DOI:
10.1039/C5RA06786C
(Paper)
RSC Adv., 2015,
5, 57006-57020
Chitosan coated curcumin nanocrystals augment pharmacotherapy via improved pharmacokinetics and interplay of NFκB, Keap1 and Nrf2 expression in Gram negative sepsis†
Received
15th April 2015
, Accepted 9th June 2015
First published on 9th June 2015
Abstract
Background and purpose: Gram negative sepsis is a leading cause of mortality in cases of complicated intra-abdominal infections due to the deficit of clinically viable options for therapy. The purpose of the present study was to investigate the potential of intracorporeal chitosan coated curcumin nanocrystals (Chi-CUR-NC-4b) as a parenteral therapeutic approach against endotoxemia-induced sepsis. Experimental approaches: curcumin (CUR) nanocrystals were fabricated by high pressure homogenization and coated with chitosan. These nanocrystals were evaluated for physiochemical and pharmacokinetic parameters. Comparative evaluations were also made between Chi-CUR-NC-4b and CUR in LPS-stimulated HepG2 and J774 cells, as well as in the murine model of LPS-induced sepsis for PK, pharmacodynamic parameters and survival studies. Key results: Chi-CUR-NC 4b (D 95% and zeta potential 443 ± 15.76 nm and +28.82 ± 1.52 mV, respectively) was found to neutralize LPS and it enhanced plasma drug concentration (16 times compared to CUR solution) with improved levels in the organs of interest, i.e. lungs and liver. In vitro and in vivo pharmacodynamic studies demonstrated that the protective effects were mediated by the up-regulation of Nrf2 (enhanced antioxidant activity, i.e. via elevated levels of SOD and GST) as well as via the down-regulation of NFκB, leading to reduced cytokine secretion in the murine model of sepsis. Furthermore, reduction in tissue injury (attributed to reduced neutrophil migration indicated by myeloperoxidase levels) also contributed to enhanced survival in the murine model of LPS-induced endotoxemia. Conclusions and implications: these results together prove that the developed curcumin-bearing nano-formulation could serve as a valuable option for the therapeutic intervention of sepsis and associated hyper-inflammatory disorders.
1. Introduction
Currently, critical care illnesses induce heavy burdens globally; even enhanced supportive care is not able to reduce the mortality and morbidity associated with severe infections like gut-derived sepsis1 and acute respiratory distress syndrome. In particular, in the case of gut-derived sepsis, prompt induction to antibiotic therapy is not capable of preventing multiple organ failure and death by overwhelming inflammatory response, indicating an unmet and urgent need for the development of novel therapies for sepsis.1
CUR is a phenolic derivative reported to have a number of properties like anticancer, anti-inflammatory,2 and antioxidant activities.3 Although CUR has emerged as an important antisepsis molecule4 that affects the development of sepsis via various molecular pathways,5 poor aqueous solubility, high metabolism after oral as well as parenteral administration and poor stability at basic pH limit its development as a curative agent,5 whereas efforts for solubilization using co-solvents resulted in the hemolysis of RBC6 during parenteral administration. Therefore, an appropriate delivery system that can avoid these complications and also delivers the drug in therapeutic concentrations at the site of action is needed. Recently, nanocrystals have emerged as a potential parenteral nanocarrier system that can be scaled-up to a commercial level. Nanocrystals can significantly increase the saturation and dissolution velocity of water insoluble drugs. Nanocrystals can be employed for targeted delivery of drugs by both passive and active targeting by modulating nanocrystal size as well as surface properties via appropriate ligands.
Hemodialysis by a polymyxin B-immobilized column is the only clinically used approach following the recent recall of human recombinant-activated protein C.4 Hemodialysis is usually very painful and introduces fatal risks associated with the procedure, indicating a need for a suitable endotoxin binding moiety for parenteral application. Chitosan is a cationic, biocompatible polyelectrolyte used widely in drug delivery applications and has been demonstrated to interact with LPS and form a stable LPS–chitosan complex. The induction of TNF-α, IL-6 secretion by a LPS–chitosan complex is less in comparison to parent LPS. Chitosan has also been found to protect mice from sepsis by decreasing oxidative tissue damage and proinflammatory mediators.3 Moreover, chitosan oligosaccharides have been reported to dampen LPS-stimulated inflammatory responses (release of TNF-α, IL-6) by preventing the LPS-mediated stimulation of TLR4/MD-2 receptor and inhibiting MAP kinase and phosphatidylinositol-3′-kinase(PI3K)/Akt signaling pathways.6
Herein, we propose the first example of an intracorporeal therapy, combining the antiendotoxin approach with an anti-inflammatory (anti-NFκB) and antioxidant approach using nanotechnology for the treatment of sepsis, i.e. Chi-CUR-NC-4b for dual targeting in the therapy of Gram-negative sepsis. Cationic nanocrystals will serve a dual purpose; first, chitosan coating is postulated to reduce the interaction of LPS (via complex formation with the coated chitosan mediated by ionic interaction); second, the CUR has anti-inflammatory actions at the cellular level and has an effect on survival in the in vivo murine model of LPS-induced endotoxemia.
2. Results
2.1 Preparation, optimization and characterization of nanocrystals
In a series of initial experiments, the formulations were prepared using high-pressure homogenization (HPH) (Micro fluidics 110P). The nanocrystals were optimized in terms of size, size distribution and zeta potential by varying the stabilizers and their concentrations (Table 1).
Table 1 Optimization and characterization of curcumin nanocrystals fabricated by Microfluidizer
Batch code |
Amount of CUR (% w/v) |
Stabilizers (% w/v) |
Z average d.nm (mean ± SD) |
PDI (mean ± SD) |
Zeta potential (mV) (mean ± SD) |
Tween 80 |
Pluronic F68 |
CUR-NC 1 |
0.2 |
1 |
— |
368.4 ± 23.4 |
0.628 ± 0.043 |
−32.62 ± 1.04 |
CUR-NC 2 |
0.2 |
2 |
— |
327.33 ± 78.60 |
0.486 ± 0.164 |
−29.46 ± 0.91 |
CUR-NC 3 |
0.2 |
— |
1 |
303.26 ± 11.68 |
0.236 ± 0.012 |
−24.42 ± 0.92 |
CUR-NC 4 |
0.2 |
— |
2 |
232.1 ± 08.46 |
0.101 ± 0.022 |
−21.86 ± 0.72 |
CUR-NC 5 |
0.4 |
1 |
— |
514.05 ± 46.54 |
0.661 ± 0.068 |
−34.15 ± 1.26 |
CUR-NC 6 |
0.4 |
2 |
— |
695.4 ± 86.28 |
0.424 ± 0.088 |
−31.62 ± 1.46 |
CUR-NC 7 |
0.4 |
— |
1 |
556.68 ± 25.62 |
0.248 ± 0.025 |
−27.56 ± 0.82 |
CUR-NC 8 |
0.4 |
— |
2 |
407.81 ± 18.46 |
0.208 ± 0.028 |
−23.32 ± 1.28 |
The main parameters affecting the size and polydispersity index (PDI) were the number of homogenization cycles, the amount of CUR and the concentrations of Pluronic F68 (PF-68) and Tween 80 stabilizers. Zeta potential was affected principally by stabilizer type, concentration and CUR amount. Increase in stabilizer concentration in all the cases led to a reduction in the particle size of the nanosuspension, whereas PDI and size were higher in batches stabilized with Tween 80 (CUR-NC 1, 2, 5 and 6) as compared to the batches stabilized with PF 68 (CUR-NC 3, 4, 7 and 8). The homogenization of the CUR nanosuspension at 1500 mPa for 20 cycles was found to be optimum to achieve smaller nanocrystals with a minimal polydispersity index (PDI) (Fig. 1).
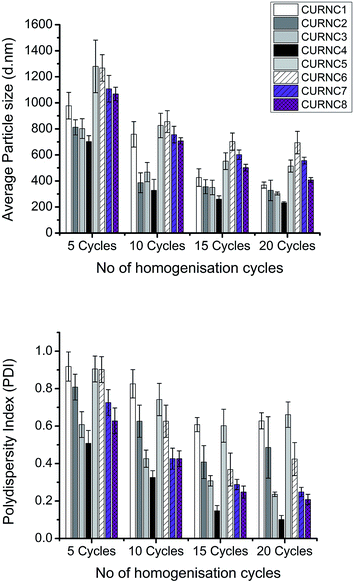 |
| Fig. 1 Change in particle size and PDI as a function of homogenization cycles in individual batches of nanocrystals at 1500 bars. Batches containing 2 mg ml−1 of CUR, i.e. CUR-NC 1 (stabilized with 1% w/v Tween 80); CUR-NC 2 (stabilized with 2% w/v Tween 80); CUR-NC 3 (stabilized with 1% w/v PF-68) and CUR-NC 4 (stabilized with 2% w/v PF 68). Batches containing 4 mg ml−1 of CUR, i.e. CUR-NC 5 (stabilized with 1% w/v Tween 80); CUR-NC 6 (stabilized with 2% w/v Tween 80); CUR-NC 7 (stabilized with 1% w/v PF-68) and CUR-NC 8 (stabilized with 2% w/v PF 68). | |
Generally in the case of batches stabilized by Tween 80 (i.e. CUR-NC 1 and CUR-NC 2, containing 0.2% w/v CUR in the dispersion, and CUR-NC 5 and CUR-NC 6, containing 0.4% w/v CUR in the dispersion) the change in particle size was not significant and they showed higher values of PDI as the number homogenization cycles was increased from 15 to 20. In the case of CUR-NC 3, stabilized with 1% w/v of PF 68, the particle size was found to be 303.26 ± 11.68 d.nm with PDI 0.236 ± 0.012, while the particle size and PDI in the case of CUR-NC 4 (stabilized with 2% w/v PF 68) were found to be 232.1 ± 8.46 d.nm and 0.101 ± 0.022, respectively, after 20 cycles of homogenization. Gradual reduction in particle size and PDI was observed when the number of homogenization cycles was increased from 15 to 20 keeping the concentration of CUR constant at 0.2% w/v. However, in the case of CUR-NC 7 and CUR-NC 8, which were prepared using 0.4% w/v of CUR, the particle size was larger compared to CUR-NC 3 and CUR-NC 4. The particle size and PDI of CUR-NC 7 were found to be 556.68 ± 25.62 d.nm and 0.248 ± 0.025, respectively, when stabilized with 1% w/v of PF-68, while in the case of CUR-NC 8, which is stabilized with 2% w/v of PF-68, the particle size and PDI were found to be 407.81 ± 18.46 d.nm and 0.208 ± 0.028, respectively. This data clearly indicates that on increasing CUR concentration from 0.2 to 0.4% w/v, the particle size also increased.
