DOI:
10.1039/C5RA12234A
(Communication)
RSC Adv., 2015,
5, 70972-70976
Superacid mediated intramolecular condensation: facile synthesis of indenones and indanones†
Received
24th June 2015
, Accepted 10th August 2015
First published on 11th August 2015
Abstract
Superacid promoted intramolecular acylation is described for the synthesis of indenones. Interestingly, in all resulting indenones, the olefin bond is rearranged to the exo position of the five membered rings. Significantly, the method is further applied to the synthesis of indanones via the formation of two C–C bonds by in situ treatment of indenones with an external arene, in one-pot. Most importantly, the present sequential method for the synthesis of indanones is advantageous, as it limits even more electron rich external arenes only to the Friedel–Crafts alkylation. This is not possible in previous reports wherein both cinnamic acid ester and the external arenes are treated together in the presence of an acid, wherein the relatively more reactive arene is preferred to facilitate the acylation step.
Among the classical reactions for the creation of C–C bonds, the Friedel–Crafts reaction turned out to be one of the most powerful techniques towards aromatic electrophilic substitutions.1 In particular, for the past few decades there were numerous applications of this reaction under different acidic conditions (Brønsted/Lewis).2–4 Particularly, the intramolecular acylation of this protocol was found to be ideal as it constructs the carbocyclic systems; many natural products and drugs are cyclic compounds. In this regard, indanones and indenones are essential carbocyclic compounds as they constitute natural as well as pharmaceutical products. Some of the biologically important natural and unnatural indanones/indenones are shown in Fig. 1.5 Their interesting biological activities prompted organic chemists to develop new synthetic methods towards their synthesis. In the past decades, many methods have been developed for the synthesis of indanones/indenones. For example, indanones were synthesized using the Friedel–Crafts, Rh-catalysis6 and Nazarov cyclizations.7 Also, indenones were prepared by employing the Friedel–Crafts and metal-catalysed reactions.8 Some notable Pd-catalyzed annulations for the synthesis of indenones have been noted from the research groups of Larock,9 Chiusoli,10 Buchwald11 and Li.12 Nagao13 et al. reported the formation of indenones from allenyl aryl ketones upon treatment with BF3·OEt2. Liang and co-workers disclosed the synthesis of indenones under gold catalyzed tandem rearrangement.14 While, the research group of Ohwada reported triflic acid promoted cyclization for the synthesis of indenes and dihydro naphthalenes.15 Womack disclosed synthesis of indanones and indenols from 2-alkylcinnamaldehydes via intramolecular Friedel–Crafts reaction using FeCl3.16 On the other hand, Klumpp17 and Fillion18 co-workers reported the synthesis of indanones under Friedel–Crafts acylation conditions.
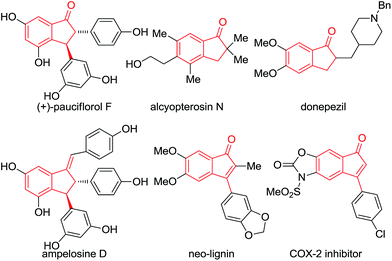 |
| Fig. 1 Naturally occurring and pharmaceutically important indanones/indene/indenones. | |
Normally, the classical intramolecular Friedel–Crafts acylation works between an acyl halide or carboxylic acid or anhydride with an aromatic ring under acid promoter (Lewis or Brønsted). Lewis acid promoted intramolecular Friedel–Crafts acylation of acyl halides are not familiar. Whereas, intramolecular acylation protocols by direct use of carboxylic acids as carbocation equivalents suffer due to the poor leaving nature of the hydroxyl group, hence require forceful conditions. For Meldrum's acids, competition studies for the rate of intramolecular acylation is determined to be tetralone > benzosuberone > indanone. Therefore, synthesis of five-membered ketones (1-indanones) might be feasible under harsh conditions. In addition, direct synthesis of indanones starting from esters using intramolecular Friedel–Crafts acylations are not familiar. However, use of Meldrum's acid for the synthesis of carbocyclic ketones under mild Friedel–Crafts conditions is very good alternative. In 2008, the research group of Baba reported19 acylation of arenes with esters by dimethylchlorosilane and indium tribromide. As part of our ongoing research interests in domino or sequential domino one-pot process, very recently, we have developed a super acid promoted synthesis of indanones starting from simple cinnamic acid esters.20 In this study, the generality and scope of this method has been well studied by synthesizing numerous compounds. We also developed a practical method for the direct synthesis of highly substituted indanones from very simple aryl isopropyl ketones and benzaldehydes promoted by super acid (Scheme 1).21 Though, the Friedel–Crafts cycloacylation was known with acid chlorides and carboxylic acids, the corresponding esters have not been used for this purpose. This may be due to their less reactivity. With this back ground, we envisioned that the direct treatment of simple cinnamic acid esters under strong acidic conditions, would lead to the formation of indenones. Here in, we describe an efficient and practical method for the synthesis of indenones using triflic acid as the promoter under Friedel-Crafts acylation conditions. To the best of our knowledge, there were no reports on the direct intramolecular acylation of cinnamic acid esters. Also, unlike our previous report(s), the present method was applied for the synthesis of indanones in a sequential one-pot manner. In the earlier report for synthesis of indanones, relatively more electron rich aromatic ring was involved in acylation step. Whereas, the present sequential strategy, allows the acylation exclusively by the ring that is derived from cinnamic acid ester, thereby limiting the external arene only to alkylation step irrespective of its reactive nature.
