DOI:
10.1039/C5RA06791J
(Paper)
RSC Adv., 2015,
5, 49459-49465
Superhydrophobic surface on copper via a one-step solvent-free process and its application in oil spill collection†
Received
15th April 2015
, Accepted 28th May 2015
First published on 28th May 2015
Abstract
A one-step and solvent-free process was developed to fabricate a superhydrophobic surface on copper by high-temperature oxidation (300 °C) in an atmosphere of gasified 1-dodecanethiol (DT). The so-obtained superhydrophobic surface on a copper mesh was used to build a miniature oil containment boom (MOCB) that was used for oil spill collection. It was found that the MOCB was efficient with a collection rate of over 89% and durable with a negligible decrease in collection rate and deterioration in superhydrophobicity even after 25 cycles of kerosene collection.
1. Introduction
Superhydrophobic surfaces with a high water contact angle (CA, above 150°) and low sliding angle (SA, below 10°) have gained significant research attention because of their wide potential application in our daily life and industrial production.1–8 Traditionally, superhydrophobic surfaces are fabricated using a two-step process: (1) creation of rough structures on different substrates; and (2) passivation by low-surface-energy molecules.9,10 However, this two-step process is complex and has limited practical application due to the emission of waste solvent. Recently, a novel one-step process based on simultaneous surface roughening and passivation has been proposed for superhydrophobic surface fabrication.11–17 For instance, after simply immersed in ethanol solution of n-tetradecanoic acid for 3–5 days, superhydrophobic Cu(CH3(CH2)12COO)2 coating was fabricated on copper.18 Such a process is time-consuming. Electrodeposition in different electrolytic solution such as ethanol solution of stearic acid,19 ethanol solution of myristic acid/nickel chloride20 and ethanol solution of myristic acid/cerium chloride21 was used to reduce the deposition time.
These reported one-step processes19–21 are simpler in comparison to the traditional two-step process. However, the used organic solvent such as ethanol can have adverse impacts on the environment. Consequently, an aqueous solution of silver nitrate/benzoic acid was used to fabricate superhydrophobic surfaces on copper substrate by galvanic silver deposition.16 In this context, the aim of this study was to develop an even more environment-friendly strategy to fabricate superhydrophobic copper by a one-step solvent-free process. The copper substrate was roughened by oxidation and passivated by the gasified 1-dodecanethiol (abridged as DT) molecules in a sealed jar (50 mL) with small amount of DT (10 μL) at high temperature (300 °C). The environmental impact of this strategy is expected to be extremely low since there is no solvent emission in the fabrication process.
Additionally, the environment-friendly feature of the superhydrophobic surfaces on copper is incorporated in the potential application. Superhydrophobic surfaces have been used in wide range of environment and energy related applications such as self-cleaning, corrosion resistance, drag reduction, and ice retardation.19,22,23 Furthermore, superhydrophobic surfaces showed excellent performance in the oil spill collection.24–29 For example, Wang et al.25–27 compared superhydrophobic surfaces based method against traditional methods of oil containment booms, skimmers, dispersants, bioremediation and in situ burning. The superhydrophobic surfaces – based oil/water separation or oil spill collection was found to be recyclable and free of secondary pollution. Inspired by these studies, miniature oil containment boom folded from the superhydrophobic copper mesh fabricated by such a one-step and solvent-free process was used to collect spilled oils including kerosene, toluene, gasoline, and diesel oil from water.
2. Materials and methods
2.1 Sample fabrication
The copper plate (99.7%, Sinopharm Chemical Reagent Co., Ltd) was ground with abrasive paper and cleaned successively for 10 min in acetone, ethanol and ultrapure water. Then, the cleaned copper substrate was introduced into a sealed jar (50 mL) containing 10 μL 1-dodecanethiol (DT, 97%, Sigma-Aldrich), which was placed in an oven and heated to the preset temperature (200 °C, 300 °C or 400 °C) with the rate of 10 °C min−1 an maintained for different time (0.5 h–2 h). After that, the samples were cooled down to room temperature naturally. The so-obtained superhydrophobic copper plate was coded as Cu-HT&DT.
