DOI:
10.1039/C5RA07854G
(Paper)
RSC Adv., 2015,
5, 58246-58254
Hydrotelluration of acetylenic esters: structural characterization of stereoisomers of methyl/ethyl β-(aryltelluro)acrylates†
Received
29th April 2015
, Accepted 23rd June 2015
First published on 23rd June 2015
Abstract
Synthesis and complete characterization of some ester functionalized vinylic tellurides bearing an aryl ligand with varying steric and electronic effects bound to tellurium is described. Hydrotelluration of methyl propiolate using Ar2Te2/NaBH4 in methanol results in a mixture of stereoisomers of methyl β-(aryltelluro)acrylates, ArTeCH
CHCOOMe (Ar = 4-MeOC6H4, 1A; 1-C10H7, 2A; 2,4,6-Me3C6H2, 3A; C5H5FeC5H4, 4A; 4-Me2NC6H4, 5A; and 2-C4H3S, 6A). The same reaction in ethanol provides isomeric mixtures of the ethyl esters ArTeCH
CHCOOEt (1B–6B). However, in the reactions between methyl propiolate and Ar2Te2 (Ar = 2,4,6-Me3C6H2, 4-Me2NC6H4) in isopropanol or t-butanol, no exchange of alkyl groups between the parent ester and the solvent is observed, instead detelluration of the Ar2Te2 to Ar2Te is a competing reaction along with almost exclusive formation of the (Z)-isomers (3Aa, 5Aa). The geometry of the separated stereoisomers is established in solution, with the help of 1H, 13C and 125Te NMR spectrometry. Of particular interest is the observation that 125Te chemical shifts {deshielded in (Z) compared to (E); Δδ = 106–136 ppm} and the geminal heteronuclear coupling constants {2J(1H–125Te) values for (E) are more than seven times that of the corresponding (Z) isomer} can be used to distinguish between liquid isomers. Structural characterization in the solid state by single-crystal X-ray diffraction for the 2Ba, 3Aa, 3Ba, 5Aa, 8 (Z)-isomers as well as for both stereoisomers of 4-Me2NC6H4TeCH
CHCOOEt (5Ba and 5Bb) is also presented. The carbonyl O atom of the ester group is invariably involved, at least in the solid state, in a secondary bonding interaction with the Te(II) atom. While an intermolecular Te⋯O interaction gives rise to one-dimensional supramolecular arrays in the crystal lattice of 5Bb with (E) configuration, it is realized intramolecularly in the case of the (Z)-isomers due to the cis position of the chalcogen atoms.
Introduction
The hydrotelluration of alkynes under reducing (Bu2Te2/NaBH4/EtOH) or non reducing (Te0/BuLi/THF) conditions is highly regio- and stereoselective and is a commonly practiced method of preparing (Z)-vinylic tellurides. Compounds of this class have proved to be useful synthetic intermediates in transition metal catalyzed cross-coupling reactions and as precursors in the preparation of highly reactive vinyllithiums, vinylcuprates or vinylzinc chlorides with retention of double bond geometry via transmetallation.1,2 In the hydrotelluration process, the aryl/alkyltellurium radical, RTe˙ and the hydrogen (from the alcohol used as reaction solvent) add to the alkynes and depending upon the nature of the substituent on the acetylene or the hydrotelluration reagent, the products often consist of mixtures of regio/stereoisomers. Thus, among terminal alkynes, while the aryl acetylenes are suitable substrates for the preparation of (Z)-vinylic tellurides, alkyl-substituted alkynes suffer from poor regioselectivity and give rise to a mixture of regioisomers, though the 1,2-addition product predominates.3 Hydrotelluration of propargylic alcohols and amines affords synthetically important allylic derivatives. In the case of the former, though the regioselectivity of the reaction is reported to favour the 1,2-addition, both the regioisomers have been characterized recently by NMR spectroscopy.4 On the other hand, hydrotelluration of tertiary propargyl amines was found to be highly regio- and stereospecific to afford (Z)-β-(organotelluro)allylic amines, but that of primary and secondary propargyl amines suffers from considerable loss of regiochemical control.5 While carbonyl functionalized alkynes were among the first to be studied under hydrotelluration reactions,6 there are only a few reports on the hydrotelluration of propargyl aldehydes7 and esters of propiolic acid8–11 as a synthetic route to obtain β-tellurovinylcarbonyl compounds with well defined geometry. Instead, β-organyltellurovinyl aldehydes and ketones have been prepared by employing β-chlorovinylaldehydes/ketones or β-acylvinyltriethylammonium chlorides as precursors.12 125Te chemical shifts (unlike δ 1H values) and 1H–125Te coupling constants have scarcely been used to characterize the stereoisomers in the product of a hydrotelluration reaction of an alkyne. Also, among the known β-substituted vinylorganyltellurides which are mostly liquids or low-melting solids, structural characterization of only a few of these in solid state is limited to either the (Z)12–15 or (E)16–19 isomers and to the best of our knowledge is not reported for both the stereoisomers of a given vinylorganyltelluride.
Keeping with our interest in the synthesis of functionalized organotelluriums and in continuation to our studies on chlorotelluration of 1-alkynes using bulky substituents on Te and the terminal acetylenes,15 we report herein hydrotelluration of methyl propiolate under reducing conditions (Ar2Te2/NaBH4/ROH; Ar = 4-MeOC6H4, 1-C10H7, 2,4,6-Me3C6H2, (C5H5)FeC5H4, 4-Me2NC6H4 and 2-C4H3S). Our aim was twofold, (i) to examine how the reaction solvent and the nature of the aryl ligand bound to tellurium affect the stereospecificity of acetylenic ester hydrotelluration and (ii) study the stereochemistry of the products in the solid state. Reactions of phenylacetylene are also included for comparison.
Results and discussion
Methyl propiolate, when subjected to hydrotelluration under reducing conditions in methanol afforded methyl β-(aryltelluro)acrylates, ArTeCH
CHCOOMe (Ar = 4-MeOC6H4, 1A; 1-C10H7, 2A; 2,4,6-Me3C6H2, 3A; (C5H5)FeC5H4, 4A; 4-Me2NC6H4, 5A; 2-C4H3S, 6A) (Scheme 1).
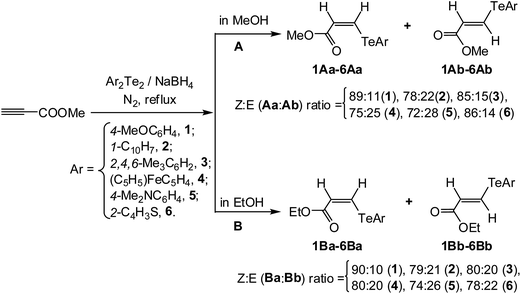 |
| Scheme 1 Hydrotelluration of methyl propiolate and Z : E ratio in the products. | |
The 1H NMR spectra recorded for the crude products showed the formation of both the (Z) and (E) stereoisomers in each case, but signals owing to the corresponding regioisomers were absent. The Z
:
E isomeric ratio (determined from the intensity of the 1H NMR doublet due to the proton attached to the β-carbon) varied between 89
:
11 (1Aa
:
1Ab) and 72
:
28 (5Aa
:
5Ab). The same reaction was repeated in ethanol, iso-propanol and tertiary butanol in place of methanol. Interestingly, the reaction in ethanol provided the aryltellurated ethyl ester analogues, 1Ba–6Ba and 1Bb–6Bb in comparable yields. In the hydrotelluration reaction of methyl propiolate using dimesitylditelluride, or bis(4-(N,N-dimethylamino)phenyl)ditelluride in iso-propanol or tertiary butanol, detelluration of the ditelluride to the corresponding telluride, Ar2Te (Ar = 3, 5) was a competing reaction along with the dominant formation of the (Z) isomers, 3Aa or 5Aa. The role of a Te moiety in the Me/Et exchange of the ester functionality appears to be little as the signal due to the methoxy protons of methyl propiolate (δ 3.80 ppm) was absent in the 1H NMR spectrum of the isolated crude product of heating methyl propiolate with NaBH4/EtOH in absence of a diarylditelluride. Hydrotelluration of phenylacetylene under the similar conditions, using the ditellurides with sterically demanding aryl ligands (2 and 3) gave exclusively the (Z)-isomers of ArTeCH
CHPh (Ar = 1-naphthyl, 7; mesityl, 8) in excellent yield. Compound 8 has been reported earlier20 but lacks complete characterization, while the (E) isomer of 7 has been isolated as an oil in the reaction between (E)-β-(phenylvinyl)phenyliodonium tetrafluoroborate and (1-C10H7)2Te2/NaBH4 in ethanol.21 Chromatographic separation of the stereoiosomers has been carried out on a silica column and complete structural characterization of each isomer was made by IR and NMR (1H, 13C and 125Te) spectroscopy and in the case of 2Ba, 3Aa, 3Ba, 5Aa, 5Ba, 5Bb and 8 by single-crystal X-ray diffraction techniques.