A similar effect was also observed in the case of formulations stabilized with Tween 80; however, the particle size was larger compared to the formulation stabilized using PF-68.
In the case of batches stabilized with Tween 80 (1% w/v), an increase in the amount of the drug in the initial suspension from 0.2% (CUR-NC 1) to 0.4% (CUR-NC 5) resulted in increases in particle size (368.4 ± 23.4 d.nm and 514.05 ± 46.54 d.nm for CUR-NC 1 and CUR-NC 5, respectively) and PDI (0.628 ± 0.043 and 0.661 ± 0.068 for CUR-NC 1 and CUR-NC 5, respectively).
Similar behavior was also observed with the batches stabilized with 2% w/v Tween 80; the particle size of CUR-NC 2 was found to be 327.33 ± 78.60 d.nm with a PDI of 0.486 ± 0.064. In the case of CUR-NC 6, the particle size and PDI were found to be 695.4 ± 86.28 d.nm and 0.424 ± 0.088, respectively (Table 1). Zeta potential was found to be negative in all the formulations; however, an increase in zeta potential was observed in the batches stabilized with PF-68 as compared to those stabilized with Tween 80 at the same surfactant concentration (Table 1). SEM and AFM analysis of the optimized batch (CUR-NC 4) confirmed the plate-like morphology with a size distribution between 100 and 350 d.nm (Fig. 2).
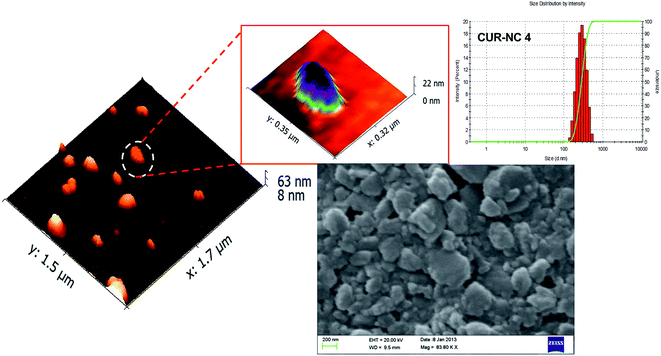 |
| Fig. 2 SEM and AFM images demonstrating plate-like morphology of optimized nanocrystals (CUR-NC 4). | |
2.1.1 Optimization of coating on nanocrystals with chitosan. Batch CUR-NC 4 was selected based on minimal size and PDI for electrostatic coating with chitosan using different concentrations of chitosan solution (0.01, 0.1 and 1% w/v concentration in nanosuspension). Coating with chitosan, in general terms, resulted in an increase in particle size and reversal of zeta potential at all the concentrations of chitosan. At higher concentrations (Chi-CUR-NC 4a) (0.1% w/v), aggregation was more evident (size and zeta potential, 448.48 ± 68 d.nm and +34.26 ± 1.46 mV, respectively) compared to the coated crystals with 0.01% chitosan solution (Chi-CUR-NC 4b) having particle size and zeta potential of 248.6 ± 18.26 d.nm and +28.82 ± 1.52 mV, respectively (Table 2).
Table 2 Optimization of coating of CUR-NC 4 with chitosan and its effect on size, PDI and zeta potential
Batch code |
Amount of chitosan in final nano-suspension (% w/v) |
Visual appearance of nanosuspension |
Z average d.nm (mean ± SD) |
PDI (mean ± SD) |
Zeta potential (mV) (mean ± SD) |
CUR-NC 4 |
0 |
Dispersed |
232.1 ± 08.46 |
0.101 ± 0.022 |
−21.86 ± 0.72 |
Chi-CUR-NC 4b |
0.01 |
Dispersed |
248.6 ± 18.26 |
0.214 ± 0.032 |
+28.82 ± 1.52 |
Chi-CUR-NC 4a |
0.1 |
Slight flocculation |
448.48 ± 68 |
0.421 ± 0.098 |
+34.26 ± 1.46 |
Chi-CUR-NC 4 |
1 |
Severe aggregation |
Not evaluated |
Not evaluated |
Not evaluated |
2.2 In vitro interaction studies between Chi-CUR-NC 4b and LPS by agarose gel electrophoresis and electrokinetic potential measurements
Lipopolysaccharide micelles were negatively charged under experimental conditions (phosphate buffer, pH 5.5) with a monomodal zeta-potential distribution. Electrostatically driven interactions of LPS with chitosan-coated NCs, i.e. Chi-CUR-NC 4b
:
LPS in the ratios of 2
:
1, 4
:
1, 6
:
1 and 8
:
1 v/v resulted in the reduction of the negative charge of LPS towards a more positive value (Fig. 3a). This observation was further confirmed by agarose gel electrophoresis of mixtures to determine the fraction of unbound LPS in the complex (Fig. 3b).
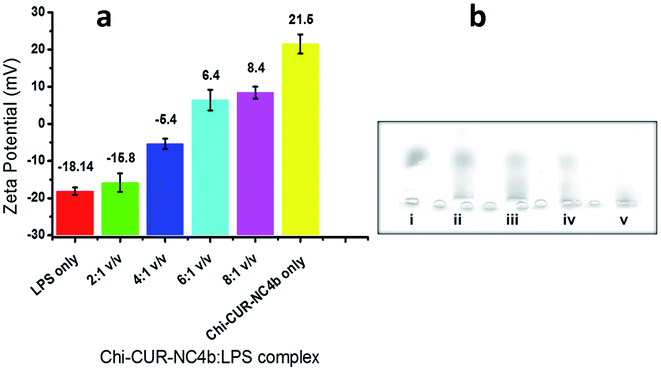 |
| Fig. 3 (a) Interaction studies between LPS and chitosan coated nanocrystals with zeta potential measurements. (b) Gel retardation assay. Fixed amount of LPS (1 mg ml−1) mixed with increasing concentrations of Chi-CUR-NC 4b. (i) 10 μl LPS alone (1 mg ml−1) (ii) Chi-CUR-NC 4b (20 μl) +LPS (10 μl) (iii) Chi-CUR-NC 4b (40 μl) + LPS (10 μl) (iv) Chi-CUR-NC 4b (60 μl) + LPS (10 μl) (v) Chi-CUR-NC 4b (80 μl) +LPS (10 μl). | |
2.3 Drug content and stability studies of NC suspension
Drug loss during homogenization was found to be minimal; drug content in the final optimized nanosuspension (CUR-NC 4) was found to be 98.56%, i.e. 1.9712 mg ml−1. Stability studies (Table 3) indicated that both the coated and uncoated nanocrystals were stable at 4 °C as well as at 25 °C, whereas the Tween 80 stabilized nanosuspension (CUR-NC 2) was found to be unstable (as indicated by an increase in the average nanocrystal size and polydispersity index). It was also demonstrated that coating of NCs stabilized by PF-68 (CUR-NC 4) with chitosan (Chi-CUR-NC 4b) led to slightly better stability at both storage conditions.