Traditional two-step process to render superhydrophobicity was also carried out. Specifically, the cleaned copper plate was firstly sealed in a jar, which was placed in an oven and heated to 300 °C with the rate of 10 °C min−1 and maintained for 1 h. The so-obtained sample was coded as Cu-HT. Then, the Cu-HT sample was immersed into an ethanol solution of DT (1.0 wt%) at room temperature for 1 h, followed by rinsing with ethanol thoroughly and drying with a stream of air. The so-obtained sample was coded as Cu-HT-DT.
Control sample coded as Cu-DT-HT was also fabricated. Firstly, the cleaned copper substrate was immersed into an ethanolic solution of DT (1.0 wt%) at room temperature for 1 h, followed by rinsing with ethanol thoroughly and drying with a stream of air. Then, the copper substrate was sealed in a jar at 300 °C for 1 h. After that, the samples were cooled down to room temperature naturally. The macroscopic appearance, microscopic surface morphology, and surface wettability of the control samples of Cu-HT, Cu-HT-DT, and Cu-DT&HT, were shown in Fig. S1.†
To fabricate superhydrophobic copper mesh (1200 #, Anhua Fardware Product Co., Ltd.), the substrate was firstly cleaned in HCl (0.1 M), acetone, ethanol, and ultrapure water successively. Then, it was introduced into a sealed jar (50 mL) containing 10 μL DT at 300 °C for 1 h.
2.2 Characterization
Contact angle (CA) and sliding angle (SA) were measured on a contact angle meter (Easydrop, Krüss, Germany) equipped with a computer-controlled liquid dispensing system at room temperature. All the reported CA and SA were determined by averaging values measured with an ultrapure water droplet of 5 μL at five different positions on each sample surface. The microscopic surface morphology and element composition were characterized on a field-emission scanning electron microscope (FE-SEM, Nova NanoSEM, FEI, USA) equipped with an energy-dispersive X-ray spectrometer (EDX, INCA 250, Oxford, UK) at an accelerating voltage of 15 kV. The crystallographic structure was characterized on an X-ray diffraction meter (XRD, Bruker-axs, D8ADVANCE, Germany) equipped with the graphite monochromatized Cu Kα radiation at a scanning speed of 4° min−1 in the range from 10° to 70°.
Miniature oil containment boom (2.0 cm × 3.5 cm × 0.5 cm) folded from the superhydrophobic copper mesh was used for the investigation of oil spill collection. Kerosene (1 #, Datang Petroleum Industry Co. Ltd), toluene (Shanghai Chemical Reagent Co. Ltd.), gasoline (93 #, Datang Petroleum Industry Co. Ltd) and diesel oil (10 #, Datang Petroleum Industry Co. Ltd) were chosen to simulate the oil spill.
3. Results and discussion
3.1 Superhydrophobic surfaces on copper plate
In this one-step process, treatment temperature and time are the key factors that influence the surface wettability (Fig. 1). For the treatment temperature of 200 and 300 °C, CA increased gradually to above 150° when the treatment time was increased from 0.5 to 2 h. The corresponding SA for the sample with CA above 150° was quite different. Specifically, when the treatment temperature was increased from 200 to 300 °C, SA decreased from 90 to below 10°. For the treatment temperature of 400 °C, CA decreased from approx. 80° (0.5 h) to approx. 52° (1 h) and finally to approx. 9° (2 h) with the increase in treatment time.
