DOI:
10.1039/C5RA11841G
(Paper)
RSC Adv., 2015,
5, 62325-62330
Highly sensitive and selective detection of biothiols by a new low dose colorimetric and fluorescent probe†
Received
19th June 2015
, Accepted 15th July 2015
First published on 15th July 2015
Abstract
In this paper, a new low dose fluorescent probe with excellent sensing properties for detection of biothiols (GSH, Cys and Hcy) was developed. This probe shows rapid response, high selectivity and sensitivity for biothiols with dual colorimetric and fluorescent turn-on signal changes. The detection limit of this probe for biothiols was found to be <10 nM based on the fluorescent detection. More importantly, this probe can be used to image biothiols effectively in living cells even with a low dose of 0.2 μM.
Introduction
Cysteine (Cys), homocysteine (Hcy) and glutathione (GSH) are essential biothiols and they play vital roles in a wide range of physiological and pathological processes for maintaining life in living systems.1 Many studies have shown that abnormal levels of these biothiols (for example, Cys and GSH deficiency) are considered to be signals for many diseases such as osteoporosis, Alzheimer's, liver damage, heart, inflammatory bowel, cardiovascular and neurodegenerative diseases as well as AIDS and cancer.2–6 Therefore, the development of highly effective and convenient detection method for these three biothiols is of considerable current interest.
The optical detection method, with small molecular fluorescent probes in particular, is highly attractive for detection of biologically important compounds due to its high sensitivity, simple manipulation, low cost, and great potential for bioimaging applications. In the past decade, many reaction-based fluorescent probes have been developed for biothiols by using the high nucleophilicity of thiols.7–36 However, many of these developed probes suffer from some drawbacks such as low sensitivity,21–23 a long response time21,24–28 or requirement of a surfactant to speed up the detection process,29,30 a complicated synthetic work27,31,32 or needing short UV light excitation.25,30 In addition, taking into account the potential contamination of organic fluorescent probes and their products after detection to biological samples, it is best to use low dose probes. However, only a few fluorescent probes can be used below a 5 μM level to detect biothiols,24,29,30,34 and the dosage of most reported biothiol probes is at a relatively high level (5–20 μM), which is even approximate to or higher than the normal level of Hcy (5–15 μM) found in living systems.37 In regard to these problems, we recently reported an acrylate functionalized 3-benzothiazolyl-7-hydroxycoumarin (BTHC) as a low dose colorimetric and fluorescent probe for biothiols.38 Although this probe shows high selectivity and sensitivity for biothiols, it needs 50% of DMSO (v/v) as co-solvent for the detection process and its maximum fluorescence enhancement for biothiols is only about 6-fold. In any case, this prompts us to develop better probes.
Compared to the acrylate group, the 2,4-dinitrobenzenesulfonate (DNBS) group is a better fluorescent quencher and is more hydrophilic, moreover, it can be easily cleaved by biothiols under mild conditions.39–44 With this in mind, we synthesised probe 1 (Scheme 1) and envisioned that it will be a better fluorescent probe for biothiols. Herein, we report that probe 1 indeed shows better sensing performance. Besides its high selectivity and sensitivity for biothiols, it only needs 20% of DMSO (v/v) as co-solvent and shows a 35-fold fluorescence enhancement for biothiols. More importantly, this probe can be used to detect biothiols with a very low dose, and even the concentration is as low as 0.2 μM, this probe can still be well used to image biothiols in living cells. Owing to this excellent sensing performance and its easy preparation process, this probe is attractive and promising.
