DOI:
10.1039/C5RA08478D
(Paper)
RSC Adv., 2015,
5, 53802-53808
A facile one-step spray-coating process for the fabrication of a superhydrophobic attapulgite coated mesh for use in oil/water separation†
Received
7th May 2015
, Accepted 4th June 2015
First published on 4th June 2015
Abstract
Oil/water separation is a worldwide problem due to the increasing emission of industrial oily waste water and the frequent oil spill accidents. Herein, we demonstrate, for the first time, superhydrophobic attapulgite coated mesh films for gravity driven oil water separation, which were fabricated by a facile one-step spray-coating process. The as-prepared attapulgite coated mesh films show both superhydrophobic and superoleophilic properties simultaneously with a high water contact angle of 155° ± 1° and an oil contact angle of 0°. Thus, they can be used to separate a series of oil/water mixtures, such as kerosene, chloroform, and petroleum ether with separation efficiency up to 97% for the kerosene/water mixture. In addition, the as-prepared coated mesh still maintained separation efficiency above 94% and stable recyclability after 40 separation cycles with the surface morphology of the attapulgite coated mesh nearly unchanged. Besides, the separation mechanism for the oil/water mixture was elaborated by interpreting the different states of water droplet on the surface before and during separation, which has been discussed scarcely. Furthermore, the as-prepared attapulgite coated mesh could keep its superhydrophobic property under various harsh conditions, not only for pure water but also for corrosive acidic, alkaline and salt solutions, which suggests attractive potential for practical oil/water separation in industry and everyday life.
1. Introduction
Oil/water separation has become an increasingly important and urgent issue in modern chemical industrial processes and environmental protection due to increasing industrial oily wastewater as well as frequent oil spill accidents.1 Because oil/water separation is governed by interfacial phenomena, using special wettability to design novel materials is a facile and effective approach.2–4 Recently, surfaces with superhydrophobic and superoleophilic properties have attracted extensive interest in the field of oil/water separation because of their capacity to absorb only oil while repelling water completely, thus exhibiting high oil/water separation efficiency and selectivity.5–12 Inspired by the superhydrophobic surface of the lotus leaf, a variety of approaches have been developed to fabricate superhydrophobic materials for the separation of water and oil, including chemical etching, electrospinning techniques, sol–gel, hydrothermal methods and self-assembly processes.13–24 However, most of the methods used for the preparation of such surfaces always involve two steps processes: creating a hierarchical rough surface and then chemically modifying the surface with low surface energy materials, and only a few studies have been reported for the fabrication of these surfaces in one single step.25,26 Therefore, if both surface roughness and a low energy surface could be obtained in just one step, the process of fabricating superhydrophobic surfaces would be simplified.
To date, numerous functional materials with various hybrid structures have been developed with the aim of realizing a high performance water/oil separation utilizing their special wettability properties, such as ZnO,27 TiO2,28,29 kapok,30 hydrophobic aerogel,31 polyvinylidene fluoride (PVDF),32 carbon-based materials,33,34 and magnetic materials.35,36 However, majority of the materials reported for the separation of oil from water generally cannot be used in the corrosive environment of a strong acid or base and thus suffer from the limitation of recyclability. For instance, the traditional ZnO, TiO2 or PVDF can be degraded by strong acid solution, consequently limiting their practical applications. Therefore, there is a great need to design chemically resistant inorganic films for practical applications in oil/water separation, which are not only suitable for all pH environments, but also realize a highly efficient oil/water separation. Among the materials already known in nature, attapulgite is a type of hydrated magnesium aluminum silicate mineral with exchangeable cations in its framework channels and reactive –OH groups on its surface, which has been applied in a wide range of fields.37–43 Compared with other materials used for the fabrication of superhydrophobic films, the advantages of attapulgite include its chemical inertness, extensive sources, as well as cheapness, and thus potential mass industrial application. Recently, there have been few reports on superhydrophobic attapulgite surfaces, and much of the work is directed to the creation of self-cleaning surfaces.44,45 However, to the best of our knowledge, superhydrophobic attapulgite coated mesh used for oil/water separation has not been reported. Moreover, the mechanism of the oil/water separation process is still unclear, and its understanding needs to be improved.46,47
