DOI:
10.1039/C5RA09099G
(Communication)
RSC Adv., 2015,
5, 62260-62264
Colorimetric detection of Al(III) in vermicelli samples based on ionic liquid group coated gold nanoparticles†
Received
15th May 2015
, Accepted 13th July 2015
First published on 14th July 2015
Abstract
We synthesized ionic liquid (1-ethyl-3-methylimidazolium thiocyanate)-coated gold nanoparticles (IL-Au NPs) using a one-pot method and introduced them in the colorimetric assay for Al3+. IL-Au NPs showed good sensitivity and selectivity. They can determine the concentration of aluminum in vermicelli samples.
Aluminum is the third most abundant element in the earth's crust, which is about 8% of the mass of the surface of the Earth. The chemical properties of aluminum metal are high activity and a cationic state of Al3+.1 As one of the most widely applied metals, aluminum exists in many products around us.2,3 Some aluminum compounds are used as the flocculating agent or swelling agent in producing food like vermicelli, fried bread sticks or other cooked wheat-based food. Meanwhile pharmaceutical drugs in human and veterinary medicines or Chinese tea contain aluminum compounds. Aluminum ion plays important roles in the etiology of neurological disorders, such as Parkinson's disease (PD),4,5 Alzheimer's disease (AD)6–8 and dialysis encephalopathy.9,10 Excess amount of Al3+ is a contributing factor for these diseases. WHO sets the permissible level of Al3+ for drinking water as 7.4 μM.11 Thus the detection of Al3+ is in high demand. Existing methods for detecting Al3+ include atomic absorption spectrometry (AAS),12 inductively coupled plasma atomic emission spectrometry (ICP-AES),13 ICP mass spectrometry (ICP-MS),14 electrochemical methods,15 colorimetric sensors16 and fluorescent analysis.17 These methods have some limitations, e.g., the need for complicated instruments, the requirements for professional operators, and high background signals. Therefore the development of rapid, effective, sensitive and selective strategy for Al3+ sensing is necessary, especially for on-site and home-based assays.
To achieve the efficient and fast determination of metal ions, our group has developed several sensors based on the special physical and chemical properties of the functionalized Au NPs. We design copper ions sensors by click chemistry18 and organic molecular ligands-coated Au NPs.19 Mercury ions can be analyzed by using quaternary ammonium group-capped Au NPs.20 Making use of meso-2,3-dimercaptosuccinic acid (DMSA)-functionalized Au NPs, chromium ions can be determined.21 Besides, we achieve the simultaneous detection of mercury, lead and copper ions by applying protein-capped Au NPs.22 Besides metal ions,23 a variety of other analytes including small molecules,24–27 proteins,28–31 DNA,32,33 and even cancerous cells34,35 could be determined as well by functional Au NPs. The aggregation of Au NPs displays a clear color change, which is useful for visual signal output without complex instruments. Some works have been published on using the color change of Au NPs for detecting Al3+.36–38 The useful Au NPs are usually coated with different ligands like pentapeptide,36 triazole-ether37 and 5-mercaptomethyltetrazole.38 To synthesize these ligands capped Au NPs typically requires complex synthesis. Citrate-coated Au NPs can be used to detect Al3+ directly, but its timing is difficult to control rigorously, because after two minutes Au NPs aggregate even without Al3+.39
Here we present a one-pot synthesis of functional Au NPs for the detection of Al3+. We synthesize ionic liquid (1-ethyl-3-methylimidazolium thiocyanate) groups coated Au NPs (IL-Au NPs) using a one-pot method, which is very straightforward to operate. Ionic liquids are molten salts and some of them are free-flowing liquids at room temperature, having attracted widespread interest in recent years. They are composed solely of cations (like 1,3-dialkylimidazolium, 1-alkylpyridinium and 1,1-dialkylpyrolidinium) and anions ([PF6]−, [BF4]−, SCN− and many other common anions) which can have many different combinations.40 Initially, they are used in traditional chemistry as solvent or catalyst. But in recent years, more new applications have been explored, in which many ionic liquids are employed in functionalizing nanomaterials, which are applied in different areas including fabricating electrochemical biosensors,41 isolating proteins42 and anti-bacteria.43 Ionic liquids can be modified on the surface of nanoparticles, such as Au NPs, quantum dots, to carry out protein assays with the changes of electrochemical signals.44,45 Ionic liquids are helpful for the enhancement of the sensitivity. Besides electrochemical sensors, other kinds of ionic liquid sensors are also reported. Ionic liquid self-assembled monolayers (SAM) can form a surface plasmon resonance (SPR) sensor for proteins.46 A fluoroionphore-ionic liquid probe can detect and extract some heavy metal ions from the aqueous solution.47 These works indicate that ionic liquids can interact with molecules and ions. No attempt is made by utilizing the optical properties of ionic liquid groups coated Au NPs to fabricate colorimetric sensors, which is more practical in the application.