Table 3 Physical stability of selected formulations (average particle size < 500 nm)
Formulation |
Temperature °C |
Z average d.nm (mean ± SD) |
PDI (mean ± SD) |
Initial |
After 3 months |
Initial |
After 3 months |
CUR-NC 2 |
4 °C |
368.4 ± 23.4 |
896.8 ± 112 |
0.486 ± 0.164 |
0.823 ± 0.264 |
25 °C |
368.4 ± 23.4 |
642.2 ± 64.4 |
0.486 ± 0.164 |
0.546 ± 0.096 |
CUR-NC 4 |
4 °C |
232.1 ± 08.46 |
238 ± 14.4 |
0.101 ± 0.022 |
0.108 ± 0.032 |
25 °C |
232.1 ± 08.46 |
234 ± 04.4 |
0.101 ± 0.022 |
0.106 ± 0.024 |
Chi-CUR-NC 4b |
4 °C |
248.6 ± 18.26 |
251 ± 19.32 |
0.214 ± .032 |
0.203 ± .012 |
25 °C |
248.6 ± 18.26 |
249.8 ± 21.32 |
0.214 ± .032 |
0.198 ± .018 |
2.4 Uptake of nanocrystals by J774 macrophages by flow cytometry
Uptake of nanocrystals by J774 macrophage, assessed using flow cytometric analysis, demonstrated that CUR-NC 4 (red histogram) was able to deliver CUR into the cells in an efficient way as compared to plain CUR (blue histogram). Coating of NCs with chitosan further improved the uptake in macrophages (green histogram) as the position of the histogram moved further to the right (Fig. 4a).
 |
| Fig. 4 (a) Flow cytometric analysis for uptake of nanocrystals in J774 macrophages (i) not treated cells (ii) CUR solution as treatment (iii) CUR-NC treated cells (iv) Chi-CUR-NC 4b treated cells. (b) Hemolytic potential of chitosan coated and plain NCs at two treatment levels (i.e. 4 and 8 mg l−1 equivalent to curcumin, respectively). (c) Effects of NCs on viability of J774 macrophages and HEK 293 cells at 8 mg l−1 equivalent to curcumin treatment level. | |
2.5 In vitro hemolysis and cellular toxicity studies
Pain-free parenteral administration of the formulation is a very important goal and can be assessed using an in vitro hemolysis test, which determines the possibility of the formulation to cause hemolysis that leads to pain during i.v. administration of the formulation. Apart from pain, free hemoglobin can lead to phlebitis, jaundice, acute renal collapse, and, in some cases, death.
For evaluation of hemolytic potential, NCs having particle sizes less than 370 d.nm were selected (i.e. CUR-NC 1, CUR-NC 2, CUR-NC 3, CUR-NC 4, and Chi-CUR-NC 4b). In all the formulations, except CUR-NC 2 at an 8 mg l−1 treatment level, hemolysis was less than 25% at both the concentrations of 4 mg l−1 and 8 mg l−1 (Fig. 4b).
Cell viability studies were carried out on J774 macrophages and HEK 293 cells, and it was found that the viability of cells in the cases of formulations stabilized by Tween 80 was less than that in formulations stabilized with PF-68 at the treatment level equivalent to 8 mg l−1 of CUR. At 2% surfactant level, the viability of J774 macrophages and HEK 293 cell lines in the case of the formulation stabilized by Tween 80 (CUR-NC 2) was found to be 93.2% ± 2.2% and 90.4% ± 3.4%, respectively, compared to the formulation stabilized by PF-68 (CUR-NC 4) (98.32% ± 1.67% and 98.46% ± 1.26%, respectively). In the case of Chi-CUR-NC 4b, the viabilities were not significantly different from those of the uncoated NC formulation (CUR-NC 4) (97.46% ± 1.57% and 98.2% ± 1.47%, respectively) (Fig. 4c).
2.6 Modulation of NO, TNF-α release from LPS-treated J774 cells
The treatment of J774 macrophages with LPS (0.5 μg ml−1; positive control) led to the release of inflammatory mediators, i.e. NO and TNF-α in the supernatant of cells after 24 h of incubation. Prior treatment with CUR solution and CUR-NC 4, as well as Chi-CUR-NC 4b, led to a major reduction (P < 0.05) in TNF-α (Fig. 5a) and NO (Fig. 5b) levels in the supernatant in a concentration dependent manner. Chi-CUR-NC 4b was found to decrease the secretion of both TNF-α and NO induced by LPS to a minimal level. At a concentration equivalent to 3 μg ml−1 of CUR, the release levels of TNF-α and NO were 3.23 ± 1.04 μM and 6.60 ± 0.85 ng ml−1, respectively, when treated with Chi-CUR-NC 4b. However, when the cells were treated with CUR-NC 4, the TNF-α and NO levels were found to be higher at 6.18 ± 0.65 μM and 13.64 ± 0.48 ng ml−1, respectively. In the case of plain CUR solution, the levels of TNF-α and NO were 11.28 ± 1.26 μM and 19.62 ± 0.83 ng ml−1, respectively. This data indicates that due to coating with chitosan, the release of proinflammatory mediators was significantly reduced. Interestingly, even at a low concentration (equivalent to 0.75 μg ml−1 of CUR), the Chi-CUR-NC 4b was found to significantly reduce the release of proinflammatory mediators as compared to CUR-NC 4 and CUR solutions.
 |
| Fig. 5 Inhibition of LPS-induced (a) TNF-α secretion and (b) NO secretion in J774 macrophages by CUR solution, PF-68 stabilized CUR nanocrystals (CUR-NC 4) and chitosan coated CUR-NC 4 (Chi-CUR-NC 4b) *** denotes P < 0.001, ** denotes P < 0.01 and * denotes P < 0.05. | |
2.7 Modulation of protein expression by NCs in J774 macrophages and HepG2 cells
Western blotting studies (Fig. 6) demonstrated the modulation of protein expression of Keap1, NFκB and Nrf2 in both HepG2 and J774 cells that were stimulated with LPS on treatment with NCs (CUR-NC 4 and Chi-CUR-NC 4b). In the case of LPS-treated HepG-2 cells, Nrf2 expression was found to be markedly down-regulated (12.72% ± 3.6%) but was restored by treatment with both CUR-NC 4 (81.61% ± 4.38%) and Chi-CUR-NC 4b (69.91% ± 4.42%) as compared to the untreated cells (100%). Apart from this, the expression of Keap1 was found to be 49.12% ± 8.63% when HepG2 cells were treated with LPS, whereas no significant change was observed on treatment with CUR-NC 4 and Chi-CUR-NC 4b, as the expression was 53.11% ± 10.74% and 51.85% ± 6.16%, respectively. NFκB levels were increased in HepG2 on treatment with LPS (284.79% ± 12.69%) as compared to the untreated cells (100%), whereas on treatment with CUR-NC 4 and Chi-CUR-NC 4b, the expression was reduced to 87.18% ± 6.34% and 91.74% ± 12.31%, respectively.
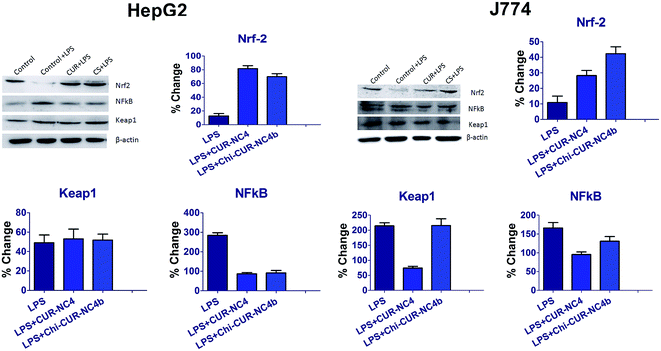 |
| Fig. 6 Western blot analysis of various markers involved in pathogenesis of LPS-induced sepsis. | |
In the case of J774 macrophages, the expression of Nrf2 was found to be 10.89% ± 4.11% when treated with LPS, taking untreated cells as 100%. On treatment with CUR-NC 4 and Chi-CUR-NC 4b along with LPS, the expression of Nrf2 was found to be 28.27% ± 3.28% and 42.41% ± 4.42%, respectively, which was significantly higher compared to the LPS-treated cells. The expression of NFκB was 165.86% ± 14.66% when J774 macrophages were treated with LPS; however, treatment with CUR-NC 4 and Chi-CUR-NC 4b along with LPS led to reduction in the levels of NFκB proteins up to a significant level, i.e. 95.54% ± 6.96% and 131.12% ± 12.31%, respectively, compared to the LPS-treated cells (p < 0.05). Keap1 expression was enhanced in LPS-treated J774 macrophages (214.67% ± 10.148%) compared with the untreated cells (100%). The treatment of LPS-stimulated J774 macrophage cells with CUR-NC 4 resulted in the significant reduction of Keap1 levels (74.39% ± 5.74%), whereas on treatment with Chi-CUR-NC 4b, Keap1 protein expression was unaltered (215.83% ± 22.16%).
2.8 Pharmacokinetic studies
Developed CUR-NCs were evaluated for change in pharmacokinetics as compared to CUR solution via i.v. administration. Plasma concentration vs. time data was analyzed by fitting to a non-compartmental model, and different pharmacokinetic descriptors were calculated using the WinNonlin software, 5.1 trial version (Table 4).