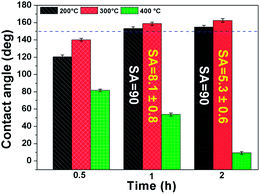 |
| Fig. 1 Influence of treatment time (0.5 h, 1 h, 2 h) and treatment temperature (200 °C, 300 °C, 400 °C) on contact angle for the Cu-HT&DT sample. SA is the abbreviation of sliding angle. | |
Field-emission scanning electron microscope (FE-SEM) images for the Cu-HT&DT samples treated for 1 h at different treatment temperature are shown in Fig. 2. Despite the different surface features, all samples were rough structured. For the Cu-HT&DT sample treated at 200 °C, micro-balls and micro-stripes were observed (Fig. 2(a)). The observation under higher magnification suggests that the micro-balls were also not smooth (Fig. 2(b)). For the Cu-HT&DT sample treated at 300 °C (Fig. 2(d)), relatively smaller micro-stripes and nano-cubes were formed, whereas the surfaces were covered by disordered micro- and nano-cubes for the sample treated at 400 °C (Fig. 2(f)). It was supposed that superhydrophobicity could be obtained on all these micro-/nano-structured surfaces if the samples were passivated properly.30 However, only the sample treated at the temperature of 300 °C was superhydrophobic (Fig. 1). It is hypothesized that the failure to obtain superhydrophobicity can be attributed to the surface chemistry rather than the surface structures. To test this hypothesis, non-superhydrophobic samples were transferred into ethanolic solution of DT for 1 h to further passivate the surface, after which the samples turned into superhydrophobic. This is demonstrated by CA and SA being 159.3 ± 1.5° and 5.7 ± 0.8°, respectively for sample treated at 200 °C, while for sample treated at 400 °C, CA and SA turned to 163.5 ± 1.6° and 3.4 ± 0.7°, respectively.
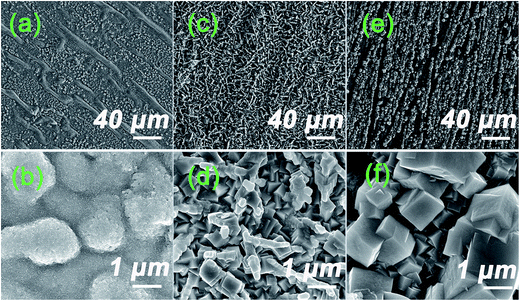 |
| Fig. 2 Field-emission scanning electron microscope images of samples treated for 1 h at different temperature of 200 °C (a and b), 300 °C (c and d), and 400 °C (e and f). All surfaces are rough-structured. However, only the sample treated for 1 h at 300 °C is superhydrophobic among these samples (as shown in Fig. 1). | |
The role of surface chemistry on the superhydrophobicity was investigated based on EDX analyses (Table 1). The sulfur element originated from the absorbed DT molecules was the highest for the Cu-HT&DT sample treated at 300 °C (11.44 weight% or 7.13 atomic%), while it was lower for the samples treated at 200 °C (1.22 weight% or 1.63 atomic%) and 400 °C (3.60 weight% or 4.60 atomic%). This can be attributed to the gasification temperature of DT, which is 266–283 °C, and stability of DT molecules at different temperatures.31–34 Specifically, when the treatment temperature is 200 °C, DT molecules are not gasified and consequently the amount of the absorbed DT onto the solid substrate is small. When the treatment temperature is 400 °C, DT molecules are decomposed. Furthermore, as the treatment time increases, the decomposition is more thorough and the surface becomes more hydrophilic (for treatment time of 2 h, surface superhydrophilicity with CA of approx. 9° was obtained, Fig. 1).
Table 1 Elemental composition obtained from the energy-dispersive X-ray spectrometer for the Cu-HT&DT samples treated for 1 h at different temperature
Element |
Weight% |
Atomic% |
200 °C |
300 °C |
400 °C |
200 °C |
300 °C |
400 °C |
C |
5.40 |
37.76 |
4.07 |
19.18 |
62.79 |
19.90 |
O |
8.28 |
15.10 |
11.45 |
22.08 |
18.85 |
29.33 |
S |
1.22 |
11.44 |
3.6 |
1.63 |
7.13 |
4.60 |
Cu |
85.09 |
35.70 |
80.88 |
57.11 |
11.22 |
52.17 |
From above discussions, it is apparent that the treatment temperature of 300 °C is effective to produce superhydrophobic surfaces. At this treatment temperature, the influence of treatment time on surface wettability was investigated. FE-SEM images of the Cu-HT&DT samples treated at 300 °C for 0.5 h and 2 h are shown in Fig. 3(a). For 0.5 h, micro-balls and micro-stripes were formed on copper substrate and the morphological microstructures were similar to the sample treated at 200 °C for 1 h. The CA for the Cu-HT&DT sample treated at 300 °C for 0.5 h was approx. 140° (Fig. 1), which was also close to that for the Cu-HT&DT sample treated at 200 °C for 1 h. The similarity in surface morphology and wettability suggested that the reaction atmosphere for these two samples were quite similar, i.e. the gasified DT molecules under the conditions of 200 °C per 1 h and 300 °C per 0.5 h were inadequate due to the lower treatment temperature (200 °C) or shorter treatment time (0.5 h). Consequently, the so-prepared the Cu-HT&DT samples were hydrophobic with similar surface morphological micro-structures. As the treatment time increased to 1 h, more DT molecules were gasified and the amount of DT molecules that were attached to the substrate had increased. Consequently, the surface morphology and wettability had changed as shown in Fig. 1 and 2(c)/2(d). When the treatment time was further increased to 2 h, rough surface structures of micro- /nano – balls were formed [Fig. 3(a)] leading to formation of the surface superhydrophobicity (Fig. 1).