1H NMR chemical shifts of the signals due to vinylic protons and their 3J(1H–1H) coupling constant are diagnostic of the regio- and stereochemistry of the isolated new β-(aryltelluro)acrylates. The vinylic proton attached to the tellurated β-carbon is appreciably deshielded {δ ranges between 8.59 (5Ab) and 8.18 (3Ba)} compared to the other attached to the ester functionalized vinylic carbon {δ ranges between 7.02 (3Aa) and 5.75 (3Ab)}. The magnitude of 3J(1H–1H) parameter for (E) isomers (lying in the range 12.0–16.5 Hz) is significantly higher compared to that of the corresponding (Z) isomers (lying in the range 8.0–9.6 Hz). The 1H–125Te magnetic couplings in the cases where measured, reveal an interesting trend. While the vicinal coupling constants are independent of the stereochemistry and have usual values {3J(1H–125Te) ≈ 12 Hz}, the geminal coupling constants are unusual and differ markedly in a pair of stereoisomers. A strong two-bond heteronuclear magnetic interaction may be inferred among the aryltellurated acrylates with E-geometry {2J(1H–125Te) = 74–91 Hz}, while it is very weak for their (Z) counterparts {2J(1H–125Te) ≤ 11 Hz}, even weaker than the three-bond heteronuclear coupling. This indicates significant intramolecular C
O⋯Te interaction for the Z-isomer in solution, effectively holding the C–Te–C
C fragment in the s-trans configuration, orientating the tellurium lone pair away from the geminal proton and resulting in a very low 2J(1H–125Te) coupling constant compared to the E-isomer.22 Considerable difference in the δ(125Te) values in an isomeric pair is also observed, with significant deshielding of Te(II) nucleus in the (Z) isomer compared to that of (E) isomer (E/Z Δδ = 106–136 ppm), suggesting the carbonyl effects can be effectively transmitted in conjugative sense in the Z-geometry via an intramolecular ring possible due to a Te⋯O secondary bonding interaction. The 125Te chemical shifts for 7 and 8 are comparable with other (Z) isomers reported herein suggesting similar influence, of the phenyl and alkoxycarbonyl β-substituents if any, on the shielding of the tellurium nucleus.
The CO stretching frequency of methyl acrylate (1732 cm−1) is significantly lowered upon telluration, probably owing to the mass effect as well as due to Te←O
C coordinative interaction. This absorption appearing in the region 1718–1687 cm−1 in the infrared spectrum of (E)-isomers is further shifted to a lower frequency in the corresponding (Z)-isomers (1680–1672 cm−1). A comparison with the solution spectra, recorded for both the stereo isomers of methyl/ethyl β-(4-N,N-dimethylaminophenyltelluro)acrylates (5Aa and 5Ab/5Ba and 5Bb) reveals appreciable shifting of ν(CO) absorption to higher frequency in solution among the (E)-isomers [ν(CO), solution(solid): 5Ab, 1703(1687); 5Bb, 1713(1688) cm−1], but little change in it in case of the (Z)-isomers [ν(CO), solution(solid): 5Aa, 1678(1680); 5Ba, 1674(1670) cm−1]. Thus, while intramolecular coordinative interaction present in the solid state of the (Z)-isomers due to the cis position of the two chalcogen atoms is retained in solution as well, the weaker intermolecular Te←O interaction in the solid state among (E)-isomers appears to vanish in solution resulting in the observed enhancement in ν(CO). These conclusions are further substantiated from interatomic distances obtained from the single-crystal diffraction data presented herein.
Crystal structures
Single crystals of compounds, 2Ba, 3Aa, 3Ba, 5Aa, 5Ba, 5Bb and 8, were obtained by slow evaporation of a hexane or hexane/ethyl acetate solvent mixture at ambient temperature. The asymmetric unit in the crystal lattice of each of these compounds consists of a single molecule, other than 3Ba where there are two independent molecules in it. The relevant bond parameters are listed in Table 1 and the crystal data are given in Table 2. ORTEP diagrams of both stereo isomers of ethyl β-(4-N,N-dimethylaminophenyltelluro)acrylate (5Ba and 5Bb) are shown in Fig. 1 while those of 2Ba, 3Aa, 3Ba, 5Aa and 8 presented as Fig. S1–S5 are part of the ESI.†
Table 1 Relevant bond parameters (Å, °) in aryl(β-substituted vinyl)tellurides
Parameter |
2Ba |
3Aa |
3Ba |
5Aa |
5Ba |
5Bb |
8 |
Te–C1 |
2.075(3) |
2.075(2) |
2.085(4), 2.076(4) |
2.081(4) |
2.073(2) |
2.078(3) |
2.094(3) |
Te–Cx, x = 5, 6 or 9 |
2.140(3) |
2.135(2) |
2.141(4), 2.138(4) |
2.114(4) |
2.114(2) |
2.108(3) |
2.135(4) |
C1–C2 |
1.337(5) |
1.331(2) |
1.334(6), 1.341(6) |
1.335(5) |
1.338(3) |
1.324(3) |
1.340(5) |
Te⋯O1 |
2.849(3) |
2.883(1) |
2.842(3), 2.842(3) |
2.970(2) |
2.848(2) |
— |
— |
C1–Te–Cx, x = 5, 6 or 9 |
90.9(1) |
91.4(2) |
92.0(2), 92.5(1) |
90.6(1) |
95.6(1) |
93.3(1) |
93.9(1) |
Cx–Te⋯O1, x = 5 or 6 |
158.6(1) |
158.8(1) |
159.8(1), 159.2(1) |
156.6(1) |
163.6(2) |
— |
— |
Table 2 Crystallographic data and structure refinement parameters of aryl(β-substituted vinyl)tellurides
|
2Ba |
3Aa |
3Ba |
5Aa |
5Ba |
5Bb |
8 |
Chemical formula |
C15H14O2Te |
C13H16O2Te |
C14H18O2Te |
C12H15NO2Te |
C13H17NO2Te |
C13H17NO2Te |
C17H18Te |
Formula weight |
353.86 |
331.86 |
345.88 |
332.85 |
346.87 |
346.88 |
349.91 |
Crystal system |
Orthorhombic |
Monoclinic |
Triclinic |
Monoclinic |
Monoclinic |
Monoclinic |
Monoclinic |
Space group |
Pbca |
P21/n |
P![[1 with combining macron]](https://www.rsc.org/images/entities/char_0031_0304.gif) |
Pn |
P21/n |
P21/n |
C2/c |
a (Å) |
16.6948(7) |
5.63177(9) |
7.4608(2) |
7.969(5) |
8.6633(3) |
6.2387(2) |
17.9956(6) |
b (Å) |
7.1386(4) |
15.3211(3) |
10.2199(3) |
5.535(5) |
11.3506(3) |
12.7873(5) |
10.9480(3) |
c (Å) |
23.0042(12) |
15.0625(3) |
18.9450(7) |
14.965(5) |
14.3428(5) |
18.0003(8) |
15.7185(5) |
α (°) |
90 |
90 |
88.279(3) |
90 |
90 |
90 |
90 |
β (°) |
90 |
98.566(2) |
86.598(3) |
103.239(5) |
103.460(3) |
93.021(4) |
109.940(3) |
γ (°) |
90 |
90 |
79.657(2) |
90 |
90 |
90 |
90 |
V (Å3) |
2741.6(2) |
1285.16(4) |
1418.28(8) |
642.5(7) |
1371.66(8) |
1434.00(10) |
2911.14(16) |
Z |
8 |
4 |
4 |
2 |
4 |
4 |
8 |
ρcalcd (Mg m−3) |
1.715 |
1.715 |
1.620 |
1.720 |
1.680 |
1.607 |
1.597 |
T (K) |
100(2) |
100(2) |
120(2) |
100(2) |
173(2) |
173(2) |
120(2) |
λ (Å) |
0.71073 |
0.71073 |
0.71073 |
0.71073 |
0.71073 |
0.71073 |
1.54184 |
μ (MoKα, mm−1) |
2.161 |
2.298 |
2.086 |
2.301 |
2.159 |
2.065 |
15.950 |
F(000) |
1376 |
648 |
680 |
324 |
680 |
680 |
1376 |
θ Range (°) |
3.228–32.840 |
2.735–41.176 |
2.925–29.844 |
2.797–41.189 |
3.011–32.825 |
3.382–32.867 |
4.811–74.214 |
Index (hkl) ranges |
−24 ≤ h ≤ 25, −10 ≤ k ≤ 10, −34 ≤ l ≤ 33 |
−5 ≤ h ≤ 10, −28 ≤ k ≤ 27, −27 ≤ l ≤ 27 |
−9 ≤ h ≤ 9, −13 ≤ k ≤ 13, −26 ≤ l ≤ 25 |