Table 4 Pharmacokinetic parameters of curcumin when administered as free drug and as nanocrystals to BALB/c mice (n = 3)
PK parameter |
Units |
CUR solution |
CUR-NC 4 |
Chi-CUR-NC 4b |
P value |
AUC0–12 h |
h ng ml−1 |
226.81 ± 43.82 |
1378.73 ± 138 |
3616.15 ± 246.48 |
<0.0001 |
Tmax |
H |
0.083 |
0.33 |
2 |
0.0002 |
Cmax |
ng ml−1 |
201.41 ± 32.48 |
443.08 ± 46.23 |
855.93 ± 42.82 |
0.0025 |
Clobserved |
ml h−1 kg−1 |
27 963.85 ± 424.62 |
5170.63 ± 148.48 |
2537.97 ± 204.24 |
0.0011 |
MRTlast |
h |
1.38 ± 0.08 |
4.17 ± 0.012 |
3.07 ± 0.064 |
0.0003 |
The pharmacokinetic parameters, i.e. AUC0–12 h, Tmax, Cmax, MRT, were increased by formulating CUR into NCs, whereas clearance (CL) and Vd were reduced in comparison to CUR solution when administered through i.v. route (Table 4). There was a 6.1-fold enhancement in AUC0–12 h in the case of CUR-NC 4 as compared to the CUR solution; in the case of Chi-CUR-NC 4b, a 16-fold enhancement in AUC0–12 h was observed (Table 4). When both the coated and non-coated formulations, i.e. CUR-NC 4 and Chi-CUR-NC 4b, were compared with each other, a 2.62-fold enhancement in AUC0–12 h was observed. Tmax also changed from 0.083 h or 5 min in the case of CUR solution to 20 min and 2 h in cases of CUR-NC 4 and Chi-CUR-NC 4b, respectively. Mean residence time (MRT) in the case of NCs (i.e. CUR-NC 4 and Chi-CUR-NC 4b) was increased (4.17 ± 0.012 and 3.07 ± 0.064 h, respectively) as compared to CUR solution (1.38 ± 0.08 h).
Tissue distribution data (Fig. 7) demonstrates that CUR-NCs improved the tissue distribution of CUR in lung, liver and spleen in significant proportions as compared to CUR solution (p < 0.05).
 |
| Fig. 7 Plasma and tissue concentration time profile of curcumin in different formulations administered (i.v.) to BALB/c mice at dose of 10 mg kg−1 body weight curcumin solution (CUR solution) and NCs (CUR-NC 4 and Chi-CUR-NC 4b). | |
2.9 In vivo efficacy studies
2.9.1 CUR nanocrystals are effective in reducing TNF-alpha, carbonyl protein and lipid peroxides in plasma. The increase in oxidative parameters is a very important outcome in the pathophysiology of sepsis, ultimately leading to oxidative tissue damage and precipitation of organ failure. Lipid peroxides7 and protein carbonyl groups8–10 are important biomarkers in patients with LPS-induced sepsis in a clinical setting.11,12 After 6 h of LPS injection in mice, elevated levels of carbonyl proteins and lipid peroxides were observed in plasma, which reduced on treatment with CUR solution or CUR nanocrystals (Fig. 8a). Chi-CUR-NC 4b was found to be more effective than CUR-NC 4 and CUR solution in reducing lipid peroxides as well as carbonylated proteins after 6 h of LPS administration.
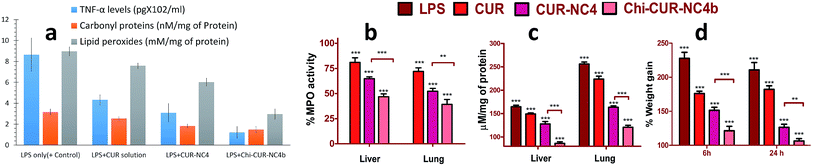 |
| Fig. 8 (a) Levels of TNF-alpha and markers of oxidative stress (i.e. carbonyl proteins and lipid peroxides) in mice plasma 6 h after LPS administration and treatment, (b) myeloperoxidase, (c) lipid peroxides in lung and liver in murine model of LPS induced endotoxemia after treatment with plain curcumin and nanocrystals (i.e. CUR-NC 4 and Chi-CUR-NC 4b). (d) Lung edema expressed as % weight gain in murine model of LPS induced endotoxemia after treatment with CUR nanocrystals. | |
Lipopolysaccharide (LPS) administration in mice also resulted in an increase of TNF-α levels (864.42 ± 158.27 pg ml−1) over the non-treated group at 6 h due to the sensitization of TLR-4 receptors in macrophages, leading to cytokine storm, whereas in the case of treatment groups, a significant reduction in TNF-α demonstrated the usefulness of the formulation in reducing cytokine storm in the early phases of sepsis. The level of TNF-α in the case of CUR solution, CUR-NC 4 and Chi-CUR-NC 4b was found to be 432.22 ± 48.46, 308.42 ± 88.36 and 120.86 ± 56.36 pg ml−1, respectively. Neutrophils are mainly involved in tissue injury mediated by the oxidative stress generated. In the case of Gram negative sepsis, myeloperoxidase (MPO) activity is frequently employed as a sensitive, quantitative measure of neutrophil sequestration to quantitate inflammation in tissues.13,14 As shown in Fig. 8b, we observed reduction in MPO levels, which clearly indicates significant reduction (p < 0.05) of neutrophil sequestration in the tissue of LPS-induced endotoxemia in the murine model, when treated with CUR solution or CUR nanocrystals.
CUR was found to be beneficial in decreasing the levels of lipid peroxides in liver up to significant levels in the case of both the plain drug as well as when treated with CUR nanocrystals as compared to the control group (LPS only) (p < 0.05) (Fig. 8c). The levels of lipid peroxides were reduced up to a significant level (p < 0.001) after 6 h of treatment with NCs (CUR-NC 4 and Chi-CUR-NC 4b) in the liver.
2.9.2 Curcumin nanocrystals lead to reduction in pulmonary edema, neutrophil migration and lipid peroxidation in lungs. We observed a significant fall in MPO levels, indicating reduction in neutrophil sequestration in tissues in the case of curcumin treated groups (CUR solution, CUR-NC 4 and ChiCURNC 4b) compared to the untreated group (LPS alone) as reported previously.15 When both the treatment groups were compared at 6 h, noteworthy reductions in MPO levels were observed in lungs when treated with CUR-NC 4 and Chi-CUR-NC 4 as compared to those treated with CUR solution (P < 0.05) (Fig. 9a and b). Similarly, in the case of lung tissues, levels of both lipid peroxides were reduced up to significant levels (p < 0.001) after 6 h of treatment (Fig. 8b and c).
 |
| Fig. 9 (a) mRNA expression of genes of enzymes involved in redox balance after 6 h of treatment with LPS, LPS + CUR-NC 4, LPS + Chi-CUR-NC 4b in liver tissues of BALB/c mice. (b) Effect of treatment with nanocrystals on mortality in murine model of LPS induced endotoxemia. | |
Acute lung injury (ALI) is a medical condition coupled with pulmonary malfunction frequently encountered as a complication of sepsis with ∼50% mortality.16 Pulmonary edema is a feature of ALI, and frequently evaluated parameter of edema is the amount of water in the lungs.17 Fig. 8d shows the wet-dry weight ratio (not corrected for residual blood) for the control (LPS-treated as +ve control), CUR solution treated, and formulation (CUR-NC 4 and Chi-CUR-NC 4b) treated animals at 6 and 24 h after endotoxin administration. The resolution of lung edema was demonstrated by reduction in wet-dry lung weight ratio in the case of treated groups even after 48 h of LPS administration.
2.9.3 Effect of CUR-NCs on the expression of glutathione peroxidase, glutathione-S-transferase and superoxide dismutase in the liver of LPS treated BALB/c mice by real time quantitative PCR. Estimation of mRNA expression by real time quantitative PCR of GPX, GST and SOD after 6 h of the administration of LPS and treatment with CUR-NC 4 and Chi-CUR-NC 4b demonstrated a significant change (p < 0.05) as compared to the group only treated with LPS in the liver (Fig. 9a).mRNA expression in the case of GPX was elevated to maximal level by LPS + Chi-CUR-NC 4b in the liver, whereas LPS + CUR-NC 4 was able to boost the expression of GPX in the liver when compared with the tissues of LPS-treated groups.18 GST mRNA expression was up-regulated in all the treatment groups, as well as in the LPS treated group only after 6 h of treatment. mRNA expression for SOD was increased for all the treatment groups as compared to the untreated control group, but the highest level of expression was demonstrated in groups treated with LPS + Chi-CUR-NC 4b.
2.9.4 Treatment with CUR NCs enhances survival in the murine model of LPS-induced endotoxemia. Survival studies clearly demonstrated improved survival in cases of treatment with Chi-CUR-NC 4b (80.00%), CUR-NC 4 (51.95%) and CUR solution (34.61%) as well as compared to the LPS-only group (13.34%) (Fig. 9b). Comparison of survival curves was done by a log rank (Mantel Cox) test with the survival significantly different at 95% confidence interval (using Graphpad Prism software).
3 Discussions
Sepsis is a multifaceted disorder having a plethora of outcomes that involve numerous molecular pathways. Oxidative stress and cytokine storm usually play a very important role in sepsis. CUR has multiple molecular targets that play a vital role in the pathophysiology of sepsis. Therefore, CUR can emerge as a potential candidate for the therapeutic intervention of sepsis. Nevertheless, bioavailability and stability issues hinder its development as an oral therapeutic agent. Moreover, in the case of sepsis, the parenteral route is usually preferred for a prompt therapeutic outcome.