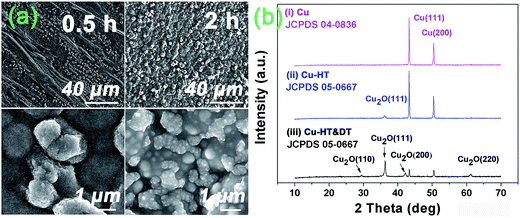 |
| Fig. 3 (a) Field-emission scanning electron microscope images of the Cu-HT&DT samples treated at 300 °C for 0.5 and 2 h; (b) X-ray diffraction patterns of copper substrates (i), copper treated for 1 h at 300 °C without 1-dodecanethiol (ii), and copper treated for 1 h at 300 °C with 10 μL 1-dodecanethiol (iii). HT is the abbreviation of hot treatment; DT is the abbreviation of 1-dodecanethiol. | |
X-ray diffraction (XRD) patterns of different samples were shown in Fig. 3(b). The bare copper substrate [Fig. 3(b)-(i)] showed characteristic peaks of Cu (111) (2 theta = 43.30°) and Cu (200) (2 theta = 50.42°). For the Cu-HT&DT sample [Fig. 3(b)-(iii)], new peaks with 2 theta values of 29.45°, 36.35°, 42.35°, and 61.43° ascribed to Cu2O (110), Cu2O (111), Cu2O (200), and Cu2O (220), respectively, appeared.35 This suggests that the copper was oxidized into Cu2O probably through the following reaction equations:36
It was supposed that, at the beginning of reaction, copper is turned into CuO (eqn (1)), the growth of which decreased gradually on account of the consumption of oxygen when the reaction time increased. Meanwhile, CuO generated on the surface could be reduced into Cu2O by Cu(0) of the copper substrate (eqn (2)). The strongest peak for the Cu-HT&DT sample located at 2 theta = 36.35° was also observed for the sample of Cu-HT [Fig. 3(b)-(ii)]. However, other weak peaks appeared in the XRD pattern of the Cu-HT&DT sample ascribed to Cu2O were not observed. This suggests that the gasified DT molecules can promote formation of Cu2O. The detailed mechanism for such promotion remains unknown. However, it is hypothesized that the reducibility of DT molecules cannot be neglected in the formation of Cu2O by the one step process. For example, as discovered by Fonder et al.,37 CuO was reduced to Cu2O by the thiol molecules. DT molecules would react with Cu2O to facilitate the surface attachment through the following reaction:38
|
2CH3(CH2)10CH2SH + Cu2O → 2CH3(CH2)10CH2SCu + H2O
| (3) |
3.2 Superhydrophobic surfaces on copper mesh
From the above discussions, it is concluded that the optimum temperature to fabricate superhydrophobic surfaces on copper plate was 300 °C and the optimum treatment time was 1 or 2 h. Superhydrophobic surfaces on copper plate fabricated at 300 °C for 1 h were found to be superoleophilic with CA of approximately 0° (Fig. S2†).39,40 For the copper mesh, after treated at 300 °C for 1 h, the surface turns into relatively rougher [Fig. 4(a-i and ii)] in comparison to the pristine one (Fig. S3†). Meanwhile, surface with superhydrophobicity (CA = 162.8 ± 2.2°, SA = 3.5 ± 0.8°) and superoleophilicity (CA of approx. 0°) was obtained.