−13 ≤ h ≤ 14, −10 ≤ k ≤ 6, −27 ≤ l ≤ 27 |
−13 ≤ h ≤ 12, −17 ≤ k ≤ 17, −13 ≤ l ≤ 21 |
−8 ≤ h ≤ 9, −19 ≤ k ≤ 18, −24 ≤ l ≤ 27 |
−22 ≤ h ≤ 17, −13 ≤ k ≤ 12, −18 ≤ l ≤ 19 |
Reflection collected |
34 454 |
19 013 |
22 076 |
8095 |
17 901 |
16 574 |
5951 |
Independent reflections |
4822 (R(int) = 0.0852) |
8312 (R(int) = 0.0315) |
7036 (R(int) = 0.0430) |
5439 (R(int) = 0.0297) |
4708(R(int) = 0.0438) |
4854 (R(int) = 0.0444) |
2885 (R(int) = 0.0339) |
Completeness to θmax (%) |
94.4 |
97.0 |
86.1 |
97.4 |
92.4 |
90.4 |
97.2 |
Absorption correction |
Multi-scan |
Analytical |
Analytical |
Analytical |
Multi-scan |
Multi-scan |
Analytical |
Refinement method |
Full-matrix least squares on F2 |
Data/restraints/parameters |
4822/0/164 |
8312/0/149 |
7036/0/315 |
5439/2/148 |
4708/0/158 |
4854/0/158 |
2885/0/166 |
GOF on F2 |
1.068 |
1.082 |
1.035 |
0.992 |
1.071 |
1.128 |
1.077 |
Final R indices (I > 2 σ(I)) |
R1 = 0.0524, wR2 = 0.0942 |
R1 = 0.0334 wR2 = 0.0716 |
R1 = 0.0405, wR2 = 0.0846 |
R1 = 0.0317 wR2 = 0.0557 |
R1 = 0.0310 wR2 = 0.0643 |
R1 = 0.0310 wR2 = 0.0796 |
R1 = 0.0329 wR2 = 0.0844 |
R indices (all data) |
R1 = 0.0736 wR2 = 0.1047 |
R1 = 0.0481 wR2 = 0.0799 |
R1 = 0.0584 wR2 = 0.0970 |
R1 = 0.0397 wR2 = 0.0607 |
R1 = 0.0451 wR2 = 0.0718 |
R1 = 0.0533 wR2 = 0.0865 |
R1 = 0.0356 wR2 = 0.0866 |
Max., min. residual electron density (e Å−3) |
1.763, −1.061 |
2.978, −1.190 |
3.011, −1.053 |
0.901, −0.930 |
0.707, −0.666 |
0.671, −0.796 |
2.669, −0.878 |
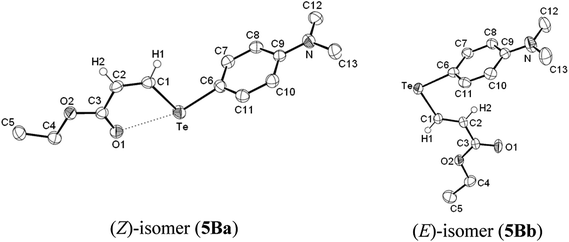 |
| Fig. 1 ORTEP diagrams for the isomeric pair of ethyl β-(4-N,N-dimethylaminophenyltelluro)acrylate. Hydrogen atoms other than the vinylic are omitted for clarity. | |
The observed molecular structures of the (β-aryltelluro)acrylates, ArTeCH
CHCOOR (Ar = 2, 3, 5; R = Me, Et) structurally characterized in the solid state in the present study, bearing a methyl/ethyl ester-functionalized vinyl ligand, are quite similar in the sense that Te(II) adopts a putative angular geometry with the Te–Csp2 bonds subtending an angle slightly greater than 90° at the Te atom (∠C–Te–C ranges between 90.89(12)° in 2Ba and 95.63(8)° in 5Ba). In each case, all the skeletal atoms of the methyl/ethyl ester-functionalized vinyl ligand are nearly coplanar and the mean planes of the two organic ligands are almost orthogonal. Compound 8 also possesses Z-configuration at the carbon–carbon double bond and the ring plane of the phenyl substituent is inclined at 29.67° with the mean plane of the disubstituted alkene. The bond parameters of the organic ligands are influenced little by the steric and electronic effects of the aryl and alkoxy groups and the Te–Cvinyl bond is always the shorter of the two Te–Csp2 bonds.
The C
C–C
O fragment of the trans disubstituted vinyl ligand, in case of the (E)-isomer of ethyl β-(4-N,N-dimethylaminophenyltelluro)acrylate (5Bb) has s-trans configuration but remarkably it adopts s-cis configuration in the cis disubstituted vinyl ligand present in the molecular structure of each of the (Z)-isomers structurally characterized in the solid state in the present study. Such a change in the orientation of the ester group may be attributed to the propensity of chalcogen atoms in these compounds with Z-geometry to be involved in an intramolecular Te⋯O(
C) secondary bonding interaction. The observed appreciably shorter interatomic distance between Te and carbonyl O atoms [d(Te, O) lies in the range 2.842(1) Å (3Ba)–2.969(2) Å (5Aa)] compared to the sum of van der Waals radii for Te and O [Σrvdr(Te, O) = 3.60 Å] is in agreement to the conclusion based on the lower ν(CO) values for the (Z) isomers compared to that observed for the corresponding (E) isomer or their parent ester (vide supra). Furthermore, the measure of the angle at the ester-functionalized Csp2 atom among the (Z)-isomers (∠C–C
C = 119.8(4)° in 3Ba–121.8(2)° in 5Aa) is close to the ideal value of 120°, compared with the larger values observed in case of 5Bb {125.1(3)°}, an (E)-isomer or 8 {127.8(3)°}, a (Z)-isomer lacking a carbonyl O atom. The latter compounds are devoid of such an intramolecular secondary bonding interaction. However, an appreciable closure approach observed between Te and carbonyl O atoms drawn from two different but adjacent molecules in the crystal lattice of 5Bb [d{Te, O1(1 + x, y, z)} = 3.278(1) Å < Σrvdr(Te, O) = 3.60 Å] appears to qualify an attractive interaction between them. Such an intermolecular Te⋯O(
C) secondary bonding interaction cooperated with the C9–H9A⋯O1 hydrogen bonding interaction (H-bond parameters: D–H, H⋯A, D–A and ∠D–H⋯A are 0.950, 2.470(1), 3.101(1) Å and 123.8(1)° respectively) gives rise to a one-dimensional supramolecular self-assembly extended along a-axis (Fig. S6†). The observed linearity of the intra- as well as intermolecular Caryl–Te⋯Ocarbonyl triads (∠C–Te⋯O ranges between 156.6(1)° in 5Aa and 163.6(1)° in 5Ba) that makes an n(O)→σ*(Te–C) overlap feasible is indicative of the significant covalent contribution to the Te⋯O bonding interaction. The chelate effect owing to the formation of a five-membered ring as a consequence of the intramolecular Te⋯O bonding among the tellurated acrylates with Z-geometry may be attributed to the predominance of this isomeric form in their formation.
Experimental
Preparative work was performed under dry nitrogen. The solvents were purified by standard procedures prior to their use. Phenyl acetylene, methyl propiolate and tellurium powder were procured from Aldrich, while ferrocene, 1-bromonaphthalene, 2-bromomesitylene, 2-bromothiophene and N,N-dimethyl aniline were obtained from Merck. All were used as received. Diaryl ditellurides were prepared by the reported method.8,23–26 Melting points were recorded in capillary tubes and are uncorrected. 1H NMR spectra (300.13 MHz and 400.16 MHz) in CDCl3 were recorded on Bruker DRX300 and Bruker Advance 400 Spectrometers using Me4Si as the internal standard. Solution 13C{1H} (100.54 MHz) and 125Te{1H} (126.19 MHz) NMR spectra were recorded in CDCl3 on a JEOL Eclipse Plus 400 NMR spectrometer and are referenced to Me4Si and Me2Te. Microanalyses were carried out using a Carlo Erba 1108 analyser. IR spectra were recorded as KBr pellets for all the tellurides reported herein as well as that of the (Z)- and (E)-isomers of methyl/ethyl β-(4-N,N-dimethylaminophenyltelluro)acrylates (5Aa and 5Ab/5Ba and 5Bb) in CHCl3 using a Perkin Elmer 577 spectrometer. The electrospray ionization-mass spectra (ESI-MS) of some representative compounds in acetonitrile solution were recorded on a Micromass Quattro II triple quadrupole mass spectrometer.