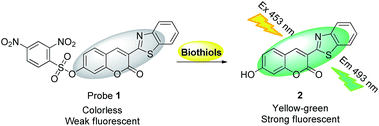 |
| Scheme 1 Probe 1 for detection of biothiols. | |
Results and discussion
1. Probe synthesis
Probe 1 can be easily prepared in a good yield from the reaction of 2 with 2,4-dinitrobenzenesulfonyl chloride at room temperature (Scheme 2). The purification of probe 1 is simple as no column chromatography is needed, which is a big advantage. Compound 2 can also be easily prepared according to a published procedure.45 The structure of probe 1 was confirmed by NMR, MS, HR-MS and elemental analysis. Detailed synthetic procedures and structure characterizations are given in the experimental section and in the ESI.†
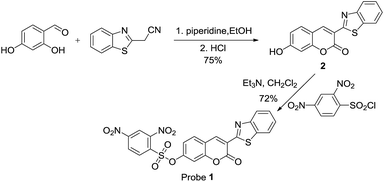 |
| Scheme 2 Synthesis of probe 1. | |
2. The selectivity of probe 1 for biothiols
With probe 1 in hand, we first investigated the sensing property of probe 1 for biothiols in PBS buffer (10 mM, pH = 7.4, 20% DMSO, v/v) by UV-vis and fluorescence spectroscopy. As shown in Fig. 1, upon addition of biothiols, a red-shift of the maximum absorption of the probe 1 solution from 373 nm to 451 nm and a significant fluorescence enhancement around 493 nm were observed. Meanwhile, the probe 1 solution showed a distinct color change and started to emit strong blue-green fluorescence. These optical changes strongly indicate that probe 1 was converted to the fluorescent compound 2 in the presence of biothiols (the optical spectra of 2, see Fig. S1, ESI†). Scanning kinetic experiments further proved this conversion process (Fig. S2, ESI†) and MS spectrum analysis of the fluorescent product from the reaction of probe 1 with Cys confirmed the produce of compound 2 (Fig. S3, ESI†).
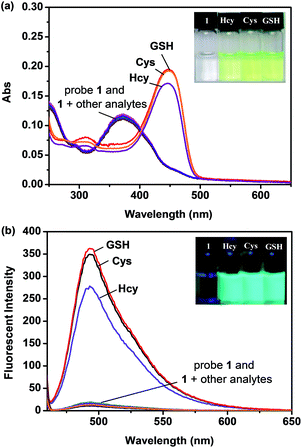 |
| Fig. 1 (a) UV-vis absorption spectra changes of probe 1 (5 μM) and (b) fluorescence spectra changes of probe 1 (1 μM) to various analytes (Ala, Arg, Asn, Asp, Gln, Glu, Gly, His, Ile, Leu, Lys, Met, Phe, Pro, Ser, Trp, Tyr, H2NCH2CH2NH2, HOCH2CH2NH2, C6H5CH2NH2, F−, Cl−, Br−, I−, NO3−, NO2−, AcO−, C2O42−, NO3−, NO2−, PO43−, CO32−, SO42−, SO32−, S2O42−, S2O72−, S2−, HS−, SCN−, IO4−, ClO4−, CN−, Cys, Hcy, and GSH. Cys, Hcy and GSH were used 50 μM, others were used 100 μM). Color changes of probe 1 upon addition of biothiols are inserted. Each spectrum was collected after 15 min of each analyte addition in PBS buffer (10 mM, pH 7.4, 20% DMSO, v/v) at 37 °C. λex = 453 nm, slit: dex 2.5 nm/dem 5 nm. | |
To evaluate the selectivity of probe 1, various other analytes including amino acids Ala, Arg, Asn, Asp, Gln, Glu, Gly, His, Ile, Leu, Lys, Met, Phe, Pro, Ser, Trp, and Tyr, strong nucleophiles such as H2NCH2CH2NH2, HOCH2CH2NH2 and C6H5CH2NH2, potential interfering anion species such as F−, Cl−, Br−, I−, NO3−, NO2−, AcO−, C2O42−, NO3−, NO2−, PO43−, CO32−, SO42−, SO32−, S2O42−, S2O72−, S2−, HS−, SCN−, IO4−, ClO4− and CN− were also added to the probe 1 solution. In contrast, these analytes showed almost no effect to the probe 1 solution (Fig. 1 and S4, ESI†). This result indicates that probe 1 is a highly selective colorimetric and fluorescent probe for biothiols. It should be noted that a dual colorimetric and fluorescent probe is particularly valuable because it offers not only the sensitive detection by fluorescence, but also provides a convenient visual sensing by the “naked eye” without the need for advanced instrumentation. Moreover, detection of GSH as a representative biothiol in the presence of various analytes is also effective, indicating that the presence of these analytes has no interference on the detection of GSH by probe 1 (Fig. S5, ESI†). This further proves the high selectivity of probe 1 for biothiols, and implies that probe 1 has good potential for practical applications.
