactones (AHLs) from various Gram-negative bacteria. | |
Pseudomonas aeruginosa is a ubiquitous Gram-negative bacterium that is responsible for many opportunistic, nosocomial10 and chronic infections. It is the major cause of infection in cystic fibrosis patients,11 AIDS patients,12 burn victims13 and neutropenic cancer patients.14 Pseudomonas aeruginosa has three main QS systems: the first two systems are LasR-LasI15,16 and RhlR-RhlI,17,18 which use Acyl Homoserine Lactones (AHLs) as signal molecules. Both systems together regulate virulence factors. The third QS system is known as PQS (Pseudomonas Quinolone Signal), which consists of PqsR as a virulence regulator and uses another kind of signalling molecule, 2-heptyl-3-hydroxy-4-quinolone (HHQ).19–22
Many AHL analogues have been reported as QS inhibitors, most of them belonging to lactone, thiolactone and furanone classes (Fig. 2).23–26 These AHL analogues have inherent limitations as QS inhibitors due to (i) rapid degradation in the presence of mammalian lactonases,27,28 (ii) high specificity towards their cognate receptors, which makes these compounds autoinducers,29 (iii) the possible toxicity of the breakdown products of AHL analogues, etc.30 Recently, already known drugs, viz. salicylic acid (13), nifuroxazide (14) and chlorzoxazone (15), have been identified as Pseudomonas aeruginosa quorum-sensing inhibitors (LasR) through a structure-based virtual screening approach.31 Hartman et al. have reported PqsR antagonists (16a and 16b)32 which inhibit the third QS system, i.e. PqsR, in Pseudomonas aeruginosa (Fig. 3).
 |
| Fig. 2 Quorum-sensing inhibitors derived from Acylated Homoserine Lactones (AHLs). 5–8 belong to the lactone class, 9–11 belong to the furanone class and 12 belongs to the thiolactone class. | |
 |
| Fig. 3 Salicylic acid (13), nifuroxazide (14) and chlorzoxazone (15) as Pseudomonas aeruginosa quorum sensing inhibitors; PqsR antagonists (16a and 16b) and designed azines 17a–y. | |
Inspired by nifuroxazide (14) and considering the limitations of analogues of AHL, we have designed unsymmetrical azines (17a–y) as a structurally unrelated class of QS inhibitors (Fig. 3 and 4). Azines are a functional class of organic compounds with the general formula R1R2C
N–N
CR3R4. Unsymmetrical azines contain different substituents on the two sides of the C
N–N
C frame. Azine derivatives have been studied for antibacterial,33 antifungal,34 antifilarial,35 anticancer,36 opiate antagonist37 and molluscacidal38 activities. Thus, the azine class of compounds can be considered as safe for the development of drug candidates. However, its other derivatives, such as hydrazine, azo and hydrazide, were reported in literature as having a reactive functional group and thus should be avoided during drug discovery.39 Apart from biological activity, tautomerism in azines is also reported.40 The majority of the azines reported in the literature are symmetric ones, which can be obtained by dimerization. In this report, the focus is on the synthesis of unsymmetrical azines, which cannot follow a dimerization approach. Azines 17a–y were prepared by simple and efficient methods (method A and method B) in moderate to good yields. These azines were screened against two Gram-negative strains, viz. Chromobacterium violaceum and Pseudomonas aeruginosa. All compounds were initially tested for their potential to inhibit CviR receptor-based QS in Chromobacterium violaceum. The bioassay screening results suggested that two compounds, viz. 17j and 17n, exhibit moderate to good QS inhibition activity against the CviR receptor. These hits were found to have potential to inhibit LasR receptor-based QS-mediated GFP production in a dose dependant manner. Compounds 17j and 17n significantly resulted in biofilm clearance. Finally, docking analysis was carried out to understand the binding mode and the interactions within the active site of the LasR protein.
 |
| Fig. 4 Design strategy for unsymmetrical azines (17a–y). | |
Results and discussion
Design of unsymmetrical azines as LasR antagonists
From the knowledge of the interactions of the natural bound ligand (OdDHL) to the AHL binding domain in LasR, a pharmacophore model for OdDHL was developed by Taboureau et al.41 The five-point pharmacophore model suggested that the lactone ring constitutes an aromatic box, the amide linkage constitutes a polar box and the carbon chain constitutes a hydrophobic box (Fig. 4). This model suggests that in order to interact with the AHL binding domain, a ligand must possess an aromatic box and hydrophobic box linked by a polar region. Thus, while designing novel QS inhibitors targeting LasR we proposed unsymmetrical azines, which contain a benzothiazole nucleus and an aryl moiety linked by azine as a spacer (Fig. 4). We hypothesized that the benzothiazole nucleus may form the hydrophobic region, the azine may represent the polar region and the aryl moiety may form the aromatic box and may show favourable interactions with the amino acids in the AHL binding domain. To verify this hypothesis, docking studies of the designed azines were carried out and compared with OdDHL. Docking studies showed that the benzothiazole nucleus occupies hydrophobic region, whereas the azine spacer is important for hydrogen bond interactions with the amino acids in the AHL binding domain. Docking studies revealed that the designed azines showed comparable interactions and docking score with that of OdDHL.
Chemistry
We have carried out the synthesis of 17a–y by two different methods, method A and method B (Schemes 1 and 2). Azines 17a–v were synthesized by adopting Scheme 1.
 |
| Scheme 1 Reaction and conditions: (i) CH3I, K2CO3, DMF, rt, 30 min, 80%; (ii) CH3I, 50–60 °C, 12 h, 65%; (iii) NH2NH2·H2O, TEA, MeCN, rt, 2 h; (iv) MeOH, 1 N HCl, 50 °C, 30 min, 90%; (v) RCHO, ethanol, reflux, 10–12 h, 65–95%; (vi) 20, NH2NH2·H2O, DMF, rt, 3 h → RCHO, ethanol, reflux, 12 h (60–80%). | |
 |
| Scheme 2 Reaction and conditions: (i) CH3I, K2CO3 DMF, rt, 30 min, 80%; (ii) HNO3, H2SO4, (0–10 °C), 75%; (iiia) CH3I, 50 °C, 12 h; (iiib) (CH3)2SO4, 180 °C, 90 min; H2O, KPF6, rt, 5 min; (iv) NH2NH2·H2O, TEA, MeCN, rt, 3 h, 62%; (iv) MeOH, 1 N HCl, 50 °C, 30 min, 90%; (v) RCHO, ethanol, reflux, 10–12 h, 60–70%; (vii) 21d, NH2NH2·H2O, DMF, rt, 2 h → RCHO, ethanol, reflux, 12 h (60–75%). | |
The synthesis starts with readily available 2-mercaptobenzothiazole (18a)/5-methoxy-2-mercaptobenzothiazole (18b), which on treatment with methyl iodide get converted to 19a/19b.42 Compound 19a and 19b were converted to their iodide salts 20a and 20b in moderate yield (∼65%) by heating with methyl iodide for 24 h.43,44 Compound 20 is a common intermediate for the synthesis of azines 17a–v using method A and method B. Intermediates 20a and 20b were reacted with hydrazine hydrate in the presence of triethylamine as a base to afford hydrazones 21a and 21b.45 Since these hydrazones were degrading during work up, they were converted to their hydrochloride salts (22a and 22b), which upon coupling with substituted aldehydes gave the desired azines 17a–v.46
Unsymmetrical azines 17w–y have the nitro substituent at R2 and were prepared by Scheme 2. The synthetic scheme starts with the nitration of 19a by using H2SO4/HNO3 as a nitrating mixture to give 20.47,48 Methylation of 20 with methyl iodide afforded compound 21c with poor yield (<20%); thus, methylation of 20 was carried out by changing the methylating agent to dimethyl sulphate. Compound 20 was heated with dimethyl sulphate and the product was precipitated with potassium hexafluorophosphate45 to get 80% yield of 21d. Finally, compounds 17w–y were prepared by the same methods A and B given in Scheme 2.
The hydrazone intermediate (21a, 21b and 22d) in method A suffers from several drawbacks, such as cumbersome work-up procedure, hydrolytic instability and thus difficulty in isolation. To circumvent this, we developed method B, in which the salt (20a/20b/21d) was treated with hydrazine hydrate in DMF at room temperature for 3 h. This leads to the in situ generation of the hydrazone. After 3 h, substituted aldehydes and ethanol were added to the reaction mixture and allowed to reflux for 6–12 h to get the desired azines 17a–17y (Table 1). The reaction mixture was allowed to cool and then filtered to get the crude product. The desired products were purified by silica gel column chromatography and obtained in moderate to good yields. The synthesized compounds were characterized by means of 1H NMR, 13C NMR and HRMS (+ESI).