General procedure
Synthesis of methyl β-(aryltelluro)acrylates (ArTeCH
CHCOOMe, 1A–6A). The hydrotelluration of methyl propiolate with Ar2Te2/NaBH4 was achieved in methanol under dry nitrogen. In a representative experiment, sodium borohydride powder (0.113 g, 3.0 mmol) was added in small portions to a stirred solution of the dianisylditelluride (0.469 g, 1.0 mmol) and the methyl propiolate (0.18 mL, 2 mmol) in dry deoxygenated methanol (25 mL) under nitrogen at room temperature until the red colored solution turned pale yellow. The reaction mixture was further refluxed for 3 h. The resulting solution was concentrated under reduced pressure and 60 mL of brine added. The organic material was extracted with ethyl acetate (4 × 15 mL) and the combined organic phase dried over anhydrous sodium sulfate, filtered and concentrated under reduced pressure. The residue was screened by 1H NMR without purification to determine the isomeric ratio in the product. It was subjected to chromatographic separation of the (Z) and (E) isomers from the unreacted ditelluride on a silica column using hexane
:
ethyl acetate (100
:
0 to 80
:
20) mixtures as eluent. The usual work up of the fractions from column yielded isomerically pure methyl β-(anisyltelluro)acrylates 1Aa and 1Ab. The reactions using other ditellurides were carried out similarly.
1Aa. Pale yellow crystalline solid, yield: 0.351 g, 55%. M.p. 46–47 °C. Elemental analysis calcd (%) for C11H12O3Te: C 41.31, H 3.78; found: C 41.56, H 3.74. 1H NMR (300.13 MHz, CDCl3, Me4Si): δ 8.44 (d, 3J(1H–1H) = 9.3 Hz, 1H, vinyl, 2J(1H–125Te) = 10.0 Hz), 7.73 (d, 3J(1H–1H) = 8.7 Hz, 2H, anisyl o-H), 6.93 (d, 3J(1H–1H) = 9.3 Hz, 1H, vinyl, 3J(1H–125Te) = 14.0 Hz), 6.82 (d, 3J(1H–1H) = 8.7 Hz, 2H, anisyl m-H), 3.82 (s, 3H, anisyl OMe), 3.81 (s, 3H, ester OMe) ppm. 13C{1H} NMR (100.54 MHz, CDCl3, Me4Si): δ 169.3 (CO), 160.0, 142.0, 140.2, 121.7, 115.2, 109.0 (anisyl and vinyl), 55.1 (anisyl OMe), 52.0 (ester OMe) ppm. 125Te{1H} NMR (126.19 MHz, CDCl3, Me2Te): δ 728 ppm. IR (KBr, cm−1): 1680 ν(C
O). ESI-MS: m/z calcd for C11H13O4Te: 338.9 [M + OH]+; found: 339.0.
1Ab. Pale yellow crystalline solid, yield: 0.014 g, 2%. M.p. 51–52 °C. 1H NMR (300.13 MHz, CDCl3, Me4Si): δ 8.56 (d, 3J(1H–1H) = 16.2 Hz, 1H, vinyl, 2J(1H–125Te) = 74.0 Hz), 7.72 (d, 3J(1H–1H) = 9.0 Hz, 2H, anisyl o-H), 6.85 (d, 3J(1H–1H) = 9.0 Hz, 2H, anisyl m-H), 6.04 (d, 3J(1H–1H) = 16.2 Hz, 1H, vinyl, 3J(1H–125Te) = 13.0 Hz), 3.83 (s, 3H, anisyl OMe), 3.68 (s, 3H, ester OMe) ppm. 13C{1H} NMR (100.54 MHz, CDCl3, Me4Si): δ 164.9 (CO), 160.7, 142.0, 130.8, 127.2, 116.0, 100.1 (anisyl and vinyl), 55.2 (anisyl OMe), 51.5 (OMe) ppm. 125Te{1H} NMR (126.19 MHz, CDCl3, Me2Te): δ 613 ppm. IR (KBr, cm−1): 1701 ν(C
O). ESI-MS: m/z calcd for C11H13O4Te: 338.9 [M + OH]+; found: 339.0.
2Aa. Pale yellow powder, yield: 0.385 g, 57%. M.p. 55–56 °C. Elemental analysis calcd (%) for C14H12O2Te: C 49.48, H 3.56; found: C 49.67, H 3.52. 1H NMR (300.13 MHz, CDCl3, Me4Si): δ 8.41 (d, 3J = 9.3 Hz, 1H, vinyl), 8.27–8.30 (m, 1H, naphthyl), 8.18 (dd, 3J = 1.2 Hz, 3J = 1.2 Hz, 1H, naphthyl), 7.89 (d, 3J = 8.1 Hz 1H, naphthyl), 7.48–7.84 (m, 3H, naphthyl), 7.36 (t, 1H, naphthyl), 6.98 (d, 3J = 9.3 Hz, 1H, vinyl), 3.85 (s, 3H, OMe) ppm. 13C{1H} NMR (100.54 MHz, CDCl3, Me4Si): δ 169.5 (CO), 141.0, 139.7, 136.2, 133.6, 132.3, 130.0, 128.9, 126.9, 126.2, 122.2, 122.0 (naphthyl and vinyl), 52.0 (OMe) ppm. 125Te{1H} NMR (126.19 MHz, CDCl3, Me2Te): δ 636 ppm. IR (KBr, cm−1): 1673 ν(C
O).
2Ab. Yellow oil, yield: 0.019 g, 3%. 1H NMR (300.13 MHz, CDCl3, Me4Si): δ 8.56 (d, 3J = 16.5 Hz, 1H, vinyl), 8.18 (t, 2H, naphthyl), 7.95 (d, 3J = 8.1 Hz, 1H, naphthyl), 7.51–7.85 (m, 3H, naphthyl), 7.38 (t, 1H, naphthyl), 5.96 (d, 3J = 16.5 Hz, 1H, vinyl), 3.63 (s, 3H, OMe) ppm. 13C{1H} NMR (100.54 MHz, CDCl3, Me4Si): δ 164.8 (CO), 141.3, 136.0, 133.7, 132.1, 131.1, 129.2, 128.1, 128.0, 127.6, 126.7, 126.6, 114.4 (naphthyl and vinyl), 51.5 (OMe) ppm. 125Te{1H} NMR (126.19 MHz, CDCl3, Me2Te): δ 516 ppm. IR (KBr, cm−1): 1707 ν(C
O).
3Aa. Pale yellow crystalline solid, yield: 0.394 g, 59%. M.p. 85–87 °C. Elemental analysis calcd (%) for C13H16O2Te: C 47.05, H 4.86; found: C 47.32, H 4.80. 1H NMR (400.16 MHz, CDCl3, Me4Si): δ 8.21 (d, 3J(1H–1H) = 8.0 Hz, 1H, vinyl, 2J(1H–125Te) = 10.0 Hz), 7.01 (s, 2H, mesityl m-H), 6.99 (d, 3J(1H–1H) = 8.0 Hz, 1H, vinyl, 3J(1H–125Te) = 13.0 Hz), 3.85 (s, 3H, OMe), 2.56 (s, 6H, o-Me), 2.32 (s, 3H, p-Me) ppm. 13C{1H} NMR (100.54 MHz, CDCl3, Me4Si): δ 169.5 (CO), 144.7, 140.9, 139.2, 127.6, 122.3, 122.0 (mesityl and vinyl), 51.9 (OMe), 29.3 (o-Me), 20.9 (p-Me) ppm. 125Te{1H} NMR (126.19 MHz, CDCl3, Me2Te): δ 516 ppm. IR (KBr, cm−1): 1675 ν(C
O).
3Ab. Yellow powder, yield: 0.019 g, 3%. M.p. 44–46 °C. 1H NMR (400.16 MHz, CDCl3, Me4Si): δ 8.51 (d, 3J(1H–1H) = 16.0 Hz, 1H, vinyl, 2J(1H–125Te) = 90.0 Hz), 6.91 (s, 2H, mesityl m-H), 5.80 (d, 3J(1H–1H) = 16.0 Hz, 1H, vinyl, 3J(1H–125Te) = 12.0 Hz), 3.60 (s, 3H, OMe), 2.47 (s, 6H, o-Me), 2.23 (s, 3H, p-Me) ppm. 13C{1H} NMR (100.54 MHz, CDCl3, Me4Si): δ 165.0 (CO), 145.4, 140.3, 129.8, 127.9, 126.2, 115.6 (mesityl and vinyl), 51.4 (OMe), 29.0 (o-Me), 21.0 (p-Me) ppm. 125Te{1H} NMR (126.19 MHz, CDCl3, Me2Te): δ 387 ppm. IR (KBr, cm−1): 1709 ν(C
O).