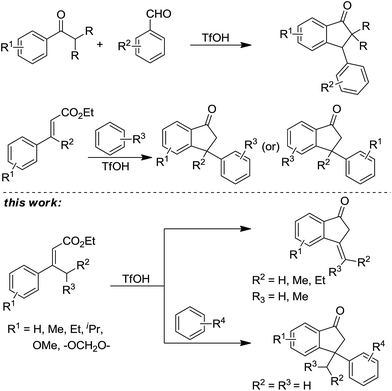 |
| Scheme 1 Comparison of the present vs. previous approaches. | |
To initiate the synthetic study, the required β-alkyl cinnamates 1 were readily synthesized from the corresponding carbonyl compounds (benzaldehydes or corresponding ketones) under standard Wittig–Horner–Wadsworth–Emmons conditions. As a model study, the β-methyl cinnamic acid ester 1a was explored under different set of acidic conditions to sort out optimized conditions and the results are as summarized in Table 1. Therefore, initially our previously established conditions for the synthesis of indanones were applied on β-methyl cinnamic acid ester 1a. However, no progress was seen and led to recovery of the starting material 1a (Table 1, entry 1). Almost same trend has been noticed with CHCl3 and Lewis acids (FeCl3 and BF3·OEt2) as well (Table 1, entries 2–4). Neither the product 2a nor the starting material 1a was isolated when H2SO4 and AlCl3 were used (Table 1, entries 5–6). Interestingly, the expected product 2a was observed but with the double bond isomerization to exo-cyclic position of the five membered ketone by increasing the amount of TfOH (Table 1, entry 7). Interestingly, further increase of TfOH (20 equiv.), gave the indenone 2a in fair yield (Table 1, entry 8). Gratifyingly, the reaction with 24 equiv. of TfOH, furnished the indenone 2a in very good yield (87%, Table 1, entry 9). However, no progress was noticed with more equivalents of mild Lewis acid BF3·OEt2 (Table 1, entry 10). Significantly, the preference of rearranged olefin bond to the exo position to ring reveals its unusual stability over the endo double bond isomer. This present study is further supported by the previous reports for the synthesis of indenones under various conditions, wherein the olefin bond is placed at exo position of the ring as well.10–14
Table 1 Optimizing conditions for the formation of indenone 2a starting from 1a
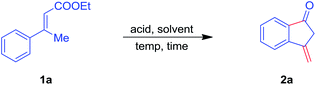
|
Entrya |
Acid (equiv.) |
Solvent (mL) |
Temp (°C) |
Time (h) |
Yieldb (%) |
2a |
All reactions were carried out on 95 mg (0.5 mmol) scale of 1a and solvent DCE (2 mL). Yields of chromatographically pure products. Only starting material 1a was recovered. Neither the product 2a nor starting material 1a was recovered. Starting material 1a was also recovered. |
1 |
TfOH (3) |
DCE (2) |
80 |
12 |
0c |
2 |
TfOH (3) |
CHCl3 (2) |
80 |
12 |
0c |
3 |
FeCl3 (3) |
DCE (2) |
80 |
12 |
0c |
4 |
BF3·OEt2 (3) |
DCE (2) |
80 |
12 |
0c |
5 |
H2SO4 (3) |
DCE (2) |
80 |
12 |
—d |
6 |
AlCl3 (3) |
DCE (2) |
80 |
12 |
—d |
7 |
TfOH (10) |
DCE (2) |
RT |
12 |
50%e |
8 |
TfOH (20) |
DCE (2) |
RT |
12 |
72% |
9 |
TfOH (24) |
DCE (2) |
RT |
8 |
87% |
10 |
BF3·OEt2 (20) |
DCE (2) |
80 |
12 |
0c |