Table 1 Unsymmetrical azines 17a–y and CviR inhibition at 200 μM concentration
Biology
QS inhibition activity against CviR receptor. Chromobacterium violaceum is a Gram-negative bacterium that produces the purple pigment violacein in response to the presence of the AHL N-hexanoyl homoserine lactone (C6-HSL). The production of violacein is regulated by acyl HSL-mediated QS. Thus, inhibition of the HSL-mediated QS system leads to a decrease in violacein production.49 In order to see the effect of the synthesised azines 17a–y on the HSL-mediated QS system, they were preliminarily checked against CviR receptor-based QS in Chromobacterium violaceum. The bioassay screening results (Table 1) suggested that compounds 17j and 17n exhibit QS inhibition against the CviR receptor. They have shown more than 50% inhibition of purple pigment violacein production by Chromobacterium violaceum (CV12472) (Fig. 5). A known QS inhibitor, 4-nitropyridine-N-oxide (4-NPO) was also included at 200 μM as a positive control. The inhibitory effect of the DMSO control (0.2%) was examined in a similar fashion. The two active compounds (17j and 17n) do not possess any substitution on the benzene ring of benzothiazole. Substitution at C5/C6 of benzothiazole with an electron donating group (OMe) or electron withdrawing group (NO2) decreases the percentage inhibition. This means that the benzothiazole nucleus cannot tolerate either electron donating (OMe) or withdrawing (NO2) groups at the C5/C6 position. In addition, hydrophobic groups (OMe, F, Cl) on the benzene ring (17d–g) lead to a decrease in activity. When R is a heterocyclic nucleus, different patterns of inhibition are observed. An inhibition of >50% was observed for 17j and 17n, which contain chloroquinoline and an imidazole nucleus as the R group, respectively.
 |
| Fig. 5 The effects of synthesized unsymmetrical azines (17a–17y) on violacein production by Chromobacterium violaceum CV12472 were tested in a liquid bioassay. Violacein was extracted as described and quantified by absorbance measurements at 568 nm. Compounds were used at 200 μM concentration. DMSO-treated cultures were used as a negative control (0.2%). A known QSI, 4-NPO (+ve control) was also included in this experiment. On comparing the extent of violacein production as compared to the control condition, it was found that compounds 17j and 17n showed good activity. | |
Effect of 17j and 17n on QS-mediated GFP production in PlasB-gfp(ASV). The two azines, 17j and 17n, were assayed against Pseudomonas aeruginosa MH602 lasB reporter strain PlasB-gfp(ASV) following the protocol described by Givskov et al., with few modifications. AHL signal production by this reporter strain leads to an increase in unstable green fluorescent protein (GFP) production as a function of the QS system. Compounds that inhibits bacterial QS systems reduce the expression of GFP. The dose-dependant effect of the compounds was checked in the concentration range 5.0–75 μM as shown in Fig. 6. Compounds 17j and 17n resulted in a reduction in GFP production as compared to the DMSO control condition, without significant effect on the growth of the organism.
 |
| Fig. 6 QS inhibition assay of compounds 17j and 17n for QS-mediated GFP production in Pseudomonas aeruginosa MH602 lasB reporter strain (PlasB-gfp(ASV)). (A) OD as a function of time. (B) RFU (Relative Fluorescence Units) as a function of time. These compounds reduce the RFU level (GFP fluorescence units divided by OD450) at various concentrations (right), indicating inhibition of LasR, while displaying no effect on growth (left). Growth is displayed as an increase in OD450 compared to the initial level. Results are representative of three independent experiments. The final concentration of DMSO in the experiments is 0.2% and an equivalent concentration was used as a negative control. | |
Antibiofilm activity. The QS system in Pseudomonas aeruginosa plays a central role in regulating virulence and biofilm formation. Biofilms make the organism resistant to all known antimicrobial agents, making pseudomonal infections complicated and life threatening. To elucidate whether the active azines inhibit QS-mediated biofilm formation or not, we checked the effect of 17j and 17n on Pseudomonas aeruginosa MH602 lasB reporter strain PlasB-gfp(ASV). For this study, biofilms were developed on microscope cover slips. Three-day-old biofilms on analysis have shown that the two compounds 17j and 17n have significantly reduced the biofilm formation as compared to the DMSO control condition, where fully matured biofilms were formed (Fig. 7).
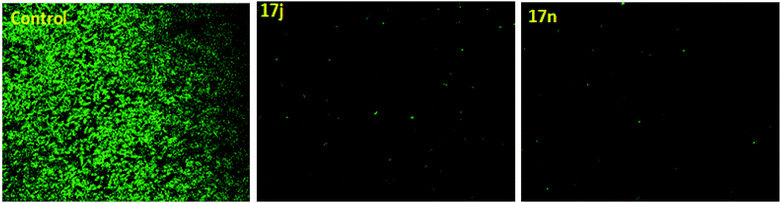 |
| Fig. 7 Inhibition of OdDHL-mediated signalling in Pseudomonas aeruginosa biofilm carrying plasB-gfp(ASV). (a) Three-day-old mature biofilm in the presence of an equivalent amount of DMSO; (b) inhibition of biofilm in the presence of compound 17n at 50 μM; (c) biofilm inhibition by compound 17j at 50 μM concentration. The final concentration of DMSO in the experiments is 0.2% and an equivalent concentration was used as a negative control. Biofilms were visualized at 60× using a Nikon Confocal Microscope and images were analyzed using NIS-Elements software. | |
Molecular docking analysis. Molecular docking studies have been carried out in order to understand the nature of the interactions between the unsymmetrical azines (17j and 17n) and the QS receptor. The LasR structure contains four monomers of the ligand binding domain, each complexing with one OdDHL ligand. The active binding site is conserved between the β-sheet and the external α-helical face made up of α3, α4 and α5 where the natural ligand OdDHL binds. The docking results suggested that the hydrogen bonding interaction and hydrophobic interactions with the amino acid residues Ser 129, Tyr 56, Trp 60 and Tyr 47 play an important role in the binding to the OdDHL binding site. The imine nitrogen (C
N) of both the azines (17j and 17n) shows hydrogen bonding with Ser 129 (1.4–2.4 Å) and the benzothiazole nucleus occupies the hydrophobic region by forming π–π stacking with Tyr 56 and Trp 60 (Fig. 8). The docking pose of 17j perfectly overlaps with the natural ligand, OdDHL (Fig. 8). However, these azines are not analogues of OdDHL, but they bear pharmacophoric features present in OdDHL. For example, the benzothiazole nucleus in the active azines overlaps with the hydrophobic acyl chain in OdDHL and thus forms hydrophobic interactions the same as OdDHL. The azine spacer –C
N–N
C– overlaps with the –CONH, constituting the polar region, and the aryl moiety overlaps with the lactone ring of OdDHL, constituting the aromatic box, and binds to the same region where the lactone ring of OdDHL binds. Thus, these azines fit very well in the active site and hence inhibit the QS system.
 |
| Fig. 8 Docking pose of active benzothiazole-hydrazone azines 17j and 17n in the Pseudomonas aeruginosa LasR ligand binding domain. (A) Representation of the overlapping of the natural ligand OdDHL (orange) with 17j (green). (B and C) Representations of the binding of 17j and 17n (green), respectively, with the LasR ligand binding domain. The black dotted line (…) represents the hydrogen bond interaction with the amino acid (yellow) in the vicinity of the active site. | |
Conclusions
Twenty five unsymmetrical azines (17a–y) were synthesised, biologically evaluated and docked into the LasR receptor to study the binding interactions, such as hydrogen bonding and hydrophobic interactions. Here, we have described an efficient one-pot synthesis of azines (17a–y) by coupling 3-methyl-2-(methylthio)benzo[d]thiazol-3-ium (20a/20b/21d), hydrazine hydrate and a substituted aldehyde. Initially, biological assays (QS inhibition) were performed using the Chromobacterium violaceum CV12472 strain. Two azines (17j and 17n) showed (>50%) QS inhibitory activity at 200 μM concentration. These active azines were further found to inhibit LasR receptor-based QS, which has been checked using a plasB-gfp(ASV)-based bioassay. This study is also supported by the results of the molecular docking study, which shows that these azines bind to the ligand binding domain of the LasR protein. Azines 17j and 17n have shown significant biofilm inhibition for Pseudomonas aeruginosa at a final concentration of 50 μM. Thus, these azines can be considered as hits for the development of potential quorum sensing inhibitors, which could be used in conjugation with known antibiotics to curb the pathogenesis of Pseudomonas aeruginosa.
Experimental
Chemistry
The reagents and chemicals required for the study were procured from Sigma-Aldrich (St. Louis, MO, USA) and Alfa Aesar (Johnson Matthey Company, Ward Hill, MA, USA). All the reagents were used without further purification unless otherwise mentioned. The progress of the reaction was monitored by Thin Layer Chromatography (TLC) performed on silica gel aluminium plates and visualization was carried out with UV light. 1H NMR and 13C NMR spectra were recorded on a Bruker Avance DX spectrometer at 400 and 100 MHz respectively, with TMS as an internal standard. The 1H NMR and 13C NMR was recorded for CDCl3 at 7.26 ppm and 77.00 ppm respectively. The 1H NMR and 13C NMR was recorded for DMSO-d6 at 2.50 ppm and 39.51 ppm respectively. Chemical shifts (δ) are reported in parts per million (ppm). Coupling constants (J) are reported in hertz (Hz). The abbreviations used to characterize the signals are as follows: s = singlet, m = multiplet, d = doublet, dd = doublet of doublets, dt = doublet of triplets, t = triplet and q = quartet. Mass spectra were measured with High Resolution Mass Spectroscopy (HRMS). Melting points were determined with an electro-thermal melting point apparatus.
