DOI:
10.1039/C5RA05564D
(Paper)
RSC Adv., 2015,
5, 42978-42989
Isolation of first row transition metal-carboxylate zwitterions†
Received
29th March 2015
, Accepted 7th May 2015
First published on 7th May 2015
Abstract
Zwitterionic 3d metal carboxylates of Zn(II), Cu(II), Ni(II) and Co(II) have been isolated and structurally authenticated by X-ray crystallography. A series of 2-hydroxymethylpyridine-carboxylate ligands with different sizes and shapes demonstrate variable coordination modes with first row transition metals under different conditions, yielding a class of 17 complexes, predominantly zwitterions. The nature of the ligands permits the carboxylates to be un-coordinated, anionic and conjugated, thereby balancing the positive charges on the metal centers.
Introduction
Zwitterions, or inner salts, are neutral molecules with opposite charges spatially separated in the same molecule.1 Naturally occurring zwitterions are exemplified by the ubiquitous amino acids, NH3+RCHCO2−.2 Synthetic metal-centered zwitterions, with metal ions as the positive charge center have been reported for the second and third row transition metals.3 The polarity and solubility of these heavier metal centered zwitterions with borate, carbanion, sulfonate, sulfate and phosphate-based ligands are important for their roles in catalysis.3a The synthesis of first row transition metal based zwitterions, particularly metal-carboxylate zwitterions, is difficult because of the strong affinity of carboxylate anions for hard metal cations.4 This hard acid–base interaction has led to a plethora of discrete metal-carboxylate clusters and, in the past 15 years, innumerable metal–organic frameworks (MOFs) assembled from first row transition metal ions and carboxylate-based organic ligands.5
Herein we report the synthesis of first row transition metal-carboxylate zwitterions by employing functionalized 2-hydroxymethylpyridine carboxylic acid or carboxylate ester ligands.6 Examples of these ligands, ethyl 2-(hydroxymethyl)isonicotinate (iso-ehmnH) and ethyl 6-(hydroxymethyl)nicotinate (ehmnH) (Chart 1) were found to react with Zn(NO3)2·6H2O to provide Zn-carboxylate zwitterions trans-Zn(iso-hmnH)2(H2O)2 (1) and cis-Zn(hmnH)2(H2O)2·2H2O (2) (iso-hmnH = 2-(hydroxymethyl)isonicotinate; hmnH = 2-(hydroxymethyl)nicotinate) which are intermediates in the formation of MOFs.7 Complexes 1 and 2 are polar species that fluoresce blue and greenish-blue. To explore the diversity of these first row transition metal-carboxylate zwitterions and to better understand the relationship between the structure of the intermediates and the final MOFs, we have synthesized a series of hydroxymethylpyridine-carboxylic acids or hydroxymethylpyridine-carboxylate esters of different size and shape (Chart 1) as ligands for Mn(II), Co(II), Ni(II), Cu(II) and Zn(II) salts. We have been able to isolate an array of 17 molecular species, predominantly zwitterions. In doing so, we have filled the literature gap in first row transition metal centered zwitterions and have devised a general strategy for the synthesis of zwitterionic coordination complexes.
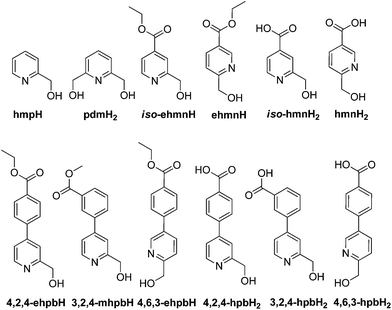 |
| Chart 1 | |
Results and discussion
Reactions of Zn(NO3)2·6H2O with iso-ehmnH or ehmnH at 95 °C in DMF/H2O (1
:
1, v/v) yielded colorless and greenish-orange solutions. Slow evaporation of these solutions at r.t. gave rise to zwitterionic complexes trans-[Zn(iso-hmnH)2(H2O)2] (1) and cis-[Zn(hmnH)2(H2O)2]·2H2O (2).7 Complex 1 and 2 are zwitterions with the positive charges from Zn2+ remotely neutralized by the two terminal carboxylates (Fig. 1). The octahedral Zn center is completed by a pair of chelating pyridine alcohol ligands and two coordinated water molecules. We propose zwitterions 1 and 2 are formed because hydroxymethylpyridine is a stronger chelator than the carboxylate under these conditions and results in overall charge neutrality. Notably, the isomeric ligands result in complexes of opposite stereochemistry, viz. trans in 1 and cis in 2 (Fig. 1). The study of the crystal packing of 1 and 2 also revealed extensive hydrogen bonding interactions between coordinated water and hydroxyl as donors and free carboxylates as acceptors (Fig. S4, ESI†). These hydrogen bondings may also play a role in directing the configuration of the ligands.