To check the scope and generality of the method, we have applied the above established conditions (Table 1, entry 9) to other β-alkyl cinnamic acid esters 1b–1t and the results are as summarized in Table 2. Delightfully, the protocol was quite successful and amenable for the systems with different substituents and gave the corresponding indenones 2a–2t with di-, tri- and tetra-substituted exo-cyclic olefins, in very good to excellent yields (Table 2). It is worth mentioning that in the case of similar aromatic systems such as phenyl/naphthyl/alkyl substituent on the ring (2a, 2f, 2g, 2o, 2p & 2q), the reaction was quite successful under established conditions [i.e. TfOH (24 equiv.), 8 to 12 h, RT]. On the other hand, usually, the reaction with electron rich aromatic rings (2b–2e, 2h–2n & 2r–2t) completed quickly even with low amount of triflic acid (3 equiv.). Notably, the substrate scope has been well studied with simple to electron rich aromatic rings and with different β-alkyl substituents (methyl, ethyl, n-propyl & iso-propyl) as well. Unless otherwise noted, for each system, the reaction was explored at least two to four times, under varying amount of the acid and time, and the best conditions are presented in Table 2. In addition to the NMR data for the stereochemistry of the exo-cyclic olefin is unambiguously confirmed from the single crystal X-ray diffraction analysis of 2m (see, ESI†).
Table 2 Synthesis of indenones 2 via intramolecular acylation from cinnamic acid esters 1a,b
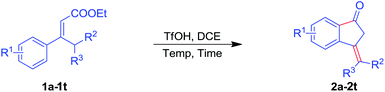
|
All reactions were carried out on 0.5 mmol scale of 1. Yields of chromatographically pure products. |
 |
Furthermore, to extend the method, synthesis of substituted indanones containing quaternary carbon atom was planned, in a sequential one-pot manner. It was anticipated that treatment of in situ generated indenone with an external arene would deliver indanones in a complimentary mode to our previous method for the synthesis of indanones (Scheme 2).20
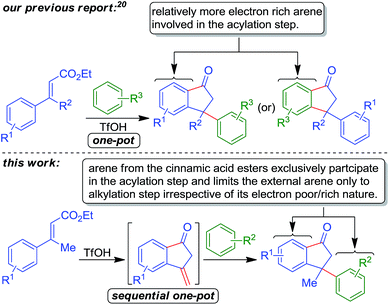 |
| Scheme 2 Comparing the technical differences between the present and previous results. | |
Gratifyingly, as expected, the sequential one-pot protocol was found successful and furnished the corresponding indanones 4a–4j (Table 3). Significantly, unlike our previous method for the synthesis of indanones (in which the relatively more electron rich aromatic ring selectively participated in the Friedel–Crafts acylation step), the present method limits the external arene to only Friedel–Crafts alkylation irrespective of its electron poor/rich nature. However, the reaction was smooth for simple acetophenone derived cinnamic acid esters, but in case of higher β-alkyl cinnamic acid esters, the reaction was not successful. This may be due to more thermodynamic stability of more substituted double bond of the intermediate indenone 2. On the other hand, under forceful conditions led to the decomposition of the reaction mixture.