2-(Methylthio)benzo[d]thiazole (19a). 2-Mercaptobenzthiazole (5.00 g, 29.94 mmol) (18a) was dissolved in DMF (2 mL) and to the reaction mixture K2CO3 (0.5 g, 119.76 mmol) was added. The reaction mixture was allowed to stir for a few minutes then CH3I (1.77 mmol) was added dropwise at lower temperature (0–10 °C). The reaction mixture was stirred at room temperature for 1 h. The completion of the reaction was monitored by TLC. The reaction solvent was removed by rotary evaporation and the product was diluted with water and extracted with ethyl acetate (3 × 70 mL). The organic layer was concentrated to give the desired product as a yellow liquid (4.30 g, 80%). 1H NMR (400 MHz, CDCl3) δ 2.77 (s, 3H, SCH3), 7.27 (t, J = 8 Hz, 1H, ArH), 7.41 (t, J = 8 Hz, 1H, ArH), 7.72 (d, J = 8 Hz, 1H, ArH), 7.88 (d, J = 8 Hz, 1H, ArH); 13C NMR (100 MHz, CDCl3) δ 15.95, 120.65, 121.38, 124.10, 126.08, 135.16, 153.37, 168.10; MALDI m/z calculated for C8H7NS2 181.002, found: 182.05 [M + H]+.
5-Methoxy-2-(methylthio)benzo[d]thiazole (19b). 5-Methoxybenzo[d]thiazole-2-thiol (18b) (5.00 g, 25.30 mmol) was dissolved in DMF (10 mL) and to the mixture K2CO3 (14.01 g, 101.52 mmol) was added. The reaction mixture was allowed to stir for a few minutes then CH3I (10.70 g, 75.90 mmol) was added dropwise at lower temperature. The reaction mixture was stirred at room temperature for 30 min (monitored by TLC). The solvent was removed by rotary evaporation and the product was diluted with water and extracted with ethyl acetate (3 × 70 mL). The organic layer was filtered through a bed of Na2SO4 and concentrated to afford the desired product as a brown crystalline solid (5.08 g, 95%).1H NMR (400 MHz, CDCl3) δ 2.80 (s, 3H, –SCH3), 3.87 (s, 3H, –OCH3), 6.95 (dd, J = 11.2 Hz, 2.4 Hz, 1H, ArH), 7.41 (d, J = 2.4 Hz, 1H, ArH), 7.60 (d, J = 8.7 Hz, 1H, ArH); 13C NMR (100, MHz, CDCl3) δ 15.98, 55.62, 104.50, 113.78, 121.14, 126.78, 154.62, 158.96, 169.27; MALDI m/z calculated for C9H9NOS2 211.0126, found 212.022 [M + H]+.
3-Methyl-2-(methylthio)benzo[d]thiazol-3-ium iodide (20a). Thioether (19a) (4.30 g, 23.70 mmol) was heated with CH3I (13.39 g, 95.02 mmol) at 50–60 °C for 12 h. After completion of the reaction the yellow salt was washed with diethyl ether to remove unreacted thioether (4.97 g, 65%). 1H NMR (400 MHz, CDCl3) δ 3.16 (s, 3H, –SCH3), 4.18 (s, 3H, –NCH3), 7.75 (t, J = 8 Hz, 1H, ArH), 7.87 (t, J = 8 Hz, 1H, ArH), 8.11 (d, J = 8 Hz, 1H, ArH), 8.26 (d, J = 8 Hz, 1H, ArH); 13C NMR (100 MHz, CDCl3) δ 17.24, 35.61, 115.11, 123.30, 127.10, 128.51, 129.38, 142.79, 181.85; MALDI m/z calculated for C9H10NS2 196.0255, found 196.085 [M]+.
5-Methoxy-3-methyl-2-(methylthio)benzo[d]thiazol-3-ium iodide (20b). Thioether (19b) (5.08 g, 24.07 mmol) was heated with CH3I (13.57 g, 96.28 mmol) at 50–60 °C for 12 h. After completion of the reaction the yellow salt was washed with diethyl ether to remove unreacted thioether. The product obtained was a yellow solid (5.36 g, 63%). 1H NMR (400 MHz, DMSO-d6): δ 3.09 (s, 3H, –SCH3), 3.95 (s, 3H, –NCH3), 4.09 (s, 3H, –OCH3), 7.34 (dd, J = 11.36 Hz, 2.3 Hz, 1H, ArH), 7.71 (d, J = 2.32 Hz, 1H, ArH), 8.22 (d, J = 9 Hz, 1H, ArH); 13C NMR (400 MHz, DMSO-d6): δ 18.49, 37.00, 56.93, 99.97, 116.68, 120.44, 125.95, 144.52, 161.06, 181.65; MALDI m/z calculated for C10H12NOS2 226.036, found 226.07 [M]+.
2-(Methylthio)-6-nitrobenzo[d]thiazole (20). Compound 19a (5.0 g, 22.22 mmol) was dissolved in 5 mL of H2SO4 and a mixture of H2SO4 (1.09 g, 11.22 mmol) and HNO3 (0.55 g, 8.88 mmol) was slowly added while cooling the reaction mixture at 0 °C. The solution was stirred in the cold for 1 h. The solution was then poured onto ice, the precipitate formed was filtered, then washed with water and aqueous ammonia solution to get a crude yellow solid. The crude compound was purified by column chromatography over silica gel using 5% ethyl acetate–hexane as eluent to give a yellow solid (4.66 g, 75%). 1H NMR (400 MHz, CDCl3) δ 2.85 (s, 3H, –SCH3), 7.91 (d, J = 8.9 Hz, 1H, ArH), 8.31 (dd, J = 11.2 Hz, 2.3 Hz, 1H, ArH), 8.69 (d, J = 2.28 Hz, 1H, ArH); 13C NMR (100 MHz, CDCl3) δ 16.0, 117.41, 121.19, 121.95, 135.57, 144.03, 157.10, 175.11; MALDI m/z calculated for C8H6N2O2S2 225.987, found 225.950 [M + H]+.
(Z)-2-Hydrazono-3-methyl-2,3-dihydrobenzo[d]thiazole (21a). Salt (20a) (4.97 g, 15.4 mmol) was dissolved in acetonitrile and to the reaction mixture a solution of triethylamine (3.11 g, 30.86 mmol) in acetonitrile was added followed by a solution of hydrazine hydrate (7.7 g, 154 mmol) in acetonitrile. The reaction mixture was stirred for 2 h. The completion of the reaction was monitored by TLC. The solvent was removed by rotary evaporation and the product was diluted with water and extracted with ethyl acetate (3 × 70 mL). The organic layer was filtered through a bed of Na2SO4 and concentrated to afford the crude product as a solid. The crude compound was purified by column chromatography over silica gel using 10% ethyl acetate–hexane as eluent to yield a white crystalline solid (1.96 g, 72%). 1H NMR (400 MHz, CDCl3) δ 3.51 (s, 3H, –NCH3), 6.96 (d, J = 8 Hz, 1H, ArH), 7.02 (t, J = 8 Hz, 1H, ArH), 7.25 (t, J = 8 Hz, 1H, ArH), 7.39 (d, J = 8 Hz, 1H, ArH); 13C NMR (100 MHz, CDCl3) δ 31.95, 108.60, 121.04, 122.13, 124.62, 125.91, 141.31, 160.62, 163.87; MALDI m/z calculated for C8H9N3S 179.051, found 179.079 [M]+.
(Z)-2-Hydrazono-5-methoxy-3-methyl-2,3-dihydrobenzo[d]thiazole (21b). Salt (20b) (5.08 g, 14.3 mmol) was dissolved in acetonitrile and to the reaction mixture, triethylamine (2.89 g, 28.7 mmol) was added followed by a solution of hydrazine hydrate (7.15 g, 143.0 mmol) in acetonitrile. The reaction mixture was stirred for 2 h. The completion of the reaction was monitored by TLC. The product was diluted with water and extracted with ethyl acetate (3 × 70 mL) and then washed with 1 N HCl three times. The crude compound was purified by column chromatography over silica gel using 5% ethyl acetate–hexane as eluent to yield a white crystalline solid (1.94 g, 65%).
3-Methyl-2-(methylthio)-6-nitrobenzo[d]thiazol-3-ium hexafluorophosphate (21d). Compound 20 (4.66 g, 20.77 mmol) was treated with Me2SO4 (13.04 g, 103.5 mmol). The mixture was heated at 180 °C for 90 min and then cooled to room temperature. Water (20 mL) and KPF6 (19.04 g, 103.5 mmol) were added, leading to the precipitation of a brown solid, which was separated by filtration, washed successively three times with water (10 mL) and three times with EtOAc (5 mL), and then dried to obtain a cream coloured solid (6.76 g, 85%); 1H NMR (400 MHz, MeOD) δ 3.22 (s, 3H, –SCH3), 4.24 (s, 3H, –NCH3), 8.29 (d, J = 9.2 Hz, 1H, ArH), 8.70 (d, J = 11.52 Hz, 1H, ArH), 9.21 (d, J = 2.2 Hz, 1H, ArH); 13C NMR (100 MHz, MeOD) δ 17.42, 36.05, 116.04, 119.73, 124.42, 129.3, 146.13; MALDI m/z calculated for C9H9N2O2S2 241.010, found 241.094 [M]+.