CUR NCs were developed using Tween 80 and PF-68 as stabilizers. It was observed that PF-68 was a better stabilizer and resulted in a lower size of nanocrystal than Tween 80. The increase in crystal size in the case of Tween 80-stabilized batches may be attributed to the attainment of higher supersaturated concentrations in the continuous phase inside the chamber (due to an increase in temperature inside the interaction chamber), leading to crystal growth when the nanosuspension was leaving the interaction chamber.5 Masking of surface charge by PF-68 was apparent in the developed formulations when compared with Tween 80; this can be attributed to the shifting of the shear plane to a greater distance as described previously.19 The coating of nanocrystals with chitosan resulted in reversal of surface charge from negative to positive as evident from zeta potential measurements. AFM and SEM studies revealed that the structure of the optimized nanocrystals possess a plate-like morphology; crystallinity of CUR was further confirmed by DSC studies. Stability studies indicated that Chi-CUR-NC 4b and CUR-NC 4 were stable up to 3 months, whereas the formulation stabilized with Tween 80 (CUR-NC 2) was found to be unstable, as indicated by an increase in particle size and zeta potential.
Chitosan served as an endotoxin neutralizer, indicating the significance of Chi-CUR-NC 4b in the therapeutic intervention of septic shock, as proven by gel interaction studies as well as zeta potential measurements, as reported previously.20,21 Chitosan also improved the uptake of Chi-CUR-NC 4b mainly due to charge–charge interaction between the positive surface of the NCs and negative cell surface apart from that of the carbohydrate recognition mechanism,22 as reported previously in enhancing uptake of chitosan-coated nanocrystals through macropinocytosis. In vitro safety studies (i.e. hemolysis and cellular viability assays) demonstrated that the optimized nanocrystals (CUR-NC 4 and Chi-CUR-NC 4b) were safe for parenteral administration as compared to CUR solution.
Chi-CUR-NC 4b also performed better than plain CUR in reducing LPS-induced TNF-α and NO secretion from J774 macrophage cell lines. This observation can be attributed to the reduced degradation,5 enhanced uptake (evident from flow cytometry) and neutralization of LPS21 by coated nanocrystals. These studies clearly demonstrated the usefulness of Chi-CUR-NC 4b in reducing the LPS-induced TNF-α and nitric oxide levels. Reduction in TNF-α and NO secretion, as observed in the studies, can be attributed to the down-regulation of NFκB, which is the upstream regulator of TNF-α and NO; this was verified by decline in the protein level of NFκB at the cellular level, as observed by the western blot analysis on the J774 as well as HepG2 cell lines. Reduction in NFκB protein levels after 6 h of treatment clearly demonstrated that the reduction in TNF-α and NO levels were due to the inhibition of NFκB expression, as reported previously.23 The up-regulation of Nrf2 in both HepG2 and J774 cell-lines can be directly correlated with the improvement of oxidative status of the liver, which was demonstrated through in vivo studies on treatment with Chi-CUR-NC 4b. The molecular mechanism is postulated to involving the phosphorylation of ERK2 into phospho-ERK2, which in turn modulates Nrf2 in such a way that Nrf2 may not be able to remain bound with Keap1.24 It was observed that Keap1 levels remain unchanged, indicating that the increase in the protein level of Nrf2 was due to the phosphorylation of ERK2. However, the treatment with uncoated CUR-NC 4 had a different effect on the expression of Keap1 protein; in HepG2 cells, the Keap1 levels remained unchanged, whereas in the case of J774 cells, the protein levels of Keap1 reduced on treatment with CUR-NC 4, indicating the decreased degradation of Nrf2 in the case of treatment with plain nanocrystals; this suggested that the increase in the level of Nrf2 was due to reduced degradation25 as well as increased expression of NFκB.
Plasma pharmacokinetics was improved significantly in the case of CUR nanocrystals (i.e. AUC0–12 h 6.1 and 16 times for CUR-NC 4 and Chi-CUR-NC 4b, respectively, as compared to plain CUR solution). CUR nanocrystals also resulted in higher tissue distribution of CUR in organs, specifically lung, liver and spleen, due to preferential uptake by the MPS system, indicating the passive targeting of CUR by nanocrystals. Altogether, the increase in the plasma concentration and improved tissue distribution may be attributed to a reduction in metabolism and excretion of CUR as compared to plain drug.
In vivo pharmacodynamic studies demonstrated reduction in the levels of markers of oxidative stress (i.e. lipid peroxides and carbonyl proteins) as well as TNF-α in plasma after 6 h post-treatment with CUR nanocrystals (i.e. CUR-NC 4 and Chi-CUR-NC 4b); this activity can be attributed to a combined effect of LPS neutralization by chitosan, as reported previously,21 and anti-oxidant and anti-inflammatory effects of CUR.26 CUR has been reported to reduce TNF-α secretion via the down-regulation of the NFκB and PPAR-γ pathway,27 whereas chitosan can directly bind with LPS to reduce interaction with TLR-4 receptors, which is in accordance with previously published reports.21,28
Chi-CUR-NC 4b also resulted in the reduction of oxidative stress as well as neutrophil migration, as indicated by the levels of myeloperoxidase in tissues, specifically the lung and liver. Tissue injury is a most important hallmark in the pathophysiology of sepsis that usually worsens to organ failure and death. Chi-CUR-NC 4b was effective in reducing tissue damage, as indicated by lipid peroxides; this may be attributed to reduced neutrophil migration in tissues as directed by the decline in myeloperoxidase levels in lungs and liver, which is in agreement with previous studies.29 Reduction in acute lung injury was also exhibited by the reduction of lung edema in addition to reduction in lipid peroxides and myeloperoxidase levels in the lungs.
The improvement of oxidative status in the liver was further confirmed by monitoring the expression of the enzymes that regulate redox balance, i.e. GPX (glutathione peroxidase), GST (glutathione-S-transferase) and SOD (superoxide dismutase), which play a very vital role in the pathophysiology of LPS-induced organ damage in sepsis.4 Glutathione peroxidases (GPX) are major cellular antioxidative enzymes that protect from oxidative damage and cytotoxicity caused by hydroperoxides (H2O2 and lipid hydroperoxides). SOD catalyzes the conversion of superoxide into oxygen and H2O2, and then into water and oxygen with the help of GPX; therefore, both SOD and GPX constitute a major enzyme system for the reduction of oxidative stress due to LPS administration in mice. GPX deficiency also up-regulates CD14 expression and enhances endothelial responses to LPS, leading to an enhanced expression of accompanying inflammatory phenotypes and adhesion receptors in endothelium.30 GPX has also been reported to protect the injury in LPS-sensitized mice by inhibiting ROS generation due to neutrophil sequestration in liver tissue.31 The induction of GST mRNA expression by CUR is clearly demonstrated in the present study and is in accordance with previous studies32,33 indicating increased metabolism of CUR, but Chi-CUR-NC 4b treatment led to less GST mRNA expression as compared to CUR-NC 4.34 Altogether, there was an improvement in oxidative status (reduction of lipid peroxides and carbonylated proteins) mainly due to increase in the levels of GPX and SOD as well as a reduction in neutrophil migration (reduced myeloperoxidase), leading to decreased injury in the liver of endotoxemic mice.
CUR has been previously reported to have favorable effects on LPS-induced toxicity by reducing cytokines (TNF-α), NO secretion and oxidative damage by inhibiting LPS-induced NFκB over-expression and Nrf2 down-regulation by LPS in various cell-lines.35–37 The efficacy of Chi-CUR-NC 4b in reducing TNF-α and NO secretion was significantly better than plain CUR due to the enhanced uptake of nanocrystals (Chi-CUR-NC 4b) in J774 macrophages and the presence of chitosan as coating on the nanocrystals.
Reduction in mortality in murine models of sepsis by CUR (as a prophylactic and/or therapeutic agent) via oral/i.v./i.p. has been widely reported.26 Low bioavailability and rapid metabolism are major impediments to the successful translation of the therapy from experimental to clinical settings, mainly due to the need of higher doses of CUR (i.e. up to 500 mg kg−1 orally in humans), leading to an emergence of GI-related side effects.5 CUR nanocrystals conferred a better protection from tissue damage and failure, as evidenced by reduced mortality in the murine model of LPS-induced sepsis via improving the tissue distribution and pharmacokinetics of CUR (CUR-NC 4 and Chi-CUR-NC 4b) and also due to the presence of chitosan in the case of Chi-CUR-NC 4b by reducing TNF-α levels, neutrophil accumulation and tissue injury (as a result of oxidative stress)38,39 in oxidative damage prone organs such as the lungs and liver. Data in the present study clearly demonstrate reduction in the levels of inflammatory mediators in J774 macrophages (TNF-alpha & NO levels), neutrophil migration and lung edema in mice with septic shock on treatment with CUR nanocrystals. Observed reduction in damage in liver by oxidative stress was mediated by induction of GPX and SOD. Defense mechanisms such as ROS production by activated phagocytes are a vital part of the immune system, but excessive ROS can cause tissue damage and can be destructive leading to death. Tissue oxidative stress was reduced, as demonstrated by a decrease in lipid peroxide levels as well as carbonylated proteins;39 the observation was in accordance with the previous reports.38,40 Protein carbonylation is a vital marker of oxidative stress in the early phase of sepsis in clinical settings.12 Reduction in total oxidative stress (demonstrated by increase in lipid peroxide and carbonylated protein levels in liver and lungs) was maximized by the use of nanocrystals as a drug carrier and may be attributed to the improvement of tissue concentration of CUR and the LPS-neutralizing effect of chitosan. The protective effect on liver injury, as indicated by reduction in myeloperoxidase and lipid peroxides, can be attributed as an outcome of PPAR-γ activation,27 leading to the inhibition of the initiation of gene transcription by NF-κB as well as to the up-regulation of Nrf2 as demonstrated in HepG2 as well as J774 cell lines.
