DOI:
10.1039/C5RA08164E
(Paper)
RSC Adv., 2015,
5, 68369-68377
Investigation on the aggregation behavior of photo-responsive system composed of 1-hexadecyl-3-methylimidazolium bromide and 2-methoxycinnamic acid
Received
4th May 2015
, Accepted 27th July 2015
First published on 27th July 2015
Abstract
A novel fluid system composed of 2-methoxycinnamic acid (trans-OMCA) and 1-hexadecyl-3-methylimidazolium bromide (C16mimBr) in an aqueous solution was investigated. The compounds trans-OMCA and C16mimBr in an aqueous solution can self-assemble and form viscoelastic worm-like micelles. The concentrations of trans-OMCA and C16mimBr have a significant influence on the rheological properties of the system. The samples were characterized by rheological measurements. The structural isomerization of trans-to-cis for trans-OMCA occurred after UV light irradiation. The transformation of the system after UV light irradiation was determined by UV-vis absorption spectroscopy, rheological measurement and cryo-TEM observation. Surface tension measurements were carried out to investigate the role of trans-OMCA and UV light in C16mimBr aqueous solution. Critical aggregation concentration (cac), effectiveness of surface tension reduction (Πcac), maximum excess surface concentration (Γmax) and minimum area occupied per surfactant molecule (as) were investigated. Critical packing parameter was introduced to express the mechanism of aggregation behavior transition.
1. Introduction
Surfactants can aggregate into highly organized structures, for example, micelles, fibers, vesicles, and lamellar phases.1–4 As one of the most important structures, worm-like micelles have received considerable attention because of their particular micellar structure and unique rheological response.5–9 These micelles with flexible and elongated characters can easily entangle into a dynamic reversible network structure. The structures can break and reform; in this way, they are usually called “living” or “equilibrium” polymers.10–12 Worm-like micelles can be applied in wide-spread fields and offer great potential in numerous aspects because of their fascinating properties, for example, rheology control, drag reduction agent, heat transfer, drug delivery, and enhanced oil recovery.13–15
Scientists have already focused on craft adaptable materials inspired by nature.16–18 The structure or functionality of these materials can be dynamically altered when stimulated by the factors, such as light, temperature, electricity, and pH.19–27 Among these systems formed by the environmentally stimulated materials, smart worm-like micelles are a particular area of highly promising development.28 The smart worm-like systems usually rely on small amphiphilic molecules and their self-assembly. The structural change may lead to macroscopic characteristic variations such as viscosity and elasticity. Smart worm-like micelles have attracted considerable interest due to their promising characteristics, and have many technological applications.27,29–31 Compared with other factors, such as pH, salinity, redox reagent, and temperature, light plays a vital and important role and has a set of significant advantages.32–34 Systems using light can be operated in a clean environment without any other additional chemicals, avoiding any changes in thermodynamic conditions. Besides, light is a reliable and cheap energy resource, and can be adjusted at an accurate distance, which is valuable in research fields such as nanoscience, nanotechnology, and medicine.35,36
Therefore, light has always been used as a stimulus by exploiting trans-to-cis isomerization in worm-like micelles.34,37–40 The investigation of the light-responsive worm-like micelles was first reported in 1980s.41,42 There are mainly two ways of obtaining light-responsive worm-like micelles: adding certain light-sensitive additives into surface active ionic liquid solutions or introducing functional groups into the molecules.27,43–45 Light-responsive worm-like micelles always take the advantage of some light-induced trans-to-cis isomerization of additives containing certain functional groups or surfactants.46,47 Obviously, these changes could affect the aggregation behavior of the molecules inside the aggregates; thus, the properties of worm-like micelles would alter as well.
In this study, we investigated the aggregation behavior of 2-methoxycinnamic acid (trans-OMCA) and 1-hexadecyl-3-methylimidazolium bromide (C16mimBr) in an aqueous solution, and the transition of micelles induced by UV irradiation was observed. Structures of C16mimBr and trans-OMCA are shown in Scheme 1. We just want to construct a photo-responsive system with the help of the surfactant and the photo-isomerized molecule. In the present study, the system is composed of C16mimBr and trans-OMCA, which contain aromatic rings in their molecules. The complex interactions, such as electrostatic, hydrophobic and π–π stacking interactions, exist in the system, which may result in the rich phase behaviors. This inspired us to carry out this investigation. We expect that this study will help to better understand the photo-responsive system and expand its application.
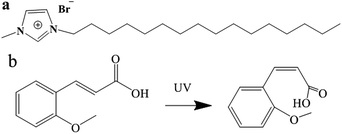 |
| Scheme 1 Chemical structure of C16mimBr (a) and OMCA (b). | |
2. Experimental section
2.1 Materials
The compounds 1-methylimidazole (99%), 1-bromohexadecane (97%), dichloromethane (99.5%), tetrahydrofuran (THF) (99%), and 2-methoxycinnamic acid (trans-OMCA) (98%) were purchased from Aladdin Chemical Reagent Co., Ltd (Shanghai, China). Ultrapure deionized water was obtained from Erie water purification systems. Solutions containing trans-OMCA were prepared with a slight excess of base (NaOH).