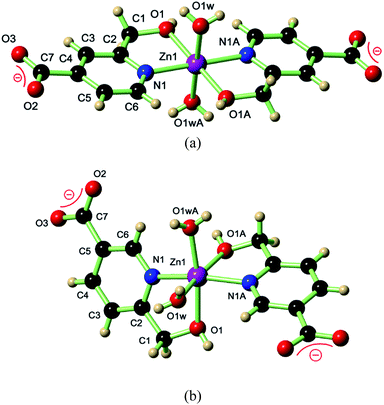 |
| Fig. 1 Structure of trans-[Zn(iso-hmnH)2(H2O)2] (1) (a) and cis-[Zn(hmnH)2(H2O)2]·2H2O (2) (b) with the dissociated H2O molecules in 2 omitted. Symmetry codes for (1): A −x, −y + 1, −z; and (2): A −x, y, 0.5 − z. Selected C–O bond distances: C7–O2 1.248(2) Å, C7–O3 1.249(2) Å (1); C7–O2 1.234(2) Å, C7–O3 1.264(2) Å (2). | |
Other metal salts MX2 (M = Co(II), Ni(II), Cu(II); X = NO3, Cl) with iso-ehmnH and ehmnH resulted in amorphous, powdery products under similar conditions as those for complexes 1 and 2. We therefore hydrolyzed iso-ehmnH and ehmnH to their respective acid form iso-hmnH2 and hmnH2 (Chart 1). Reactions of iso-hmnH2 and hmnH2 with Co(NO3)2·6H2O or Ni(NO3)2·6H2O in DMF/H2O/MeCN in the presence of trace amount of aqueous HNO3 provided diffraction quality crystals of zwitterionic complexes trans-Co(iso-hmnH)2(H2O)2 (3), trans-Ni(iso-hmnH)2(H2O)2 (4) and cis-[Ni(hmnH)2(H2O)2]·2H2O (5) (Fig. S1, ESI†). Reaction of the corresponding metal chlorides, MCl2 (M = Co, Ni, Zn) yielded the same zwitterionic complexes as determined by single-crystal X-ray diffraction.
The strong Jahn–Teller effect for Cu(II) (d9) makes the reaction more susceptible to reaction variables such as ligand and metal sources, counterions, solvents, temperatures etc. It was not surprising, therefore, that we obtained different complexes (6–11) from reactions involving Cu(II) and iso-hmnH2/hmnH2 under different reaction conditions (Scheme 1).
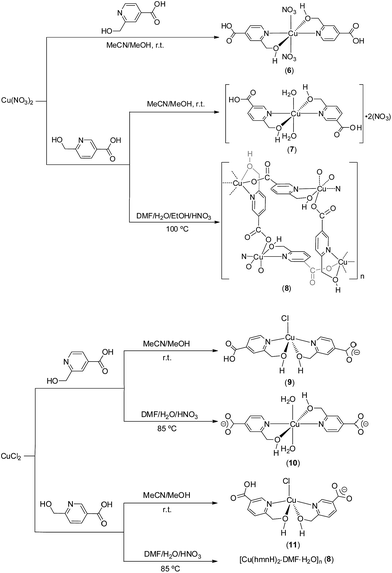 |
| Scheme 1 Schematic presentation of the synthesis of complexes 6–11. | |
Reaction of Cu(NO3)2·3H2O with iso-hmnH2 or hmnH2 in MeCN/MeOH at r.t. provided non-zwitterionic, octahedral trans-[Cu(iso-hmnH2)2(NO3)2]·2H2O (6) and trans-[Cu(hmnH2)2(H2O)2][NO3]2 (7) (Fig. 2). The ligands in both cases invariably prefer a trans-arrangement, and could accommodate different secondary coordination groups (NO3 anion in 6 and H2O in 7).
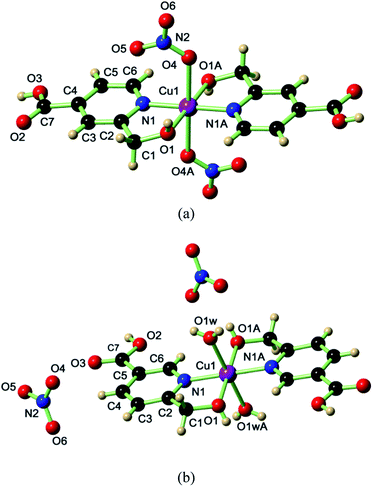 |
| Fig. 2 Structures of trans-[Cu(iso-hmnH2)2(NO3)2]·2H2O (6) (a) and trans-[Cu(hmnH2)2(H2O)2][NO3]2 (7) (b) with the dissociated H2O molecules in 6 omitted. Symmetry codes for (6): A −x, −y + 1, −z; and (7): A −x + 1, y +1, −z. Selected C–O bond distances: C7–O2 1.213(5) Å, C7–O3 1.332(5) Å (6); C7–O2 1.324(4) Å, C7– O3 1.203(3) Å (7). | |
When CuCl2·2H2O was used as the metal source, zwitterionic, trigonal bipyramidal complexes [CuCl(iso-hmnH2)(iso-hmnH)]·H2O (9) and CuCl(hmnH2)(hmnH) (11) were isolated in which the 2+ charge on Cu is balanced by one Cl and one carboxylate (Fig. 3a and c). The equatorial plane of the trigonal bipyramid is formed by the terminal Cl and two cis O atoms from the pyridine alcohol ligands while the apical positions are occupied by two trans located N atoms. By contrast, the reactions of CuCl2 with iso-hmnH2 in DMF/H2O or DMF/H2O/HNO3 under solvothermal conditions resulted in the isolation of zwitterion trans-Cu(iso-hmnH)2(H2O)2 (10) which is structurally analogous to complex 1 (Fig. 3b).