NF-κB pathway down-regulation by Chi-CUR-NC 4b at various levels leads to the amelioration of sepsis-associated inflammation and tissue damage at many stages, i.e. by reduction in the release of detrimental agents in the interstitium via reduction in the excitation and interaction of leukocytes and endothelial cells and leukocyte transmigration. It also inhibited the migration of neutrophils across the endothelium and the release of myeloperoxidase and hastened apoptosis of neutrophils, leading to a reduction in lung and liver tissue injury. Moreover, Chi-CUR-NC 4b up-regulated the Nrf2 pathway, leading to the expression of antioxidant enzymes (i.e. GPX and SOD) and protection of organs from oxidative damage. Other mechanisms that play a role in guarding of mice against LPS-induced sepsis can be predicted because CUR controls the activity of a number of decisive transcription factors such as STAT3 and PPAR-γ, in addition to Nrf2 and NF-κB therefore effecting cell proliferation, apoptosis and anti-oxidation. These mechanisms may also lead to the reduction in mortality due to LPS-induced septic shock.
4 Materials and method
4.1 Materials
CUR (95% purity) was provided as a gift sample from Sanat Products Ltd, (New Delhi, India). 3-[4,5-Dimethylthiazolyl]-2,5-diphenyltetrazolium bromide (MTT reagent), Tween 80, Pluronic F68 (PF-68), chitosan (85% deacetylation), cell culture media and other routine chemicals were purchased from Sigma Chemicals (St. Louis, MO). All other chemicals and solvents were of analytical or pharmaceutical grade.
4.2 Preparation and optimization of plain and chitosan coated NCs
CUR NCs were prepared by high pressure homogenization using a Microfluidizer (MP-110). Briefly, CUR was dispersed in 50 ml of aqueous solutions having different stabilizers (Tween 80 and PF-68) at different concentrations, as shown in Table 1. High pressure homogenization was carried out at 1500 bar (cooled with a heat exchanger set at 5 ± 2 °C) for 20 homogenization cycles, after pre-milling (10
000 rpm for 5 minutes) the dispersion with an Ultra Turrax (IKA).
The samples were analyzed for changes in size and zeta potential after pre-milling and then after 5, 10, 15, and 20 homogenization cycles. The formulation was optimized by varying the type (Tween 80 or PF-68) and concentration (at 1 and 2% w/v) of the stabilizers; number of homogenization cycles (pre-milling, 5, 10, 15 and 20 cycles) and their outcomes were evaluated in terms of NC size, PDI, and zeta potential.
The fabricated nanocrystals were then coated with chitosan via electrostatic interactions by incubating with chitosan solution at different concentration levels (i.e. at 0.1 and 0.01% w/v of chitosan). During the coating procedure, the average size and zeta potential were investigated and optimization was carried out on the basis of minimal increase in size after coating and reversal of surface charge.
4.3 Characterization of NC
4.3.1 Particle size and zeta potential and DSC studies. The NCs were evaluated for average particle size, distribution and zeta potential after suitable dilution using a Zetasizer Nano-ZS (Malvern Instruments, UK) as described previously.41
4.3.2 Total drug content in nanocrystal suspension. Drug content in NCs was determined to check the loss of drug during homogenization. The drug in the nanosuspension was extracted by diluting an appropriate amount of nanosuspension in HPLC grade methanol and analyzing by HPLC as described previously.26
4.3.3 In vitro interaction of LPS with cationic NC. Zeta-potential distribution assay and gel electrophoresis were employed to study interaction between LPS (lipopolysaccharide from E. coli 055 B5) and the optimized batch of Chi-CUR-NC 4b nanocrystals. Electrokinetic potential of the LPS micelles and LPS–cationic CUR-NC complexes were evaluated by a Zetasizer, Nano ZS (Malvern, UK). Prior to measurement, the samples were allowed to stand for 1 h at 25 °C for incubation.21 Briefly, LPS (1 mg ml−1) and nanocrystals (containing 2 mg ml−1 of CUR) were incubated with different ratios (2
:
1, 4
:
1, 6
:
1 and 8
:
1 v/v) of Chi-CUR-NC 4b to LPS. These findings were further confirmed by employing gel electrophoresis as reported previously with slight modifications.42 Briefly, 0.5% agarose gel plates (4–5 mm in thickness) in 0.04 M barium acetate buffer, pH 5.8 were casted. Ten μl of plain LPS (1 mg ml−1) as well as LPS incubated with different ratios of Chi-CUR-NC 4b nanocrystals, as described in electrokinetic measurements, were layered by micropipettes. The run was performed in 0.05 M 1,2-diaminopropane (buffered at pH 9.0 with acetic acid) for 150 min at 50 mA. After the completion of run gel was kept for 15 min in a solution of 0.2% cetylpyridinium chloride. After drying, the plate was stained with freshly prepared toluidine blue (0.2% in ethanol–water–acetic acid 50
:
49
:
1) for 30 min, and de-stained with ethanol–water–acetic acid 50
:
49
:
1, followed by the Stains-All staining procedure (25 mg in 500 ml ethanol–water 50
:
50 overnight in the dark and de-staining with water).43
4.4 Cellular uptake and in vitro cytotoxicity of NCs
4.4.1 In vitro cytotoxicity of NCs. For the evaluation of in vitro cytotoxicity, cells (J774 macrophages, HepG2 and HEK) were seeded at a density of 1 × 103 cells per well in a 96-well cell culture plate and allowed to grow for 24 h. The formulations diluted appropriately with PBS were added into the individual wells. Blank PBS was taken as untreated control and in vitro cytotoxicity was evaluated as reported previously.5 Cytotoxicity was calculated according to the following equation.
Viability(%) = (Abs treatment/Abs control) × 100 |
4.4.2 Uptake studies using flow cytometry. To assess cellular uptake, flow cytometry was performed at 4 h post-treatment with the NCs in J774 (1 × 105 cells per chamber) in six-well plates using a FACS Calibur Cell Quest (BD Biosciences San Jose, CA). At the end of incubation, cells were trypsinized, washed with physiological saline three times and then analyzed by fluorescence-activated cell sorting (FACS) analysis in accordance with the fluorescence exhibited by CUR at λex = 420 nm and λem = 470 nm. Similarly, a blank formulation was also compared with CUR containing NCs. Flow cytometry results were analyzed using Flow Jo software.
4.4.3 In vitro hemolytic assay. Formulations equivalent to 4 and 8 mg l−1 of CUR in each well were added to the suspension of RBCs. PBS and triple distilled water were taken as negative and positive control, respectively (n = 3). The percent hemolysis was evaluated as described elsewhere.5Percentage hemolysis is calculated from the formula
%Hemolysis = [Abstreatment − Abs(−ve control) × 100]/Abs(+ve control) |
4.5 Effect of nanocrystals on the release of NO and TNF-α from J774 cell line
To demonstrate the effect of formulations on the stimulation of NO and cytokine release, a 48-well culture plate was seeded with J774 macrophage and after 24 h (at 37 °C) cells were incubated with LPS alone and formulations at different dose levels in the presence of LPS (500 ng ml−1) for 18 h. TNF-α, IL-6 and NO in cell supernatant of plain CUR and NC treated groups were determined using murine specific sandwich enzyme-linked immunosorbent assays (ELISAs) (R&D system, USA) and Griess reagent,21 respectively, according to standard procedures as per the manufacturer's instructions.
4.6 Western blot analysis in J774 and HepG2 cell lines
Proteins were isolated from cells (J774 and HepG2 cell lines) using a modified protocol of Ghribi et al. (2001). Cells were sonicated with 5–10 volumes of lysis buffer (200 mM HEPES, 10 mM KCl, 1.5 mM MgCl2, 1 mM EDTA, 1 mM EGTA, 1 mM DTT, 2 mM PMSF, 1× protease inhibitor cocktail). Cellular debris were spun down at 20
000×g for 30 min at 4 °C and supernatants were used as whole protein extract. Isolated proteins were quantified using Bradford reagent. 70 μg protein from each sample was separated on 15% SDS-PAGE and transferred onto a nitrocellulose membrane using a semi-dry electroblotting apparatus (GE Healthcare, UK). Transfer was examined by Ponceau S stain and washed with TDW until the stain disappeared. The membrane was blocked overnight in 5% non-fat dried milk at 4 °C. The blocking membrane was washed with 0.1% PBST and probed with 1° antibodies. After 1° antibody incubation, further washing was done in 0.1% PBST. The membrane was incubated in HRP-conjugated 2° antibody and washed again. Enhanced chemiluminescent detection reagent was used to develop the blots. Blots were further used for densitometric analysis (ImageJ) and normalization.44 Experiments were carried out in triplicate and values were averaged.