4Aa. Orange crystalline solid, yield: 0.486 g, 61%. M.p. 98–99 °C. Elemental analysis calcd (%) for C14H14FeO2Te: C 42.28, H 3.55; found: C 42.53, H 3.53. 1H NMR (300.13 MHz, CDCl3, Me4Si): δ 8.40 (d, 3J(1H–1H) = 9.6 Hz, 1H, vinyl, 2J(1H–125Te) = 8.0 Hz), 6.85 (d, 3J(1H–1H) = 9.6 Hz, 1H, vinyl, 3J(1H–125Te) = 12.0 Hz), 4.38 (t, 2H, ferrocenyl), 4.27 (t, 2H, ferrocenyl), 4.18 (s, 5H, ferrocenyl), 3.81 (s, 3H, OMe) ppm. 13C{1H} NMR (100.54 MHz, CDCl3, Me4Si): δ 169.5 (CO), 143.2, 121.1 (vinyl), 78.3, 71.3, 69.0, 51.9 (ferrocenyl), 50.2 (OMe) ppm. 125Te{1H} NMR (126.19 MHz, CDCl3, Me2Te): δ 585 ppm. IR (KBr, cm−1): 1677 ν(C
O). ESI-MS: m/z calcd for C14H15FeO2Te: 398.9 [M + H]+, found: 398.4; m/z calcd for C14H17FeO3Te: 416.9 [M + H2O + H]+, found: 417.2.
4Ab. Yellow powder, yield: 0.032 g, 4%. M.p. 124–126 °C. 1H NMR (400.16 MHz, CDCl3, Me4Si): δ 8.46 (d, 3J(1H–1H) = 16.5 Hz, 1H, vinyl, 2J(1H–125Te) = 77.0 Hz), 6.05 (d, 3J(1H–1H) = 16.5 Hz, 1H, vinyl, 3J(1H–125Te) = 11.0 Hz), 4.39 (t, 2H, ferrocenyl), 4.34 (t, 2H, ferrocenyl), 4.19 (s, 5H, ferrocenyl), 3.67 (s, 3H, OMe) ppm. 13C{1H} NMR (100.54 MHz, CDCl3, Me4Si): δ 164.9 (CO), 131.7, 127.0 (vinyl), 79.1, 72.2, 69.3, 51.4 (ferrocenyl), 43.3 (OMe) ppm. 125Te{1H} NMR (126.19 MHz, CDCl3, Me2Te): δ 464 ppm. IR (KBr, cm−1): 1718 ν(C
O).
5Aa. Pale yellow crystalline solid, yield: 0.395 g, 59%. M.p. 99–100 °C. Elemental analysis calcd (%) for C12H15NO2Te: C 43.30, H 4.54, N 4.21; found: C 43.53, H 4.52, N 4.10. 1H NMR (400.16 MHz, CDCl3, Me4Si): δ 8.50 (d, 3J = 8.0 Hz, 1H, vinyl), 7.70 (d, 3J = 12.0 Hz, 2H, N,N-dimethylaniline o-H), 6.93 (d, 3J = 8.0 Hz, 1H, vinyl), 6.65 (d, 3J = 12.0 Hz, 2H, N,N-dimethylaniline m-H), 3.83 (s, 3H, OMe), 2.99 (s, 6H, NMe2) ppm. 13C{1H} NMR (100.54 MHz, CDCl3, Me4Si): δ 169.2 (CO), 150.5, 143.0, 140.1, 121.4, 113.3, 103.0 (N,N-dimethylaniline and vinyl), 51.8 (OMe), 40.2 (NMe2) ppm. 125Te{1H} NMR (126.19 MHz, CDCl3, Me2Te): δ 715 ppm. IR (KBr, cm−1): 1680 ν(C
O). ESI-MS: m/z calcd for C12H16NO2Te: 336.0 [M + H]+; found: 336.0.
5Ab. Pale yellow crystalline solid, yield: 0.035 g, 5%. M.p. 119–120 °C. 1H NMR (400.16 MHz, CDCl3, Me4Si): δ 8.59 (d, 3J = 16.0 Hz, 1H, vinyl), 7.64 (d, 3J = 12.0 Hz, 2H, N,N-dimethylaniline o-H), 6.64 (d, 3J = 12.0 Hz, 2H, N,N-dimethylaniline m-H), 6.04 (d, 3J = 16.0 Hz, 1H, vinyl), 3.70 (s, 3H, OMe), 3.03 (s, 6H, NMe2) ppm. 13C{1H} NMR (100.54 MHz, CDCl3, Me4Si): δ 165.0 (CO), 151.0, 141.8, 132.0, 126.7, 113.7, 94.0 (N,N-dimethylaniline and vinyl), 51.4 (OMe), 40.1 (NMe2) ppm. 125Te{1H} NMR (126.19 MHz, CDCl3, Me2Te): δ 594 ppm. IR (KBr, cm−1): 1687 ν(C
O). ESI-MS: m/z calcd for C12H16NO2Te: 336.0 [M + H]+; found: 336.0.
6Aa. Pale yellow crystalline solid, yield: 0.338 g, 57%. M.p. 43–45 °C. Elemental analysis calcd (%) for C8H8SO2Te: C 32.48, H 2.73; found: C 32.72, H 2.70. 1H NMR (400.16 MHz, CDCl3, Me4Si): δ 8.43 (d, 3J(1H–1H) = 8.0 Hz, 1H, vinyl, 2J(1H–125Te) = 8.0 Hz), 7.43 (d, 3J(1H–1H) = 4.0 Hz, 1H, thiophene), 7.37 (d, 3J(1H–1H) = 4.0 Hz, 1H, thiophene), 6.93 (dd, 1H, thiophene), 6.95 (d, 3J(1H–1H) = 8.0 Hz, 1H, vinyl, 3J(1H–125Te) = 14.0 Hz), 3.76 (s, 3H, OMe) ppm. 13C{1H} NMR (100.54 MHz, CDCl3, Me4Si): δ 170.0 (CO), 143.2, 140.4, 133.8, 128.9, 121.5, 108.0 (thiophene and vinyl), 52.2 (OMe) ppm. 125Te{1H} NMR (126.19 MHz, CDCl3, Me2Te): δ 610 ppm. IR (KBr, cm−1): 1672 ν(C
O). ESI-MS: m/z calcd for C8H9O3STe: 314.9 [M + OH]+, found: 315.4; m/z calcd for C4H5O2Te: 215.0 [MeOOCCH
CHTe]+, found: 215.4.
6Ab. Yellow oil, yield: 0.018 g, 3%. 1H NMR (400.16 MHz, CDCl3, Me4Si): δ 8.57 (d, 3J = 16.0 Hz, 1H, vinyl), 7.58 (d, 3J = 4.0 Hz, 1H, thiophene), 7.50 (d, 3J = 4.0 Hz, 1H, thiophene), 7.06 (dd, 1H, thiophene), 6.05 (d, 3J = 16.0 Hz, 1H, vinyl), 3.69 (s, 3H, OMe) ppm. 13C{1H} NMR (100.54 MHz, CDCl3, Me4Si): δ 164.8 (CO), 142.9, 136.0, 130.5, 129.5, 127.7, 123.8 (thiophene and vinyl), 51.6 (OMe) ppm. 125Te{1H} NMR (126.19 MHz, CDCl3, Me2Te): δ 475 ppm. IR (KBr, cm−1): 1717 ν(C
O).
Synthesis of ethyl β-(aryltelluro)acrylates (ArTeCH
CHCOOEt) 1B–6B. Compounds 1B–6B were prepared following the similar procedure mentioned above, except ethanol was used in the place of methanol as the solvent. The isolated crude products were almost all solids except 1B, 2B and 6B.