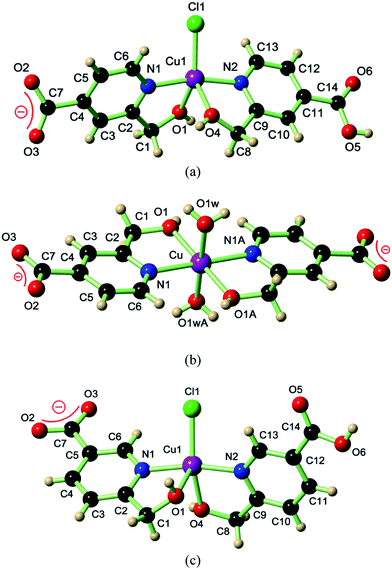 |
| Fig. 3 Structures of [CuCl(iso-hmnH2)(iso-hmnH)]·H2O (9) (a), trans-[Cu(iso-hmnH)2(H2O)2] (10) (b) and [CuCl(hmnH2)(hmnH)] (11) (c) with the dissociated H2O molecules in 9 omitted. Selected C–O bond distances: C7–O2 1.264(3) Å, C7–O3 1.237(3) Å, C14–O5 1.321(3) Å, C14–O6 1.206(3) Å (9); C7–O2 1.239(2) Å, C7–O3 1.260(2) Å (10); C7–O2 1.263(2) Å, C7–O3 1.241(2) Å, C14–O5 1.200(2) Å, C14–O6 1.315(2) Å (11). | |
The reaction of hmnH2 with either Cu(NO3)2·3H2O or CuCl2·2H2O under solvothermal conditions provided the same 2D, non-zwitterionic polymeric complex, {[Cu(hmnH)2]·DMF·H2O}n (8) (Scheme 1). The Cu(II) center is octahedral with the equatorial plane containing a pair of chelating N and O atoms from two trans-configuration hmnH ligands. The axial positions are occupied by two monodentate carboxylates. This results in an inter-connecting square-grid structure that is further stabilized by strong hydrogen bonding between the OH group of the pyridine alcohol moiety and the uncoordinated O atoms in the neighboring carboxylate (O1⋯O3a 2.648(6) Å, ∠O1–H⋯O3a 179(7)°; a: −0.5 − x, 0.5 + y, 1.5 − z) (Fig. 4a). The 2D network extends within the bc plane (Fig. 4b) and stack of these sheets along the a direction yields one dimensional channels wherein disordered DMF and H2O solvents reside (Fig. 4c). Powder X-ray diffraction confirmed the phase purity of 8 (Fig. S2, ESI†). Thermogravimetric analysis indicated that the framework of 8 is stable till around 210 °C, after which decomposition follows accompanied by the loss of DMF solvates (Fig. S3, ESI†).
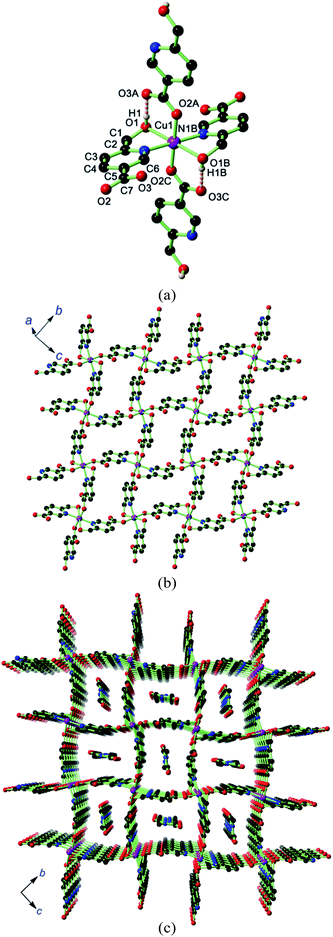 |
| Fig. 4 Structure of {[Cu(hmnH)2]·DMF·H2O}n (8) showing, (a) coordination environment around Cu1, (b) 2D layer in bc plane and (c) stacking of the 2D layers to generate 1D channels along the a axis. Symmetry codes: A −0.5 − x, 0.5 + y, 1.5 − z; B −x, 1 − y, 2 − z; C 0.5 + x, 0.5 − y, 0.5 + z. | |
We extended the spacers and conjugations in these ligands by inserting a phenyl group between the ethyl carboxylate and pyridinemethanol, viz. 4,2,4-ehpbH, 3,2,4-mhpbH and 4,6,3-ehpbH (4,2,4-ehpbH = ethyl 4-(2-(hydroxymethyl)pyridin-4-yl)benzoate; 3,2,4-mhpbH = methyl 3-(2-(hydroxymethyl)pyridin-4-yl)benzoate; 4,6,3-ehpbH = ethyl 4-(6-(hydroxymethyl)pyridin-3-yl)benzoate) (Chart 1). Reactions of Zn(NO3)2·6H2O with 4,2,4-ehpbH, 3,2,4-mhpbH or 4,6,3-ehpbH in DMF/H2O under solvothermal conditions gave rise to zwitterions cis-Zn(4,2,4-hpbH)2(H2O)2 (12), trans-Zn(3,2,4-hpbH)2(H2O)2 (13) and cis-[Zn(4,6,3-hpbH)2(H2O)2]·H2O (14) (4,2,4-hpbH = 4-(2-(hydroxymethyl)pyridin-4-yl)benzoate; 3,2,4-hpbH = 3-(2-(hydroxymethyl)pyridin-4-yl)benzoate; 4,6,3-hpbH = 4-(6-(hydroxymethyl)pyridin-3-yl)benzoate) (Scheme 2). Similar reactions of 3,2,4-mhpbH with Co(NO3)2·6H2O and Ni(NO3)2·6H2O yielded trans-Co(3,2,4-hpbH)2(H2O)2 (15) and trans-Ni(3,2,4-hpbH)2(H2O)2 (16) (Fig. S1, ESI†) which are isostructural with zinc analogue 13. Reaction of 4,6,3-ehpbH with Mn(NO3)2·4H2O gave rise to a 2D polymer [Mn(4,6,3-hpbH)2]n (17).