2.1.1 Synthesis of 1-hexadecyl-3-methylimidazolium bromide (C16mimBr).
C16mimBr was synthesized according to a previously reported study.48 The solution of 1-bromohexadecane in dichloromethane was added to 1-methylimidazole dissolved in dichloromethane. The mixture was stirred under a nitrogen atmosphere for 48 hours at 75–80 °C. Then, the product was cooled to room temperature and dichloromethane was evaporated. The product was recrystallized from THF at least three times and dried under vacuum for 48 hours at 50 °C. The product was characterized by 1H-NMR spectroscopy (400 MHz) using D2O as the solvent. The 1H-NMR result was recorded as follows:
C16mimBr δH: 9.03 (s, 1H), 7.37 (t, 2H), 4.00 (t, 2H), 3.72 (s, 3H), 1.68 (s, 2H), 1.13 (m, 26H), 0.61 (t, 3H).
2.2 Characterization
2.2.1 UV irradiation.
Samples were irradiated by a CHF-XM500-500W ultrahigh pressure short arc mercury lamp with a 365 nm filter for two hours. The experiment was carried out in a water bath at 25 °C with constant stirring.
2.2.2 UV-vis spectroscopy measurement.
The UV-vis spectroscopy measurements of the samples before and after UV irradiation were carried out on a UNIC-2802 UV-vis spectrophotometer at room temperature. For this measurement, water was used as the solution.
2.2.3 Rheology measurements.
An AR2000 rheometer (TA Instruments, New Castle, DE) was employed to investigate rheological properties of the samples. Samples were run on a cone and plate geometry (diameter, 40 mm; cone angle, 2°). Dynamic frequency sweep measurement was conducted in the linear viscoelastic region. The region was determined from the dynamic strain sweep measurement. The shearing rate ranged from 0.001 to 1000 s−1. The zero-shear viscosity (η0) was obtained from viscosity curve to the vertical coordinates. All the experiments were conducted at 25 °C.
2.2.4 Cryo-TEM.
5 µL of the solution was loaded onto a TEM carbon grid using a micropipette. TEM carbon grid was blotted with two pieces of filter paper to obtain a thin film suspended on the mesh hole. About 5 seconds later, samples were plunged into a reservoir of liquid ethane at −165 °C. The samples were stored in liquid nitrogen till they were transferred to a cryogenic sample holder (Gatan 626) and examined with a JEOL JEM-1400 TEM (120 kV) at −174 °C. The phase contrast was enhanced by under-focus. Images were obtained on a Gatan Multiscan CCD and processed with a Digital Micrograph. The cryo-TEM observations were performed at the China University of Petroleum (Eastern China).
2.2.5 Surface tension measurements.
Surface tension measurements were carried out using a model JYW-200B surface tensiometer (Chengde Dahua Testing Instrument Co., Ltd, Hebei, China). The temperature was maintained at 25 °C with the help of a thermostatic bath. Surface tension was measured through a ring method, and all the tests were repeated at least twice until the results were repeatable.
3. Results and discussion
3.1 Phase behavior of C16mimBr/trans-OMCA system
To investigate the phase behavior of C16mimBr/trans-OMCA system, different amounts of trans-OMCA were added into a series of C16mimBr solutions with a constant concentration. Fig. 1 shows the phase diagram of the C16mimBr/trans-OMCA system at 25 °C. Phase I and phase II are divided by a solid line; these regions have different aggregation behaviors. As shown in this figure, samples in phase I are clear and transparent with no viscoelasticity. In particular, when C16mimBr concentration is lower than 80 mM, the liquid is not viscoelastic even when the concentration of trans-OMCA is up to 100 mM. However, with the addition of trans-OMCA in certain C16mimBr aqueous solutions, changes can be observed, and the samples turn into highly viscoelastic solutions. As observed in the figure, the fluid in the bottle cannot flow even when the bottle is inverted. It is predicted that worm-like micelles are formed in this region, which will be proved in the following sections.
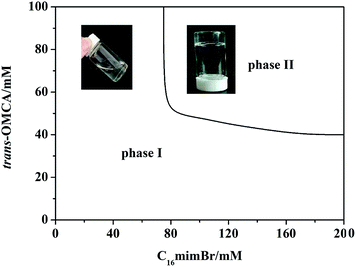 |
| Fig. 1 Phase diagram of C16mimBr and trans-OMCA system at 25 °C. | |
3.2 Rheological properties of trans-OMCA and C16mimBr system
3.2.1 Effect of trans-OMCA concentration on rheological properties.
To investigate the effect of trans-OMCA concentration on the micelle formation of C16mimBr/trans-OMCA system at 25 °C, samples were prepared by adding different amounts of trans-OMCA to C16mimBr solutions with a fixed concentration (150 mM). Fig. 2a shows the steady-shear rate viscosity curves of these systems. The viscosity of the system is very low and displays a Newtonian behavior49,50 under low trans-OMCA concentrations. However, changes appear when a certain amount of trans-OMCA is added. The fluid exhibits high viscosity at low shear rates, and the solution displays a Newtonian property. Nevertheless, viscosity decreases while the shear rate increases, and shear-thinning behavior is observed. High viscosity in the Newtonian plateau can be attributed to the presence of entangled networks of worm-like micelles, and perhaps this is an indication of long worm-like micelles, which will be studied in the following section. Many reports have shown that the entangled network is aligned as the shear rate increases.51 However, the fluid cannot reach an equilibrium condition above a critical shear rate, and viscosity falls at this instance.