In the present study, the fabrication of APT-coated mesh films with robust superhydrophobic and superoleophilic properties is reported using a facile one-step process by spraying hydrophobic attapulgite (APT) modified with low energy material, such as octadecyltrichlorosilane (OTCS) and waterborne polyurethane (PU) mixtures, onto stainless steel mesh, eliminating the complexity of two different steps involved in the conventional methods. The waterborne PU was added to increase the binding force between the APT powders and stainless steel mesh. The spray-coating is a fairly facile and commercially available method for widespread applications, which is not specific to a particular substrate and can be easily applied to a large surface area.48,49 Because water remained exclusively on the APT-coated mesh surfaces and oil permeated through the mesh surfaces quickly, the as-prepared superhydrophobic APT-coated mesh could be successfully used for the separation of oil from water. Furthermore, the as-prepared APT-coated mesh maintained high separation efficiency and stable recyclability after separating experiments were repeated 40 times. Besides, the separation mechanism for the oil/water mixture was elaborated by interpreting the different states of water droplet on the surface before and during separation. More importantly, the as-prepared APT-coated mesh film possesses superhydrophobic properties not only for pure water but for water in corrosive acidic, alkaline and salt solutions. This means that the as-prepared APT-coated mesh could be better applied in a realistic environment and even under harsh conditions.
2. Experimental
Materials
APT micro-powder supplied from Jiuchuan Clay Technology Co., Jiangsu, China, is composed of CaO (1.29%), Al2O3 (10.47%), Na2O (1.52%), MgO (20.41%), SiO2 (64.31%), K2O (0.13%) and Fe2O3 (0.87%). The APT powder was first activated with a 4 M HCl solution before use. Octadecyltrichlorosilane (OTCS) was obtained from Alfa Aesar. Waterborne polyurethane (PU) was purchased from Sinopharm Chemical Reagent Co., Ltd.
Preparation of superhydrophobic mesh
The synthesis of hydrophobic APT powder was a key part of our strategy for the fabrication of superhydrophobic APT-coated mesh. The hydrophilic APT powder was modified with sufficient amount of OTCS. In a typical procedure, 1 g of APT powder and 40 mL of toluene were placed into a round-bottomed flask. After adding 1 mL of OTCS, they were refluxed for 3 h. The procedure imparts hydrophobicity to the APT powders by immobilizing octadecyltrichloro groups on their surface. After filtration, the as-obtained APT powder was dried at 353 K and ground to a finer powder using a mortar. It is important to perform multistep washing and centrifugation to ensure the complete removal of the unreacted and partially functionalized APT powder. The fabrication process of superhydrophobic APT-coated mesh is shown in Fig. 1. 0.05 g of waterborne polyurethane (PU) was first dissolved in 20 mL acetone and then 0.3 g APT powder was dispersed in the solution and stirred with a magnetic stirrer for at least 1 h to obtain a homogeneous suspension. The suspension was then sprayed onto the stainless steel mesh substrate (200 mesh size) with 0.2 MPa compressed air gas using a spray gun. Finally, the APT-coated mesh surface was dried at ambient temperature for 1 h to allow the acetone to evaporate completely.
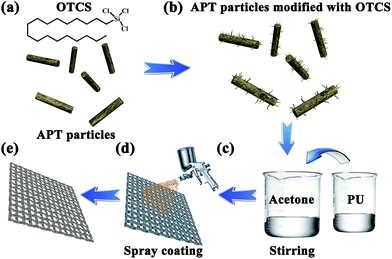 |
| Fig. 1 Schematic illustration of the fabrication of superhydrophobic APT-coated mesh through a facile one-step spray-coating process. | |
Oil/water separation
Five types of oils and organic solvents, including kerosene, chloroform, petroleum ether, tetrachloroethane and toluene, were used in this study. They were colored with Oil Red O and mixed with water that was colored with methylene blue. For oil/water separation, the superhydrophobic APT-coated mesh was fixed between two Teflon fixtures, both of which were fitted with glass tubes. The device was obliquely fixed to make it easier for oil to be in good contact with the mesh film, because the density of the oil tested in these cases is less than that of water. Mixtures of oil and water (50% v/v) were poured slowly into a test tube through the APT-coated mesh. The driving force during the separation process is gravity. The separation efficiency was calculated according to η = (m1/m0) × 100, where m0 and m1 are the mass of the water before and after the separation process, respectively.50
Recycling test
The kerosene/water mixture was used to test the separation efficiency of the as-prepared coated mesh versus the recycle number during oil/water separation. After each oil/water separation experiment, the contaminated mesh was rinsed with alcohol to remove the absorbed oil. Subsequently, the cleaned mesh was dried in an oven at 80 °C for 30 min and the superhydrophobicity and the superoleophilicity could be recovered.