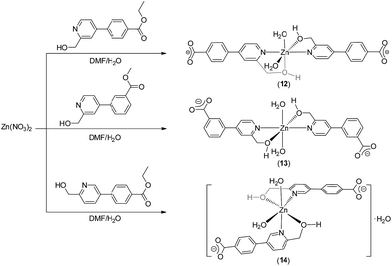 |
| Scheme 2 Schematic presentation of the synthesis of cis-Zn(4,2,4-hpbH)2(H2O)2 (12), trans-Zn(3,2,4-hpbH)2(H2O)2 (13) and cis-[Zn(4,6,3-hpbH)2(H2O)2]·H2O (14). | |
There are some notable differences in the structures of complexes 12–16. The structures of 12, 13, 15 and 16 mirror those of 2 and 1 (Scheme 2, Fig. 5a and b and S1, ESI†). However, the stereochemistry of cis 14 in which the two pyridine N atoms occupy equatorial and axial positions is in contrast to homologue cis 2 in which both pyridine N atoms are trans equatorial. Similarly, the cis stereochemistry of 12 and 14 is in contrast to the trans stereochemistry of 13. The notable structural diversity among these zwitterions presumably suggests the varied σ-donor ability and, therefore, trans influence of the pyridine atom in their respective ligand.
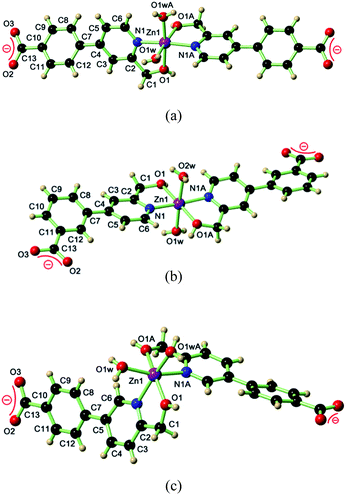 |
| Fig. 5 Structures of cis-Zn(4,2,4-hpbH)2(H2O)2 (12) (a), trans-Zn(3,2,4-hpbH)2(H2O)2 (13) (b) and cis-Zn(4,6,3-hpbH)2(H2O)2·H2O (14) (c) with the dissociated H2O molecules in 14 omitted for clarity. Symmetry codes for (12): A −x, y, 0.5 − z; (13): A −x, y, 0.5 − z; (14): A −x + 1, y, 0.5 − z. Selected C–O bond distances: C13–O2 1.251(5) Å, C13–O3 1.271(4) Å (12); C13–O2 1.239(2) Å, C13–O3 1.253(2) Å (13); C13–O2 1.268(2) Å, C13– O3 1.239(2) Å (14). | |
Although polymeric compound 17 has the same metal-to-ligand ratio as that of compound 8, the coordination environments around each metal center are distinctively different. The pyridine alcohol pair chelating Mn in 17 resembles that found in 14, where the N atoms are cis and O atoms trans. This disposition imposes the remaining sites, occupied by a pair of monodentate carboxylates, into a cis orientation (Fig. 6a). Each Mn(II) center connects to four adjacent centers through a pair of pyridine alcohol chelators and a pair of monodentate carboxylates to generate a two-dimensional layered structure in the ac plane (Fig. 6b). The different ligand arrangement in 17 relative to that of 8 also prevents hydrogen bonding between the alcoholic OH group and the proximate C
O group from the carboxylate on the same metal center. Instead, inter-layer hydrogen bonding (O1⋯O5a 2.564(3) Å, ∠O1–H1O⋯O5a 165(3)°; O4⋯O3b 2.559(3) Å, ∠O4–H4O⋯O3b 164(3)°; a: −x + 3, −y, −z + 2; b: −x + 2, −y + 1, −z + 4) is evident and the close stacking of adjacent layers block the apertures of the 2D layers, making 17 non-porous (Fig. S5, ESI†).