Table 3 Synthesis of indanones 4 from cinnamic acid esters 1 via intramolecular acylation and intermolecular arylationa,b

|
All reactions were carried out on 0.5 mmol scale of 1. Yields of chromatographically pure products. |
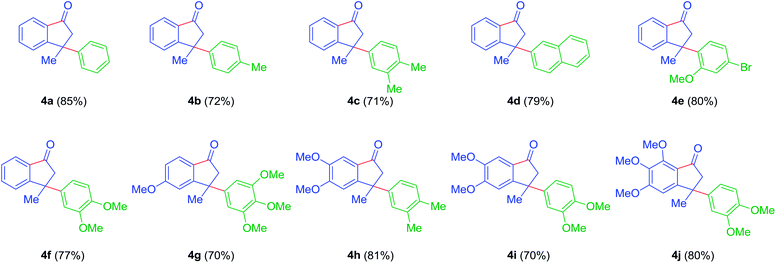 |
A plausible reaction mechanism for the formation of indenones 2 and indanones 4 is shown in Scheme 3. Initially, the protic acid could activate and isomerize the double bond of the ester 1 to yield the equilibrating structures A and B Intramolecular acylation of B and rearomatization leads to the indenone 2. Further, in situ treatment of indenone 2 with an external arene 3 affords indanone 4.
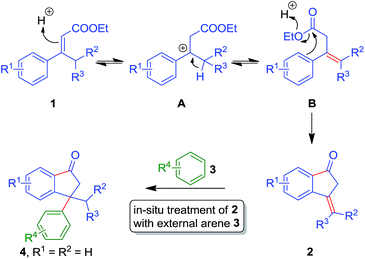 |
| Scheme 3 Plausible reaction mechanism for the formation of indenones 2 and indanones 4. | |
Conclusions
We have disclosed an efficient method for the synthesis of indenones via intramolecular Friedel–Crafts acylation and double bond isomerization of cinnamic acid esters. Further, the protocol was extended to the synthesis of indanones by treatment of in situ generated indenones with external arenes. Notably, the present method limits the external arene only to the Friedel–Crafts alkylation which was not possible in our previous protocol for the synthesis of indanones.
Acknowledgements
We are grateful to the Department of Science and Technology-Science and Engineering Research Board (DST-SERB) [No.: SB/S1/OC-39/2014], New Delhi, for the financial support. B. V. R. thanks CSIR, New Delhi, for the award of research fellowship.
Notes and references
- C. Friedel and J. M. Crafts, C. R. Hebd. Seances Acad. Sci., 1877, 84, 1450 Search PubMed.
- For monographs on the Friedel–Crafts acylation reaction, see;
(a) H. Heaney, in Comprehensive Organic Synthesis, ed. B. M. Trost and I. Fleming, Pergamon Press, Oxford, UK, 1991, vol. 2, p. 733 Search PubMed;
(b) Friedel−Crafts and Related Reactions, ed. G. A. Olah, John Wiley & Sons Inc., London, 1964, vol. 3, Part I, p. 1 Search PubMed;
(c) G. A. Olah, D. A. Klumpp, Superelectrophiles and Their Chemistry, Wiley, New York, 2008 Search PubMed.
- For reviews, see:
(a) H. Heaney in Comprehensive Organic Synthesis, ed. B. M. Trost and I. Fleming, Pergamon Press, Oxford, UK, 1991, vol. 2, p. 753 Search PubMed;
(b) S. Kobayashi, M. Sugiura, H. Kitagawa and W. W.-L. Lam, Chem. Rev., 2002, 102, 2227 CrossRef CAS PubMed;
(c) T. B. Poulsen and K. A. Jorgensen, Chem. Rev., 2008, 108, 2903 CrossRef CAS PubMed;
(d) G. Sartori and R. Maggi, Chem. Rev., 2011, 111, 181 CrossRef PubMed;
(e) M. Rueping and B. J. Nachtsheim, Beilstein J. Org. Chem., 2010, 6(6) DOI:10.3762/bjoc.6.6;
(f) M. Shi, J.-M. Lu, Y. Wei and L.-X. Shao, Acc. Chem. Res., 2012, 45, 641 CrossRef CAS PubMed;
(g) A. Corma and H. Garcia, Chem. Rev., 2003, 103, 4307 CrossRef CAS PubMed;
(h) P. H. Gore, Chem. Rev., 1955, 55, 229 CrossRef CAS;
(i) W. S. Johnson, Org. React., 1944, 2, 114 Search PubMed.