Chemical stability test
Different water droplets with pH values ranging from 1 to 14 were used to test the chemical stability of the coated mesh. The chemical stability performance of the as-prepared APT-coated mesh was also studied by immersing the coated mesh into corrosive media of 1 M HCl, 1 M NaCl, 1 M NaOH, acetone and toluene for 24 h and then cleaning with deionized water.
Characterization
The morphological structures of the as-prepared surfaces were examined by field emission scanning electron microscopy (FE-SEM, JSM-6701F). The phase structure of the as-prepared samples was characterized by an X-ray diffractometer (XRD) (Rigaku Corp., D/max-2400) equipped with graphite monochromatized Cu Kα radiation. Fourier transform infrared (FT-IR) spectroscopy was performed with a Bio-Rad FTS-165 instrument. The water and oil contact angle, sliding angle and slipping angle were measured with a SL200KB apparatus at ambient temperature. The volume of the individual water and oil droplets in all the measurements was 5 μL. The average water/oil contact and water sliding and slipping values were obtained by measuring the same sample in at least five different positions.
3. Results and discussion
A superhydrophobic APT-coated mesh was created by the subsequent introduction of roughness and low energy surface via a facile, one-step spray-coating deposition of hydrophobic APT powder (Fig. 1). The waterborne PU was added to increase the binding force between the APT powder and stainless steel mesh. The XRD pattern of APT is shown in Fig. 2a. The XRD patterns of the APT demonstrate a (110) characteristic diffraction peak at 8.4° of APT, revealing that the structure of APT is maintained very well in the preparation process.41 The FT-IR spectra of APT before and after modification with OTCS are shown in Fig. 2b. In the spectrum of APT, the absorption bands at 3614, 3551 and 1636 cm−1 are ascribed to the –OH stretching and bending vibrations of APT, and the absorption bands at 1031 and 981.5 cm−1 are ascribed to the Si–OH groups of APT.44 After modification with OTCS, besides the –OH and Si–OH groups, new bands at 2926, 2855 cm−1 (the asymmetric and symmetric stretching of CH2) and 1465 cm−1 (the bending of CH2) appeared in the spectra of modified APT, indicating that the APT was successfully modified with the low energy material, OTCS.11,51
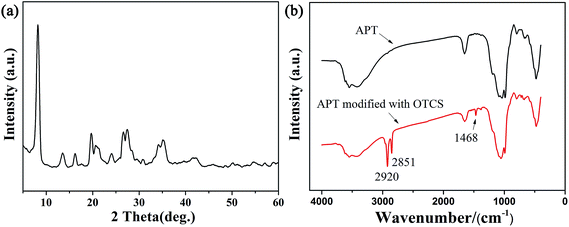 |
| Fig. 2 (a) XRD pattern of the APT and (b) FT-IR spectra of APT and APT modified with OTCS. | |
The surface morphologies of the pristine and the coated meshes were investigated by FE-SEM. Fig. 3a is a typical image of the porous stainless steel mesh substrate with an average diameter of approximately 75 μm (200 mesh size), and the mesh wires have a diameter of 60 μm. The magnified view in the inset of Fig. 3a reveals that the original wires have smooth surfaces. After coating with the mixture of hydrophobic APT and PU, the macroscopic morphology of the mesh shows little change (Fig. 3b). The low-magnification image of Fig. 3c shows that the APT is densely and randomly distributed on the surface of the wires at the microscale. From the highly magnified FE-SEM image of Fig. 3d, it is obvious that the APT was relatively aggregated with the diameter of a single fibril being about 100 nm and the length of a single fibril being about several hundred nanometers, leading to the hierarchical micro- and nano-scale roughness on the APT-coated meshes. This hierarchical micro- and nano-scale roughness of the APT-coated mesh surface is essential for superhydrophobicity.