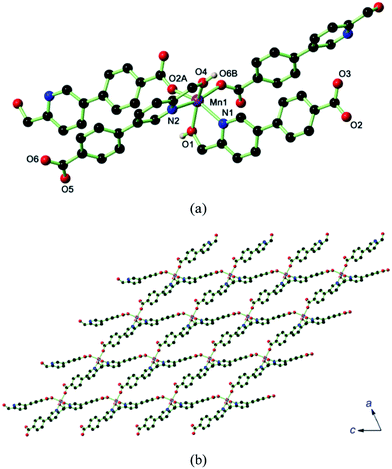 |
| Fig. 6 Crystal structure of [Mn(4,6,3-hpbH)2]n (17) showing, (a) the coordination environment around Mn1, and (b) the 2D layered structure in the ac plane. Hydrogen atoms except those on O4 and O1 in (a) are omitted. | |
Conclusions
Hydroxymethylpyridine-carboxylate (ester) ligands exhibit rich coordination chemistry by virtue of cooperative functionality that reacts differently under a diverse conditions of metal cations, solvents and temperatures, predominantly yielding unprecedented zwitterions of first row transition metals. This variable coordination behavior includes: (I) both the hydroxymethylpyridine and carboxylic acid remaining protonated (6 and 7); (II) hydroxymethylpyridine proton remains intact but the carboxylic acid is deprotonated to yield a zwitterion (1–5, 10, 12–16); (III) admixture of types I and II (9 and 11); (IV) the hydroxymethylpyridine proton remains intact and the carboxylic acid proton removed and bridges another metal center (8 and 17) and (V) both the hydroxymethylpyridine proton and carboxylic acid protons are removed to form high dimensional MOFs. The reasons behind these structural and stereochemical outcomes may originate from the different σ-donor ability of the ligand or/and the rich hydrogen bonding interactions between coordinated water/alcoholic OH (donor) and free carboxylate (acceptor), and is one of the subjects of our future study. Zwitterion is a type of polar functionality, and including such function within MOFs may result MOFs with increased uptake capabilities towards gases such as CO2. We are working towards these goals using pyridine alcohol ligands presented herein and their derivatives.
Experimental
All chemicals and solvents used were of A.R. grade and purchased from commercial suppliers. They were used without further purification unless otherwise specified. 1H NMR spectra were recorded on a Bruker (400 MHz) NMR spectrometer using CDCl3 or DMSO-d6 as solvent. MS (ESI) experiments were performed on an LCQ ion-trap mass spectrometer. Infrared spectra were obtained on a Perkin Elmer 2000 FT-IR spectrometer using KBr pellet. Elemental analyses were performed on a Perkin-Elmer PE 2400 CHNS elemental analyzer. Thermogravimetric analysis (TGA) was performed on a TA Instruments Q500 thermogravimetric analyzer at a heating rate of 10 °C min−1 under a nitrogen gas flow in an Al2O3 pan. Powder X-ray diffraction (PXRD) spectra were recorded with a Bruker D8 GADDS (General Area Detector Diffraction System) micro-diffractometer equipped with a VANTEC-2000 area detector with Φ rotation method. The X-ray generated from a sealed Cu tube was monochromated by a graphite crystal and collimated by a 0.5 mm MONOCAP (λ Cu-Kα = 1.54178 Å). The tube voltage and current were 40 kV and 40 mA, respectively.
Synthesis of ethyl 2-(hydroxymethyl)isonicotinate (iso-ehmnH) and ethyl 2-(hydroxymethyl)nicotinate (ehmnH)
Ethyl 2-(hydroxymethyl)isonicotinate (iso-ehmnH) and ethyl 2-(hydroxymethyl)nicotinate (ehmnH) were synthesized following the published procedure.6a Yield 50% for iso-ehmnH over two steps from pyridine-2,4-dicarboxylic acid. 1H NMR (400 MHz, CDCl3): δ 8.69 (d, J = 5.0 Hz, 1H), 7.86 (s, 1H), 7.77 (d, J = 4.9 Hz, 1H), 4.84 (s, 2H), 4.40 (q, J = 7.1 Hz, 2H), 3.59 (br, 1H), 1.40 (t, J = 7.1 Hz, 3H) ppm. 13C {1H} NMR (101 MHz, CDCl3): δ 165.3, 161.8, 149.6, 138.9, 121.8, 120.3, 64.8, 62.2, 14.5 ppm. MS (ESI, CHCl3, 150 °C): 182.1 m/z: [M + H]+. Anal. calcd (%) for C9H11NO3: C 59.66, H 6.12, N 7.73; found: C 59.84, H 5.45, N 7.82. IR (KBr pellet): 3241 (br), 2988 (w), 2908 (w), 2830 (w), 1728 (s), 1607 (s), 1567 (s), 1479 (m), 1448 (m), 1398 (s), 1366 (m), 1299 (s), 1232 (m), 1204 (s), 1098 (s), 1063 (s), 1024 (s), 923 (w), 905 (w), 868 (m), 813 (w), 767 (s), 717 (m), 687 (s), 600 (w), 493 (w) cm−1.