3. The sensitivity of probe 1 for biothiols
The sensitivity of probe 1 for biothiols was also investigated. Kinetic experiments showed that the reaction of probe 1 (1 μM) with a low concentration (5 μM) of Cys, Hcy or GSH went smoothly in PBS buffer (10 mM, pH 7.4, with 20% DMSO, v/v) at 37 °C and all the reactions completed within 15 min (Fig. S6, ESI†), indicating that probe 1 is highly reactive for biothiols. Based on this, titration experiments were conducted. As shown in Fig. 2a, titration of GSH to a solution of 1 (1 μM) resulted in concentration dependent increases in the emission intensity of the probe solution at 493 nm and a saturation could be observed after the addition of more than 5 equiv. GSH. In addition, we also observed a good linearity between the fluorescent intensity at 493 nm and the concentration of GSH in the range of 0–4.5 μM (Fig. 2b), thus, the detection limit of probe 1 for GSH was determined to be 6 nM. Similar results were obtained for Cys and Hcy, and the detection limit for Cys and Hcy was calculated to be 7 nM and 9 nM, respectively (Fig. S7 and S8, ESI†). These values are lower than that of the most reported biothiol probes including our recently reported acrylate-based biothiol probe,38 indicating that probe 1 is highly sensitive to biothiols. We attribute the high sensitivity of probe 1 to its low background fluorescence, strong fluorescent product 2 and its high reactivity to biothiols (The fluorescence quantum yield (Φ) for 1 and 2 under this experimental condition was determined to be 0.07 and 0.65, respectively, using rhodamine B as standard).
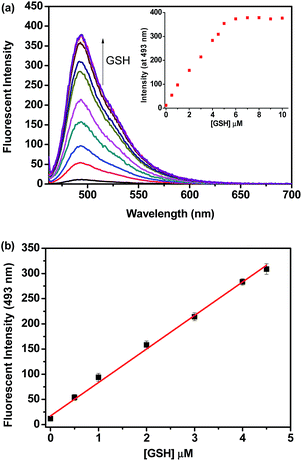 |
| Fig. 2 (a) Fluorescence spectra changes of probe 1 (1 μM) in DMSO–PBS buffer (10 mM, pH 7.4, 20% DMSO) at 37 °C upon addition of different concentrations of GSH (0–10 μM). Insert: fluorescence intensity changes at 493 nm as a function of [GSH]. Each spectrum was obtained 15 min after GSH addition. λex = 453 nm, slit: 2.5 nm/5 nm. (b): Linear relationship of fluorescence intensity at 493 nm as a function of GSH concentration (0–4.5 μM), y = 22.0312 + 64.8383 × [GSH] with R2 = 0.9956. | |
Moreover, we found that detection of biothiols (GSH as the representative) using probe 1 can still be effective even when the dosage of probe 1 was reduced to a submicromolar or even nanomolar level. As shown in Fig. 3, when the concentration of probe 1 is reduced from 0.1 μM to 10 nM, noticeable fluorescence signal changes can still be observed after addition of a low concentration of GSH (even at a nanomolar level). These results clearly indicate that probe 1 can be used in a very low dosage to detect biothiols with high sensitivity. Thus, a low dose, highly selective and sensitive colorimetric and fluorescent probe for biothiols was successfully developed.