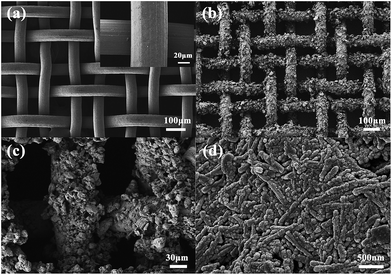 |
| Fig. 3 FE-SEM images of (a) the original stainless steel mesh and (b–d) the as-prepared APT-coated mesh surface at low and high magnifications, respectively. The inset (a) is the magnified image of the original mesh. | |
The wettability properties of the APT-coated meshes were evaluated by water and oil contact angle measurements. The as-prepared APT-coated mesh showed superhydrophobic and superoleophilic properties simultaneously with a water contact angle as high as 158° ± 1° and a sliding angle as low as 8° (Fig. 4a and S1, ESI†) and an oil (kerosene) contact angle of 0° (Fig. 4b). Thus, the water droplet was repelled and stayed on the APT-coated mesh with a highly spherical shape, while oil droplets permeated through the mesh quickly within a few seconds (Fig. 4c).
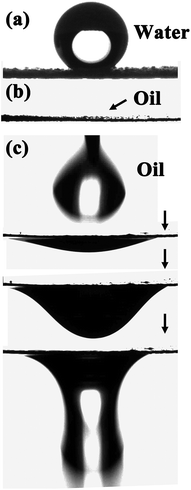 |
| Fig. 4 The as-prepared APT-coated mesh shows both superhydrophobic and superoleophilic properties. (a) Shape of a water droplet on the coating mesh with a contact angle of 158° ± 1°; (b) shape of an oil droplet on the coating mesh with a contact angle close to zero; (c) spreading and permeating behavior of kerosene droplets on the coating mesh. The kerosene oil spreads and penetrates through the mesh quickly (within few seconds). | |
The schematic of the separation mechanism for the oil/water mixture is shown in Fig. 5. Before separation, when a water droplet was dropped onto the as-prepared APT-coated mesh surface, it was thought to be in Cassie state and could easily move away due to the low adhesive property of the as-prepared superhydrophobic APT-coated mesh (Fig. 5, left). Moreover, the oil droplet could quickly permeate through the superoleophilic APT-coated mesh. However, the situation is quite different during the separation. When the oil permeated through the superhydrophobic APT-coated mesh, the mesh surface was wetted by oil. The rough structure on the mesh surface was filled with oil and it became relative flat; at this time, when a water droplet dropped onto the surface, it preferred to maintain the Wenzel state with a low water contact angle about 75° (Fig. 5, right). Moreover, the surface wetted by oil facilitated easy flow for water with a water slipping angle as low as 10° owing to the low resistance between the water and the oil-wetted surface (the lower right corner of Fig. 5 and Movie S1, ESI†). After separation, the APT-coated mesh recovered its superhydrophobic and superoleophilic properties, when the contaminated mesh was thoroughly rinsed with ethanol and deionized water to remove the oil residuals (Fig. S2, ESI†). Thus, the as-prepared superhydrophobic–superoleophilic APT-coated mesh could be successfully used for the separation of oil and water mixtures.