2-Hydrazono-3-methyl-2,3-dihydrobenzo[d]thiazole hydrochloride (22a). Hydrazone 21a (1.96 g, 9.11 mmol) was dissolved in methanol and conc. HCl (2 mL) was added to the reaction mixture. The reaction mixture was heated at 50 °C for 1 h. The cooling of the reaction mixture led to the formation of yellow precipitate. The precipitate was filtered to obtain a yellow solid 22a (2.11 g, 90%); 1H NMR (400 MHz, MeOD) δ 3.53 (s, 3H, –NCH3), 3.85 (brs, 2H, –NH2), 7.20–7.24 (m, 1H, ArH), 7.43–7.46 (m, 2H, ArH), 7.81 (d, J = 8 Hz, 1H, ArH); 13C NMR (100 MHz, MeOD) δ 31.22, 111.19, 121.32, 122.84, 127.32, 140.16; MALDI m/z calculated for C8H9N3S 179.051, found 179.07 [M]+.
(Z)-2-Hydrazono-5-methoxy-3-methyl-2,3-dihydrobenzo [d]thiazole hydrochloride (22b). Hydrazone 21b (1.94 g, 7.91 mmol) was dissolved in methanol and 2 mL conc. HCl was added to the reaction mixture. The reaction mixture was heated at 50 °C for 1 h. The solvent was removed by rotary evaporation to yield a yellow crystalline solid 22b (2.04 g, 90%). 1H NMR (400 MHz, MeOD) δ 3.55 (s, 3H, –NCH3), 3.83 (s, 3H, –OCH3), 6.83 (d, J = 8 Hz, 1H, ArH), 7.03 (s, 1H, ArH), 7.69 (d, J = 8 Hz, 1H, ArH); 13C NMR (100 MHz, MeOD) δ 39.34, 56.29, 98.08, 123.99, 141.94, 159.97; MALDI m/z calculated for C9H11N3OS 209.062, found 210.098 [M + H]+.
(Z)-2-Hydrazono-3-methyl-6-nitro-2,3-dihydrobenzo[d] thiazole (22d). Salt (21d) (6.76 g, 17.52 mmol) was dissolved in acetonitrile. To the reaction mixture, a solution of triethylamine (3.54 g, 35.05 mmol) in acetonitrile was added followed by a solution of hydrazine hydrate (8.76 g, 175.2 mmol) in acetonitrile. The reaction mixture was stirred for 2 h. The completion of the reaction was monitored by TLC. The solvent was removed by rotary evaporation and the product was diluted with water and extracted with ethyl acetate (3 × 70 mL). The organic layer was filtered through a bed of Na2SO4 and concentrated to afford the crude product as a solid. The crude compound was purified by column chromatography over silica gel using 15% ethyl acetate–hexane as eluent to yield a yellow crystalline solid (1.96 g, 50%).
(Z)-2-Hydrazono-3-methyl-6-nitro-2,3-dihydrobenzo[d] thiazole hydrochloride (23d). Hydrazone (22d) (1.96 g, 8.77 mmol) was dissolved in methanol and 2 mL conc. HCl was added to the mixture. The mixture was heated at 50 °C for 1 h. The solvent was removed from the reaction mixture to yield a yellow crystalline solid (1.97 g, 87%). 1H NMR (400 MHz, MeOD) δ 3.67 (s, 3H, –NCH3), 7.61–7.63 (d, J = 8 Hz, 1H, ArH), 8.42–8.44 (d, J = 8 Hz, 1H, ArH), 8.81 (s, 1H, ArH); 13C NMR (100 MHz, MeOD) δ 32.70, 112.44, 120.48, 124.28, 125.28, 144.60, 146.92; MALDI m/z calculated for C8H8N4O2S 224.036, found 225.440 [M + H]+.
General procedure for the synthesis of compounds (17a–y) by method B. Salt (20a/20b/21d) was treated with hydrazine hydrate in DMF at room temperature for 3 h. This led to the in situ generation of hydrazone (GC-MS). After 3 h, substituted aldehydes and ethanol were added to the reaction mixture and allowed to reflux for 6–12 h to get the desired azines 17a–y. The reaction mixture was allowed to cool and then filtered to get the crude product. The crude compound was purified by column chromatography over silica gel using ethyl acetate–hexane as eluent to yield the desired products in moderate to good yields.
2-(Benzylidenehydrazono)-3-methyl-2,3-dihydrobenzo[d] thiazole (17a). White crystalline solid (208 mg, 78%); 1H NMR (400 MHz, DMSO-d6): δ 3.62 (s, 3H, NCH3), 7.13–7.18 (m, 1H, ArH), 7.34–7.48 (m, 5H, ArH), 7.67 (d, J = 8 Hz, 1H, ArH), 7.76 (d, J = 8 Hz, 2H, ArH), 8.46 (s, 1H, N
CH); 13C NMR (100 MHz, DMSO-d6): δ 31.77, 111.04, 122.70, 122.94, 123.89, 127.17, 127.61, 129.29, 130.48, 135.07, 141.15, 152.68, 167.31; HRMS (+ESI) m/z calculated for C15H13N3S 267.0830, found 268.0904 [M + H]+.
3-Methyl-2-((pyridine-4-ylmethylene)hydrazono)-2,3-dihydrobenzo[d]thiazole (17b). Pale yellow solid (193 mg, 72%); 1H NMR (400 MHz, DMSO-d6): δ 3.68 (s, 3H, NCH3), 7.22 (t, J = 8 Hz, 1H, ArH), 7.42–7.46 (m, 2H, ArH), 7.74 (d, J = 8 Hz, 1H, ArH), 8.14 (d, J = 4 Hz, 2H, ArH), 8.51 (s, 1H, N
CH), 8.81 (d, J = 4 Hz, 2H, ArH); 13C NMR (100 MHz, DMSO-d6): δ 31.92, 111.83, 123.09, 123.22, 123.46, 124.01, 140.95, 143.55, 147.21, 171.59; HRMS (+ESI) m/z calculated for C14H12N4S 268.0783, found 269.0869 [M + H]+.
4-(((3-Methylbenzo[d]thiazol-2(3H)-ylidene)hydrazono)methyl)phenol (17c). Yellow solid (226 mg, 80%); 1H NMR (400 MHz, DMSO-d6): δ 3.51 (s, 3H, NCH3), 6.86 (d, J = 8 Hz, 2H, ArH), 7.13–7.17 (m, 1H, ArH), 7.34–7.38 (m, 2H, ArH), 7.60 (d, J = 8 Hz, 2H, ArH), 7.68 (d, J = 8 Hz, 1H, ArH), 8.38 (s, 1H, N
CH); 13C NMR (100 MHz, DMSO-d6): δ 31.91, 111.10, 122.78, 122.98, 123.87, 125.82, 127.24, 129.51, 141.12, 152.91, 160.07, 166.07; HRMS (+ESI) m/z calculated for C15H13N3OS 283.0779, found 284.0902 [M + H]+.
2-((4-Methoxybenzylidene)hydrazono)-3-methyl-2,3-dihydrobenzo[d]thaizole (17d). Yellow solid (274 mg, 79%); 1H NMR (400 MHz, DMSO-d6): δ 3.60 (s, 3H, NCH3), 3.80 (s, 3H, OCH3), 7.02 (d, J = 8 Hz, 2H, ArH), 7.14 (t, J = 8 Hz, 1H, ArH), 7.33–7.39 (m, 2H, ArH), 7.65–7.71 (m, 3H, ArH), 8.41 (s, 1H, N
CH); 13C NMR (100 MHz, DMSO-d6): δ 31.79, 55.79, 111.00, 114.81, 122.69, 122.94, 123.86, 127.19, 127.52, 129.29, 141.14, 152.51, 161.36, 166.39; HRMS (+ESI) m/z calculated for C15H15N3OS 297.0935, found: 298.1019 [M + H]+.
2-((2,3-Dimethoxybenzylidene)hydrazono)-3-methyl-2,3-dihydrobenzo[d]thiazole (17e). Yellow solid (241 mg, 74%); 1H NMR (400 MHz, DMSO-d6): δ 3.63 (s, 3H, NCH3), 3.79 (s, 3H, OCH3), 3.83 (s, 3H, OCH3), 7.10–7.18 (m, 3H, ArH), 7.36–7.40 (m, 2H, ArH), 7.47 (dd, J = 2 Hz, 9 Hz, 1H, ArH), 7.68 (d, J = 8 Hz, 1H, ArH), 8.63 (s, 1H); 13C NMR (100 MHz, DMSO-d6): δ 31.85, 56.23, 61.69, 111.17, 114.69, 117.68, 122.84, 122.97, 123.89, 124.84, 127.23, 128.26, 141.09, 147.94, 148.35, 153.23, 167.46; HRMS (+ESI) m/z calculated for C17H17N3O2S 327.1041, found 328.1169 [M + H]+.