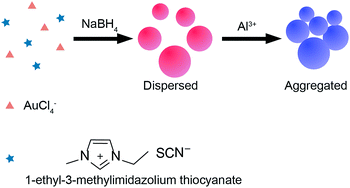 |
| Scheme 1 The synthetic approach of IL-Au NPs and their application in Al3+ sensing. | |
In this work, we demonstrate a colorimetric, ionic liquid-capped Au NPs sensor for Al3+ for the first time (Scheme 1). Through this attempt, we can use Au NPs coated with various ionic liquid groups to develop more colorimetric Au NP based sensors further.
Results and discussion
Synthesis and characterization of IL-Au NPs
Our method to synthesize IL-Au NPs is only one step by reducing Au3+ with NaBH4 using ionic liquid (1-ethyl-3-methylimidazolium thiocyanate) as protecting ligands in an aqueous solution. After the synthesis of IL-Au NPs, we characterized their stability by visual inspection, and we detected the zeta potential. Its zeta potential was −37.5 eV, which was lower than −30 eV. The high absolute value of zeta potential indicates increased electrostatic repulsion to inhibit agglomeration and precipitation, thus the stability of IL-Au NPs is good.48 The good stability is the precondition for the application in sensing. Next we used X-ray photoelectron spectroscopy (XPS) to characterize the surface of Au NPs. After purifying IL-Au NPs, we carried out XPS characterization. Elements Au, N and S (typical element in the ionic liquid) were detected, so we concluded the ionic liquid was present on the surface of Au NPs as a protecting agent (Fig. 1). After confirming that we synthesized ionic liquid capped Au NPs successfully, we tried to explore their application as a colorimetric sensor in screening metal ions in the samples.
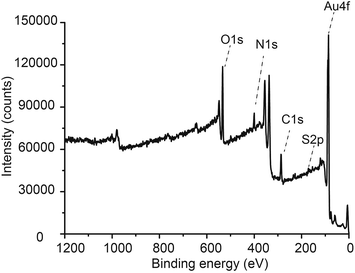 |
| Fig. 1 XPS characterization of ionic liquid groups coated Au NPs. | |
Selectivity of the detection system
To test the response of IL-Au NPs to different metal ions, we added different metal ions (Na+, K+, Fe2+, Fe3+, Al3+, Zn2+, Ca2+, Pb2+, Ba2+, Mn2+, Ni2+, Cr3+, Ce3+, Cu2+, Hg2+, Ag+) into the solution of IL-Au NPs to yield final concentrations of the ions at 10 μM. Interestingly, only Al3+ can cause the aggregation of IL-Au NPs and induce colour change of IL-Au NPs. We recorded the pictures after the addition of different metal ions for 10 minutes (Fig. 2a) and detected the absorption at 520 nm and 650 nm of IL-Au NPs added with metal ions. We calculated the ratio of A650 to A520 (Fig. 2b). The ratio of A650 to A520 for Al3+ was much higher than that for a panel of other cations. The color of IL-Au NPs with the addition of Al3+ changed from red to purple obviously compared with the blank one. Meanwhile the color of IL-Au NPs with the addition of other metal ions almost did not change at all, which means Al3+ can cause the aggregation of IL-Au NPs indeed. Furthermore, we tested the response of IL-Au NPs after adding Na+, K+ with higher concentration of 100 μM and Ca2+, Fe3+ with higher concentration of 20 μM. And no obvious color change was observed (Fig. S1†).