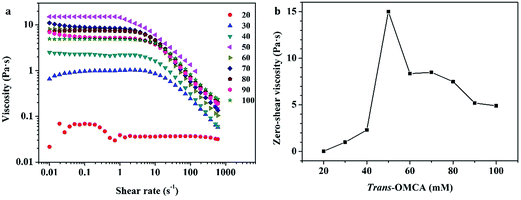 |
| Fig. 2 Viscosity measurement as a function of trans-OMCA concentration (20–100 mM) at a fixed C16mimBr solution (150 mM) at 25 °C: (a) viscosity versus shear rate curves; (b) zero-shear viscosity versus trans-OMCA concentration. | |
Zero-shear viscosity (η0) can be obtained according to the Carreau model,50 and it is the value of viscosity at the plateau in Fig. 2a. Fig. 2b presents the curve of η0 against trans-OMCA concentration for this system. In this figure, we can observe that η0 increases with trans-OMCA concentration and reaches a peak, and then decreases. This phenomenon is similar to the behavior of some ionic surfactants and aqueous systems of salts.52 The increase in η0 may be an indication of growth in micellar length. The addition of trans-OMCA can stimulate the electrostatic repulsions among the headgroups of the surfactant molecules. Consequently, the micellar length increases, micelles begin to overlap, and an entangled network is formed. It has been considered that the decrease in η0 is a result of branched worm-like micelles.53 The branched structures tend to slide along the micelles, and thus the viscosity will decrease.
Viscoelastic properties of the wormlike micellar samples were studied by frequency sweep measurement. The dynamic rheological behavior for the C16mimBr/trans-OMCA system was studied as a function of trans-OMCA concentration at a certain C16mimBr concentration, which is shown in Fig. 3a. The lines show the relationship of elastic modulus (G′) and viscous modulus (G″) versus oscillation frequency (ω). At low ω, G′ is smaller than G″, and the sample shows viscous behavior. Elastic behavior is presented at high ω, when G′ is higher than G″ and G′ shows a plateau value at that time. The samples show viscoelastic property, which indicates the formation of a rigid network of entangled worm-like micelles. A crossover point exists according to the lines; the crossover frequency is called ωco, and the crossover modulus corresponds to Gco. As shown in Fig. 5a, ωco moves backward and then forward as trans-OMCA concentration increases from 40 mM to 60 mM. A higher ωco value means poorer viscoelastic behavior. The values of G′ and G″ are fitted as eqn (1) and (2) below.54
| 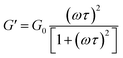 | (1) |
| 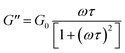 | (2) |
where
G0 stands for the plateau value of shear modulus and
τ stands for the stress relaxation time.
G0 and
τ can be calculated from
eqn (3) and
(4).
|  | (4) |
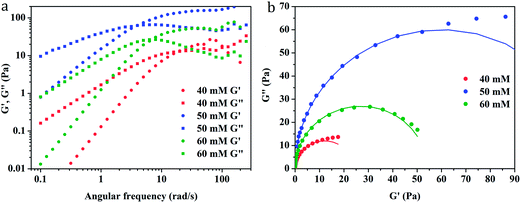 |
| Fig. 3 (a) Dynamic frequency sweep for different trans-OMCA concentration (40 mM, 50 mM, and 60 mM) in a 150 mM C16mimBr solution at 25 °C; (b) Cole–Cole plots of this system. | |
To better understand whether the Maxwell model fits the data, Cole–Cole plots are commonly investigated. The Cole–Cole plot is a curve of G″ as a function of G′. It can be obtained from eqn (5).
| 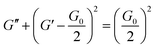 | (5) |
The Cole–Cole plots are shown in Fig. 3b. It can be clearly seen that at low frequency, all the experimental points follow the Maxwell fluid, which means that the solution behaves as an ideal Maxwell material within the lower shear frequency range. This is a proof that worm-like micelles are formed in this situation. However, when trans-OMCA concentration is lower than 30 mM, the system has no viscoelastic response.
3.2.2 Effect of surfactant concentration on rheological properties.
It can be seen clearly that the concentration of trans-OMCA has great influence on the viscosity of the C16mimBr/trans-OMCA system. The impact of surfactant (C16mimBr) concentration on this system was studied. The steady state curves for different surfactant concentration solutions are shown in Fig. 4a. It can be seen from the figure that samples are Newtonian fluids at low concentrations; the viscosities of these samples are low and are not affected by the shear rate. However, when C16mimBr concentration is higher than 80 mM, these samples are non-Newtonian fluids exhibiting a high constant viscosity at low shear rates and shear thinning behavior at high shear rates, which is a typical indication of worm-like micelles.55Fig. 4b shows the curve of η0 obtained from Fig. 4a. According to this figure, the value of η0 increases with the addition of C16mimBr. Low viscosity at low C16mimBr concentrations represents spherical or short rod-like micelles, and the addition of C16mimBr may favor the growth of micelles.56
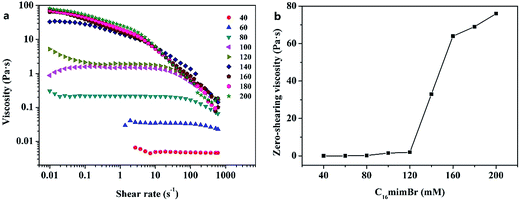 |
| Fig. 4 (a) Viscosity versus shear rate curves measurement as a function of C16mimBr (40–200 mM) concentration at a fixed trans-OMCA solution (50 mM) at 25 °C; (b) zero-shear viscosity versus trans-OMCA concentration. | |