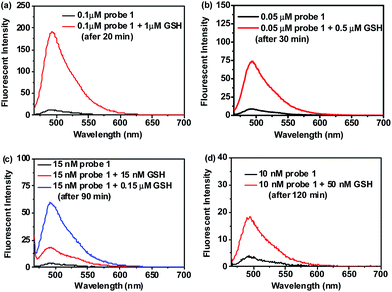 |
| Fig. 3 Detection of GSH using low concentration of probe 1 in PBS buffer (10 mM, pH 7.4, 20% DMSO) at 37 °C. (a) [Probe 1] = 0.1 μM; (b) [probe 1] = 0.05 μM; (c) [probe 1] = 15 nM; (d) [probe 1] = 10 nM. λex = 453 nm, slit width for (a) and (b): dex = 5 nm, dem = 10 nm and for (c) and (d): dex = dem = 10 nm. | |
4. The effect of pH and the amount of DMSO
To evaluate the working pH range for probe 1, fluorescent detection of Cys at different pHs was investigated. Our recently reported acrylate-based low dose biothiol probe is likely to be hydrolysed under basic conditions (pH > 8.5).38 In contrast, the current probe 1 works well even under basic conditions. As shown in Fig. 4, the fluorescence of probe 1 itself showed very small changes over a wide pH range from 2 to 10, however, in the presence of Cys, significant emission enhancement was observed at pH over 5, and the best pH was found over 7. This result indicates that probe 1 is able to work over a relatively wide pH range from 5 to 10, including at physiological pH. The effect of the amount of DMSO was also investigated, and the results showed that reducing the amount of DMSO resulted in a slower reaction, while increasing the amount of DMSO made the reaction faster, but decreased the fluorescence enhancement (Fig. S9, ESI†).
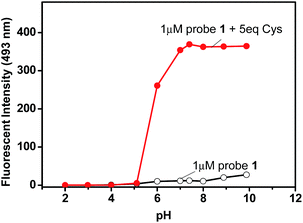 |
| Fig. 4 Fluorescence intensity changes of probe 1 (1 μM) at 493 nm in absence and presence of Cys (5 equiv.) in 10 mM PBS buffer with 20% DMSO at various pH values. Each data was recorded after 15 min at 37 °C. | |
5. Practical applications of probe 1
We first investigated the cytotoxicity of probe 1 by MTT assays, and the results show that probe 1 is of low cytotoxicity to cultured cells (Fig. S10, ESI†). Then, fluorescent imaging of biothiols in living cells by probe 1 was investigated. As shown in Fig. 5, as background, HeLa cells show no fluorescence (A2). However, when HeLa cells were incubated with probe 1 (1 μM) for 30 min, the cells started to show strong green fluorescence (B2). In addition, when a known thiol trapping reagent N-ethylmaleimide (NEM) was added to the cell culture prior to the addition of probe 1, the cells showed no fluorescence (C2). As another control experiment, after HeLa cells were pretreated with NEM, with the addition of Cys as a representative biothiol, and then incubated with probe 1, bright green fluorescence was observed again (D2). These results clearly indicate that probe 1 can be used to image biothiols in living cells. Moreover, the dosage of probe 1 can be further reduced to 0.5 μM (E1 and E2) or even 0.2 μM (F1 and F2). We can see that, obvious fluorescence in the cells can still be observed (F2) even using 0.2 μM of probe 1, which indicates that probe 1 has great advantages for cellular imaging applications.