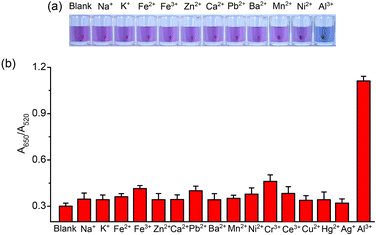 |
| Fig. 2 IL-Au NPs can selectively bind to Al3+. (a) Color change of the IL-Au NPs solution in the presence of partial metallic ions at concentrations of 10 μM, (b) the A650/A520 responses for different metallic ions. | |
To confirm the aggregation of IL-Au NPs caused by Al3+, we scan the UV-vis absorption for IL-Au NPs without and with the addition of Al3+ (10 μM). The color of the solution and UV-vis absorption of the IL-Au NP solution with Al3+ changed obviously compared with the blank one (Fig. S2†). We characterized the TEM image of IL-Au NPs without and with the addition of Al3+ (Fig. S3†). The aggregation of IL-Au NPs with the addition of Al3+ was obvious, which gives us confidence to explore the sensitivity of IL-Au NPs further.
We try to explore the origin of the selectivity of IL-Au NPs. We carried out an XPS analysis of IL-Au NPs, and the data showed some evidence that ionic liquid was covered on the surface of Au NPs. The zeta potential value of IL-Au NPs is negative, which means that the thiocyanate anions are on the outer sphere of Au NPs, and the imidazolium cations may be in the inner sphere. We tried to synthesize the Au NPs with other thiocyanate salts (such as KSCN), however, Au NPs could not be obtained successfully, because KSCN was not a good stabilizer for NPs. The stability of IL-Au NPs should come from the imidazolium group. Due to the interaction between Al and N,49,50 the specificity of IL-Au NPs for Al3+ may come from the synergistic action of cationic and anionic moiety of the ionic liquid, which both have N atom. Aluminum ion is hard acid, and prefers to coordination with hard base, such as N-atom base. Multiple N atoms of the sensor system are helpful to recognize Al3+ compared with other ones.51,52
Sensitivity of the detection system
Besides good selectivity, a satisfying sensitivity is one of the necessary factors for a qualified sensor. To evaluate the minimum detectable concentration of Al3+ in an aqueous solution by color change, different concentrations of AlCl3 were added into the IL-Au NPs solution, and their final concentrations were set to be 0, 1 μM, 2 μM, 5 μM, 10 μM, 20 μM. We found that the color of the solutions changed gradually from red to purple depending on the concentration of Al3+. Fig. 3a shows the color changes of IL-Au NPs dependent on the concentrations of Al3+, which were further confirmed by UV-vis absorption showed in Fig. 3b. The shapes of UV/vis spectra were consistent with the solution color changes. With the addition of Al3+ to IL-Au NPs, the absorption of IL-Au NPs at 520 nm decreased and that of 650 nm increased with the increase of concentrations of Al3+. The plots of A650/A525 versus various concentrations of Al3+ indicated the linear range of the assay. When the concentrations of Al3+ were within the range between 0 μM and 5 μM, the calibration curve displayed a linearity with R2 = 0.9585 (Fig. 3c). Higher A650/A520 values indicated greater degrees of IL-Au NPs aggregation with the higher concentrations of Al3+. Meanwhile the average hydrodynamic diameter of well-dispersed IL-Au NPs and that of the 10 μM Al3+-induced aggregation of IL-Au NPs characterized by DLS were around 22.1 nm and 130.2 nm respectively (Fig. S4†). The lowest concentration based on naked-eye readout is 1 μM, well meeting the WHO standards (∼7.4 μM) for drinking water.