-
(a) K. K. S. Sai, M. J. Tokarz, A. P. Malunchuk, C. Zheng, T. M. Gilbert and D. A. Klumpp, J. Am. Chem. Soc., 2008, 130, 14388 CrossRef CAS PubMed;
(b) Y. Zhang, M. R. Sheets, E. K. Raja, K. N. Boblak and D. A. Klumpp, J. Am. Chem. Soc., 2011, 133, 8467 CrossRef CAS PubMed;
(c) D. A. Evans and K. R. Fandrick, Org. Lett., 2006, 8, 2249 CrossRef CAS PubMed;
(d) M. D. Rose, M. P. Cassidy, P. Rashatasakhon and A. Padwa, J. Org. Chem., 2007, 72, 538 CrossRef CAS PubMed;
(e) Y.-C. Wu, L. Liu, Y.-L. Liu, D. Wang and Y.-J. Chen, J. Org. Chem., 2007, 72, 9383 CrossRef CAS PubMed.
-
(a) T. Ito, T. Tanaka, M. Iinuma, K.-i. Nakaya, Y. Takahashi, R. Sawa, J. Murata and D. Darnaedi, J. Nat. Prod., 2004, 67, 932 CrossRef CAS PubMed;
(b) J. A. Palermo, M. F. R. Brasco, C. Spagnuolo and A. M. Seldes, J. Org. Chem., 2000, 65, 4482 CrossRef CAS;
(c) H. Sugimoto, Y. Iimura, Y. Yamanishi and K. Yamatsu, J. Med. Chem., 1995, 38, 4821 CrossRef CAS;
(d) Y. Oshima and Y. Ueno, Phytochemistry, 1993, 33, 179 CrossRef CAS;
(e) L. M. X. Lopes, M. Yoshida and O. R. Gottlieb, Phytochemistry, 1984, 23, 2021 CrossRef CAS;
(f) D. C. Harrowven, N. A. Newman and C. A. Knight, Tetrahedron Lett., 1998, 39, 6757 CrossRef;
(g) C. H. Park, X. Siomboing, S. Yous, B. Gressier, M. Luyckx and P. Chavatte, Eur. J. Med. Chem., 2002, 37, 461 CrossRef CAS.
-
(a) R. Shintani and T. Hayashi, Org. Lett., 2005, 7, 2071 CrossRef CAS PubMed;
(b) H. Yamabe, A. Mizuno, H. Kusama and N. Iwasawa, J. Am. Chem. Soc., 2005, 127, 3248 CrossRef CAS PubMed;
(c) R. Shintani, K. Okamoto and T. Hayashi, J. Am. Chem. Soc., 2005, 127, 2872 CrossRef CAS PubMed.
-
(a) N. J. Lawrence, E. M. S. Armitage, B. Greedy, D. Cook, S. Ducki and A. T. McGown, Tetrahedron Lett., 2006, 47, 1637 CrossRef CAS PubMed;
(b) W. Yin, Y. Ma, J. Xu and Y. Zhao, J. Org. Chem., 2006, 71, 4312 CrossRef CAS PubMed.
-
(a) T. Fukuyama, N. Chatani, F. Kakiushi and S. Murai, J. Org. Chem., 1997, 62, 5647 CrossRef CAS;
(b) Z. Xi, R. Guo, S. Mito, H. Yan, K.-I. Kanno, K. Nakajima and Y. Takahashi, J. Org. Chem., 2003, 68, 1252 CrossRef CAS PubMed;
(c) D. K. Rayabarapu, C.-H. Yang and C.-H. Cheng, J. Org. Chem., 2003, 68, 6726 CrossRef CAS PubMed;
(d) K.-J. Chang, D. K. Rayabarapu and C.-H. Cheng, J. Org. Chem., 2004, 69, 4781 CrossRef CAS PubMed.
-
(a) R. C. Larock, M. J. Doty and S. Cacchi, J. Org. Chem., 1993, 58, 4579 CrossRef CAS;
(b) V. Gevorgyan, L. G. Quan and Y. Yamamoto, Tetrahedron Lett., 1999, 40, 4089 CrossRef CAS;
(c) L. G. Quan, V. Gevorgyan and Y. Yamamoto, J. Am. Chem. Soc., 1999, 121, 3545 CrossRef CAS;
(d) B. Chen, X. Xie, J. Lu, Q. Wang, J. Zhang, S. Tang, X. She and X. Pan, Synlett, 2006, 259 CAS.