4.7 In vivo evaluations
4.7.1 Pharmacokinetic studies. The investigational procedure was approved by the IAEC (Central Drug Research Institute, Lucknow; study number IAEC 2012/38). Overnight fasted BALB/c mice (20 to 30 g) were used in the pharmacokinetic studies and were maintained on a standard animal diet at 25 °C ± 2 °C and 30% to 70% relative humidity. Mice (n = 3 for each time point) were randomized based on their body weights and distributed equally into two groups. Each group of mice (n = 36) received CUR solution and NCs (plain as well as Chi-CUR-NC 4b NCs) administered by intravenous (i.v.) route at the dose equivalent to 10 mg kg−1 of CUR. Following administration, approximately 1 ml of blood samples were collected from the retro-orbital plexus in pre-labeled Eppendorf tubes after anesthetizing with ketamine from a group of 3 animals per time point from the respective group at the time intervals of 0.25, 0.5, 1, 2, 4, 8, 12 h post-dosing. The samples were analyzed as described previously.26
4.7.2 Pharmacodynamic studies. Sepsis was prompted by i.p. injection of E. coli LPS in saline (serotype O55: B5; Sigma-Aldrich, St. Louis, MO). Before commencing studies, mice were weighed, and randomized in a blinded fashion to receive CUR solution, plain and Chi-CUR-NC 4b NCs equivalent to 20 mg kg−1 of CUR and normal saline via i.v. route (n = 6). Thirty minutes after the administration of treatment, 10 mg kg−1 of LPS was administered through the i.p. route. Tissue samples and plasma was collected at different time points by sacrificing animals and storing at −80 °C for further analysis.
4.7.2.1 TNF-alpha estimation in plasma. Plasma TNF-α levels were determined by enzyme-linked immunosorbent assay (ELISA) as described above using commercial kits that are selective to rat cytokines (R&D system, USA). Cytokine concentrations in plasma have been expressed as pg ml−1.
4.7.2.2 Determination of lung edema. Death in case of sepsis is often caused after multiple organ failure and the lung is amongst the first organ to collapse in sepsis after acute respiratory distress syndrome (ARDS). Lung edema is a very relevant outcome that can be related experimentally to the severity of sepsis and death. Lungs were excised from mice and evaluated for lung edema as described in a previous study.5 The wet tissue weight was divided by the dried tissue weight to obtain the wet-dry lung mass ratio, which is the fraction of the wet lung weight that was water.45
4.7.2.3 Leukocyte myeloperoxidase (MPO) assay for determining neutrophil migration to tissues. Neutrophils are the initial cells stimulated in case of host immune reaction to pathogenic assault or damage and are decisive cellular effectors in both humoral and innate immunity, which are fundamental to the pathogenesis of sepsis and multiple organ injury. Myeloperoxidase activity is widely employed to assess neutrophil accumulation and often correlates with tissue injury.46 To assess tissue injury, MPO assay was performed as described in a previous study.47
4.7.2.4 Measurement of lipid peroxides and carbonylated proteins in tissues. Free radical mediated lipid peroxidation and protein carbonylation are indicators of oxidative burden in sepsis5 and can be employed as marker for tissue damage in sepsis.7 Protein carbonyl measurements27 provide a sensitive index to oxidative damage occurring at the initial stages of sepsis.11,12 Lipid peroxidation level was estimated using methods described elsewhere.5Plasma protein oxidation was evaluated by the estimation of carbonyl groups based on the reaction with DNPH, as described in a previous study.48
4.7.2.5 RT-PCR to monitor the expression of glutathione-S-transferase, superoxide dismutase, and glutathione peroxidase in liver.
4.7.2.5.1 RNA isolation from tissues.
50 mg frozen tissue (liver) was crushed manually in liquid nitrogen, immediately homogenized (Lab GEN7, Cole-Parmer) and extracted using TRIzol reagent (Quiagen) as per manufacturer's instructions. Finally, the RNA pellet was air dried and re-suspended in nuclease-free water (Sigma). RNA concentration and purity were determined at an optical density ratio of 260/280 and 260/230 using a UV-Vis spectrophotometer (Q5000, Quawell, USA).
4.7.2.5.2 cDNA synthesis and qRT-PCR analysis.
Five μg of total RNA was used for cDNA synthesis, which was performed using a cDNA synthesis kit (High Capacity cDNA Reverse Transcription Kit, Applied Biosystems, USA) according to manufacturer's protocol. The program for cDNA synthesis included incubation steps at 25 °C for 10 min, 37 °C for 120 min, 85 °C 5 min and 4 °C till use. Briefly, 50 ng of reverse-transcripted RNA from each sample were mixed with appropriate concentrations of tested gene primers, i.e. glutathione peroxidase (GPX) 5′ ATGTCGCGTCTCTCTGAGG 3′, 3′ CCGAA-CTGATTGCACGGG 5′; glutathione-S-transferase (GST) 5′ TTTTTGCGCGGTCCTTTCCTG 3′, 3′ GGTTCACC-GCTTGCCTTCTG 5′; Superoxide Dismutase (SOD) 5′ GCTGCGGCTGGAGTGGAGT 3′, 3′ GGATGGCCC-TGGTCTGTGTC 5′ and were examined using qRT-PCR on a LightCycler 480 PCR system (Roche Diagnostics, Germany). PCR amplifications were carried out as follows: 2 min at 50 °C (UDG incubation); 10 min at 95 °C; 45 cycles (15 s at 95 °C, 30 s at 58 °C and 20 s at 72 °C) using a LightCycler 480 SYBR Green I master (Roche Diagnostics, Germany); subsequently Cp values were averaged. For each primer pair, a melting curve analysis was performed according to the instrument. Differential expression was calculated by the 2−ΔΔCT method. β-actin was used as internal control and used to normalize ratios between the samples.49
4.8 Survival studies
Survival of mice (n = 20) was observed after i.p. administration of LPS and treatment comprising plain CUR solution and plain and coated CUR nanocrystals (containing CUR equivalent to 10 mg kg−1). The control group received isotonic sodium chloride solution through i.p. route twice a day (at 12 h intervals) for 7 days. Survival was monitored on a daily basis for 7 days after the inoculation of LPS (i.p.) and deaths were recorded as they occurred. Live animals were euthanized at the end of the investigational duration.
5. Conclusion
LPS-induced toxicity leads to hyper-inflammation, which is indicated by the over secretion of inflammatory mediators and oxidative damage of organs due to the reduced levels of antioxidant defenses and ultimately precipitates to septic shock and death. It is a major contributor to mortality in cases of complicated intra-abdominal infections. In the present study, cationic CUR NCs have been offered as an alternative parenteral therapy of LPS-induced endotoxemia, organ damage septic shock and death. Plain CUR NCs were successfully fabricated by high pressure homogenization employing PF-68 as a stabilizer and were coated with chitosan as a cationic polyelectrolyte. Plain as well as coated CUR NCs demonstrated suitability for parenteral administration with improved pharmacokinetics, tissue distribution and pharmacodynamics in mice models of LPS-induced sepsis. Chi-CUR-NC 4b NCs were demonstrated to cause reduction in the discharge of inflammatory mediators (TNF-α and NO), reduction in oxidative stress, neutrophil migration in both cell lines (J774 and HepG2) and in vivo models of LPS-induced septic shock (as indicated by reduced TNF-alpha, LPO and carbonylated protein levels in plasma as well as in lung and liver) and a reduction in lung edema. Chi-CUR-NC 4b offers a viable approach demonstrating dual modality: first, LPS neutralization (i.e. antiendotoxin strategy) by chitosan and has multifaceted effects of CUR (i.e. anti-inflammatory and antioxidant properties of CUR) in subsiding LPS-induced hyper-inflammation, oxidative injury and death in case of mice model of sepsis via NFκB down regulation (leading to reduced cytokine and NO secretion), Nrf2 upregulation (leading to improved levels of antioxidant enzymes, most notably GPX and SOD). In conclusion, the developed formulation has a potential to be evaluated as a viable approach for the treatment of LPS-induced sepsis and septic shock in a clinical setting.
Conflict of interest statement
The authors declare they have no competing financial interest.
Acknowledgements
The author would like to thank CSIR New-Delhi, India, for providing SRF fellowship, CSIR-CDRI Lucknow, India, and to CSIR Network Project UNDO (BSC 0103) for providing financial assistance.