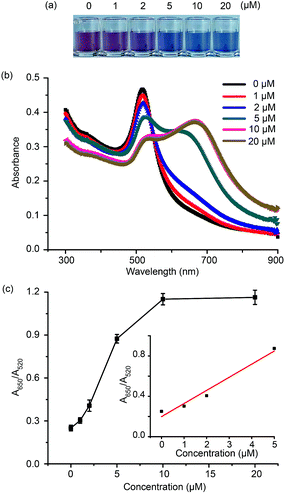 |
| Fig. 3 Sensitivity of IL-Au NPs for Al3+. (a) Photographs of various concentrations of Al3+ from 0 μM to 20 μM in IL-Au NPs, (b) the UV/vis spectra of various concentrations of Al3+ in IL-Au NPs, (c) a dose–response curve for Al3+ detection (A650/A520 vs. Al3+ concentrations), inset: the linear calibration plot for the concentration of Al3+ from 0 μM to 5 μM (A650/A520 vs. Al3+ concentrations). | |
Application of the detection system
To further investigate the potential practical application of this colorimetric assay, we attempted to detect Al3+ in the vermicelli (starchy food, made from sweet potato) sold in the market. In the manufacturing process of vermicelli, alum (its chemical formula is KAl(SO4)2·12H2O) is added as the flocculating agent, but the abuse of alums may be harmful for human health. So the addition of KAl(SO4)2·12H2O in many kinds of food is forbidden in China now. We found the vermicelli sample with very little Al (whose concentration was too low to detect by our method), which came from the supermarket with quality certification. We used HNO3 and H2O2 to digest the vermicelli53 and detected the concentration of metal ions in treated vermicelli sample using ICP-OES (Table S1†). We spiked KAl(SO4)2·12H2O into the treated vermicelli solution to get the linear calibration plot for the concentration of KAl(SO4)2·12H2O. Next, we applied IL-Au NPs to assay the samples, and the final concentrations of KAl(SO4)2·12H2O in the IL-Au NPs were 0, 1 μM, 2 μM, 5 μM, 10 μM. We found that the color of the solutions changed gradually from red to purple depending on the concentration of KAl(SO4)2·12H2O. Fig. 4a showed the color changes of IL-Au NPs dependent on the concentrations of KAl(SO4)2·12H2O, which were further confirmed by UV-vis absorption shown in Fig. 4b. The shapes of UV/vis spectra were consistent with the solution color changes. The plots of A650/A520 versus various concentrations of KAl(SO4)2·12H2O indicated the linear range of the assay. When the concentrations of KAl(SO4)2·12H2O were within the range between 0 μM and 5 μM, the calibration curve displayed a linearity with R2 = 0.9694 (Fig. 4c).
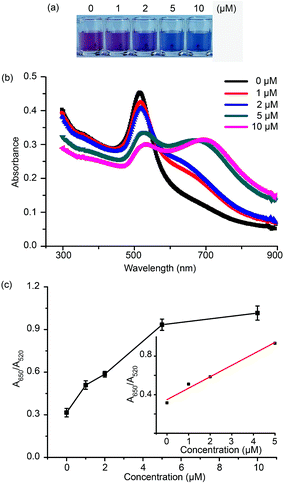 |
| Fig. 4 Sensitivity of IL-Au NPs for KAl(SO4)2·12H2O in spiked vermicelli. (a) Photographs of various concentrations of KAl(SO4)2·12H2O from 0 μM to 10 μM in IL-Au NPs, (b) the UV/vis spectra of various concentrations of KAl(SO4)2·12H2O in IL-Au NPs, (c) a dose–response curve for KAl(SO4)2·12H2O detection (A650/A520 vs. KAl(SO4)2·12H2O concentrations), inset: the linear calibration plot for the concentration of KAl(SO4)2·12H2O from 0 μM to 5 μM (A650/A520 vs. KAl(SO4)2·12H2O concentrations). | |
Next, we tried to detect Al3+ in the vermicelli sample containing alum, which came from a street vendor without quality certification. Our straightforward, naked-eye readout method would make detection of Al3+ easier in the daily life. According to the results of ICP-OES (Fig. 5a), the final concentration of interfering metal ions in the IL-Au NPs did not reach 10 μM, which is 9.57 μM in the detected sample. On the basis of the results of selectivity, 10 μM other metal ions would will not induce the aggregation of IL-Au NPs. 10 μL treated sample solution of vermicelli was added into the IL-Au NPs (1 mL), inducing the aggregation of IL-Au NPs, and the solution color changed from red to purple. UV-vis spectra were consistent with the color changes (Fig. 5b). We employed the value of A650/A520 and the calibration curve obtained in detecting KAl(SO4)2·12H2O (y = 0.1187x + 0.3486) to calculate the concentrations of Al3+ in the vermicelli sample (Fig. 5c). Compared with the ICP-OES result of the concentration of Al3+, the recovery rate was 94.65%.