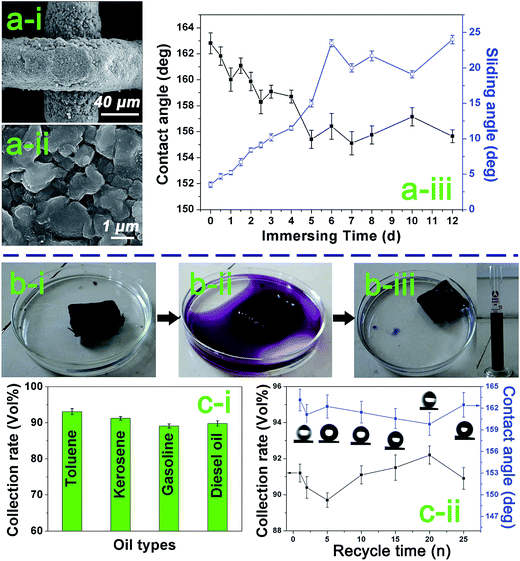 |
| Fig. 4 Field-emission scanning electron microscope images of copper mesh treated for 1 h at 300 °C (a-i, a-ii). Evolution of contact angles and sliding angles for superhydrophobic surfaces immersed into water (a-iii). The miniature oil containment boom floating on water (b-i), the collecting of kerosene from water (b-ii, the volume of kerosene is 10 mL), and the collected kerosene (b-iii, the collected kerosene is 9.1 mL). Collection rate of the miniature oil containment boom for different oils in the first cycle (c-i). Effect of kerosene/water collection cycle on the collection rate and contact angles (c-ii). | |
The special surface wettability, i.e., superhydrophobicity and superoleophilicity, of copper mesh for the oil spill collection was investigated. The primary factor influencing the use of the mesh in these potential applications is the stability of superhydrophobic surfaces,24–26,38,41 which was simply evaluated by measuring the variation of surface wettability for the superhydrophobic copper mesh immersed into water [Fig. 4(a-iii)]. Before measuring, the superhydrophobic copper mesh was taken out from the water and dried using nitrogen stream. The experimental results showed that the mesh exhibited good water repellency after immersed for 12 days (CA = 155.7 ± 2.5°, SA = 24.0 ± 2.2°). The superhydrophobic copper mesh was also immersed into ethanol for 30 min under ultrasonication (the power and frequency for the ultrasonic cleaner is 100 W and 40 kHz). After that, the surface superhydrophobicity was still retained (CA = 156.8 ± 1.2°, SA = 11.2 ± 1.5°).
Miniature oil containment boom (MOCB, 2.0 cm × 3.5 cm × 0.5 cm) was folded from a sheet of superhydrophobic and superoleophilic copper mesh. The MOCB was found to be floating freely on the water surface [Fig. 4(b-i)]. MOCB readily absorbed the kerosene when 10 mL of it was poured into the water [Fig. 4(b-ii)]. The contained kerosene was continuously collected using a pump and the final kerosene volume collected was 9.1 mL [Fig. 4(b-iii)]. As shown in Mov. S1,† the time taken for the collection was less than 2 min. The oil collection rate (k) of the MOCB was calculated using the following formula
where
ν0 is initial volume of oil deliberately poured into the water surface and
ν is the volume of the collected oil. The collection rate of the MOCB for other oils such as toluene, gasoline, and diesel oil was also investigated [
Fig. 4(c-i)]. The
k values were greater than 89% for all cases after the first collection cycle, which were comparable with the reported data.
24,42,43 Moreover, the repeated use of the MOCB was studied. After a cycle, the MOCB was contaminated by oil. The superhydrophobicity in contaminated MOCB was regenerated by cleaning it with the ethanol and then drying it at room temperature for 1 h. The regeneration facilitated the repeated use of the MOCB. As illustrated in
Fig. 4(c-ii), the collection rate
k was greater than 89% even after using the MOCB for 25 cycles in kerosene collection from the water surface. Additionally, CA was greater than 150° and SA was smaller than 10°, which demonstrate its good reproducibility.