2-((4-Chlorobenzylidene)hydrazono)-3-methyl-2,3-dihydrobenzo[d]thiazole (17f). Yellow solid (243 mg, 81%); 1H NMR (400 MHz, DMSO-d6): δ 3.60 (s, 3H, NCH3), 7.12–7.17 (m, 1H, ArH), 7.33–7.39 (m, 2H, ArH), 7.52 (d, J = 8 Hz, 2H, ArH), 7.65 (d, J = 8 Hz, 1H), 7.77 (d, J = 8 Hz, 2H, ArH), 8.43 (s, 1H, N
CH); 13C NMR (100 MHz, DMSO-d6): δ 31.65, 111.00, 122.68, 122.91, 123.84, 127.17, 129.13, 129.38, 134.10, 134.78, 141.13, 151.35, 167.65; HRMS (+ESI) m/z calculated for C15H12ClN3S 301.0440, found: 302.0522 [M + H]+.
2-((4-Fluorobenzylidene)hydrazono)-3-methyl-2,3-dihydro benzo[d]thiazole (17g). White solid (196 mg, 69%); 1H NMR (400 MHz, DMSO-d6): δ 3.60 (s, 3H, NCH3), 7.14 (t, J = 8 Hz, 1H, ArH), 7.27–7.38 (m, 4H, ArH), 7.65 (d, J = 8 Hz, 1H, ArH), 7.78–7.82 (m, 2H, ArH), 8.44 (s, 1H, N
CH); 13C NMR (100 MHz, DMSO-d6): δ 31.72, 111.01, 116.25, 116.47, 122.69, 122.91, 123.83, 127.17, 129.66, 131.68, 141.11, 151.49, 162.32, 164.78, 167.30; HRMS (+ESI) m/z calculated for C15H12FN3S 285.0736, found 286.0835 [M + H]+.
3-Methyl-2-((pyridine-2-yl-methylene)hydrazono)-2,3-dihydrobenzo[d]thiazole (17h). Orange coloured solid (187 mg, 70%); 1H NMR (400 MHz, DMSO-d6): δ 3.68 (s, 3H, NCH3), 7.21 (t, J = 8 Hz, 1H, ArH), 7.39–7.47 (m, 2H, ArH), 7.72–7.79 (m, 2H, ArH), 8.23 (d, J = 8 Hz, 1H, ArH), 8.34 (t, J = 8 Hz, 1H, ArH), 8.53 (s, 1H, N
CH), 8.77 (d, J = 8 Hz, 1H, ArH); 13C NMR (100 MHz, DMSO-d6): δ 31.33, 111.71, 122.84, 123.05, 123.29, 123.99, 125.86, 127.35, 140.96, 143.23, 145.56, 170.38; HRMS (+ESI) m/z calculated for C14H12N4S 268.0782, found 269.0867 [M + H]+.
2-(((3-Methylbenzo[d]thiazol-2(3H)ylidene)hydrazono)methyl)quinolin-8-ol (17i). Fine orange solid (263 mg, 79%); 1H NMR (400 MHz, DMSO-d6): δ 3.71 (s, 3H, NCH3), 7.21 (t, J = 8 Hz, 1H, ArH),7.37–7.48 (m, 5H, ArH), 7.55–7.61 (m, 1H, ArH), 7.74 (d, J = 8 Hz, 1H, ArH), 8.27 (d, J = 8 Hz, 1H, ArH), 8.70 (s, 1H, ArH), 8.72 (s, 1H, N
CH); 13C NMR (100 MHz, DMSO-d6): δ 31.95, 111.66, 118.66, 123.05, 123.26, 124.08, 127.34, 129.42, 141.07; HRMS (+ESI) m/z calculated for C18H14N4OS 334.0888, found 335.0982 [M + H]+.
2-(((2-Chloroquinolin-3-yl)methylene)hydrazono)-3-methyl-2,3-dihydrobenzo[d]thiazole (17j). Yellow solid (246 mg, 70%); 1H NMR (400 MHz, DMSO-d6): δ 3.62 (s, 3H, NCH3), 7.14–7.17 (m, 1H, ArH), 7.35–7.40 (m, 2H, ArH), 7.68 (d, J = 8 Hz, 1H, ArH), 7.97–8.01 (m, 1H, ArH), 8.52 (s, 1H, N
CH), 8.69 (d, J = 8 Hz, 1H, ArH), 8.84 (d, J = 8 Hz, 1H, ArH), 9.08 (s, 1H, ArH); 13C NMR (100 MHz, DMSO-d6): δ 31.63, 111.24, 122.85, 122.93, 123.84, 127.15, 127.19, 134.20, 140.32, 141.06, 142.26, 143.1, 147.01, 169.47; HRMS (+ESI) m/z calculated for C18H13ClN4S 352.0549, found 353.0635 [M + H]+.
3-Methyl-2-((pyridine-3-ylmethylene)hydrazono)-2,3-dihydrobenzo[d]thiazole (17k). Yellow solid (174 mg, 65%); 1H NMR (400 MHz, DMSO-d6): δ 3.62 (s, 3H, NCH3), 7.35–7.38 (m, 2H, ArH), 7.66–7.71 (m, 2H, ArH), 7.85 (t, J = 8 Hz, 1H, ArH), 7.97 (d, 1H, ArH), 8.17 (s, 1H, ArH), 8.70 (s, 1H, N
CH), 8.89 (d, J = 8 Hz, 1H, ArH); 13C NMR (100 MHz, DMSO-d6): δ 31.61, 111.05, 122.71, 122.83, 123.99, 127.17, 127.40, 128.18, 128.29, 129.23, 131.95, 135.95, 141.20, 147.33, 147.51, 168.82; HRMS (+ESI) m/z calculated for C14H12N4S 268.0783, found 269.0869 [M + H]+.
2-(((5-Bromo-1H-indol-3-yl)methylene)hydrazono)-3-methyl-2,3-dihydrobenzo[d]thiazole (17l). White solid (311 mg, 81%); 1H NMR (400 MHz, DMSO-d6): δ 3.68 (s, 3H, NCH3), 7.16–7.20 (m, 1H, ArH), 7.36–7.43 (m, 3H, ArH), 7.47 (d, J = 8 Hz, 1H, ArH), 7.79 (d, J = 8 Hz 1H, ArH), 7.94 (d, J = 4 Hz, 1H, ArH), 8.40 (d, J = 4 Hz, 1H, ArH), 8.71 (s, 1H, N
CH); 13C NMR (100 MHz, DMSO-d6): δ 32.15, 111.12, 111.79, 113.87, 114.59, 122.76, 123.17, 123.73, 124.54, 125.73, 126.43, 127.30, 133.16, 136.37, 141.29, 149.49, 164.44; HRMS (+ESI) m/z calculated for C17H13BrN4S 386.0044, found 387.0091 [M + H]+.
3-Methyl-2-((thiophen-2-ylmethylene)hydrazono)-2,3-dihydrobenzo[d]thiazole (17m). Light green solid (218 mg, 80%; 1H NMR (400 MHz, DMSO-d6): δ 3.59 (s, 3H, NCH3), 7.11–7.15 (m, 2H, ArH), 7.32–7.38 (m, 2H, ArH), 7.44 (d, J = 4 Hz, 1H, ArH), 7.63–7.67 (m, 2H, ArH), 8.60 (s, 1H, N
CH); 13C NMR (100 MHz, DMSO-d6): δ 30.98, 110.11, 121.83, 122.27, 123.54, 126.46, 127.75, 128.33, 129.98, 139.75, 140.83, 146.76, 165.80; HRMS (+ESI) m/z calculated for C13H11N3S2 273.0394, found 274.0463 [M + H]+.
3-Methyl-2-(((4-methyl-1H-imidazol-5yl)methylene) hydrazono)-2,3-dihydrobenzo[d] thiazole (17n). Yellow solid (211 mg, 78%); 1H NMR (400 MHz, DMSO-d6): δ 2.50 (s, 3H, CH3), 3.59 (s, 3H, NCH3), 7.13 (t, J = 8 Hz, 1H, ArH), 7.33–7.38 (m, 2H, ArH), 7.66 (d, J = 8 Hz, 1H, ArH), 8.41 (s, 1H, N
CH), 9.07 (s, 1H, ArH); 13C NMR (100 MHz, DMSO-d6): δ 10.40, 31.50, 110.93, 122.55, 123.82, 124.83, 127.10, 129.79, 134.48, 140.58, 141.14, 167.89; HRMS (+ESI) m/z calculated for C13H13N5S 271.0892, found 294.0782 [M + Na]+.
3-Methyl-2-(((4-methylthiazol-2-yl)methylene)hydrazono)-2,3-dihydrobenzo[d]thiazole (17o). Pale yellow solid (237 mg, 75%); 1H NMR (400 MHz, DMSO-d6): δ 2.46 (s, 3H, CH3), 3.79 (s, 3H, NCH3), 7.13–7.21 (m, 1H, ArH), 7.33–7.44 (m, 2H, ArH), 7.53 (s, 1H, ArH), 7.69 (d, J = 8 Hz, 1H, ArH), 8.08 (s, 1H, N
CH); 13C NMR (100 MHz, DMSO-d6): δ 16.31, 31.78, 110.95, 120.00, 122.59, 123.65, 126.73, 140.40, 141.61, 151.70, 155.33; HRMS (+ESI) m/z calculated for C13H12N4S2 288.0503, found 311.0397 [M + Na]+.