3.3 Effect of UV-vis light irradiation on C16mimBr/trans-OMCA system
3.3.1 UV-vis absorption spectra of C16mimBr/trans-OMCA system subjected to UV irradiation.
Molecules of trans-OMCA and cis-OMCA exhibit different wavelength absorptions in the ultraviolet region; the structural transition can be detected by UV-vis spectra. In this way, the absorbance spectra of trans-OMCA before and after UV irradiation were obtained to prove the trans-to-cis transition in Fig. 5. Fig. 5a shows that, before irradiation, trans-OMCA has absorbance peaks at 269 and 312 nm. However, UV light irradiation results in blue shifts to 255 and 292 nm. Significant reduction in the intensity is also presented. According to the literature, cis-isomer has higher steric hindrance and lower conjugation degree, indicating that the maximum absorption wavelength and the value of intensity are lower than that of the trans-isomer.27 This blue shift is a proof of trans-to-cis transition. Besides, the UV irradiated sample was irradiated by visible light for 24 hours, and there was no change in the absorbance spectra, as shown by the black line in Fig. 5a. This phenomenon is in accordance with a previously published study.42
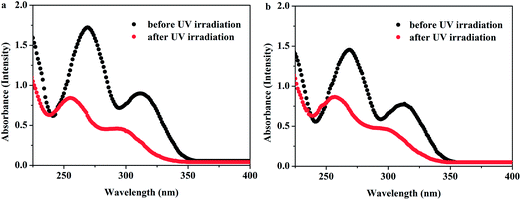 |
| Fig. 5 UV-vis spectra before and after UV irradiation for 2 hours of (a) trans-OMCA (50 mM) and (b) trans-OMCA (50 mM) + C16mimBr (50 mM). All the samples were diluted 100 times before investigation. | |
3.3.2 Rheological properties of C16mimBr/trans-OMCA system after UV irradiation.
Then, we studied the effect of UV light irradiation on the system. After being irradiated for 2 hours, the sample changed into a flowing fluid with low viscosity from the viscoelastic fluid. Fig. 6a shows the steady shear rheological curve of 150 mM C16mimBr and 50 mM trans-OMCA solution after UV irradiation. It can be found that the solution is a Newtonian fluid. The value of η0 decreases sharply compared with the value of 150 mM C16mimBr and 50 mM trans-OMCA solution before irradiation, as shown in Fig. 2a. This interesting phenomenon indicates the length of worm-like micelles decreases, and they even turn into spherical micelles. Fig. 6b shows the dynamic rheology of this sample. It can be clearly seen that G″ > G′ in all frequency regions without a crossover, and this is a characteristic of a viscous fluid. We can conclude that UV irradiation could convert the viscoelastic fluid to a thin, viscous one, and the length of wormlike micelles may decrease, which could be ascribed to the phase transition from worm-like micelles to rod-like or spherical micelles.
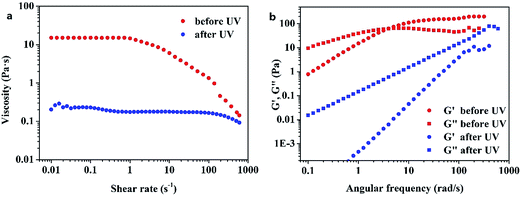 |
| Fig. 6 Typical rheology results of a solution containing 150 mM C16mimBr and 50 mM trans-OMCA after UV irradiation for 2 hours at 25 °C: (a) viscosity versus shear rate; (b) dynamic frequency sweep. | |
3.3.3 Cryo-TEM investigation on C16mimBr/trans-OMCA system.
Cryo-TEM technique was further employed to confirm the effect of UV irradiation for this system. Cryo-TEM images before and after 120 min of UV irradiation are shown in Fig. 7. As shown in Fig. 7a, a typical worm-like micelle network could be observed, and the network is formed by numerous worm-like micelles. After UV irradiation, as shown in Fig. 7b, worm-like micellar structures are damaged and cannot be detected anymore. The change in worm-like micelles structure could be attributed to the trans-to-cis isomerization induced by UV irradiation. This phenomenon is in accordance with the rheological results of the trans-OMCA (50 mM) + C16mimBr (150 mM) before and after UV irradiation.
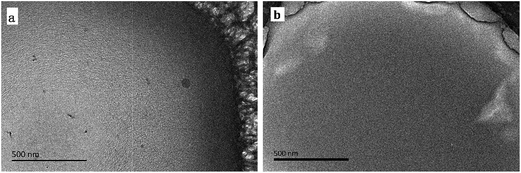 |
| Fig. 7 Cryo-TEM images of 150 mM C16mimBr + 50 mM trans-OMCA (a) before UV irradiation and (b) after UV irradiation. | |