4. Conclusion
An environmentally benign route was proposed to fabricate superhydrophobic surfaces on copper. The optimum treatment temperature for the fabrication route was 300 °C, which is close to the gasification temperature of DT and the optimum treatment time was 1 h or 2 h. The so-fabricated superhydrophobic surface was superoleophilic. The unique surface wettability, i.e., superhydrophobicity and superoleophilicity, facilitates the use for collecting oil spill from water surface. The MOCB was folded from a superhydrophobic and superoleophilic copper mesh and was efficient with a collection rate of over 89% and durable with the negligible decrease in collection rate and the deterioration in superhydrophobicity after 25 cycles of kerosene collection.
Acknowledgements
This work was supported by the National Natural Science Foundation of China (Grant no. 21203089).
References
- L. P. Wen, Y. Tian and L. Jiang, Bioinspired Super-wettability from fundamental research to practical applications, Angew. Chem., Int. Ed., 2015, 54, 3387–3399 CrossRef CAS PubMed.
- Y. Tian, B. Su and L. Jiang, Interfacial material system exhibiting superwettability, Adv. Mater., 2014, 26, 6872–6897 CrossRef CAS PubMed.
- Y. Li, G. T. Duan, G. Q. Liu and W. P. Cai, Physical processes-aided periodic micro/nanostructured arrays by colloidal template technique: fabrication and applications, Chem. Soc. Rev., 2013, 42, 3614–3627 RSC.
- J. F. Ou, W. H. Hu, M. S. Xue, F. J. Wang and W. Li, Superhydrophobic surfaces on light alloy substrates fabricated by a versatile process and their corrosion protection, ACS Appl. Mater. Interfaces, 2013, 5, 3101–3107 CAS.
- C. Y. Wang, M. Zhang, Y. Xu, S. L. Wang, F. Liu, M. L. Ma, D. L. Zang and Z. X. Gao, One-step synthesis of unique silica particles for the fabrication of bionic and stably superhydrophobic coatings on wood surface, Adv. Powder Technol., 2014, 25, 530–535 CrossRef CAS PubMed.
- A. Chaudhary and H. C. Barshilia, Nanometric multiscale rough Cu/Cu(OH)2 superhydrophobic surface prepared by a facile one-step solution-immersion process: transition to superhydrophilicity with oxygen plasma treatment, J. Phys. Chem. C, 2011, 115, 18213–18220 CAS.
- D. A. Wang, T. C. Hu, L. T. Hu, B. Yu, Y. Q. Xia, F. Zhou and W. M. Liu, Micro-structured TiO2 nanotube arrays for improved photoelectrocatalysis and mechanical stability, Adv. Funct. Mater., 2009, 19, 1930–1938 CrossRef CAS PubMed.
- S. Nagappana and C. S. Ha, Emerging trends in superhydrophobic surface based magnetic materials: fabrications and their potential applications, J. Mater. Chem. A, 2015, 3, 3224–3251 Search PubMed.
- Y. Li, C. C. Li, S. O. Cho, G. T. Duan and W. P. Cai, Silver hierarchical bowl-like array: synthesis, superhydrophobicity, and optical properties, Langmuir, 2007, 23, 9802–9807 CrossRef CAS PubMed.
- Y. Li, E. J. Lee and S. O. Cho, Superhydrophobic coatings on curved surfaces featuring remarkable supporting force, J. Phys. Chem. C, 2007, 111, 14813–14817 CAS.
- T. Ning, W. G. Xu and S. X. Lu, One-step controllable fabrication of superhydrophobic surfaces with special composite structure on zinc substrates, J. Colloid Interface Sci., 2011, 361, 388–396 CrossRef CAS PubMed.
- Z. Chen, L. M. Hao, A. Q. Chen, Q. J. Song and C. L. Chen, A rapid one-step process for fabrication of superhydrophobic surface by electrodeposition method, Electrochim. Acta, 2012, 59, 168–171 CrossRef CAS PubMed.
- R. Qiu, P. Wang, D. Zhang and J. J. Wu, One-step preparation of hierarchical cobalt structure with inborn superhydrophobic effect, Colloids Surf., A, 2011, 377, 144–149 CrossRef CAS PubMed.
- J. Li, H. Q. Wan, Y. P. Ye, H. D. Zhou and J. M. Chen, One-step process for the fabrication of superhydrophobic surfaces with easy repairability, Appl. Surf. Sci., 2012, 258, 3115–3118 CrossRef CAS PubMed.