Yield 52% for ehmnH over two steps from pyridine-2,5-dicarboxylic acid. 1H NMR (400 MHz, CDCl3): δ 8.85 (s, 1H), 8.07 (d, J = 8 Hz, 1H), 7.34 (d, J = 8 Hz, 1H), 5.26 (s, 1H), 4.65 (s, 2H), 4.20 (q, J = 7 Hz, 2H), 1.21 (t, J = 7 Hz, 3H). 13C {1H} NMR (101 MHz, CDCl3): δ 165.5, 164.0, 150.3, 138.1, 125.6, 120.3, 64.7, 61.8, 14.6 ppm. MS (ESI, CHCl3, 150 °C): 182.3 m/z: [M + H]+, 204.1 m/z: [M + Na]+. Anal. calcd for C9H11NO3: C, 59.66; H, 6.12; N, 7.73%. Found: C, 60.01; H, 6.35; N, 7.92%. IR (KBr pellet): 3417 (sh), 3214 (s), 2986 (m), 2910 (m), 2840 (m), 2776 (w), 2704 (m), 2646 (w), 2574 (w), 2417 (w), 2262 (w), 2138 (w), 1992 (w), 1877 (w), 1860 (w), 1716 (s), 1659 (sh), 1626 (w), 1604 (s), 1575 (s), 1496 (w), 1476 (s), 1453 (s), 1399 (w), 1383 (s), 1366 (s), 1294 (s), 1272 (s), 1253 (s), 1216 (s), 1135 (s), 1110 (s), 1077 (s), 1027 (s), 878 (m), 859 (s), 812 (m), 785 (m), 761 (s), 722 (m), 694 (m), 667 (m), 643 (m), 561 (w), 476 (w), 457 (m), 422 (w), 407 (w) cm−1.
Synthesis of 2-(hydroxymethyl)isonicotinic acid (iso-hmnH2)
iso-EhmnH (4.84 g, 26.7 mmol) was dissolved in THF/H2O (40 mL, v/v = 1
:
1). KOH (4.58 g, 81.6 mmol, 3 eq.) was added as solid and the mixture stirred at 100 °C for 20 h and then cooled to r.t. The mixture was further cooled in ice-water and then HNO3 added to adjust the pH to 4–5. The solvent was removed under vacuum. The organic part in the solid mixture was then extracted twice with 400 mL of ethanol and the solvent removed. The powder contains inorganic material was added in hot iso-propanol and then filtered, evaporation of iso-propanol yielded iso-hmnH2 as white powder. Yield 2.93 g, 72%. 1H NMR (400 MHz, DMSO-d6): δ 8.61 (d, J = 5.0 Hz, 1H), 7.91 (s, 1H), 7.65 (d, J = 4.8 Hz, 1H), 4.62 (s, 2H), 4.42 (broad, 9H) ppm. 13C {1H} NMR (101 MHz, DMSO-d6): δ 167.6, 163.9, 150.1, 141.3, 121.8, 120.1, 64.9 ppm. MS (ESI, MeOH, 150 °C): 152.07 m/z: [M − H]−. Anal. calcd (%) for C7H7NO3: C 54.90, H 4.61, N 9.15; found: C 54.64, H 4.16, N 9.15. IR (KBr pellet): 3420 (s, br), 3048 (m, sh), 2939 (w), 2870 (w), 2642 (w), 1959 (w), 1667 (s), 1630 (s), 1618 (s), 1584 (s), 1546 (s), 1450 (m), 1413 (m), 1384 (s), 1334 (m), 1281 (w), 1252 (w), 1215 (w), 1117 (w), 1102 (w), 1061 (s), 1045 (s), 1027 (m), 985 (w), 974 (w), 946 (w), 905 (w), 879 (m), 788 (s), 773 (s), 731 (m), 697 (m), 681 (m), 661 (m), 627 (m), 569 (m), 500 (m), 421 (m) cm−1.
Synthesis of 2-(hydroxymethyl)nicotinic acid (hmnH2)
2-(Hydroxymethyl)nicotinic acid (hmnH2) was prepared using the same method as iso-hmnH2. Yield: approximately 75%. 1H NMR (400 MHz, DMSO-d6): δ 8.95 (s, 1H), 8.20–8.23 (m, 1H), 7.50 (d, J = 8.0 Hz, 1H), 5.38 (br, 4H), 4.61 (s, 2H) ppm. 13C {1H} NMR (101 MHz, DMSO-d6): δ 167.4, 164.1, 149.5, 137.1, 129.3, 119.4, 64.2 ppm. MS (ESI, MeOH, 150 °C): 152.07 m/z: [M − H]−. Anal. calcd (%) for C7H7NO3: C 54.90, H 4.61, N 9.15; found: C 53.19, H 4.45, N 8.75. IR (KBr pellet): 3390 (s), 2914 (br), 2631 (w), 2524 (m), 2481 (m), 2450 (m), 2024 (w), 2001 (w), 1971 (w), 1935 (w), 1724 (s), 1641 (s), 1607 (s), 1542 (m), 1449 (s), 1396 (s), 1331 (m), 1315 (m), 1241 (m), 1134 (s), 1077 (s), 1026 (m), 1001 (m), 964 (w), 865 (s), 822 (s), 743 (s), 687 (m), 637 (s), 525 (m), 483 (m), 456 (m) cm−1.