2-Hydroxy-5-(((3-methylbenzo[d]thiazol-2(3H)-ylidene)hydrazono)methyl)benzoic acid (17p). Yellow coloured solid (258 mg, 79%); 1H NMR (400 MHz, DMSO-d6): δ 3.61 (s, 3H, NCH3), 7.06 (d, J = 8 Hz, 1H, ArH) 7.12–7.14 (m, 1H, ArH), 7.33–7.38 (m, 2H, ArH), 7.68 (d, J = 8 Hz, 1H, ArH), 7.91 (dd, J = 4 Hz, 10 Hz, 1H, ArH), 7.90 (d, J = 8 Hz, 1H, ArH), 8.17 (s, 1H, OH), 8.44 (s, 1H, N
CH); 13C NMR (100 MHz, DMSO-d6): δ 31.17, 110.98, 113.87, 118.35, 122.98, 123.89, 126.52, 127.13, 129.92, 134.25, 141.17, 151.64, 162.86, 166.76, 171.97; HRMS (+ESI) m/z calculated for C16H13N3O3S 327.0677, found 328.0765 [M + H]+.
2-((4-Chlorobenzylidene)hydrazono)-5-methoxy-3-methyl-2,3-dihydrobenzo[d]thiazole (17q). Yellow coloured solid (235 mg 71%); 1H NMR (400 MHz, DMSO-d6): δ 3.60 (s, 3H, NCH3), 3.82 (s, 3H, OCH3), 6.73–6.75 (dd, J = 2.4 Hz, 8 Hz, 1H, ArH), 6.96–6.97 (d, J = 2.4 Hz, 1H, ArH), 7.50–7.53 (m, 3H, ArH), 7.75–7.77 (d, J = 8 Hz, 2H, ArH), 8.43 (s, 1H, N
CH); 13C NMR (100 MHz, DMSO-d6): δ 31.81, 56.14, 97.71, 109.03, 114.88, 123.40, 129.10, 129.36, 134.75, 142.32, 151.24, 159.56, 168.63; HRMS (+ESI) calculated for C16H14ClN3OS 331.0546, found 332.0633 [M + H]+.
5-Methoxy-3-methyl-2-((3,4,5-trimethoxybenzylidene)-hydrazono)-2,3-dihydrobenzo[d]thiazole (17r). Light yellow solid (301 mg, 85%); 1H NMR (400 MHz, DMSO-d6): δ 3.61 (s, 3H, NCH3), 3.71 (s, 3H, OCH3), 3.83 (s, 6H, OCH3), 6.72–6.75 (dd, J = 4 Hz, 8 Hz, 1H, ArH), 6.96–6.97 (d, J = 2 Hz, 1H, ArH), 7.08 (s, 2H, ArH), 7.53–7.55 (d, J = 8 Hz, 1H, ArH), 8.37 (s, 1H, N
CH); 13C NMR (100 MHz, DMSO-d6): δ 31.85, 56.14, 60.60, 97.65, 104.79, 108.99, 114.93, 123.42, 130.64, 139.64, 142.38, 152.41, 153.59, 159.56, 167.92; HRMS (+ESI) m/z calculated for C19H21N3O4S 387.1252, found 388.1337 [M + H]+.
2-(((2-Chloroquinolin-3-yl)methylene)hydrazono)-5-methoxy-3-methyl-2,3-dihydrobenzo[d]thiazole (17s). Yellow solid (298 mg, 78%); 1H NMR (400 MHz, DMSO-d6): δ 3.65 (s, 3H, NCH3), 3.84 (s, 3H, OCH3), 6.78–6.81 (dd, J = 4 Hz, 12 Hz, 1H, ArH), 7.04–7.05 (d, J = 4 Hz, 1H, ArH), 7.57–7.68 (m, 2H, ArH), 8.14–8.20 (m, 2H, ArH), 8.41 (s, 1H, N
CH), 8.72–8.73 (d, J = 4, 1H, ArH); 13C NMR (100 MHz, DMSO-d6) 31.22, 55.76, 97.43, 108.75, 122.79, 126.89, 127.66, 127.75, 128.66, 131.39, 135.42, 146.76, 147.01, 148.42, 159.25, 169.34; HRMS (+ESI) (m/z) calculated for C19H15ClN4OS 382.0655, found 382.0618 [M]+.
(E)-5-Methoxy-3-methyl-2-((E)-(pyridine-3-yl-methylene) hydrazono)-2,3-dihydrobenzo[d]thiazole (17t). Pale yellow solid (190 mg, 64%); 1H NMR (400 MHz, DMSO-d6): δ 3.61 (s, 3H, NCH3), 3.83 (s, 3H, OCH3), 6.74–6.77 (dd, J = 4 Hz, 8 Hz, 1H, ArH), 6.99 (d, J = 2.4 Hz, 1H, ArH), 7.53–7.55 (d, J = 8 Hz, 1H, ArH), 7.84–7.86 (m, 1H, ArH), 8.49–8.54 (m, 2H, ArH), 8.76–8.77 (d, J = 4 Hz, 1H, ArH), 9.03 (s, 1H, N
CH); 13C NMR (100 MHz, DMSO-d6): δ 31.13, 55.78, 97.42, 108.66, 122.80, 125.41, 137.13, 141.90, 144.59, 146.11, 147.76, 159.24; HRMS (+ESI) (m/z) calculated for C15H14N4OS: 298.0888, found 299.0971 [M + H]+.
5-Methoxy-3-methyl-2-((thiophene-3-ylmethylene) hydrazono)-2,3-dihydrobenzo[d]thiazole (17u). Light green solid (239 mg, 79%); 1H NMR (400 MHz, DMSO-d6): δ 3.58 (s, 3H, NCH3), 3.82 (s, 3H, OCH3), 6.72–6.74 (dd, J = 2.4 Hz, 8 Hz, 1H, ArH), 6.95–6.96 (d, J = 4 Hz, 1H, ArH), 7.13–7.15 (m, 1H, ArH), 7.43–7.44 (d, J = 4 Hz, 1H, ArH), 7.52–7.54 (d, J = 8 Hz, 1H, ArH), 7.62–7.63 (d, J = 4 Hz, 1H, ArH), 8.59 (s, 1H, N
CH); 13C NMR (100 MHz, DMSO-d6): δ 31.77, 56.14, 97.61, 108.90, 114.90, 123.44, 128.41, 129.12, 131.04, 139.99, 142.36, 147.16, 159.54, 167.48; HRMS (+ESI) (m/z) calculated for C14H13N3OS2 303.0500, found 304.0596 [M + H]+.
(E)-5-Methoxy-3-methyl-2-((E)-(pyridine-4-yl-methylene) hydrazono)-2,3-dihydrobenzo[d]thiazole (17v). Brown solid (202 mg, 68%; 1H NMR (400 MHz, DMSO-d6): δ 3.67 (s, 3H, NCH3), 3.85 (s, 3H, OCH3), 6.81–6.83 (d, J = 8 Hz, 1H, ArH), 7.07 (s, 1H, ArH), 7.59–7.61 (d, J = 8 Hz, 1H, ArH), 8.05–8.03 (d, J = 8 Hz, 2H, ArH), 8.48 (s, 1H,
CH), 8.79–8.80 (d, J = 4 Hz, 1H, ArH); 13C NMR (100 MHz, DMSO-d6): δ 31.13, 55.78, 97.42, 108.66, 122.80, 125.41, 137.13, 141.97, 144.59, 146.13, 147.76, 159.24; HRMS (+ESI) (m/z) calculated for C15H14N4OS 298.0888, found 299.0954 [M + H]+.
(E)-3-Methyl-5-nitro-2-((E)-(3,4,5-trimethoxybenzylidine) hydrazono)-2,3-dihydrobenzo[d]thiazole (17w). Orange solid (289 mg, 72%); 1H NMR (400 MHz, DMSO-d6): δ 3.63 (s, 3H, NCH3), 3.72 (s, 3H, OCH3), 3.85 (s, 6H, OCH3), 7.12 (s, 2H, ArH), 7.43–7.44 (d, J = 8.8 Hz, 1H, ArH), 8.20–8.23 (dd, J = 2 Hz, 9.2 Hz, 1H, ArH), 8.38 (s, 1H, N
CH), 8.61 (d, J = 1.6 Hz, 1H, ArH); 13C NMR (100 MHz, DMSO-d6): δ 31.43, 56.18, 60.18, 105.41, 109.54, 118.09, 123.06, 125.11, 129.93, 140.22, 141.81, 146.30, 153.25, 154.48, 165.77; HRMS (+ESI) calculated for C18H18N4O5S 402.0997, found 425.0893 [M + Na]+.