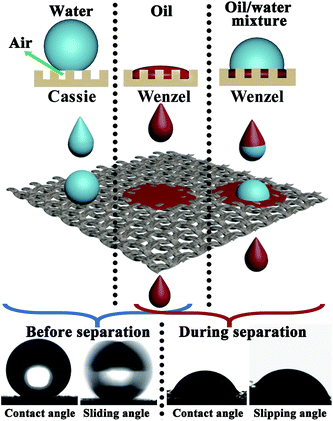 |
| Fig. 5 The separation mechanism for the oil/water mixture. | |
The as-prepared APT-coated mesh has great potential to separate oil/water mixtures due to the robust superhydrophobic and superoleophilic properties. A series of studies was carried out to test the oil/water separation capacities of the as-prepared APT-coated mesh. As shown in Fig. 6a and b, the coated mesh was fixed between two Teflon fixtures, both of which were fitted with glass tubes. Because the density of the tested oil, kerosene in this case, is less than that of water, the device was obliquely fixed to make it easier for the oil to be in good contact with the mesh film. As the mixture of kerosene (dyed with Oil Red O) and water (dyed with methylene blue) was poured onto the APT-coated mesh film, kerosene passed through the mesh quickly with the driving force of gravity, while water was repelled and remained in the upper glass tube. More importantly, neither oil nor water is observed in the collected water or oil, respectively, indicating a high purity and effectiveness of the separation of the oil/water mixture (see details in the Movie S2, ESI†). Mixtures of chloroform/water, petroleum ether/water, tetrachloroethane/water, and toluene/water were also successfully separated with high efficiency. The separation efficiency of the as-prepared APT-coated mesh for the series of oil/water mixtures is shown in Fig. 6c. The separation efficiency was calculated according to η = (m1/m0) × 100, where m0 and m1 are the mass of the water before and after the separation process, respectively. The separation efficiency of the APT-coated mesh was calculated to be up to 97% for the kerosene/water mixture and above 93% for other oils. After each oil/water separation experiment, the contaminated mesh was rinsed with alcohol to remove the absorbed oil. The APT-coated meshes still retain high separation efficiency after 40 separation cycles and efficiency was always above 94% for the kerosene/water mixture, indicating the good recyclability of the as-prepared APT-coated meshes (Fig. 6d). In addition, the surface morphology of the APT-coated mesh was not destroyed (Fig. 6e). The inset in Fig. 6e illustrates that the surface of the mesh maintains highly hydrophobicity with a water contact angle of about 142° after 40 cycles of use, showing its prolonged wettability.
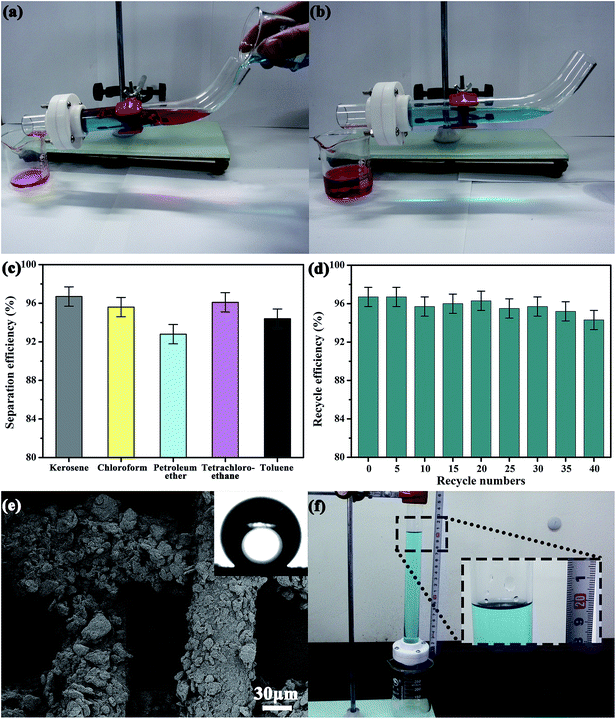 |
| Fig. 6 Oil/water separation studies of the as-prepared APT-coated mesh (water is dyed with methylene blue and oil is dyed with Oil Red O to enhance the visual effect): (a) during separation and (b) after separation; (c) the separation efficiency of APT-coated mesh for a selection of oil and water; (d) separation efficiency remains high after using 40 times by taking kerosene/water mixture as an example; (e) surface morphology has no change after 40 times use. The inset illustrates the shape of a water droplet on the mesh after use 40 times; (f) the intrusion pressure of water. | |
To further study the separation ability of the as-prepared APT-coated meshes, the intrusion pressure of water was introduced. The maximum height of water that the APT-coated mesh can support determines the intrusion pressure of water. The intrusion pressure (P) was calculated by the following equation:
where
ρ is the density of water,
g is the acceleration of gravity, and
hmax is the maximum height of water the APT-coated mesh can support. As shown in
Fig. 6f, the average maximum bearable height achieved is 20.4 cm, and the intrusion pressure is about 2.0 kPa. Water does not flow through the mesh at pressures below this. Oil flux is another evaluation for the oil/water separation mesh. The oil (kerosene) flux was measured under a fixed column of oil from 5 experiments. The kerosene permeates through the APT-coated mesh with an average flux as high as 20 L m
−2 s
−1. Consequently, the as-prepared APT-coated mesh is capable of separating a large amount of oil/water mixtures.