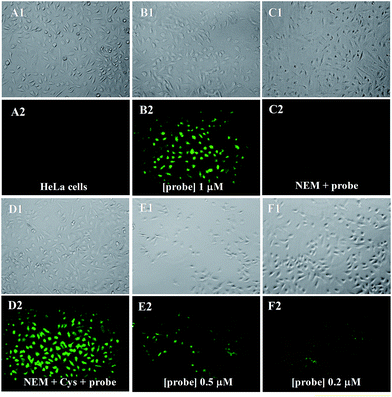 |
| Fig. 5 Imaging of biothiols in living HeLa cells by probe 1. Images A1 and F1 are bright field images. Images A2 and F2 are fluorescent images of A1 and F1, respectively. A1 and A2: HeLa cells as background control. B1 and B2: HeLa cells were incubated with probe 1 (1 μM) for 30 min. C1 and C2: HeLa cells were incubated with NEM (500 μM) prior to incubate with probe 1 (1 μM). D1 and D2: HeLa cells were pre-incubated with NEM (500 μM) for 30 min, then treated with Cys (50 μM) for 30 min, and followed by incubation with probe 1 (1 μM) for 30 min. E1 and E2: HeLa cells were incubated with probe 1 (0.5 μM) for 30 min. F1 and F2: HeLa cells were incubated with probe 1 (0.2 μM) for 30 min. The excitation wavelength was set at 450–490 nm and the emission wavelength was collected at 515–590 nm. | |
Experimentals
1. General
All chemicals were purchased from commercial suppliers and used without further purification. All solvents were purified prior to use. Distilled water was used after passing through a water ultrapurification system. TLC analysis was performed using precoated silica plates. 1H NMR and 13C NMR spectra were recorded on a Varian Mercury 400 spectrometer, and resonances (δ) are given in parts per million relative to tetramethylsilane (TMS). Coupling constants (J) are reported in hertz. IR spectra were recorded on a FT-IR spectrophotometer as KBr pellets and were reported in cm−1. The low-resolution MS spectra were performed on an electron ionization mass spectrometer. HR-MS data were obtained with an LC/Q-TOF MS spectrometer. UV-vis and fluorescence spectra were recorded on a UV-vis spectrophotometer and a fluorescence spectrophotometer with a temperature controller, respectively. Standard quartz cuvettes with a 10 mm light path were used for all optical spectra measurements. Cell imaging was performed in an inverted fluorescence microscopy with a 20× objective lens.
Synthesis of 3-benzothiazolyl-7-hydroxycoumarin (2). Compound 2 was synthesized from 2,4-dihydroxybenzaldehyde and 2-(1,3-benzothiazol-2-yl)acetonitrile according to previously reported methods.34,41 TLC (silica plate): Rf 0.3 (petroleum ether
:
ethyl acetate 2
:
1, v/v); 1H NMR (400 MHz, d6-DMSO, Me4Si): δ (ppm) 9.14 (d, J = 1.7 Hz, 1H), 8.15 (d, J = 7.9 Hz, 1H), 8.04 (d, J = 8.2 Hz, 1H), 7.92–7.89 (m, 1H), 7.56 (t, J = 7.4 Hz, 1H), 7.45 (t, J = 7.1 Hz, 1H), 6.94 (d, J = 8.6 Hz, 1H), 6.88 (s, 1H). 13C NMR (100 MHz, d6-DMSO, Me4Si): δ (ppm) 188.3, 164.2, 160.9, 160.2, 156.2, 152.2, 143.0, 136.0, 132.3, 126.9, 125.4, 122.5, 115.0, 114.7, 111.8, 102.5. IR (KBr) νmax (cm−1): 2965, 2737, 1721 (s), 1707, 1615, 1599, 1585, 1558, 1525, 1452 (s), 1344, 1298, 1257 (s), 858, 755, 508. EI-MS: m/z found 295.14 (M+, 100%), 267.15 (M+ − C2H4, 35%).
Synthesis of probe 1. Compound 2 (148 mg, 0.5 mmol) was dissolved in 40 mL of dry CH2Cl2 and 1 mL Et3N was added. The mixture was stirred at room temperature for 30 min, followed by addition of a solution of 2,4-dinitrobenzenesulfonyl chloride (159 mg, 0.6 mmoL) in 5 mL dry CH2Cl2. The mixture was stirred at room temperature for another 4 h. The resulting yellow residue was collected by filtration and washed with petroleum ether, the organic phase mixture was washed with diluted HCl and brine, dried over MgSO4 and the solvent was removed under reduced pressure. The crude product was purified by recrystallization from ethanol to give probe 1 (183 mg, 72%) as yellow powder. Mp 236–239 °C. 1H NMR (400 MHz, d6-DMSO, Me4Si): δ (ppm) 9.26 (s, 1H), 9.14 (s, 1H), 8.64 (d, J = 8.3 Hz, 1H), 8.36 (d, J = 8.6 Hz, 1H), 8.17 (d, J = 7.8 Hz, 2H), 8.08 (d, J = 8.0 Hz, 1H), 7.63–7.55 (m, 1H), 7.50 (d, J = 10.3 Hz, 2H), 7.31 (d, J = 7.2 Hz, 1H). 13C NMR (100 MHz, d6-DMSO, Me4Si): δ (ppm) 169.34, 157.88, 153.58, 146.59, 136.44, 136.36, 136.18, 130.87, 130.69, 126.27, 125.34, 125.22, 124.75, 124.67, 124.50, 124.28, 123.24, 123.08, 119.87, 119.76, 117.75, 109.93. EI-MS: m/z found 525.20 (M+). HR-MS: calcd for C22H12N3O9S2+ (M + H+), 526.0009; found 526.0009. Elemental analysis calcd (%) for C22H11N3O9S2 (MW 525.2) C 50.29, H 2.11, N 8.00, S 12.20; found C 50.45, H 2.08, N 8.07, S 12.11.