Synthesis of ethyl 4-(2-(hydroxymethyl)pyridin-4-yl)benzoate (4,2,4-ehpbH)
Step 1. Methyl 4-chloro-2-pyridinecarboxylate (0.86 g, 5.0 mmol), 4-(methoxycarbonyl)phenylboronic acid (1.30 g, 7.2 mmol) and K3PO4 (10.6 g, 50 mmol) were mixed in toluene (80 mL), and de-aerated using N2. PdCl2(dppf) (0.29 g, 0.36 mmol) was added, and the mixture stirred at 110 °C for 48 h under N2 atmosphere. The solution was evaporated to dryness under vacuum, extracted with CHCl3 and then dried over anhydrous MgSO4. Subsequent column purification with EA/hexane (1
:
1, v/v) gave a white solid of methyl 4-(2-(methoxycarbonyl)pyridin-4-yl)benzoate. Yield: 1.20 g, 88% based on methyl 4-chloro-2-pyridinecarboxylate. 1HNMR (400 MHz, CDCl3): δ 8.82 (d, J = 4.8 Hz, 1H), 8.41 (s, 1H), 8.37 (s, 1H), 8.15 (d, J = 7.4 Hz, 1H), 7.88 (d, J = 7.5 Hz, 1H), 7.78–7.70 (m, 1H), 7.60 (m, 1H), 3.97 (s, 3H), 4.05 (s, 3H) ppm. 13C {1H} (101 MHz, CDCl3): δ 166.9, 166.0, 150.8, 149.2, 149.0, 141.8, 131.5, 130.9, 127.5, 125.1, 123.5, 53.4, 52.7 ppm. MS (ESI, MeOH, 150 °C): 272.08 m/z: [M + H]+. Anal. calcd for C15H13NO4: C 66.41, H 4.83, N 5.16; found: C 66.44, H 4.31, N 5.15. IR (KBr pellet): 3417 (w), 3078 (w), 3042 (w), 3001 (w), 2952 (w), 2851 (w), 1955 (w), 1824 (w), 1729 (s), 1716 (s), 1600 (s), 1575 (w), 1543 (w), 1451 (m), 1441 (s), 1420 (w), 1397 (w), 1377 (w), 1321 (s), 1292 (s), 1251 (s), 1238 (m), 1195 (w), 1185 (w), 1133 (m), 1120 (s), 1105 (m), 1050 (m), 1016 (w), 997 (w), 914 (w), 874 (w), 847 (m), 830 (w), 788 (w), 767 (s), 721 (m), 708 (w), 700 (w), 656 (w), 583 (w), 528 (w), 499 (w), 477 (w), 435 (w), 407 (w) cm−1.
Step 2. To a degassed EtOH (100 mL) solution containing methyl 4-(2-(methoxycarbonyl)pyridin-4-yl)benzoate (1.20 g, 4.4 mmol) and NaBH4 (0.11 g, 3.0 mmol) was slowly added CaCl2 (0.50 g, 4.42 mmol) at 0 °C with stirring. After the addition completed, the mixture was stirred for 3 h at the same temperature. The reaction was quenched by dropwise addition of concentrated H2SO4. The resultant mixture was evaporated to dryness under vacuum, extracted with CHCl3, then dried over anhydrous MgSO4. Removal of solvent followed by column purification with EA/hexane (3
:
1, v/v) gave a white solid of the transesterification product ethyl 4-(2-(hydroxymethyl)pyridin-4-yl)benzoate. Yield: 0.90 g, 79%. 1H NMR (400 MHz, CDCl3): δ 8.64 (d, J = 5.1 Hz, 1H), 8.16 (d, J = 8.4 Hz, 2H), 7.70 (d, J = 8.4 Hz, 2H), 7.50 (s, 1H), 7.45 (d, J = 5.1 Hz, 1H), 4.85 (s, 2H), 4.42 (q, J = 7.1 Hz, 2H), 3.67 (s, 1H), 1.42 (t, J = 7.1 Hz, 3H) ppm. 13C NMR (101 MHz, CDCl3): δ 166.5, 160.3, 149.6, 148.5, 142.7, 131.5, 130.7, 127.5, 121.0, 118.8, 64.8, 61.6, 14.7 ppm. MS (ESI, MeOH, 150 °C): 258.15 m/z: [M + H]+. Anal. calcd for C15H15NO3: C 70.02, H 5.88, N 5.44; found: C 70.23, H 5.55, N 5.28. IR (KBr pellet): 3428 (w), 3154 (w), 2983 (w), 2908 (w), 2844 (w), 2822 (w), 1713 (s), 1665 (w), 1636 (w), 1603 (s), 1577 (w), 1547 (w), 1480 (w), 1465 (w), 1444 (w), 1419 (w), 1398 (w), 1364 (w), 1315 (w), 1290 (m), 1276 (s), 1183 (w), 1126 (m), 1117 (m), 1105 (m), 1066 (m), 1048 (w), 1029 (w), 1018 (w), 1104 (w), 981 (w), 880 (w), 867 (w), 849 (w), 831 (m), 771 (s), 742 (w), 733 (w), 702 (w), 659 (w), 612 (w), 586 (w), 521 (w), 495 (w), 443 (w), 435 (w), 428 (w) cm−1.
Synthesis of methyl 3-(2-(hydroxymethyl)pyridin-4-yl)benzoate (3,2,4-mhpbH)
Ligand 3,2,4-mhpbH was synthesized following the same route as described for 4,2,4-ehpbH.