The as-prepared APT-coated meshes showed stable superhydrophobicity towards many corrosive solutions such as acidic, basic and salt solutions. Fig. 7a shows the relationship between pH values and CAs on the as-obtained APT-coated meshes. The as-prepared APT-coated meshes show superhydrophobicity at pH values ranging from 1 to 14 (within experimental error, at all pH values), showing superhydrophobicity not just for pure water but also for corrosive liquids, such as acidic, basic and some aqueous salt solutions (Fig. 7a and S3, ESI†). Furthermore, the as-prepared APT-coated meshes also show remarkable stability of wettability to harsh conditions, such as corrosive solutions (1 M HCl, NaCl and NaOH), acetone and toluene when the APT-coated meshes were immersed into the corresponding solutions for 24 h. Surprisingly, superhydrophobicity is still retained with water contact angles larger than 150° after cleaning with deionized water, showing robust chemical resistance to the extreme conditions (Fig. 7b and S4, ESI†). The chemical inertness of APT-coated mesh is a very vital consideration in practical applications, which can be attributed to the unique chemical properties of the APT-coated meshes.
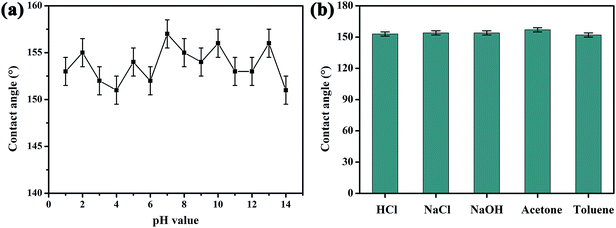 |
| Fig. 7 (a) Variation of water CAs on the as-prepared superhydrophobic APT-coated mesh as a function of pH value. (b) Water CAs on the treated superhydrophobic APT-coated mesh after being immersed in 1 M HCl, 1 M NaCl, 1 M NaOH, acetone and toluene for 24 h. | |
4. Conclusions
In summary, robust and chemically stable APT-coated meshes with superhydrophobic and superoleophilic properties have been fabricated by a facile one-step spray-coating approach. The as-prepared superhydrophobic APT-coated meshes can separate oils from water with high separation efficiency of up to 97% for the kerosene/water mixture. This process can be repeated for more than 40 times with stable high hydrophobicity and nearly constant high separation efficiency. Besides, the separation mechanism for the oil/water mixture was elaborated by interpreting the different states of water droplet on the surface before and during separation. In addition, the obtained APT-coated mesh can possess superhydrophobic properties not only for pure water but also for corrosive solutions under acidic, basic and salt conditions. Therefore, the excellent stability of the superhydrophobic APT-coated meshes has applications under realistic environments and even under harsh conditions.
Acknowledgements
The National Nature Science Foundation of China (Grant no. 21301141), the program for Changjiang Scholars and Innovative Research Team in University, China (IRT1177), the Nature Science Foundation of Gansu Province, China (145RJYA241), and the Scientific and Technical Innovation Project of Northwest Normal University (NWNU-LKQN-12-6) have financially supported this work.
References
- M. A. Shannon, P. W. Bohn, M. Elimelech, J. G. Georgiadis, B. J. Marinas and A. M. Mayes, Nature, 2008, 452, 301 CrossRef CAS PubMed.
- B. Wang, W. Liang, Z. Guo and W. Liu, Chem. Soc. Rev., 2015, 44, 336 RSC.
- Z. Xue, Y. Cao, N. Liu, L. Feng and L. Jiang, J. Mater. Chem. A, 2014, 2, 2445 CAS.