(E)-3-Methyl-6-nitro-2-((E)-(pyridin-4-ylmethylene)hydrazono)-2,3-dihydrobenzo[d]thiazole (17x). Pale yellow solid (192 mg, 61%); 1H NMR (400 MHz, DMSO-d6): δ 3.66 (s, 3H, NCH3), 7.59–7.61 (d, J = 12 Hz, 1H, ArH), 8.13–8.14 (d, J = 4 Hz, 2H, ArH), 8.27–8.30 (dd, J = 2 Hz, 12 Hz, 1H, ArH), 8.60 (s, 1H, ArH), 8.72 (d, J = 2.4 Hz, 1H, ArH), 8.86–8.87 (d, J = 4 Hz, 1H, ArH); 13C NMR (100 MHz, DMSO-d6): δ 37.15, 115.98, 116.31, 118.85, 121.72, 123.83, 124.57, 128.79, 130.20, 147.30, 150.86, 154.08, 155.53. HRMS (+ESI) calculated for C14H11N5O2S 313.0633, found 314.0724 [M + H]+.
4-((E)-((E)-(3-Methyl-6-nitrobenzo[d]thiazol-2(3H)-ylidene)hydrazono)methyl)-2-nitrophenol (17y). Brown solid (268 mg, 72%); 1H NMR (400 MHz, DMSO-d6): δ 3.56 (s, 3H, NCH3), 7.22–7.24 (d, J = 8 Hz, 1H, ArH), 7.44–7.46 (d, J = 8 Hz, 1H, ArH), 7.95–7.97 (d, J = 8 Hz, 1H, ArH), 818–8.27 (m, 2H, ArH), 8.45 (s, 1H, ArH), 8.61 (s, 1H, ArH); HRMS (+ESI) (m/z) calculated for C14H11N5O2S 373.0480, found 373.0478 [M]+.
Biology
Assessment of QSI activity using Chromobacterium violaceium-based bioassay. All the synthesized unsymmetrical azines (17a–y) were preliminarily tested using a Chromobacterium violaceium CV12472-based bioassay for their inhibition of violacein production, with compound 4-NPO (4-nitropyridine-N-oxide) at final concentration of 50 μM being included in this study as a positive control. We have performed the liquid bioassay to quantify the violacein contents in the presence of the synthesized azines (17a–y), which is also indicative of the percentage inhibition being calculated by comparing the inhibition scores of the synthesized compounds with the equivalent DMSO (control) condition for violacein inhibition. The percentage inhibition mentioned in Table 1 has been calculated based on the absorbance values taken at 568 nm as compared to the negative control DMSO condition, which does not result in any significant inhibition of violacein production. Both the QS inhibition assay and quantification of the violacein contents were performed as described by Singh et al., with few modifications.50 The sixteen-hour-grown culture was 1
:
100 diluted with sterile PBS and 20 μL of this diluted culture was added to 1450 μL of LB broth in test tubes, with 30 μL stock solutions (10 mM) of each of the test compounds (17a–y), resulting in a final concentration of 200 μM, and incubated for 36 h at 30 °C. Subsequently, the violacein was extracted by adding 1000 μL of a solution of butanol and acetone (3
:
1) to the test tubes, kept for 10 min in lukewarm water and afterwards vortexed for 5 min. 1 mL of this solution was centrifuged at 14
000 rpm for 5 min and then 200 μL of the supernatant was added to the 96-well plate. The absorbance of the extracted violacein was measured at 568 nm using a BioTek Power Wave XS2 plate reader using Gen 5 1.10 software. All the experiments were done in triplicate, and data was recorded for two independent sets of experiments.
Assessment of QSI activity using pLasB-gfp(ASV). The leads found active in the preliminary assay using Chromobacterium violaceum were further checked for their QS inhibitory potential using pLasB-gfp(ASV) as described by the group of Michael Givskov with very few modifications.26,51 In a test tube, 10 mL of Luria Bertani broth inoculated with the pLasB-gfp(ASV) strain and incubated overnight at 37 °C. The overnight-grown culture was diluted 1
:
40 (about 125 μL of O/N culture added to 4.875 mL of LB broth). In each row, 170 μL of the LB broth was added to the 1st well and 100 μL to the rest of the wells in a 96 well micro-titre plate. 30 μL from 1 mM stock of compounds 17j and 17n was added to the first well in each row and 100 μL serially transferred to the next wells, up to the fifth well. To the last well in each row was added DMSO as a negative control. Finally, 100 μL of 1
:
40 diluted O/N culture was added to all the wells resulting in 1
:
80 dilution of the culture and a concentration dose (75, 37.5, 18.75, 9.375 and 4.6875) of 17j and 17n. The effect of compounds 17j and 17n on the growth and gfp expression was measured by taking the OD at 450 nm and fluorescence with excitation at 485 nm and emission at 535 nm.
Effect of compounds on pLasB-gfp(ASV) biofilms. The biofilms of plasB-gfp(ASV) were established on microscope coverslips. 10 μL of an overnight-grown culture was added to chambers having 200 μL of LB broth and this assembly was incubated at 37 °C. After every six hours the medium was replaced with fresh LB broth. After about 24 h of incubation 10 μL of 1 mM solutions of each compound was added to the chambers and then again incubated for another 24 h. The three-day-old biofilms were stained with propidium iodide for at least 12 h prior to visualization and washed twice with PBS to remove the unattached cells, then fixed using fixative. The biofilms on coverslips were visualized on a Nikon Confocal microscope using NIS-Elements software.
Molecular docking
Molecular docking analysis was carried out using the Glide 5.8 module in Maestro 9.3 (ref. 52 and 53) to study the binding potential of the active compounds in the active site of the LasR protein. The docking calculations were carried out using an X-ray crystal structure of the LasR protein (PDB code: 2UV0, resolution: 1.8 Å) bound to its natural ligand OdDHL.54 The natural ligand OdDHL binds in the Ligand Binding Domain (LBD). The docking protocol was standardized by performing the redocking of bound ligand OdDHL; the lactone head group of OdDHL forms an H-bond with the nearby Trp 60 residue, while the amide group shows H-bonding with the Tyr 56 and Asp 73 residues.
Protein and ligand structure preparation. The structure of the LasR protein (PDB code: 2UV0) bound to its natural ligand OdDHL was taken from the protein data bank. The protein structures were prepared using the Protein Preparation Wizard incorporated in the Schrodinger package Maestro version 9.3.5. This tool helps in (i) elimination of water molecules; (ii) assigning the right bond orders to amino acids and (iii) adding hydrogen atoms to the protein. Impref (Impact Refinement module) minimization was done up to a state where the average root mean square deviation (RMSD) of all the atoms came down to 0.3 Å. For the preparation of the ligands, the Lig-prep module of Maestro was utilized, where low energy ionized states of the ligands within a pH range of 7.0 ± 2.0 were generated using the OPLS (Optimized Potentials for Liquid Simulations) 2005 force field.52
Receptor Grid generation. The Receptor Grid generation module of the GLIDE (Grid-based Ligand Docking with Energetics) software was used to generate the grid for molecular docking purposes. The co-crystallized synthetic ligand OdDHL was used as a reference for grid generation. The inner grid box of 10 Å was defined around the centroid of the bound ligand, whereas the outer box was extended up to 20 Å.
Ligand docking. For the validation of the docking protocol, the bound ligand was extracted and then re-docked to generate the same docking pose as found with its co-crystallize form. Finally, the set of optimized ligands were docked using the Ligand Docking module of GLIDE; they were analyzed based on their GLIDE docking score and intermolecular interactions.
Acknowledgements
S. S. C. is thankful to the Department of Science and Technology (DST), New Delhi for financial assistance. S. Singh profoundly acknowledges Thomas Bjarnsholt (University of Copenhagen, Denmark) for providing the Pseudomonas aeruginosa lasB-gfp(ASV) strain used in this study. S. Singh is thankful to Council of Scientific and Industrial Research (CSIR), India for providing a senior research fellowship.
Notes and references
- WHO online, http://www.who.int/leishmaniasis/burden/en/ (accessed March 2015).
- M. Hentzer, L. Eberl, J. Nielsen and M. Givskov, BioDrugs, 2003, 17, 241–250 CrossRef CAS PubMed.
- L. Yang, Y. Liu, H. Wu, Z. Song, N. Høiby, S. Molin and M. Givskov, FEMS Immunol. Med. Microbiol., 2012, 65, 146–157 CrossRef CAS PubMed.
- S. Swift, J. A. Downie, N. A. Whitehead, A. M. L. Barnard, G. P. C. Salmond and P. Williams, Adv. Microb. Physiol., 2001, 45, 199–270 CrossRef CAS PubMed.
- J. S. Dickschat, Nat. Prod. Rep., 2010, 27, 343–369 RSC.
- C. Fuqua and E. P. Greenberg, Nat. Rev. Mol. Cell Biol., 2002, 3, 685–695 CrossRef CAS PubMed.
- C. Fuqua, M. R. Parsek and E. P. Greenberg, Annu. Rev. Genet., 2001, 35, 439–468 CrossRef CAS PubMed.
- P. Williams, M. Camara, A. Hardman, S. Swift, D. Milton, V. J. Hope, K. Winzer, B. Middleton, D. I. Pritchard and B. W. Bycroft, Philos. Trans. R. Soc. London, Ser. B, 2000, 355, 667–680 CrossRef CAS PubMed.
- T. R. de Kievit and B. H. Iglewski, Infect. Immun., 2000, 68, 4839–4849 CrossRef CAS PubMed.