Procedures for optical sensing. Stock solutions of probe 1 (1 mM) were prepared in DMSO (HPLC grade). Stock solutions (1–10 mM) of the analytes were prepared in ultrapure water. For a typical optical measurements, a solution of probe 1 (1 μM) was prepared in DMSO-PBS buffer solution (1
:
4, v/v, 10 mM PBS). Then 3.0 mL of the probe 1 solution was placed in a quartz cuvette until the temperature reached at 37 °C (controlled by a temperature controller). The UV-vis or fluorescent spectra were then recorded upon addition of the analyte of interest.
Cell imaging experiments. HeLa cells were cultured in Dulbecco's Modified Eagle's Medium (DMEM) according to our previous reports.46–51 For a typical living cells imaging experiment of probe 1, cells were incubated with 1 μM of probe 1 (with 0.2% DMSO, v/v) for 30 min at 37 °C and washed three times with prewarmed PBS, and then imaged immediately.
Conclusions
In summary, we developed a new low dose fluorescent probe for biothiols. This probe can be readily prepared and shows high selectivity and sensitivity for biothiols with rapid response and distinct colorimetric and fluorescence turn-on signal changes. Notably, the detection limit of this probe for biothiols is below 10 nM and a very low dosage (0.2 μM) of this probe can be used to detect biothiols effectively in living cells. Taken together, we believe that the excellent sensing properties of this probe will make it a real useful tool for imaging and detection of biothiols.
Acknowledgements
We thank the National Natural Science Foundation of China (Grant nos 21472066 and 21172086) and the Natural Science Foundation of Hubei Province (no. 2014CFA042) for financial support.
Notes and references
- C. E. Paulsen and K. S. Carroll, Chem. Rev., 2013, 113, 4633–4679 CrossRef CAS PubMed.
- B. de Quay, R. Malinverni and B. H. Lauterburg, AIDS, 1992, 6, 815–819 CrossRef CAS.
- L. A. Herzenberg, S. C. de Rosa and J. G. Dubs, Proc. Natl. Acad. Sci. U. S. A., 1997, 94, 1967–1972 CrossRef CAS.
- S. Shahrokhian, Anal. Chem., 2001, 73, 5972–5978 CrossRef CAS.
- R. Franco, O. J. Schoneveld and A. Pappa, Arch. Physiol. Biochem., 2007, 113, 234–258 CrossRef CAS PubMed.
- S. Seshadri, A. Beiser and J. Selhub, N. Engl. J. Med., 2002, 346, 476–483 CrossRef CAS PubMed.
- X. Chen, Y. Zhou, X. Peng and J. Yoon, Chem. Soc. Rev., 2010, 39, 2120–2135 RSC.
- H. S. Jung, X. Chen, J. S. Kim and J. Yoon, Chem. Soc. Rev., 2013, 42, 6019–6031 RSC.
- C. Yin, F. Huo, J. Zhang, R. Martínez-Máñez, Y. Yang, H. Lv and S. Li, Chem. Soc. Rev., 2013, 42, 6032–6059 RSC.