1Ba. Pale yellow crystalline solid, yield: 0.335 g, 50%. M.p. 30–31 °C. Elemental analysis calcd (%) for C12H14O3Te: C 43.17, H 4.23; found: C 43.53, H 4.20. 1H NMR (300.13 MHz, CDCl3, Me4Si): δ 8.42 (d, 3J(1H–1H) = 9.3 Hz, 1H, vinyl, 2J(1H–125Te) = 9.0 Hz), 7.73 (d, 3J(1H–1H) = 8.7 Hz, 2H, anisyl o-H), 6.92 (d, 3J(1H–1H) = 9.3 Hz, 1H, vinyl, 3J(1H–125Te) = 14.0 Hz), 6.82 (d, 3J(1H–1H) = 8.7 Hz, 2H, anisyl m-H), 4.27 (q, 3J = 7.2 Hz, 2H, OCH2), 3.81 (s, 3H, OMe), 1.32 (t, 3J = 7.2 Hz, 3H, Me) ppm. 13C{1H} NMR (100.54 MHz, CDCl3, Me4Si): δ 169.0 (CO), 160.0, 141.5, 140.2, 122.1, 115.2, 109.1 (anisyl and vinyl), 61.0 (OCH2), 55.1 (OMe), 14.3 (Me) ppm. 125Te{1H} NMR (126.19 MHz, CDCl3, Me2Te): δ 726 ppm. IR (KBr, cm−1): 1674 ν(C
O).
1Bb. Yellow oil, yield: 0.013 g, 2%. 1H NMR (300.13 MHz, CDCl3, Me4Si): δ 8.54 (d, 3J(1H–1H) = 16.2 Hz, 1H, vinyl), 7.65 (d, 3J(1H–1H) = 8.7 Hz, 2H, anisyl o-H), 6.73 (d, 3J(1H–1H) = 8.7 Hz, 2H, anisyl m-H), 6.05 (d, 3J(1H–1H) = 16.2 Hz, 1H, vinyl), 4.15 (q, 3J = 7.2 Hz, 2H, OCH2), 3.82 (s, 3H, OMe), 1.27 (t, 3J = 7.2 Hz, 3H, Me) ppm. 13C{1H} NMR (100.54 MHz, CDCl3, Me4Si): δ 164.7 (CO), 160.6, 145.4, 144.8, 127.5, 115.9, 102.0 (anisyl and vinyl), 60.4 (OCH2), 55.2 (OMe), 14.2 (Me) ppm. 125Te{1H} NMR (126.19 MHz, CDCl3, Me2Te): δ 611 ppm. IR (KBr, cm−1): 1710 ν(C
O).
2Ba. Pale yellow crystalline solid, yield: 0.349 g, 49%. M.p. 48–50 °C. Elemental analysis calcd (%) for C15H14O2Te: C 50.91, H 3.99; found: C 50.74, H 3.96. 1H NMR (300.13 MHz, CDCl3, Me4Si): δ 8.40 (d, 3J = 9.6 Hz, 1H, vinyl), 8.29–7.36 (m, 7H, naphthyl), 6.98 (d, 3J = 9.6 Hz, 1H, vinyl), 4.31 (q, 3J = 7.2 Hz, 2H, OCH2), 1.35 (t, 3J = 7.2 Hz, 3H, Me) ppm. 13C{1H} NMR (100.54 MHz, CDCl3, Me4Si): δ 169.0 (CO), 140.3, 139.6, 136.2, 133.5, 132.2, 129.9, 128.8, 126.8, 126.2, 122.6, 122.2 (naphthyl and vinyl), 61.0 (OCH2), 14.3 (Me) ppm. 125Te{1H} NMR (126.19 MHz, CDCl3, Me2Te): δ 634 ppm. IR (KBr, cm−1): 1672 ν(C
O). ESI-MS: m/z calcd for C15H15O3Te: 373.0 [M + OH]+; found: 373.3, m/z calcd for C5H7O2Te: 229.0 [EtOOCCH
CHTe]+; found: 229.2.
2Bb. Yellow oil, yield: 0.015 g, 2%. 1H NMR (300.13 MHz, CDCl3, Me4Si): δ 8.55 (d, 3J = 16.5 Hz, 1H, vinyl), 7.38–8.27 (m, 7H, naphthyl), 5.96 (d, 3J = 16.5 Hz, 1H, vinyl), 4.09 (q, 3J = 7.2 Hz, 2H, OCH2), 1.20 (t, 3J = 7.2 Hz, 3H, Me) ppm. 13C{1H} NMR (100.54 MHz, CDCl3, Me4Si): δ 169.1 (CO), 140.4, 139.7, 136.3, 133.6, 132.3, 130.0, 128.9, 126.9, 126.3, 122.7, 122.2 (naphthyl and vinyl), 61.0 (OCH2), 14.4 (Me) ppm. 125Te{1H} NMR (126.19 MHz, CDCl3, Me2Te): δ 512 ppm. IR (KBr, cm−1): 1708 ν(C
O).
3Ba. Pale yellow crystalline solid, yield: 0.352 g, 51%. M.p. 66–68 °C. Elemental analysis calcd (%) for C14H18O2Te: C 48.61, H 5.25; found: C 48.94, H 5.20. 1H NMR (300.13 MHz, CDCl3, Me4Si): δ 8.18 (d, 3J = 9.3 Hz, 1H, vinyl), 6.98 (s, 2H, mesityl m-H), 6.98 (d, 3J = 9.3 Hz, 1H, vinyl), 4.28 (q, 3J = 7.2 Hz, 2H, OCH2), 2.55 (s, 6H, o-Me), 2.29 (s, 3H, p-Me), 1.33 (t, 3J = 7.2 Hz, 3H, Me) ppm. 13C{1H} NMR (100.54 MHz, CDCl3, Me4Si): δ 169.0 (CO), 144.7, 140.4, 139.1, 127.5, 122.6, 122.2, (mesityl and vinyl), 60.7 (OCH2), 29.3 (o-Me), 20.9 (p-Me), 14.3 (Me) ppm. 125Te{1H} NMR (126.19 MHz, CDCl3, Me2Te): δ 514 ppm. IR (KBr, cm−1): 1676 ν(C
O). ESI-MS: m/z calcd for C14H19O3Te: 365.0 [M + OH]+; found: 365.3, m/z calcd for C5H7O2Te: 228.9 [EtOOCCH
CHTe]+; found: 229.3.
3Bb. Yellow oil, 0.016 g, 2%. 1H NMR (300.13 MHz, CDCl3, Me4Si): δ 8.47 (d, 3J(1H–1H) = 16.0 Hz, 1H, vinyl, 2J(1H–125Te) = 91.0 Hz), 6.88 (s, 2H, mesityl m-H), 5.78 (d, 3J(1H–1H) = 16.0 Hz, 1H, vinyl, 3J(1H–125Te) = 12.0 Hz), 4.04 (q, 3J = 7.2 Hz, 2H, OCH2), 2.28 (s, 6H, o-Me), 2.19 (s, 3H, p-Me), 1.19 (t, 3J = 7.2 Hz, 3H, Me) ppm. 13C{1H} NMR (100.54 MHz, CDCl3, Me4Si): δ 164.5 (CO), 145.3, 140.1, 129.1, 127.8, 126.6, 115.5 (mesityl and vinyl), 60.1 (OCH2), 29.0 (o-Me), 21.0 (p-Me), 14.1 (Me) ppm. 125Te{1H} NMR (126.19 MHz, CDCl3, Me2Te): δ 384 ppm. IR (KBr, cm−1): 1717 ν(C
O).
4Ba. Orange crystalline solid, yield: 0.448 g, 54%. M.p. 79–80 °C. Elemental analysis calcd (%) for C15H16FeO2Te: C 43.76, H 3.92; found: C 43.98, H 3.89. 1H NMR (300.13 MHz, CDCl3, Me4Si): δ 8.39 (d, 3J = 9.6 Hz, 1H, vinyl), 6.85 (d, 3J = 9.6 Hz, 1H, vinyl), 4.23–4.31 (m, 6H (2H for OCH2 and 4H for ferrocenyl)), 4.17 (s, 5H, ferrocenyl), 1.32 (t, 3J = 7.2 Hz, 3H, Me) ppm. 13C{1H} NMR (100.54 MHz, CDCl3, Me4Si): δ 169.2 (CO), 142.7, 121.6 (vinyl), 78.3, 71.3, 69.0, 60.9 (ferrocenyl), 50.3 (OCH2), 14.3 (Me) ppm. 125Te{1H} NMR (126.19 MHz, CDCl3, Me2Te): δ 582 ppm. IR (KBr, cm−1): 1673 ν(C
O).
4Bb. Yellow solid, yield: 0.026 g, 3%. M.p. 61–62 °C. 1H NMR (300.13 MHz, CDCl3, Me4Si): δ 8.45 (d, 3J = 16.5 Hz, 1H, vinyl), 6.10 (d, 3J = 16.5 Hz, 1H, vinyl), 4.33–4.40 (m, 6H, (2H for OCH2 and 4H for ferrocenyl)), 4.19 (s, 5H, ferrocenyl), 1.23 (t, 3J = 7.2 Hz, 3H, Me) ppm. 13C{1H} NMR (100.54 MHz, CDCl3, Me4Si): δ 166.2 (CO), 146.8, 121.0 (vinyl), 79.0, 71.4, 69.3, 69.1 (ferrocenyl), 50.20 (OCH2), 14.31 (Me) ppm. 125Te{1H} NMR (126.19 MHz, CDCl3, Me2Te): δ 475 ppm. IR (KBr, cm−1): 1713 ν(C
O).