- D. S. Blanc, C. Petignat, B. Janin, J. Bille and P. Francioli, Clin. Microbiol. Infect., 1998, 4, 242–247 CrossRef PubMed.
- C. Koch and N. Hoiby, Lancet, 1993, 341, 1065–1069 CrossRef CAS.
- D. H. Shepp, I. T. Tang, M. B. Ramundo and M. K. Kaplan, J. Acquired Immune Defic. Syndr., 1994, 7, 823–831 CAS.
- V. L. Sutter and V. Hurst, Ann. Surg., 1966, 3, 597–602 CrossRef.
- G. Todeschini, M. Franchini, C. Tecchio, V. Meneghini, G. Pizzolo, D. Veneri, C. Murari, M. M. Ricetti and G. Perona, Int. J. Infect. Dis., 1998, 3, 99–104 CrossRef PubMed.
- M. J. Gambello and B. H. Iglewski, J. Bacteriol., 1991, 173, 3000–3009 CrossRef CAS PubMed.
- L. Passador, J. M. Cook, M. J. Gambello, L. Rust and B. H. Iglewski, Science, 1993, 260, 1127–1130 CrossRef CAS PubMed.
- U. A. Ochsner, A. K. Koch, A. Fiechter and J. Reiser, J. Bacteriol., 1994, 176, 2044–2054 CrossRef CAS PubMed.
- U. A. Ochsner and J. Reiser, Proc. Natl. Acad. Sci. U. S. A., 1995, 92, 6424–6428 CrossRef CAS.
- E. C. Pesci, J. B. Milbank, J. P. Pearson, S. McKnight, A. S. Kende, E. P. Greenberg and B. H. Iglewski, Proc. Natl. Acad. Sci. U. S. A., 1999, 96, 11229–11234 CrossRef CAS.
- C. Lu, B. Kirsch, C. K. Maurer, J. C. de Jong, A. Braunshausen, A. Steinbach and R. W. Hartmann, Eur. J. Med. Chem., 2014, 79, 173–183 CrossRef CAS PubMed.
- M. P. Storz, G. Allegretta, B. Kirsch, M. Empting and R. W. Hartmann, Org. Biomol. Chem., 2014, 12, 6094–6104 Search PubMed.
- T. Klein, C. Henn, J. C. de Jong, C. Zimmer, B. Kirsch, C. K. Maurer, D. Pistorius, R. Mu, A. Steinbach and R. W. Hartmann, ACS Chem. Biol., 2012, 7, 1496–1501 CrossRef CAS PubMed.
- G. Chen, L. R. Swem, D. L. Swem, D. L. Stauff, C. T. O’Loughlin, P. D. Jeffrey, B. L. Bassler and F. M. Hughson, Mol. Cell, 2011, 42, 199–209 CrossRef CAS PubMed.
- W. R. J. D. Galloway, J. T. Hodgkinson, S. D. Bowden, M. Welch and D. R. Spring, Chem. Rev., 2011, 111, 28–67 CrossRef CAS PubMed.
- C. E. McInnis and H. E. Blackwell, Bioorg. Med. Chem., 2012, 19, 4820–4828 CrossRef PubMed.
- G. S. Shetye, N. Singh, X. Gao, D. Bandyopadhyay, A. Yan and Y.-Y. Luk, MedChemComm, 2013, 4, 1079–1084 RSC.
- E. A. Ozer, A. Pezzulo, D. M. Shih, C. Chun, C. Furlong, A. J. Lusis, E. P. Greenberg and J. Zabner, FEMS Microbiol. Lett., 2005, 253, 29–37 CrossRef CAS PubMed.
- F. G. Glansdorp, G. L. Thomas, J. K. Lee, J. M. Dutton, G. P. C. Salmond, M. Welch and D. R. Spring, Org. Biomol. Chem., 2004, 2, 3329–3336 Search PubMed.
- N. Amara, R. Mashiach, D. Amar, P. Krief, A. H. Spieser, M. J. Bottomley, A. Aharoni and M. M. Meijler, J. Am. Chem. Soc., 2009, 131, 10610–10619 CrossRef CAS PubMed.
- S. Flagan, W.-K. Ching and J. R. Leadbetter, Appl. Environ. Microbiol., 2003, 69, 909–916 CrossRef CAS PubMed.
- L. Yang, M. T. Rybtke, T. H. Jakobsen, M. Hentzer, T. Bjarnsholt, M. Givskov and T. Tolker-Nielsen, Antimicrob. Agents Chemother., 2009, 53, 2432–2443 CrossRef CAS PubMed.
- C. Lu, B. Kirsch, C. Zimmer, J. C. de Jong, C. Henn, C. K. Maurer, M. Müsken, S. Häussler, A. Steinbach and R. W. Hartmann, Chem. Biol., 2012, 19, 381–390 CrossRef CAS PubMed.
- K. Veena, M. Ramaiah, K. Shashikaladevi, T. S. Avinash and V. P. Vaidya, J. Chem. Pharm. Res., 2011, 3, 130–135 CAS.
- J. Jayabharathi, V. Thanikachalam, A. Thangamani and M. Padmavathy, Med. Chem. Res., 2007, 16, 266–279 CrossRef CAS.
- M. Chandra, A. N. Sahay, D. S. Pandey, R. P. Tripathi, J. K. Saxena, V. J. M. Reddy, M. C. Puerta and P. Valerga, J. Organomet. Chem., 2004, 689, 2256–2267 CrossRef CAS.
- C. Liang, J. Xia, D. Lei, X. Li, Q. Yao and J. Gao, Eur. J. Med. Chem., 2013, 74, 742–750 CrossRef PubMed.
- E. F. Hahn, M. Carroll-Buatti and G. W. Pasternak, J. Neurosci., 1982, 2, 572–576 CAS.
- N. Latif and I. Fathy, J. Org. Chem., 1960, 25, 1614–1617 CrossRef CAS.
- S. Siroisa, G. Hatzakis, D. Wei, Q. Du and K. C. Chou, Comput. Biol. Chem., 2005, 29, 55–67 CrossRef PubMed.
- A. Ramakrishnan, S. S. Chourasiya and P. V. Bharatam, RSC Adv., 2015, 5, 55938–55947 RSC.
- S. Skovstrup, S. T. Le Quement, T. Hansen, T. H. Jakobsen, M. Harmsen, T. Tolker-Nielsen, T. E. Nielsen, M. Givskov and O. Taboureau, ChemMedChem, 2013, 8, 157–163 CrossRef CAS PubMed.
- A.-M. M. E. Omar, N. S. Habib and O. M. Aboulwafa, J. Pharm. Sci., 1982, 71, 991–993 CrossRef CAS PubMed.
- D. J. Brondani, D. R. de M. Moreira, M. P. A. de Farias, F. R. D. S. Souza, F. F. Barbosa and A. C. L. Leite, Tetrahedron Lett., 2007, 48, 3919–3923 CrossRef CAS.
- R. N. Salvatore, A. S. Nagle, S. E. Schmidt and K. W. Jung, Org. Lett., 1999, 1, 1893–1896 CrossRef CAS.
- Z. Casar, D. Guérin, R. T. Časar and D. Lorcy, Acta Chim. Slov., 2010, 57, 77–89 CAS.
- M. M. Sprung, Chem. Rev., 1940, 26, 297–338 CrossRef CAS.
- D. T. Hurst, Adv. Heterocycl. Chem., 1993, 58, 216–260 CrossRef.
- J. Teppema and L. B. Sebrell, J. Am. Chem. Soc., 1927, 40, 1779–1785 CrossRef.
- S. Gatard, S. Blanchard, B. Schollhorn, P. Gouzerh, A. Proust and K. Boubekeur, Chem.–Eur. J., 2010, 16, 8390–8399 CrossRef CAS PubMed.
- T. Morohoshi, M. Kato, K. Fukamachi, N. Kato and T. Ikeda, FEMS Microbiol. Lett., 2008, 279, 124–130 CrossRef CAS PubMed.
- S. Singh, P. J. Wanjari, S. Bhatia, V. C. Sonawane, A. K. Chakraborti and P. V. Bharatam, Med. Chem. Res., 2015, 24, 1975–1987 Search PubMed.
- R. A. Friesner, R. B. Murphy, M. P. Repasky, L. L. Frye, J. R. Greenwood, T. A. Halgren, P. C. Sanschagrin and D. T. Mainz, J. Med. Chem., 2006, 49, 6177–6196 CrossRef CAS PubMed.
- R. A. Friesner, J. L. Banks, R. B. Murphy, T. A. Halgren, J. J. Klicic, D. T. Mainz, M. P. Repasky, E. H. Knoll, M. Shelley, J. K. Perry, D. E. Shaw, P. Francis and P. S. Shenkin, J. Med. Chem., 2004, 47, 1739–1749 CrossRef CAS PubMed.
- M. J. Bottomley, E. Muraglia, R. Bazzo and A. Carfì, J. Biol. Chem., 2007, 282, 13592–13600 CrossRef CAS PubMed.
Footnote |
† Electronic supplementary information (ESI) available: 1H NMR, 13C NMR and HRMS (+ESI) spectra (S1–S50). See DOI: 10.1039/c5ra12925g |
|
This journal is © The Royal Society of Chemistry 2015 |