3.3.4 Surface tension measurements.
Surface tension measurements were carried out to evaluate the aggregation behavior of the aqueous solutions. The aqueous solution of trans-OMCA was irradiated by UV light for 120 min before the experiment, and it turned into the cis form. Fig. 8 shows the surface tensions (γ) of the aqueous solutions of C16mimBr, C16mimBr/trans-OMCA and C16mimBr/cis-OMCA. All the experiments were carried out at 25 °C. It can be suggested that surface tensions of all the three solutions decrease with the addition of surfactant before reaching a plateau at certain C16mimBr concentrations. The critical aggregation concentrations (cac) obtained from this figure are listed in Table 1. The C16mimBr/trans-OMCA solution and C16mimBr/cis-OMCA solution have lower cac values. Both trans-OMCA and cis-OMCA molecules have opposite charges against surfactant headgroups. They can compress the electric double layer surrounding the micelles and finally reduce the electrostatic repulsion effect among the headgroups. Finally, cac values decrease. The effectiveness of surface tension reduction (Πcac)5 can be calculated from the following equation:where γ0 is the surface tension of pure solvent and γcac is the surface tension of the sample at the cac. Πcac shows the equilibrium surface tension reduction caused by the introduction of these molecules. The values in Table 1 shows that Πcac increases in the order C16mimBr < cis-C16mimBr/trans-OMCA < C16mimBr/trans-OMCA.
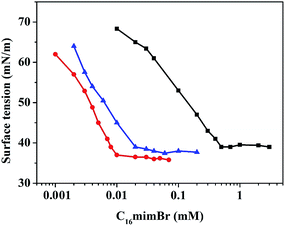 |
| Fig. 8 Surface tension (γ) of C16mimBr aqueous solution, C16mimBr/trans-OMCA (50 mM) aqueous solution and the C16mimBr/cis-OMCA (50 mM) aqueous solution at 25 °C. | |
Table 1 Surface properties of C16mimBr, C16mimBr/trans-OMCA and C16mimBr/cis-OMCA systems at 25 °C (trans-OMCA and cis-OMCA concentrations are 50 mM)
|
cac (mM) |
Γ
cac (mN m−1)s |
Π
cmc (mN m−1) |
Γ
max (µmol m−2) |
a
s (Å2) |
P
|
C16mimBr |
0.566 |
38.98 |
33.05 |
2.06 |
80.7 |
0.261 |
C16mimBr/trans-OMCA |
0.010 |
37.37 |
34.63 |
3.31 |
50.2 |
0.420 |
C16mimBr/cis-OMCA |
0.019 |
38.60 |
33.39 |
2.99 |
55.6 |
0.379 |
The maximum excess surface tension (Γmax) and the minimum area occupied per surfactant molecule (as) are introduced to clearly describe the aggregation behavior in an aqueous solution.5 They are obtained from the Gibbs adsorption isotherm:
| 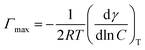 | (7) |
| 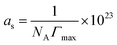 | (8) |
where
R is the gas constant (8.314 J mol
−1 K
−1),
T is the absolute temperature,
NA is Avogadro's number (6.022 × 10
23 mol
−1). As indicated in
Table 1, the C
16mimBr/
trans-OMCA system obtains the highest
Γmax value and the lowest
as value. The C
16mimBr headgroups can adsorb the anionic counterion in
trans-OMCA, which makes the surfactant molecules pack more closely. However,
cis-OMCA is more hydrophilic than
trans-OMCA and fewer molecules are absorbed.
27 This is the reason why the C
16mimBr/
cis-OMCA system is different from the C
16mimBr/
trans-OMCA system.
3.4 Mechanism of micelle transition induced by UV irradiation
The mechanism of micelle transition can be explained by the theory of molecular packing parameter.57 |  | (9) |
where υ0 is the hydrocarbon core volume of a surfactant, as is the equilibrium area per molecule at the surfactant–water interface, and l0 is the length of hydrocarbon chain. The value of P means the critical packing parameter, which can be used to determine the shape of micelles. The critical condition for the formation of different micelles are P = 1/3, P = 1/2, and P = 1, respectively. When P < 1/3, spherical micelles can be observed; when 1/3 < P < 1/2, rod-like or worm-like micelles are obtained; when 1/2 < P < 1, vesicles exists; when P ≈ 1, planar bilayers are observed; and when P > 1, inverted micelles are obtained.
In our system, the value of υ0 and l0 can be calculated by the experimental formula introduced by Tanford.58
where
Nc is the carbon atom number of the alkyl chain.
According to formulas (10) and (11), the value of υ0 and l0 for C16mimBr are 457.8 Å3 and 21.7 Å, respectively. The value of as has been calculated and shown in Table 1. As a result, the critical packing parameter can be calculated, and the value is 0.261. It is apparent that spherical micelles can be observed in the aqueous solution of C16mimBr and no worm-like micelles would appear theoretically, which is in correspondence with the experimental results. We can calculate the value of P for the other two systems, and the results are shown in Table 1. They are 0.420 and 0.379, respectively, which are in the range of 1/3–1/2 where rod-like micelles and worm-like micelles can be detected. Once introduced, the compensation ion with opposite charge in trans-OMCA tends to insert into the spherical micelles, and the surface charges can be shielded because of electrostatic repulsion. This will lead to the reduction of as, and the value of P increases at the same time. As a result, worm-like micelles appear with the addition of a certain amount of trans-OMCA. After UV irradiation, trans-OMCA would transform to cis-OMCA. As reported before, cis-OMCA is more hydrophobic than trans-OMCA. The different hydrophobicity is attributed to the net dipole moment. The dipoles tend to add up in the cis-OMCA; however, they tend to cancel each other in the trans-OMCA.27 Less cis-OMCA could combine with C16mimBr because of the hydrophobic interaction and steric hindrance. Thus, the value of as increases and P decreases, and therefore spherical or rod-like micelles will appear after UV irradiation. This process can be clearly seen in Fig. 9.