5Ba. Pale yellow crystalline solid, yield: 0.368 g, 53%. M.p. 93–94 °C. Elemental analysis calcd (%) for C13H17NO2Te: C 45.01, H 4.94, N 4.04; found: C 45.23, H 4.92, N 4.01. 1H NMR (300.13 MHz, CDCl3, Me4Si): δ 8.45 (d, 3J(1H–1H) = 9.6 Hz, 1H, vinyl, 2J(1H–125Te) = 9.0 Hz), 7.66 (d, 3J(1H–1H) = 9.0 Hz, 2H, N,N-dimethylaniline o-H), 6.90 (d, 3J(1H–1H) = 9.6 Hz, 1H, vinyl, 3J(1H–125Te) = 13.0 Hz), 6.61 (d, 3J(1H–1H) = 9.0 Hz, 2H, N,N-dimethylaniline m-H), 4.26 (q, 3J = 7.2 Hz, 2H, OCH2), 2.96 (s, 6H, NMe2), 1.32 (t, 3J = 7.2 Hz, 3H, Me) ppm. 13C{1H} NMR (100.54 MHz, CDCl3, Me4Si): δ 168.8 (CO), 150.4, 142.4, 140.0, 121.8, 113.3, 103.2 (N,N-dimethylaniline and vinyl), 60.7 (OCH2), 40.2 (N–Me), 14.3 (Me) ppm. 125Te{1H} NMR (126.19 MHz, CDCl3, Me2Te): δ 712 ppm. IR (KBr, cm−1): 1670 ν(C
O). ESI-MS: m/z calcd for C13H18NO2Te: 350.0 [M + H]+, found: 350.1; m/z calcd for C13H18NO3Te: 366.0 [M + OH]+, found: 366.0.
5Bb. Pale yellow crystalline solid, yield: 0.030 g, 4%. M.p. 74–76 °C. 1H NMR (300.13 MHz, CDCl3, Me4Si): δ 8.56 (d, 3J(1H–1H) = 16.5 Hz, 1H, vinyl, 2J(1H–125Te) = 78.0 Hz), 7.63 (d, 3J(1H–1H) = 9.0 Hz, 2H, N,N-dimethylaniline o-H), 6.63 (d, 3J(1H–1H) = 9.0 Hz, 2H, N,N-dimethylaniline m-H), 6.02 (d, 3J(1H–1H) = 16.5 Hz, 1H, vinyl, 3J(1H–125Te) = 13.0 Hz), 4.13 (q, 3J = 7.2 Hz, 2H, OCH2), 2.99 (s, 6H, NMe), 1.24 (t, 3J = 7.2 Hz, 3H, Me) ppm. 13C{1H} NMR (100.54 MHz, CDCl3, Me4Si): δ 164.6 (CO), 150.9, 141.8, 131.4, 127.2, 113.7, 94.0 (N,N-dimethylaniline and vinyl), 60.2 (OCH2), 40.1 (NMe2), 14.2 (Me) ppm. 125Te{1H} NMR (126.19 MHz, CDCl3, Me2Te): δ 591 ppm. IR (KBr, cm−1): 1688 ν(C
O). ESI-MS: m/z calcd for C13H18NO2Te: 350.0 [M + H]+, found: 350.0.
6Ba. Yellow oil, yield: 0.299 g, 49%. Elemental analysis calcd (%) for C9H10O2STe: C 34.89, H 3.25; found: C 34.98, H 3.22. 1H NMR (400.16 MHz, CDCl3, Me4Si): δ 8.40 (d, 3J(1H–1H) = 8.0 Hz, 1H, vinyl, 2J(1H–125Te) = 8.0 Hz), 7.43 (d, 3J(1H–1H) = 4.0 Hz, 1H, thiophene), 7.36 (d, 3J(1H–1H) = 4.0 Hz, 1H, thiophene), 6.93 (dd, 1H, thiophene), 6.94 (d, 3J(1H–1H) = 8.0 Hz, 1H, vinyl, 3J(1H–125Te) = 14.0 Hz), 4.23 (q, 3J = 7.2 Hz, 2H, OCH2), 1.26 (t, 3J = 7.2 Hz, 3H, Me) ppm. 13C{1H} NMR (100.54 MHz, CDCl3, Me4Si): δ 169.5 (CO), 142.7, 140.3, 133.8, 128.8, 121.9, 108.2 (thiophene and vinyl), 61.2 (OCH2), 14.3 (Me) ppm. 125Te{1H} NMR (126.19 MHz, CDCl3, Me2Te): δ 608 ppm. IR (KBr, cm−1): 1667 ν(C
O).
6Bb. Yellow oil, yield: 0.014 g, 2%. 1H NMR (400.16 MHz, CDCl3, Me4Si): δ 8.44 (d, 3J = 12.0 Hz, 1H, vinyl), 7.52 (d, 3J = 4.0 Hz, 1H, thiophene), 7.45 (d, 3J = 4.0 Hz, 1H, thiophene), 7.03 (dd, 1H, thiophene), 6.97 (d, 3J = 12.0 Hz, 1H, vinyl), 4.32 (q, 3J = 7.2 Hz, 2H, OCH2), 1.36 (t, 3J = 7.2 Hz, 3H, Me) ppm. 13C{1H} NMR (100.54 MHz, CDCl3, Me4Si): δ 164.3 (CO), 142.8, 136.0, 130.1, 129.5, 128.2, 121.9 (thiophene and vinyl), 60.9 (OCH2), 14.2 (Me) ppm. 125Te{1H} NMR (126.19 MHz, CDCl3, Me2Te): δ 472 ppm. IR (KBr, cm−1): 1713 ν(C
O).
Synthesis of arylvinyltellurides (ArTeCH
CHPh). Hydrotelluration of phenyl acetylene (0.21 mL, 2 mmol) in ethanol using dinaphthyl- or dimesitylditelluride (1 mmol) following the general procedure gave 7 or 8 as yellow solid.
7. (Ar = 1-C10H7). Yield: 0.467 g, 65%. M.p. 38–40 °C. Elemental analysis calcd (%) for C18H14Te: C 60.41, H 3.94; found: C 60.64, H 3.88. 1H NMR (400.16 MHz, CDCl3, Me4Si): δ 8.23 (d, 3J = 8.0 Hz, 1H, naphthyl), 8.14 (d, 3J = 8.0 Hz, 1H, naphthyl), 7.79–7.86 (dd, 3J = 8.0 Hz, 3J = 8.0 Hz, 2H, naphthyl), 7.21–7.55 (m, 8H, aryl), 7.48 (d, 3J = 8.0 Hz, 1H, vinyl), 7.03 (d, 3J = 8.0 Hz, 1H, vinyl) ppm. 13C{1H} NMR (100.54 MHz, CDCl3, Me4Si): δ 139.1, 138.9, 136.9, 135.9, 133.6, 132.1, 129.8, 128.8, 128.5, 127.5, 127.5, 127.0, 126.3, 126.3, 118.3, 108.9 (aryl and vinyl) ppm. 125Te{1H} NMR (126.19 MHz, CDCl3, Me2Te): δ 451 ppm. ESI-MS: m/z calcd for C18H15OTe: 377.0 [M + OH]+, found: 377.3.
8. (2,4,6-Me3C6H2). Yield: 0.463 g, 66%. M.p. 88–89 °C. Elemental analysis calcd (%) for C17H18Te: C 58.35, H 5.18; found: C 58.43, H 5.16. 1H NMR (400.16 MHz, CDCl3, Me4Si): δ 7.31 (d, 3J = 8.0 Hz, 1H, vinyl), 7.22–7.43 (m, 5H, Ph), 6.98 (s, 2H, mesityl, m-H), 6.77 (d, 3J = 8.0 Hz, 1H, vinyl), 2.57 (s, 6H, o-Me), 2.29 (s, 3H, p-Me) ppm. 13C{1H} NMR (100.54 MHz, CDCl3, Me4Si): δ 144.8, 139.2, 139.0, 136.0, 128.4, 127.5, 127.2, 119.3, 109.1 (aryl and vinyl), 29.5 (o-Me), 20.9 (p-Me) ppm. 125Te{1H} NMR (126.19 MHz, CDCl3, Me2Te): δ 337 ppm. ESI-MS: m/z calcd for C17H19OTe: 369.0 [M + OH]+, found: 369.1.