- I. Bernagozzi, C. Antonini1, F. Villa and M. Marengo, Fabricating superhydrophobic aluminum: an optimized one-step wet synthesis using fluoroalkyl silane, Colloids Surf., A, 2014, 441, 919–924 CrossRef CAS PubMed.
- D. K. Sarkar and N. Saleema, One-step fabrication process of superhydrophobic green coatings, Surf. Coat. Technol., 2010, 204, 2483–2486 CrossRef CAS PubMed.
- J. Song, S. Huang, Y. Lu, X. Bu, J. E. Mates, A. Ghosh, R. Ganguly, C. J. Carmalt, I. P. Parkin, W. Xu and C. M. Megaridis, Self-driven one-step oil removal from oil spill on water via selective-wettability steel mesh, ACS Appl. Mater. Interfaces, 2014, 6, 19858–19865 CAS.
- S. T. Wang, L. Feng and L. Jiang, One-step solution-immersion process for the fabrication of stable bionic superhydrophobic surfaces, Adv. Mater., 2006, 18, 767–770 CrossRef CAS PubMed.
- Y. Huang, D. K. Sarkar and X.-G. Chen, A one-step process to engineer superhydrophobic copper surfaces, Mater. Lett., 2010, 64, 2722–2724 CrossRef CAS PubMed.
- Z. Chen, L. M. Hao, A. Q. Chen, Q. J. Song and C. L. Chen, A rapid one-step process for fabrication of superhydrophobic surface by electrodeposition method, Electrochim. Acta, 2012, 59, 168–171 CrossRef CAS PubMed.
- Y. Liu, S. Y. Li, J. J. Zhang, Y. M. Wang, Z. W. Han and L. Q. Ren, Fabrication of biomimetic superhydrophobic surface with controlled adhesion by electrodeposition, Chem. Eng. J., 2014, 248, 440–447 CrossRef CAS PubMed.
- J. Xu, J. L. Xu, Y. Cao, X. B. Ji and Y. Y. Yan, Fabrication of non-flaking, superhydrophobic surfaces using a one-step solution-immersion process on copper foams, Appl. Surf. Sci., 2013, 286, 220–227 CrossRef CAS PubMed.
- H. J. Wang, J. Yu, Y. Z. Wu, W. J. Shao and X. L. Xu, A facile two-step approach to prepare superhydrophobic surfaces on copper substrates, J. Mater. Chem. A, 2014, 2, 5010–5017 CAS.
- L. Feng, Z. G. Zhang, Z. H. Mai, Y. M. Ma, B. Q. Liu, L. Jiang and D. B. Zhu, A super-hydrophobic and super-oleophilic coating mesh film for the separation of oil and water, Angew. Chem., Int. Ed., 2004, 43, 2012–2014 CrossRef CAS PubMed.
- F. J. Wang, S. Lei, C. Q. Li, J. F. Ou, M. S. Xue and W. Li, Superhydrophobic Cu mesh combined with a superoleophilic polyurethane sponge for oil spill adsorption and collection, Ind. Eng. Chem. Res., 2014, 53, 7141–7148 CrossRef CAS.
- F. J. Wang, S. Lei, M. S. Xue, J. F. Ou, C. Q. Li and W. Li, Superhydrophobic and superoleophilic miniature device for the collection of oils from water surfaces, J. Phys. Chem. C, 2014, 118, 6344–6351 CAS.
- F. J. Wang, S. Lei, M. S. Xue, J. F. Ou and W. Li, In situ separation and collection of oil from water surface via a novel superoleophilic and superhydrophobic oil containment boom, Langmuir, 2014, 30, 1281–1289 CrossRef CAS PubMed.
- L. H. Kong, X. H. Chen, L. G. Yu, Z. S. Wu and P. Y. Zhang, Superhydrophobic cuprous oxide nanostructures on phosphor-copper meshes and their oil–water separation and oil spill cleanup, ACS Appl. Mater. Interfaces, 2015, 7, 2616–2625 CAS.
- B. Wang, W. Liang, Z. Guo and W. Liu, Biomimetic super-lyophobic and super-lyophilic materials applied for oil/water separation: a new strategy beyond nature, Chem. Soc. Rev., 2015, 44, 336–361 RSC.