- E. Brocato, C. Castagnoli, M. Catellani and G. P. Chiusoli, Tetrahedron Lett., 1992, 33, 7433 CrossRef CAS.
- A. Minatti, X.-L. Zheng and S. L. Buchwald, J. Org. Chem., 2007, 72, 9253 CrossRef CAS PubMed.
- S.-F. Pi, X.-H. Yang, X.-C. Huang, Y. Liang, G.-N. Yang, X.-H. Zhang and J.-H. Li, J. Org. Chem., 2010, 75, 3484 CrossRef CAS PubMed.
- Y. Nagao, W.-S. Lee and K. Kim, Chem. Lett., 1994, 389 CrossRef CAS.
-
(a) L.-J. Wang, H.-T. Zhu, A.-Q. Wang, Y.-F. Qiu, X.-Y. Liu and Y.-M. Liang, J. Org. Chem., 2014, 79, 204 CrossRef CAS PubMed;
(b) F. Yang, Y.-F. Qiu, K.-G. Ji, Y.-N. Niu, S. Ali and Y.-M. Liang, J. Org. Chem., 2012, 77, 9029 CrossRef CAS PubMed;
(c) Y.-F. Qiu, F. Yang, Z.-H. Qiu, M.-J. Zhong, L.-J. Wang, Y.-Y. Ye, B. Song and Y.-M. Liang, J. Org. Chem., 2013, 78, 12018 CrossRef CAS PubMed.
- H. Kurouchi, H. Sugimoto, Y. Otani and T. Ohwada, J. Am. Chem. Soc., 2010, 132, 807 CrossRef CAS PubMed.
-
(a) G. B. Womack, J. G. Angeles, V. E. Fanelli and C. A. Heyer, J. Org. Chem., 2007, 72, 7046 CrossRef CAS PubMed;
(b) D. H. Dethe and G. M. Murhade, Chem. Commun., 2013, 49, 8051 RSC;
(c) D. H. Dethe and G. Murhade, Org. Lett., 2013, 15, 429 CrossRef CAS PubMed;
(d) D. H. Dethe and G. M. Murhade, Chem. Commun., 2015, 51, 10891 RSC.
-
(a) R. Rendy, Y. Zhang, A. McElrea, A. Gomez and D. A. Klumpp, J. Org. Chem., 2004, 69, 2340 CrossRef CAS PubMed;
(b) K. N. Boblak and D. A. Klumpp, J. Org. Chem., 2014, 79, 5852 CrossRef CAS PubMed;
(c) D. A. Klumpp, D. N. Baek, G. K. S. Prakash and G. A. Olah, J. Org. Chem., 1997, 62, 6666 CrossRef CAS;
(d) E. K. Raja, D. J. DeSchepper, S. O. N. Lill and D. A. Klumpp, J. Org. Chem., 2012, 77, 5788 CrossRef CAS PubMed.
-
(a) E. Fillion, D. Fishlock, A. Wilsily and J. M. Goll, J. Org. Chem., 2005, 70, 1316 CrossRef CAS PubMed;
(b) E. Fillion, A. M. Dumas, B. A. Kuropatwa, N. R. Malhotra and T. C. Sitler, J. Org. Chem., 2006, 71, 409 CrossRef CAS PubMed.
-
(a) Y. Nishimoto, S. A. Babu, M. Yasuda and A. Baba, J. Org. Chem., 2008, 73, 9465 CrossRef CAS PubMed;
(b) S. A. Babu, M. Yasuda and A. Baba, Org. Lett., 2007, 9, 405 CrossRef CAS PubMed.
-
(a) B. V. Ramulu, A. G. K. Reddy and G. Satyanarayana, Synlett, 2013, 24, 863 Search PubMed;
(b) B. V. Ramulu, P. Niharika and G. Satyanarayana, Synthesis, 2015, 47, 1255 CrossRef CAS.
- A. Das, A. G. K. Reddy, J. Krishna and G. Satyanarayana, RSC Adv., 2014, 4, 26662 RSC.
Footnote |
† Electronic supplementary information (ESI) available. CCDC 1408325. For ESI and crystallographic data in CIF or other electronic format see DOI: 10.1039/c5ra12234a |
|
This journal is © The Royal Society of Chemistry 2015 |
Click here to see how this site uses Cookies. View our privacy policy here.