References
- R. Bosurgi, Lancet Infect. Dis., 2015, 15, 498–499 CrossRef
. - A. Duvoix, R. Blasius, S. Delhalle, M. Schnekenburger, F. Morceau, E. Henry, M. Dicato and M. Diederich, Cancer Lett., 2005, 223, 181–190 CrossRef CAS PubMed
. - V. P. Menon and A. R. Sudheer in The Molecular Targets and Therapeutic Uses of Curcumin in Health and Disease, Springer, 2007, pp. 105–125 Search PubMed
. - P. Shukla, G. M. Rao, G. Pandey, S. Sharma, N. Mittapelly, R. Shegokar and P. R. Mishra, Br. J. Pharmacol., 2014, 171, 5011–5031 CAS
. - P. Shukla, P. Dwivedi, P. K. Gupta and P. R. Mishra, Expert Opin. Drug Delivery, 2014, 11, 1697–1712 CrossRef CAS PubMed
. - A. Storka, B. Vcelar, U. Klickovic, G. Gouya, S. Weisshaar, S. Aschauer, L. Helson and M. Wolzt, Anticancer Res., 2013, 33, 3629–3634 CAS
. - P. Scheiermann and S. E. Pischke, Crit. Care, 2013, 17, 120 CrossRef PubMed
. - S. Abe, Y. Tanaka, N. Fujise, T. Nakamura, H. Masunaga, T. Nagasawa and M. Yagi, J. Parenter. Enteral Nutr., 2007, 31, 181–187 CrossRef CAS PubMed
. - S. Vittorini, C. Paradiso, A. Donati, G. Cavallini, M. Masini, Z. Gori, M. Pollera and E. Bergamini, J. Gerontol., Ser. A, 1999, 54, B318–B323 CrossRef CAS PubMed
. - A. M. Mohamadin, A. A. Elberry, M. A. Elkablawy, H. S. A. Gawad and F. A. Al-Abbasi, Pathophysiology, 2011, 18, 235–242 CrossRef CAS PubMed
. - I. Dalle-Donne, R. Rossi, D. Giustarini, A. Milzani and R. Colombo, Clin. Chim. Acta, 2003, 329, 23–38 CrossRef CAS
. - C. C. Winterbourn, I. H. Buss, T. P. Chan, L. D. Plank, M. A. Clark and J. A. Windsor, Crit. Care Med., 2000, 28, 143–149 CrossRef CAS PubMed
. - D. J. Wickel, W. G. Cheadle, M. A. Mercer-Jones and R. N. Garrison, Ann. Surg., 1997, 225, 744–753 CrossRef CAS PubMed
; discussion 753–746. - D. J. Hadjiminas, K. M. McMasters, J. C. Peyton, M. D. Cook and W. G. Cheadle, Shock, 1994, 2, 376–380 CrossRef CAS PubMed
. - V. Vachharajani, S. W. Wang, N. Mishra, M. El Gazzar, B. Yoza and C. McCall, Microcirculation, 2010, 17, 407–416 CAS
. - S. W. Chang, Semin. Respir. Crit. Care Med., 1994, 15, 463–472 CrossRef PubMed
. - S. Hagiwara, H. Koga, H. Iwasaka, J. Kusaka, T. Uchida, K. Goto, I. Yokoi and T. Noguchi, J. Surg. Res., 2011, 171, 734–741 CrossRef CAS PubMed
. - C. J. Harvey, R. K. Thimmulappa, A. Singh, D. J. Blake, G. Ling, N. Wakabayashi, J. Fujii, A. Myers and S. Biswal, Free Radical Biol. Med., 2009, 46, 443–453 CrossRef CAS PubMed
. - P. R. Mishra, L. A. Shaal, R. H. Müller and C. M. Keck, Int. J. Pharm., 2009, 371, 182–189 CrossRef CAS PubMed
. - I. M. Yermak, V. N. Davidova, V. I. Gorbach, P. A. Luk'yanov, T. F. Solov'eva, A. J. Ulmer, U. Buwitt-Beckmann, E. T. Rietschel and Y. S. Ovodov, Biochimie, 2006, 88, 23–30 CrossRef CAS PubMed
. - V. Jain, P. Shukla, R. Pal and P. R. Mishra, Pharmaceut. Res., 2014, 31, 2630–2642 CrossRef CAS PubMed
. - Y. Ikehara, T. Niwa, L. Biao, S. K. Ikehara, N. Ohashi, T. Kobayashi, Y. Shimizu, N. Kojima and H. Nakanishi, Cancer Res., 2006, 66, 8740–8748 CrossRef CAS PubMed
. - F. Zhong, H. Chen, L. Han, Y. Jin and W. Wang, Biol. Pharm. Bull., 2011, 34, 226–232 CAS
. - R. K. Thimmulappa, H. Lee, T. Rangasamy, S. P. Reddy, M. Yamamoto, T. W. Kensler and S. Biswal, J. Clin. Invest., 2006, 116, 984–995 CAS
. - C. Yang, X. Zhang, H. Fan and Y. Liu, Brain Res., 2009, 1282, 133–141 CrossRef CAS PubMed
. - P. Shukla, V. Mathur, A. Kumar, V. Khedgikar, B. V. Teja, D. Chaudhary, P. Kushwaha, H. K. Bora, R. Konwar, R. Trivedi and P. R. Mishra, J. Biomed. Nanotechnol., 2014, 10, 3381–3391 CrossRef CAS PubMed
. - A. M. Siddiqui, X. Cui, R. Wu, W. Dong, M. Zhou, M. Hu, H. H. Simms and P. Wang, Crit. Care Med., 2006, 34, 1874–1882 CrossRef CAS PubMed
. - I. M. Yermak, V. N. Davidova, V. I. Gorbach, P. A. Luk'yanov, T. F. Solov'eva, A. J. Ulmer, U. Buwitt-Beckmann, E. T. Rietschel and Y. S. Ovodov, Biochimie, 2006, 88, 23–30 CrossRef CAS PubMed
. - Q. S. Zang, H. Sadek, D. L. Maass, B. Martinez, L. Ma, J. A. Kilgore, N. S. Williams, D. E. Frantz, J. G. Wigginton, F. E. Nwariaku, S. E. Wolf and J. P. Minei, Am. J. Physiol.: Heart Circ. Physiol., 2012, 302, H1847–H1859 CrossRef CAS PubMed
. - E. Lubos, C. E. Mahoney, J. A. Leopold, Y. Y. Zhang, J. Loscalzo and D. E. Handy, FASEB J., 2010, 24, 2525–2532 CrossRef CAS PubMed
. - H. Jaeschke, Y.-S. Ho, M. A. Fisher, J. A. Lawson and A. Farhood, Hepatology, 1999, 29, 443–450 CrossRef CAS PubMed
. - R. Sharma, A. Gescher and W. Steward, Eur. J. Cancer, 2005, 41, 1955–1968 CrossRef CAS PubMed
. - T. Nishinaka, Y. Ichijo, M. Ito, M. Kimura, M. Katsuyama, K. Iwata, T. Miura, T. Terada and C. Yabe-Nishimura, Toxicol. Lett., 2007, 170, 238–247 CrossRef CAS PubMed
. - Y.-H. Shon, I.-K. Park, I.-S. Moon, H. W. Chang, I.-K. Park and K.-S. Nam, Biol. Pharm. Bull., 2002, 25, 1161–1164 CAS
. - K. K. Jung, H. S. Lee, J. Y. Cho, W. C. Shin, M. H. Rhee, T. G. Kim, J. H. Kang, S. H. Kim, S. Hong and S. Y. Kang, Life Sci., 2006, 79, 2022–2031 CrossRef CAS PubMed
. - G. Kang, P.-J. Kong, Y.-J. Yuh, S.-Y. Lim, S.-V. Yim, W. Chun and S.-S. Kim, J. Pharmacol. Sci., 2004, 94, 325–328 CrossRef CAS
. - M.-H. Pan, S.-Y. Lin-Shiau and J.-K. Lin, Biochem. Pharmacol., 2000, 60, 1665–1676 CrossRef CAS
. - G. Yilmaz Savcun, E. Ozkan, E. Dulundu, U. Topaloglu, A. O. Sehirli, O. E. Tok, F. Ercan and G. Sener, Turkish Journal of Trauma and Emergency Surgery, 2013, 19, 507–515 CrossRef PubMed
. - K. Sompamit, U. Kukongviriyapan, S. Nakmareong, P. Pannangpetch and V. Kukongviriyapan, Eur. J. Pharmacol., 2009, 616, 192–199 CrossRef CAS PubMed
. - F. Xu, S. H. Lin, Y. Z. Yang, R. Guo, J. Cao and Q. Liu, Int. Immunopharmacol., 2013, 16, 1–6 CrossRef CAS PubMed
. - S. Sharma, A. Verma, B. V. Teja, P. Shukla and P. R. Mishra, Eur. J. Pharm. Sci., 2015, 69, 51–60 CrossRef CAS PubMed
. - F. Maccari and N. Volpi, Anal. Biochem., 2003, 322, 185–189 CrossRef CAS PubMed
. - N. Volpi, F. Maccari and J. Titze, J. Chromatogr. B: Anal. Technol. Biomed. Life Sci., 2005, 820, 131–135 CrossRef CAS PubMed
. - P. Singh, S. K. Mishra, S. Noel, S. Sharma and S. K. Rath, PLoS One, 2012, 7, e31964 CAS
. - J. Yang, W. Li, M. Duan, Z. Zhou, N. Lin, Z. Wang, J. Sun and J. Xu, Inflammation Res., 2005, 54, 133–137 CrossRef CAS PubMed
. - M. Merza, E. Wetterholm, S. Zhang, S. Regner and H. Thorlacius, J. Leukocyte Biol., 2013, 94, 493–502 CrossRef CAS PubMed
. - P. Shukla, A. K. Verma, P. Dwivedi, A. Yadav, P. K. Gupta, S. K. Rath and P. R. Mishra, Mol. Pharm., 2014, 11, 4314–4326 CrossRef CAS PubMed
. - J. M. Fagan, B. G. Sleczka and I. Sohar, Int. J. Biochem. Cell Biol., 1999, 31, 751–757 CrossRef CAS
. - K. J. Livak and T. D. Schmittgen, Methods, 2001, 25, 402–408 CrossRef CAS PubMed
.
Footnote |
† This is CSIR-CDRI communication number 9014. |
|
This journal is © The Royal Society of Chemistry 2015 |
Click here to see how this site uses Cookies. View our privacy policy here.