- Y. Xiang, J. Shen, Y. Wang, F. Liu and L. Xue, RSC Adv., 2015, 5, 23530 RSC.
- L. Feng, Z. Zhang, Z. Mai, Y. Ma, B. Liu, L. Jiang and D. Zhu, Angew. Chem., Int. Ed., 2004, 43, 2012 CrossRef CAS PubMed.
- P. Mishra and K. Balasubramanian, RSC Adv., 2014, 4, 53291 RSC.
- Y. Cao, X. Zhang, L. Tao, K. Li, Z. Xue, L. Feng and Y. Wei, ACS Appl. Mater. Interfaces, 2013, 5, 4438 CAS.
- A. Li, H. X. Sun, D. Z. Tan, W. J. Fan, S. H. Wen, X. J. Qing, G. X. Li, S. Y. Li and W. Q. Deng, Energy Environ. Sci., 2011, 4, 2062 CAS.
- L. Peng, H. Li, Y. Zhang, J. Su, P. Yu and Y. Luo, RSC Adv., 2014, 4, 46470 RSC.
- L. Li, B. Li, L. Wu, X. Zhao and J. Zhang, Chem. Commun., 2014, 50, 7831 RSC.
- J. Li, L. Yan, Y. Zhao, F. Zha, Q. Wang and Z. Lei, Phys. Chem. Chem. Phys., 2015, 17, 6451 RSC.
- B. Cortese, D. Caschera, F. Federici, G. M. Ingoc and G. Gigliade, J. Mater. Chem. A, 2014, 2, 6781–6789 CAS.
- C. Wang, T. Yao, J. Wu, C. Ma, Z. Fan, Z. Wang, Y. Cheng, Q. Lin and B. Yang, ACS Appl. Mater. Interfaces, 2009, 1, 2613 CAS.
- J. Lin, B. Ding, J. Yang, J. Yu and G. Sun, Nanoscale, 2012, 4, 176 RSC.
- J. Wu, N. Wang, L. Wang, H. Dong, Y. Zhao and L. Jiang, ACS Appl. Mater. Interfaces, 2012, 4, 3207 CAS.
- S. Tao, Y. Wang and Y. An, J. Mater. Chem., 2011, 21, 11901 RSC.
- C. Gao, Z. Sun, K. Li, Y. Chen, Y. Cao, S. Zhang and L. Feng, Energy Envrion. Sci., 2013, 6, 1147 RSC.
- X. Liu, L. Ge, W. Li, X. Wang and F. Li, ACS Appl. Mater. Interfaces, 2015, 7, 791–800 CAS.
- M. Li, J. Xu and Q. Lu, J. Mater. Chem., 2007, 17, 4772 RSC.
- Y. Shang, Y. Si, A. Raza, L. Yang, X. Mao, B. Ding and J. Yu, Nanoscale, 2012, 4, 7847 RSC.
- Q. Zhu and Q. Pan, ACS Nano, 2014, 8, 1402 CrossRef CAS PubMed.
- V. H. Pham and J. H. Dickerson, ACS Appl. Mater. Interfaces, 2014, 6, 14181 CAS.
- Y. Lu, S. Sathasivam, J. Song, F. Chen, W. Xu, C. J. Carmalt and I. P. Parkin, J. Mater. Chem. A, 2014, 2, 11628 CAS.
- X. Zhou, Z. Zhang, X. Xu, F. Guo, X. Zhu, X. Men and B. Ge, ACS Appl. Mater. Interfaces, 2013, 5, 7208 CAS.
- Q. An, Y. Zhang, K. Lv, X. Luan, Q. Zhang and F. Shi, Nanoscale, 2015, 7, 4553 RSC.
- J. Song, S. Huang, Y. Lu, X. Bu, J. E. Mates, A. Ghosh, R. Ganguly, C. J. Carmalt, I. P. Parkin, W. Xu and C. M. Megaridis, ACS Appl. Mater. Interfaces, 2014, 6, 19858 CAS.
- D. Tian, X. Zhang, X. Wang, J. Zhai and L. Jiang, Phys. Chem. Chem. Phys., 2011, 13, 14606 RSC.