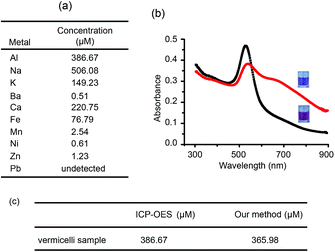 |
| Fig. 5 (a) The concentrations of different metal ions in the commercially available vermicelli detected by ICP-OES, (b) vermicelli sample detection, color change with adding vermicelli containing Al and UV-vis absorbance response, (c) Al3+ determination in the vermicelli containing Al3+. | |
In conclusion, we develop a colorimetric method for the detection of Al3+, which is helpful for monitoring alums in roadside food samples. Meanwhile the application of ionic liquid capped Au NPs in colorimetric sensor expands the application of ionic liquid groups coated nanomaterials. This kind of sensor is easy to operate, and suitable for on-site test. In the future work, through exploring the mechanism of ionic liquid groups coated Au NPs responding to metal ions, we may find out more sensors for metal ions and other analytes, broadening the application of ionic liquid groups coated nanomaterials further.
Acknowledgements
This research was supported by National Natural Science Foundation of China (21222502, 91213305), the Ministry of Science and Technology (2011CB933201), Youth Innovation Promotion Association (CAS), the CAS/SAFEA International Partnership Program for Creative Research Teams, the “Strategic Priority Research Program” of the Chinese Academy of Sciences, Grant No. XDA09030305, National High Technology Research and Development Program of China (2012AA022703).
Notes and references
- M. Witt and H. W. Roesky, Curr. Sci., 2000, 78, 410 CAS.
- B. Bogdanovic and M. Schwickardi, J. Alloys Compd., 1997, 253, 1 CrossRef.
- A. Heinz, A. Haszler, C. Keidel, S. Moldenhauer, R. Benedictus and W. S. Miller, Mater. Sci. Eng., A, 2000, 280, 102 CrossRef.
- S. Sanchez-Iglesias, E. Mendez-Alvarez, J. Iglesias-Gonzalez, A. Munoz-Patino, I. Sanchez-Sellero, J. L. Labandeira-Garcia and R. Soto-Otero, J. Neurochem., 2009, 109, 879 CrossRef CAS PubMed.
- C. Exley and E. R. House, Monatsh. Chem., 2011, 142, 357 CrossRef CAS.
- R. A. Yokel, Neurotoxicology, 2000, 21, 813 CAS.
- T. P. Flaten, Brain Res. Bull., 2001, 55, 187 CrossRef CAS.
- E. Andrasi, N. Pali, Z. Molnar and S. Kosel, J. Alzheimer's Dis., 2005, 7, 273 CAS.
- J. R. McDermott, A. I. Smith, M. K. Ward, I. S. Parkinson and D. N. S. Kerr, Lancet, 1978, 1, 901 CrossRef CAS.
- E. Nathan and S. E. Pedersen, Acta Paediatr. Scand., 1980, 69, 793 CrossRef CAS PubMed.
- WHO, Guidelines for drinking water quality, Geneva, 2008, p. 301 Search PubMed.