- M. Li, X. Wu, Y. Wang, Y. Li, W. Zhu and T. D. James, Chem. Commun., 2014, 50, 1751–1753 RSC.
- J. Liu, Y.-Q. Sun, H. Zhang, Y. Huo, Y. Shi and W. Guo, Chem. Sci., 2014, 5, 3183–3188 RSC.
- X.-F. Yang, Q. Huang, Y. Zhong, Z. Li, H. Li, M. Lowry, J. O. Escobedo and R. M. Strongin, Chem. Sci., 2014, 5, 2177–2183 RSC.
- S. Bhuniya, S. Maiti, E.-J. Kim, H. Lee, J. L. Sessler, K. S. Hong and J. S. Kim, Angew. Chem., Int. Ed., 2014, 53, 4469–4474 CrossRef CAS PubMed.
- D. Lee, G. Kim, J. Yin and J. Yoon, Chem. Commun., 2015, 51, 6518–6520 RSC.
- Y. Hu, C. H. Heo, G. Kim, E. J. Jun, J. Yin, H. M. Kim and J. Yoon, Anal. Chem., 2015, 87, 3308–3313 CrossRef CAS PubMed.
- Q. Miao, Q. Li, Q. Yuan, L. Li, Z. Hai, S. Liu and G. Liang, Anal. Chem., 2015, 87, 3460–3466 CrossRef CAS PubMed.
- H. Zhang, C. Zhang, R. Liu, L. Yi and H. Sun, Chem. Commun., 2015, 51, 2029–2032 RSC.
- L. A. Montoya and M. D. Pluth, Anal. Chem., 2014, 86, 6032–6039 CrossRef CAS PubMed.
- J. Yin, Y. Kwon, D. Kim, D. Lee, G. Kim, Y. Hu, J.-H. Ryu and J. Yoon, J. Am. Chem. Soc., 2014, 136, 5351–5358 CrossRef CAS PubMed.
- D. Yu, Q. Zhang, S. Ding and G. Feng, RSC Adv., 2014, 4, 46561–46567 RSC.
- P. Wang, J. Liu, X. Lv, Y. Liu, Y. Zhao and W. Guo, Org. Lett., 2012, 14, 520–523 CrossRef CAS PubMed.
- J. H. Lee, C. S. Lim, Y. S. Tian, J. H. Han and B. R. Cho, J. Am. Chem. Soc., 2010, 132, 1216–1217 CrossRef CAS PubMed.
- C. S. Lim, G. Masanta, H. J. Kim, J. H. Han, H. M. Kim and B. R. Cho, J. Am. Chem. Soc., 2011, 133, 11132–11135 CrossRef CAS PubMed.
- F. Kong, R. Liu, R. Chu, X. Wang, K. Xu and B. Tang, Chem. Commun., 2013, 49, 9176–9178 RSC.
- M. Wei, P. Yin, Y. Shen, L. Zhang, J. Deng, S. Xue, H. Li, B. Guo, Y. Zhang and S. Yao, Chem. Commun., 2013, 49, 4640–4642 RSC.
- S.-Y. Lim, K.-H. Hong, D. I. Kim, H. Kwon and H.-J. Kim, J. Am. Chem. Soc., 2014, 136, 7018–7025 CrossRef CAS PubMed.
- M. H. Lee, J. H. Han, P.-S. Kwon, S. Bhuniya, J. Y. Kim, J. L. Sessler, C. Kang and J. S. Kim, J. Am. Chem. Soc., 2012, 134, 1316–1322 CrossRef CAS PubMed.
- D. Kand, A. M. Kalle, S. J. Varma and P. Talukdar, Chem. Commun., 2012, 48, 2722–2724 RSC.
- L. Wang, H. Chen, H. Wang, F. Wang, S. Kambam, Y. Wang, W. Zhao and X. Chen, Sens. Actuators, B, 2014, 192, 708–713 CrossRef CAS PubMed.
- J. Liu, Y.-Q. Sun, Y. Huo, H. Zhang, L. Wang, P. Zhang, D. Song, Y. Shi and W. Guo, J. Am. Chem. Soc., 2014, 136, 574–577 CrossRef CAS PubMed.