- M. A. Gondal, M. S. Sadullah, M. A. Dastageer, G. H. McKinley, D. Panchanathan and K. K. Varanasi, ACS Appl. Mater. Interfaces, 2014, 6, 13422 CAS.
- J. Y. Huang, S. H. Li, M. Z. Ge, L. N. Wang, T. L. Xing, G. Q. Chen, X. F. Liu, S. S. Al-Deyab, K. Q. Zhang, T. Chen and Y. K. Lai, J. Mater. Chem. A, 2015, 3, 2825 CAS.
- X. Huang and T. T. Lim, Desalination, 2006, 190, 295 CrossRef CAS PubMed.
- J. T. Korhonen, M. Kettunen, R. H. A. Ras and O. Ikkala, ACS Appl. Mater. Interfaces, 2011, 3, 1813 CAS.
- M. Tao, L. Xue, F. Liu and L. Jiang, Adv. Mater., 2014, 26, 2943 CrossRef CAS PubMed.
- H. Sun, A. Li, Z. Zhu, W. Liang, X. Zhao, P. La and W. Deng, ChemSusChem, 2013, 6, 1057 CrossRef CAS PubMed.
- Y. Dong, J. Li, L. Shi, X. Wang, Z. Guo and W. Liu, Chem. Commun., 2014, 50, 5586 RSC.
- L. Wu, L. Li, B. Li, J. Zhang and A. Wang, ACS Appl. Mater. Interfaces, 2015, 7, 4936 CAS.
- J. Wu, N. Wang, Y. Zhao and L. Jiang, Nanoscale, 2015, 7, 2625 RSC.
- J. Zhang, Q. Wang and A. Wang, Carbohydr. Polym., 2007, 68, 367 CrossRef CAS PubMed.
- J. Huang, Y. Liu, Q. Jin, X. Wang and J. Yang, J. Hazard. Mater., 2007, 143, 541 CrossRef CAS PubMed.
- H. Chen, Y. Zhao and A. Wang, J. Hazard. Mater., 2007, 149, 246 CrossRef PubMed.
- W. Wang and A. Wang, Carbohydr. Polym., 2010, 82, 83 CrossRef CAS PubMed.
- B. Mu and A. Wang, J. Mater. Chem. A, 2015, 3, 281 CAS.
- H. Liu, T. Chen, D. Chang, D. Chen, D. Kong, X. Zou and R. L. Frost, Chem. Eng. J., 2012, 188, 108 CrossRef CAS PubMed.
- L. An, Y. Pan, X. Shen, H. Lu and Y. Yang, J. Mater. Chem., 2008, 18, 4928 RSC.
- B. Li, J. Zhang, L. Wu and A. Wang, ChemPlusChem, 2013, 78, 1503 CrossRef CAS PubMed.
- W. Liang, Y. Liu, H. Sun, Z. Zhu, X. Zhao, A. Li and W. Deng, RSC Adv., 2014, 4, 12590 RSC.
- K. Li, X. Zeng, H. Li, X. Lai and H. Xie, Mater. Lett., 2014, 120, 255 CrossRef CAS PubMed.
- E. Bormashenko, S. Balter, Y. Bormashenko and D. Aurbach, Colloids Surf., A, 2012, 415, 394 CrossRef CAS PubMed.
- J. Li, Z. Jing, F. Zha, Y. Yang, Q. Wang and Z. Lei, ACS Appl. Mater. Interfaces, 2014, 6, 8868 CAS.
- J. Li, L. Yan, Q. Ouyang, F. Zha, Z. Jing, X. Li and Z. Lei, Chem. Eng. J., 2014, 246, 238 CrossRef CAS PubMed.
- Q. M. Pan, M. Wang and H. B. Wang, Appl. Surf. Sci., 2008, 254, 6002 CrossRef CAS PubMed.
- M. E. Yazdanshenas and M. Shateri-Khalilabad, Ind. Eng. Chem. Res., 2013, 52, 12846 CrossRef CAS.
Footnote |
† Electronic supplementary information (ESI) available. See DOI: 10.1039/c5ra08478d |
|
This journal is © The Royal Society of Chemistry 2015 |
Click here to see how this site uses Cookies. View our privacy policy here.