- N. Satiroglu and I. Tokgoz, Int. J. Environ. Anal. Chem., 2010, 90, 560 CrossRef CAS PubMed.
- A. V. Karyakin and S. G. Beksaev, Gematol. Transfuziol., 2013, 58, 22 CAS.
- M. H. Nagaoka and T. Maitani, J. Health Sci., 2009, 55, 161–168 CrossRef CAS.
- C. W. K. Chow, S. D. Thomas, D. E. Davey, D. E. Mulcahy and M. Drikas, Anal. Chim. Acta, 2003, 499, 173 CrossRef CAS PubMed.
- S. Sen, T. Mukherjee, B. Chattopadhyay, A. Moirangthem, A. Basu, J. Marek and P. Chattopadhyay, Analyst, 2012, 137, 3975 RSC.
- T. Keawwangchai, N. Morakot and B. Wanno, J. Mol. Model., 2013, 19, 1435 CrossRef CAS PubMed.
- Y. Zhou, S. X. Wang, K. Zhang and X. Y. Jiang, Angew. Chem., Int. Ed., 2008, 47, 7454 CrossRef CAS PubMed.
- D. B. Liu, W. W. Chen, K. Sun, K. Deng, W. Zhang, Z. Wang and X. Y. Jiang, Angew. Chem., Int. Ed., 2011, 50, 4103 CrossRef CAS PubMed.
- D. B. Liu, W. S. Qu, W. W. Chen, W. Zhang, Z. Wang and X. Y. Jiang, Anal. Chem., 2010, 82, 9606 CrossRef CAS PubMed.
- W. W. Chen, F. J. Cao, W. S. Zheng, Y. Tian, Y. L. Xianyu, P. Xu, W. Zhang, Z. Wang, K. Deng and X. Y. Jiang, Nanoscale, 2015, 7, 2042 RSC.
- Y. M. Guo, Z. Wang, W. S. Qu, H. W. Shao and X. Y. Jiang, Biosens. Bioelectron., 2011, 26, 4064 CrossRef CAS PubMed.
- I. L. Lee, Y. M. Sung and S. P. Wu, RSC Adv., 2014, 4, 25251 RSC.
- Y. L. Xianyu, J. S. Sun, Y. X. Li, Y. Tian, Z. Wang and X. Y. Jiang, Nanoscale, 2013, 5, 6303 RSC.
- J. Zhang, L. H. Wang, D. Pan, S. P. Song, F. Y. C. Boey, H. Zhang and C. H. Fan, Small, 2008, 4, 1196 CrossRef CAS PubMed.
- Y. Jiang, H. Zhao, Y. Q. Lin, N. N. Zhu, Y. R. Ma and L. Q. Mao, Angew. Chem., Int. Ed., 2010, 49, 4800 CrossRef CAS PubMed.
- M. L. Yin, L. F. Zhao, Q. Wei and H. Li, RSC Adv., 2015, 5, 32897 RSC.
- W. X. Xue, G. X. Zhang and D. Q. Zhang, Analyst, 2011, 136, 3136 RSC.
- W. S. Qu, Y. Y. Liu, D. B. Liu, Z. Wang and X. Y. Jiang, Angew. Chem., Int. Ed., 2011, 50, 3442 CrossRef CAS PubMed.
- Z. Wu, Z. K. Wu, H. Tang, L. J. Tang and J. H. Jiang, Anal. Chem., 2013, 85, 4376 CrossRef CAS PubMed.
- C. Luo, W. Wen, F. G. Lin, X. H. Zhang, H. S. Gu and S. F. Wang, RSC Adv., 2015, 5, 10994 RSC.
- R. Elghanian, J. J. Storhoff, R. C. Mucic, R. L. Letsinger and C. A. Mirkin, Science, 1997, 277, 1078 CrossRef CAS.
- P. D. Howes, R. Chandrawati and M. M. Stevens, Science, 2014, 346, 1247390 CrossRef PubMed.
- C. D. Medley, J. E. Smith, Z. Tang, Y. Wu, S. Bamrungsap and W. H. Tan, Anal. Chem., 2008, 80, 1067 CrossRef CAS PubMed.