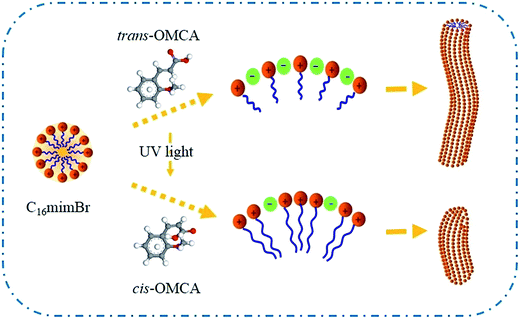 |
| Fig. 9 Mechanism of the aggregation behavior for the photo-responsive system. | |
4. Conclusions
In this study, we have investigated the rheological behavior and UV light influence of the C16mimBr/trans-OMCA system in an aqueous solution. Rheological measurements were used to observe the influence of trans-OMCA and C16mimBr concentrations. UV-vis absorption spectroscopy, rheological measurement and cryo-TEM observations were carried out to investigate the influence of UV irradiation on the structural change of trans-OMCA and the micelle transition from worm-like micelles to spherical or rod-like micelles. Surface tension measurements were employed to investigate the effect of trans-OMCA and UV light irradiation on the micelle formation process. Critical packing parameter theory was used to discover the mechanism of micelle transition.
Acknowledgements
The study was supported by the National Science Fund for Distinguished Young Scholars (51425406), National Natural Science Foundation of China (51174221).
References
- Y. Qiao, Y. Lin, Y. Wang, Z. Li and J. Huang, Metal-driven viscoelastic wormlike micelle in anionic/zwitterionic surfactant systems and template-directed synthesis of dendritic silver nanostructures, Langmuir, 2011, 27, 1718–1723 CrossRef PubMed.
- D. S. Yakovlev and E. S. Boek, Molecular dynamics simulations of mixed cationic/anionic wormlike micelles, Langmuir, 2007, 23, 6588–6597 CrossRef PubMed.
- C. Dai, M. Du, M. Zhao, Q. You, B. Guan, X. Wang and P. Liu, Study of Micelle Formation by Fluorocarbon Surfactant N-(2-hydroxypropyl) perfluorooctane Amide in Aqueous Solution, J. Phys. Chem. B, 2013, 117, 9922–9928 CrossRef CAS.
- H. Hoffmann, Fascinating phenomena in surfactant chemistry, Adv. Mater., 1994, 6, 116–129 CrossRef CAS PubMed.
- C. Dai, M. Du, Y. Liu, S. Wang, J. Zhao, A. Chen, D. Peng and M. Zhao, Aggregation Behavior of Long-Chain Piperidinium Ionic Liquids in Ethylammonium Nitrate, Molecules, 2014, 19, 20157–20169 CrossRef PubMed.
- H. Afifi, G. r. Karlsson, R. K. Heenan and C. A. Dreiss, Solubilization of oils or addition of monoglycerides drives the formation of wormlike micelles with an elliptical cross-section in cholesterol-based surfactants: A study by rheology, SANS, and cryo-TEM, Langmuir, 2011, 27, 7480–7492 CrossRef CAS PubMed.
- S. R. Raghavan and E. W. Kaler, Highly viscoelastic wormlike micellar solutions formed by cationic surfactants with long unsaturated tails, Langmuir, 2001, 17, 300–306 CrossRef CAS.
- R. Zana and Y. Talmon, Dependence of aggregate morphology on structure of dimeric surfactants, Nature, 1993, 362, 228–230 CrossRef CAS PubMed.
- Y. Han, Y. Feng, H. Sun, Z. Li, Y. Han and H. Wang, Wormlike micelles formed by sodium erucate in the presence of a tetraalkylammonium hydrotrope, J. Phys. Chem. B, 2011, 115, 6893–6902 CrossRef CAS PubMed.
- Y.-Y. Won, H. T. Davis and F. S. Bates, Giant wormlike rubber micelles, Science, 1999, 283, 960–963 CrossRef CAS.
- M. Cates, Reptation of living polymers: dynamics of entangled polymers in the presence of reversible chain-scission reactions, Macromolecules, 1987, 20, 2289–2296 CrossRef CAS.
- H. Rehage and H. Hoffmann, Viscoelastic surfactant solutions: model systems for rheological research, Mol. Phys., 1991, 74, 933–973 CrossRef CAS PubMed.
- Y. Qi and J. L. Zakin, Chemical and rheological characterization of drag-reducing cationic surfactant systems, Ind. Eng. Chem. Res., 2002, 41, 6326–6336 CrossRef CAS.
- M. R. Stukan, E. S. Boek, J. T. Padding, W. J. Briels and J. P. Crawshaw, Flow of wormlike micelles in an expansion-contraction geometry, Soft Matter, 2008, 4, 870–879 RSC.
- S. Ezrahi, E. Tuval and A. Aserin, Properties, main applications and perspectives of worm micelles, Adv. Colloid Interface Sci., 2006, 128, 77–102 CrossRef PubMed.
- J.-M. Lehn, Toward self-organization and complex matter r, Science, 2002, 295, 2400–2403 CrossRef CAS PubMed.