- B. K. McMahon and T. Gunnlaugsson, J. Am. Chem. Soc., 2012, 134, 10725–10728 CrossRef CAS PubMed.
- H. Lv, X.-F. Yang, Y. Zhong, Y. Guo, Z. Li and H. Li, Anal. Chem., 2014, 86, 1800–1807 CrossRef CAS PubMed.
- H. Wang, G. Zhou, H. Gai and X. Chen, Chem. Commun., 2012, 48, 8341–8343 RSC.
- H. Wang, G. Zhou, C. Mao and X. Chen, Dyes Pigm., 2013, 96, 232–236 CrossRef CAS PubMed.
- X. Chen, S.-K. Ko, M. J. Kim, I. Shin and J. Yoon, Chem. Commun., 2010, 46, 2751–2753 RSC.
- H. Wang, G. Zhou and X. Chen, Sens. Actuators, B, 2013, 176, 698–703 CrossRef CAS PubMed.
- O. Nygård, S. E. Vollset, H. Refsum, I. Stensvold, A. Tverdal, J. E. Nordrehaug, M. Ueland and G. Kvåle, JAMA, J. Am. Med. Assoc., 1995, 274, 1526–1533 CrossRef.
- Q. Zhang, D. Yu, S. Ding and G. Feng, Chem. Commun., 2014, 50, 14002–14005 RSC.
- H. Maeda, H. Matsuno, M. Ushida, K. Katayama, K. Saeki and N. Itoh, Angew. Chem., Int. Ed., 2015, 44, 2922–2925 CrossRef PubMed.
- L. Yuan, W. Lin, S. Zhao, W. Gao, B. Chen, L. He and S. Zhu, J. Am. Chem. Soc., 2012, 134, 13510–13523 CrossRef CAS PubMed.
- M. Lan, J. Wu, W. Liu, H. Zhang, W. Zhang, X. Zhuang and P. Wang, Sens. Actuators, B, 2011, 156, 332–337 CrossRef CAS PubMed.
- H. Guo, Y. Jing, X. Yuan, S. Ji, J. Zhao, X. Li and Y. Kan, Org. Biomol. Chem., 2011, 9, 3844–3853 CAS.
- S.-P. Wang, W.-J. Deng, D. Sun, M. Yan, H. Zheng and J.-G. Xu, Org. Biomol. Chem., 2009, 7, 4017–4020 CAS.
- J. Li, C.-F. Zhang, Z.-Z. Ming, W.-C. Yang and G.-F. Yang, RSC Adv., 2013, 3, 26059–26065 RSC.
- W. Lin, L. Long and W. Tan, Chem. Commun., 2010, 46, 1503–1505 RSC.
- D. Yu, F. Huang, S. Ding and G. Feng, Anal. Chem., 2014, 86, 8835–8841 CrossRef CAS PubMed.
- Y. Liu, D. Yu, S. Ding, Q. Xiao, J. Guo and G. Feng, ACS Appl. Mater. Interfaces, 2014, 6, 17543–17550 CAS.
- Z. Huang, S. Ding, D. Yu, F. Huang and G. Feng, Chem. Commun., 2014, 50, 9185–9187 RSC.
- S. Xue, S. Ding, Q. Zhai, H. Zhang and G. Feng, Biosens. Bioelectron., 2015, 68, 316–321 CrossRef CAS PubMed.
- Q. Zhang, Y. Zhang, S. Ding, H. Zhang and G. Feng, Sens. Actuators, B, 2015, 211, 377–384 CrossRef CAS PubMed.
- S. Ding, Q. Zhang, S. Xue and G. Feng, Analyst, 2015, 140, 4687–4693 RSC.
Footnote |
† Electronic supplementary information (ESI) available: Structure characterizations for probe 1, data for investigation of the sensing mechanism, and additional spectra data. See DOI: 10.1039/c5ra11841g |
|
This journal is © The Royal Society of Chemistry 2015 |