- Q. F. Cheng, L. Jiang and Z. Y. Tang, Bioinspired layered materials with superior mechanical performance, Acc. Chem. Res., 2014, 47, 1256–1266 CrossRef CAS PubMed.
- J. A. Dean, Lange's Handbook of Chemistry, 1991, vol. 7, p. 370 Search PubMed.
- D. Chen, P. L. Cui, H. B. Cao and J. Yang, A 1-dodecanethiol-based phase transfer protocol for the highly efficient extraction of noble metal ions from aqueous phase, J Environ. Sci., 2015, 29, 146–150 CrossRef PubMed.
- L. X. Mo, D. Z. Liu, W. Li, L. H. Li, L. C. Wang and X. Q. Zhou, Effects of dodecylamine and dodecanethiol on the conductive properties of nano-Ag films, Appl. Surf. Sci., 2011, 257, 5746–5753 CrossRef CAS PubMed.
- L. Chen, J. M. Chen, H. D. Zhou, D. J. Zhang and H. Q. Wa, Synthesis of dodecanethiol monolayer-stabilized nickel nanoparticles, Mater. Sci. Eng., A, 2007, 452–453, 262–266 CrossRef PubMed.
- S. Dehghanpour, A. Mahmoudi, M. M. Ghazi, N. Bazvand, S. Shadpour and A. Nemati, Cu2O microsphere, microspherical composite of Cu2O/Cu nanocrystals and various Cu microcrystals: in situ hydrothermal conversion of Cu-aminodiphosphonate complexes, Powder Technol., 2013, 246, 148–156 CrossRef CAS PubMed.
- G. F. Ma, S. Liu, P. Wang, J. X. Chang, M. H. Zhang and R. Li, Synthesis of pod-like Cu2O nanowire arrays on Cu substrate, Mater. Lett., 2014, 120, 212–215 CrossRef CAS PubMed.
- G. Fonder, F. Laffineur, J. Delhalle and Z. Mekhalif, Alkanethiol-oxidized copper interface: the critical influence of concentration, J. Colloid Interface Sci., 2008, 326, 333–338 CrossRef CAS PubMed.
- L. Boinovich and A. Emelyanenko, A wetting experiment as a tool to study the physicochemical processes accompanying the contact of hydrophobic and superhydrophobic materials with aqueous media, Adv. Colloid Interface Sci., 2012, 179–182, 133–141 CrossRef CAS PubMed.
- Q. F. Cheng, M. Z. Li, Y. M. Zheng, B. Su, S. T. Wang and L. Jiang, Janus interface materials: superhydrophobic air/solid interface and superoleophobic water/solid interface inspired by a lotus leaf, Soft Matter, 2011, 7, 5948–5951 RSC.
- Q. F. Cheng, M. Z. Li, F. Yang, M. J. Liu, L. Li, S. T. Wang and L. Jiang, An underwater pH-responsive superoleophobic surface with reversibly switchable oil-adhesion, Soft Matter, 2012, 8, 6740–6743 RSC.
- L. Boinovich, A. Domantovskiy, A. Emelyanenko, A. Pashinin, A. Ionin, S. Kudryashov and P. Saltuganov, Femtosecond laser treatment for the design of electro-insulating superhydrophobic coatings with enhanced wear resistance on glass, ACS Appl. Mater. Interfaces, 2014, 6, 2080–2085 CAS.
- M. H. Jin, J. Wang, X. Yao, M. Y. Liao, Y. Zhao and L. Jiang, Underwater oil capture by a three-dimensional network architectured organosilane surface, Adv. Mater., 2011, 23, 2861–2864 CrossRef CAS PubMed.
- Z. X. Xue, S. T. Wang, L. Lin, L. Chen, M. J. Liu, L. Feng and L. Jiang, A novel superhydrophilic and underwater superoleophobic hydrogel-coated mesh for oil/water separation, Adv. Mater., 2011, 23, 4270–4273 CrossRef CAS PubMed.
Footnote |
† Electronic supplementary information (ESI) available. See DOI: 10.1039/c5ra06791j |
|
This journal is © The Royal Society of Chemistry 2015 |
Click here to see how this site uses Cookies. View our privacy policy here.