- J. H. Fuhrhop and W. Helfrich, Fluid and solid fibers made of lipid molecular bilayers, Chem. Rev., 1993, 93, 1565–1582 CrossRef CAS.
- S. Svenson, Controlling surfactant self-assembly, Curr. Opin. Colloid Interface Sci., 2004, 9, 201–212 CrossRef.
- M. Johnsson, A. Wagenaar and J. B. Engberts, Sugar-based gemini surfactant with a vesicle-to-micelle transition at acidic pH and a reversible vesicle flocculation near neutral pH, J. Am. Chem. Soc., 2003, 125, 757–760 CrossRef CAS PubMed.
- H. Maeda, A. Yamamoto, M. Souda, H. Kawasaki, K. S. Hossain, N. Nemoto and M. Almgren, Effects of protonation on the viscoelastic properties of tetradecyldimethylamine oxide micelles, J. Phys. Chem. B, 2001, 105, 5411–5418 CrossRef CAS.
- F. P. Hubbard Jr and N. L. Abbott, A small angle neutron scattering study of the thicknesses of vesicle bilayers formed from mixtures of alkyl sulfates and cationic bolaform surfactants, Soft Matter, 2008, 4, 2225–2231 RSC.
- F. P. Hubbard, G. Santonicola, E. W. Kaler and N. L. Abbott, Small-angle neutron scattering from mixtures of sodium dodecyl sulfate and a cationic, bolaform surfactant containing azobenzene, Langmuir, 2005, 21, 6131–6136 CrossRef CAS PubMed.
- P. R. Majhi and A. Blume, Temperature-induced micelle-vesicle transitions in DMPC-SDS and DMPC-DTAB mixtures studied by calorimetry and dynamic light scattering, J. Phys. Chem. B, 2002, 106, 10753–10763 CrossRef CAS.
- S. Ghosh, K. Irvin and S. Thayumanavan, Tunable disassembly of micelles using a redox trigger, Langmuir, 2007, 23, 7916–7919 CrossRef CAS PubMed.
- Y. Yan, W. Xiong, X. Li, T. Lu, J. Huang, Z. Li and H. Fu, Molecular packing parameter in bolaamphiphile solutions: adjustment of aggregate morphology by modifying the solution conditions, J. Phys. Chem. B, 2007, 111, 2225–2230 CrossRef CAS PubMed.
- Z. Chu and Y. Feng, Thermo-switchable surfactant gel, Chem. Commun., 2011, 47, 7191–7193 RSC.
- A. M. Ketner, R. Kumar, T. S. Davies, P. W. Elder and S. R. Raghavan, A simple class of photorheological fluids: surfactant solutions with viscosity tunable by light, J. Am. Chem. Soc., 2007, 129, 1553–1559 CrossRef CAS PubMed.
- M. A. C. Stuart, W. T. Huck, J. Genzer, M. Müller, C. Ober, M. Stamm, G. B. Sukhorukov, I. Szleifer, V. V. Tsukruk and M. Urban, Emerging applications of stimuli-responsive polymer materials, Nat. Mater., 2010, 9, 101–113 CrossRef PubMed.
- K. Bohon and S. Krause, An electrorheological fluid and siloxane gel based electromechanical actuator: working toward an artificial muscle, J. Polym. Sci., Part B: Polym. Phys., 1998, 36, 1091–1094 CrossRef CAS.
- E. Boek, A. Jusufi, H. Löwen and G. Maitland, Molecular design of responsive fluids: molecular dynamics studies of viscoelastic surfactant solutions, J. Phys.: Condens. Matter, 2002, 14, 9413 CrossRef CAS.
-
M. Morvan, G. Degre, J. Beaumont, A. Colin, G. Dupuis, A. Zaitoun and H. H. Al-Sharji, Viscosifying surfactant technology for heavy oil reservoirs, in SPE Heavy Oil Conference Canada, SPE, January, 2012 Search PubMed.
- Y. Lin, X. Cheng, Y. Qiao, C. Yu, Z. Li, Y. Yan and J. Huang, Creation of photo-modulated multi-state and multi-scale molecular assemblies via binary-state molecular switch, Soft Matter, 2010, 6, 902–908 RSC.
- D. Wang, R. Dong, P. Long and J. Hao, Photo-induced phase transition from multilamellar vesicles to wormlike micelles, Soft Matter, 2011, 7, 10713–10719 RSC.
- J. Eastoe and A. Vesperinas, Self-assembly of light-sensitive surfactants, Soft Matter, 2005, 1, 338–347 RSC.
- P. H. Rasmussen, P. Ramanujam, S. Hvilsted and R. H. Berg, A remarkably efficient azobenzene peptide for holographic information storage, J. Am. Chem. Soc., 1999, 121, 4738–4743 CrossRef CAS.
- T. Ikeda and O. Tsutsumi, Optical switching and image storage by means of azobenzene liquid-crystal films, Science, 1995, 268, 1873–1875 CAS.
- X. Pei, J. Zhao and X. Wei, Wormlike micelles formed by mixed cationic and anionic gemini surfactants in aqueous solution, J. Colloid Interface Sci., 2011, 356, 176–181 CrossRef CAS PubMed.
- P. Baglioni, E. Braccalenti, E. Carretti, R. Germani, L. Goracci, G. Savelli and M. Tiecco, Surfactant-based photorheological fluids: Effect of the surfactant structure, Langmuir, 2009, 25, 5467–5475 CrossRef CAS PubMed.