Crystallography
Single crystals of compounds 2Ba, 3Aa, 3Ba, 5Aa, 5Ba, 5Bb and 8 were obtained by slow evaporation of hexane and hexane
:
ethyl acetate solvent mixture at ambient temperature. Intensity data for the crystals of 2Ba, 5Ba and 5Bb were collected on a Xcalibur, EOS, Gemini diffractometer equipped with a graphite monochromated Mo-Kα radiation (λ = 0.71073 Å), while data for crystal of other compounds was collected on a Supernova dual Atlas diffractometer system using Mo-Kα radiation (λ = 0.71073 Å for 3Aa, 3Ba and 5Aa and Cu-Kα radiation (λ = 1.54184 Å for 8)). CrysAlisPro27 was used for data collection, cell refinement and data reduction with either empirical or analytical absorption corrections applied. The structures were solved by direct methods and difference Fourier synthesis using Sir92 (ref. 28) or Superflip.29 SHELXL30 was used to perform full-matrix least-squares refinements on F2, using all data, with anisotropic displacement parameters applied to non-hydrogen atoms. Hydrogen atoms attached to carbon were included in geometrically calculated positions using a riding model and were refined isotropically. Figures of molecular structures showing 50% probability displacement ellipsoids were generated using ORTEP-3 version 2.02.†31
Conclusions
Hydrotelluration of methyl propiolate using Ar2Te2/NaBH4 in methanol provides stereoisomers of arylvinyltellurides, ArTeCH
CHCOOMe. In ethanol, however the corresponding ethyl esters ArTeCH
CHCOOEt are formed while in higher alcohols detelluration to result in the diaryltellurides, Ar2Te, is a strongly competing reaction and the alkyl group of the ester functionality remains unaltered. The presence of sterically demanding aryl ligands at Te, while not affecting the mode of hydrotelluration, tends to provide crystalline products suitable for X-ray analysis. The present study signifies the usefulness of δ(125Te) and 2J(1H–125Te) NMR parameters in distinguishing the stereoisomers, especially when the products are oils or in absence of suitable crystals for X-ray diffraction. The secondary bonding Te⋯O(C) interaction observed, at least in the solid state, among the (Z)-vinylic tellurides appears to improve the stereospecificity of the hydrotelluration reaction of carbonyl functionalized alkynes towards Z-geometry.
Acknowledgements
B. S. thanks University Grant Commission, New Delhi, India, for the award of ‘Dr D. S. Kothari post-doctoral fellowship’. The structure solutions provided by Dr Jerry Jasinski for compounds 2Ba, 5Ba and 5Bb and Dr Jan Wikaira for 8 is gratefully acknowledged.
Notes and references
- G. Zeni, D. S. Lüdtke, R. B. Panatieri and A. L. Braga, Chem. Rev., 2006, 106, 1032 CrossRef CAS PubMed.
- J. L. Princival, A. A. D. Santos and J. V. Comasseto, J. Braz. Chem. Soc., 2010, 21, 2042 CrossRef CAS.
- S. M. Barros, M. J. Dabdoub, V. M. B. Dabdoub and J. V. Comasseto, Organometallics, 1989, 8, 1661 CrossRef CAS.
- J. C. R. Freitas, D. J. Palmeira, R. A. Oliveira, P. H. Menezes and R. O. Silva, Magn. Reson. Chem., 2012, 50, 481 CrossRef CAS PubMed.
- G. Zeni, O. S. do R. Barros, A. V. Moro, A. L. Braga and C. Peppe, Chem. Commun., 2003, 1258 RSC.
-
(a) S. R. Buzilova, L. I. Vereshchagin, I. D. Sadekov and V. I. Minkin, Zh. Obshch. Khim., 1976, 46, 932 (Chem. Abstr., 1976, 85, 20761g) CAS;
(b) S. R. Buzilova, I. D. Sadekov, T. V. Lipovich, T. M. Filippova and L. I. Vereshchagin, Zh. Obshch. Khim., 1977, 47, 1999 (Chem. Abstr., 1978, 88, 22289v) CAS.
- X.-S. Mo and Y.-Z. Huang, Tetrahedron Lett., 1995, 36, 3539 CrossRef CAS.
- M. R. Detty, B. J. Murray, D. L. Smith and N. Zumbulyadis, J. Am. Chem. Soc., 1983, 105, 875 CrossRef CAS.
- M. R. Detty and B. J. Murray, J. Am. Chem. Soc., 1983, 105, 883 CrossRef CAS.
- S. Uemura, S.-I. Fukuzawa and S. R. Patil, J. Organomet. Chem., 1983, 243, 9 CrossRef CAS.
- H. Takashashi, K. Ohe, S. Uemura and N. Sugita, Nippon Kagaku Kaishi, 1987, 7, 1508 CrossRef.
- V. I. Minkin, I. D. Sadekov, B. B. Rivkin, A. V. Zakharov, V. L. Nivorozhkin, O. E. Kompan and Y. T. Struchkov, J. Organomet. Chem., 1997, 536–537, 233 CrossRef.
- S. Fujiwara, Y. Shimizu, T. Shin-ike and N. Kambe, Org. Lett., 2001, 3, 2085 CrossRef CAS PubMed.
- X. Huang, C.-G. Liang, Q. Xu and Q.-W. He, J. Org. Chem., 2001, 66, 74 CrossRef CAS PubMed.
- A. K. S. Chauhan, S. N. Bharti, R. C. Srivastava, R. J. Butcher and A. Duthie, J. Organomet. Chem., 2012, 708–709, 75 CrossRef CAS.
- G. Le Guillanton, A. V. Martynov, J. Delaunay, N. Mercier and A. Riou, J. Chem. Res., 1998, 681, 3001 Search PubMed.
- S. Yamago, M. Miyoshi, H. Miyazoe and J. Yoshida, Angew. Chem., Int. Ed., 2002, 41, 1407 CrossRef CAS.
- S. Kawaguchi, T. Ohe, T. Shirai, A. Nomoto, M. Sonoda and A. Ogawa, Organometallics, 2010, 29, 312 CrossRef CAS.
- A. Nomoto, S. Hayashi, T. Fujiyama, T. Ikeda, K. Hirata, J. Tohoma, K. Kakiuchi, W. Nakanishi and A. Ogawa, Bull. Chem. Soc. Jpn., 2014, 87, 550 CrossRef CAS.
- J. Terao, N. Kambe and N. Sonoda, Tetrahedron Lett., 1998, 39, 5511 CrossRef CAS.
- J. Yan and Z.-C. Chen, Synth. Commun., 2000, 30, 2359 CrossRef CAS.
- Y. Yu. Rusakov, L. B. Krivdin, F. F. Østerstrøm, S. P. A. Sauer, V. A. Potapov and S. V. Amosova, Phys. Chem. Chem. Phys., 2013, 15, 13101 RSC.
- M. Akiba, M. V. Lakshmikantham, K.-Y. Jen and M. P. Cava, J. Org. Chem., 1984, 49, 4819 CrossRef CAS.
- G. Mugesh, A. Panda, S. Kumar, S. D. Apte, H. B. Singh and R. J. Butcher, Organometallics, 2002, 21, 884 CrossRef CAS.
- L. Engman and J. Persson, J. Organomet. Chem., 1990, 388, 71 CrossRef CAS.
- L. Engman and M. P. Cava, Organometallics, 1982, 1, 470 CrossRef CAS.
- CrysAlis PRO, Agilent Technologies, Yarnton, England, 2014 Search PubMed.
- A. Altomare, G. Cascarano, C. Giacovazzo and A. Guagliardi, J. Appl. Crystallogr., 1993, 26, 343–350 CrossRef.
- L. Palatinus and G. Chapuis, J. Appl. Crystallogr., 2007, 40, 786–790 CrossRef CAS.
- G. M. Sheldrick, Acta Crystallogr., Sect. C: Struct. Chem., 2015, 71, 3–8 CrossRef PubMed.
- L. J. Farrugia, J. Appl. Crystallogr., 1997, 30, 565 CrossRef CAS.
Footnote |
† Electronic supplementary information (ESI) available: ORTEP diagram for compounds 2Ba, 3Aa, 3Ba, 5Aa and 8 are given in Fig. S1–S5. One-dimensional supramolecular architecture in 5Bb are given in Fig. S6. Bond parameters for C–H⋯O interaction in 5Bb are given in Table S1. CCDC 1061067–1061073. For ESI and crystallographic data in CIF or other electronic format see DOI: 10.1039/c5ra07854g |
|
This journal is © The Royal Society of Chemistry 2015 |