- Y. J. Guo, G. M. Sun, L. Zhang, Y. J. Tang, J. J. Luo and P. H. Yang, Sens. Actuators, B, 2014, 191, 741 CrossRef CAS PubMed.
- X. K. Li, J. N. Wang, L. L. Sun and Z. X. Wang, Chem. Commun., 2010, 46, 988 RSC.
- Y. C. Chen, I. L. Lee, Y. M. Sung and S. P. Wu, Talanta, 2013, 117, 70 CrossRef CAS PubMed.
- D. S. Xue, H. Y. Wang and Y. B. Zhang, Talanta, 2014, 119, 306 CrossRef CAS PubMed.
- S. Chen, Y. M. Fang, Q. Xiao, J. Li, S. B. Li, H. J. Chen, J. J. Sun and H. H. Yang, Analyst, 2012, 137, 2021 RSC.
- T. Welton, Coord. Chem. Rev., 2004, 248, 2459 CrossRef CAS PubMed.
- K. Kwak, S. S. Kumar, K. Pyo and D. Lee, ACS Nano, 2014, 8, 671 CrossRef CAS PubMed.
- L. Han, Y. Shu, X. F. Wang, X. W. Chen and J. H. Wang, Anal. Bioanal. Chem., 2013, 405, 8799 CrossRef CAS PubMed.
- R. S. Patil, M. R. Kokate, P. P. Salvi and S. S. Kolekar, C. R. Chim., 2011, 14, 1122 CrossRef CAS PubMed.
- R. Li, K. B. Wu, C. X. Liu, Y. Huang, Y. Y. Wang, H. F. Fang, H. J. Zhang and C. Y. Li, Anal. Chem., 2014, 86, 5300 CrossRef CAS PubMed.
- X. Yu, Y. Wang, X. Chen, K. Wu, D. Chen, M. Ma, Z. Huang, W. Wu and C. Li, Anal. Chem., 2015, 87, 4237 CrossRef CAS PubMed.
- M. Ratel, A. Provencher-Girard, S. S. Zhao, J. Breault-Turcot, J. Labrecque-Carbonneau, M. Branca, J. N. Pelletier, A. R. Schmitzer and J. F. Masson, Anal. Chem., 2013, 85, 5770 CrossRef CAS PubMed.
- Z. Jin, D. X. Xie, X. B. Zhang, Y. J. Gong and W. H. Tan, Anal. Chem., 2012, 84, 4253 CrossRef CAS PubMed.
- D. Hanaor, M. Michelazzi, C. Leonelli and C. C. Sorrell, J. Eur. Ceram. Soc., 2012, 32, 235 CrossRef CAS PubMed.
- P. G. Ding, J. H. Wang, J. Y. Cheng, Y. F. Zhao and Y. Ye, New J. Chem., 2015, 39, 342 RSC.
- S. Sasic, D. Zoric, M. Jeremic and A. Antic-Jovanovic, Polyhedron, 2001, 20, 839 CrossRef CAS.
- J. Q. Wang, L. Huang, L. Gao, J. H. Zhu, Y. Wang, X. X. Fan and Z. G. Zou, Inorg. Chem. Commun., 2008, 11, 203 CrossRef CAS PubMed.
- Y. H. Ma, R. Yuan, Y. Q. Chai and X. L. Liu, Mater. Sci. Eng., C, 2010, 30, 209 CrossRef CAS PubMed.
- A. A. Momen, G. A. Zachariadis, A. N. Anthemidis and J. A. Stratis, Anal. Chim. Acta, 2006, 565, 81 CrossRef CAS PubMed.
Footnote |
† Electronic supplementary information (ESI) available: Experimental section, pictures, UV-vis absorption, TEM images and DLS results for IL-Au NPs before and after adding Al3+, ICP-OES result of vermicelli sample with very little Al are provided. See DOI: 10.1039/c5ra09099g |
|
This journal is © The Royal Society of Chemistry 2015 |