- H.-Y. Lee, K. K. Diehn, S. Kunshan, C. Tianhong and S. R. Raghavan, Reversible Photorheological Fluids Based on Spiropyran-Doped Reverse Micelles, J. Am. Chem. Soc., 2011, 133, 8461–8463 CrossRef CAS PubMed.
- S. Dupuy, L. Crawford, M. Bühl and S. P. Nolan, The Gold(I)-Catalysed Protodecarboxylation Mechanism, Chem.–Eur. J., 2015, 21, 3399–3408 CrossRef CAS PubMed.
- N. Müller, T. Wolff and G. von Bünau, Light-induced viscosity changes of aqueous solutions containing 9-substituted anthracenes solubilized in cetyltrimethylammonium micelles, J. Photochem., 1984, 24, 37–43 CrossRef.
- Z. Chu, C. A. Dreiss and Y. Feng, Smart wormlike micelles, Chem. Soc. Rev., 2013, 42, 7174–7203 RSC.
- H. Oh, V. Javvaji, N. A. Yaraghi, L. Abezgauz, D. Danino and S. R. Raghavan, Light-induced transformation of vesicles to micelles and vesicle-gels to sols, Soft Matter, 2013, 9, 11576–11584 RSC.
- M. Akamatsu, P. A. FitzGerald, M. Shiina, T. Misono, K. Tsuchiya, K. Sakai, M. Abe, G. G. Warr and H. Sakai, Micelle Structure in a Photoresponsive Surfactant with and without Solubilized Ethylbenzene from Small-Angle Neutron Scattering, J. Phys. Chem. B, 2015, 119, 5904–5910 CrossRef CAS PubMed.
- H. Oh, A. M. Ketner, R. Heymann, E. Kesselman, D. Danino, D. E. Falvey and S. R. Raghavan, A simple route to fluids with photo-switchable viscosities based on a reversible transition between vesicles and wormlike micelles, Soft Matter, 2013, 20, 5025–5033 RSC.
- Y. Bi, H. Wei, Q. Hu, W. Xu, Y. Gong and L. Yu, Wormlike Micelles with Photoresponsive Viscoelastic Behavior Formed by Surface Active Ionic Liquid/Azobenzene Derivative Mixed Solution, Langmuir, 2015, 31, 3789–3798 CrossRef CAS PubMed.
- H. Yan, Y. Long, K. Song, C. H. Tung and L. Zheng, Photo-induced transformation from wormlike to spherical micelles based on pyrrolidinium ionic liquids, Soft Matter, 2014, 10, 115–121 RSC.
- J. Dupont, C. S. Consorti, P. A. Suarez and R. F. de Souza, Preparation of 1‐Butyl‐3‐Methyl Imidazolium‐Based Room Temperature Ionic Liquids, Org. Synth., 2003, 236 Search PubMed.
- X. Wang, R. Wang, Y. Zheng, L. Sun, L. Yu, J. Jiao and R. Wang, Interaction between zwitterionic surface activity ionic liquid and anionic surfactant: Na+-driven wormlike micelles, J. Phys. Chem. B, 2013, 117, 1886–1895 CrossRef CAS PubMed.
- R. G. Shrestha, L. K. Shrestha and K. Aramaki, Formation of wormlike micelle in a mixed amino-acid based anionic surfactant and cationic surfactant systems, J. Colloid Interface Sci., 2007, 311, 276–284 CrossRef CAS PubMed.
- F. Bautista, J. F. A. Soltero, E. R. Macías, J. E. Puig and O. Manero, Irreversible Thermodynamics Approach and Modeling of Shear-Banding Flow of Wormlike Micelles, J. Phys. Chem. B, 2002, 106, 13018–13026 CrossRef CAS.
- A. Khatory, F. Lequeux, F. Kern and S. Candau, Linear and nonlinear viscoelasticity of semidilute solutions of wormlike micelles at high salt content, Langmuir, 1993, 9, 1456–1464 CrossRef CAS.
- P. A. Hassan, S. J. Candau, F. Kern and C. Manohar, Rheology of wormlike micelles with varying hydrophobicity of the counterion, Langmuir, 1998, 14, 6025–6029 CrossRef CAS.
- C. A. Dreiss, Wormlike micelles: where do we stand? Recent developments, linear rheology and scattering techniques, Soft Matter, 2007, 3, 956–970 RSC.
- H. Rehage and H. Hoffmann, Rheological properties of viscoelastic surfactant systems, J. Phys. Chem. B, 1988, 92, 4712–4719 CrossRef CAS.
- V. Aswal, P. Goyal and P. Thiyagarajan, Small-angle neutron-scattering and viscosity studies of CTAB/NaSal viscoelastic micellar solutions, J. Phys. Chem. B, 1998, 102, 2469–2473 CrossRef CAS.
- J. N. Israelachvili, D. J. Mitchell and B. W. Ninham, Theory of self-assembly of hydrocarbon amphiphiles into micelles and bilayers, J. Chem. Soc., 1976, 72, 1525–1568 Search PubMed.
- C. Tanford, Micelle shape and size, J. Phys. Chem. B, 1972, 76, 3020–3024 CrossRef CAS.
|
This journal is © The Royal Society of Chemistry 2015 |