Step 1. Methyl 3-(2-(methoxycarbonyl)pyridin-4-yl)benzoate was formed as a white solid in 92% yield. 1H NMR (400 MHz, CDCl3) δ 8.81 (d, J = 4.7 Hz, 1H), 8.41 (d, J = 1.2 Hz, 1H), 8.36 (s, 1H), 8.14 (d, J = 7.8 Hz, 1H), 7.88 (d, J = 7.8 Hz, 1H), 7.73 (d, J = 3.3 Hz, 1H), 7.59 (t, J = 7.8 Hz, 1H), 4.04 (s, 3H), 3.96 (s, 3H). 13C NMR (101 MHz, CDCl3): δ 166.8, 166.1, 150.8, 149.2, 149.1, 137.9, 131.7, 131.0, 129.8, 128.6, 125.0, 123.5, 53.4, 52.8 ppm. MS (ESI, MeOH, 150 °C): 272.11 m/z: [M + H]+. Anal. calcd (%) for C15H13NO4: C 66.41, H 4.83, N 5.16; found: C 66.66, H 4.28, N 5.11. IR (KBr pellet): 3410 (w), 3025 (w), 2958 (w), 1747 (m), 1716 (s), 1600 (m), 1589 (w), 1549 (w), 1508 (w), 1468 (w), 1439 (s), 1406 (w), 1329 (s), 1303 (s), 1277 (s), 1243 (s), 1185 (w), 1136 (w), 1109 (w), 1084 (w), 1059 (w), 1002 (w), 977 (w), 914 (w), 897 (w), 878 (w), 857 (w), 835 (w), 785 (m), 777 (w), 755 (s), 723 (m), 709 (w), 688 (w), 676 (w), 623 (w), 594 (w), 535 (w), 486 (w), 419 (w) cm−1.
Step 2. Methyl 3-(2-(hydroxymethyl)pyridin-4-yl)benzoate was formed in degassed MeOH solution and purified as a white solid in 80% yield. In situ transesterification was not observed. 1H NMR (400 MHz, CDCl3): δ 8.60 (d, J = 4.9 Hz, 1H), 8.30 (s, 1H), 8.09 (d, J = 7.8 Hz, 1H), 7.81 (d, J = 7.7 Hz, 1H), 7.55 (m, 2H), 7.43 (d, J = 4.7 Hz, 1H), 4.84 (s, 2H), 3.95 (s, 3H) ppm. 13C NMR (101 MHz, CDCl3): δ 166.9, 160.6, 149.6, 148.5, 138.8, 131.7, 130.5, 129.6, 128.6, 120.8, 118.8, 64.9, 52.7 ppm. MS (ESI, MeOH, 150 °C): 244.07 m/z: [M + H]+. Anal. calcd (%) for C14H13NO3: C 69.12, H 5.39, N 5.76; found: C 69.48, H 4.89, N 5.63. IR (KBr pellet): 3424 (w), 3146 (w), 3069 (w), 3039 (w), 2949 (w), 2919 (w), 2847 (w), 2637 (w), 2054 (w), 1982 (w), 1933 (w), 1888 (w), 1835 (w), 1768 (w), 1725 (s), 1612 (m), 1599 (m), 1585 (w), 1551 (w), 1493 (w), 1478 (w), 1438 (w), 1402 (m), 1362 (w), 1321 (m), 1309 (m), 1293 (m), 1258 (s), 1215 (w), 1203 (m), 1173 (w), 1113 (s), 1088 (m), 1067 (w), 1034 (s), 1005 (m), 980 (w), 966 (w), 916 (w), 898 (w), 864 (m), 834 (w), 824 (m), 774 (w), 758 (s), 698 (m), 678 (w), 664 (w), 628 (m), 580 (w), 545 (w), 500 (w), 465 (w), 422 (w) cm−1.
Synthesis of ethyl 4-(6-(hydroxymethyl)pyridin-3-yl)benzoate (4,6,3-ehpbH)
Ligand 4,6,3-ehpbH was synthesized following the same route as described for 4,2,4-ehpbH.
Step 1. Methyl 4-(6-(methoxycarbonyl)pyridin-3-yl)benzoate was formed as a white solid in 72% yield. 1H NMR (400 MHz, CDCl3): δ 8.98 (d, J = 1.8 Hz, 1H), 8.23 (d, J = 8.1 Hz, 1H), 8.18 (s, 1H), 8.16 (s, 1H), 8.06 (m, 1H), 7.71 (s, 1H), 7.68 (s, 1H), 4.04 (s, 3H), 3.96 (s, 3H) ppm. 13C NMR (101 MHz, CDCl3): δ 166.9, 165.9, 148.7, 147.6, 141.4, 139.1, 135.8, 130.9, 127.8, 125.6, 53.4, 52.7 ppm. MS (ESI, MeOH, 150 °C): 271.99 m/z: [M + H]+. Anal. calcd (%) for C15H13NO4: C 66.41, H 4.83, N 5.16; found: C 66.49, H 4.97, N 5.15. IR (KBr pellet): 3418 (w), 2959 (w), 2919 (w), 2840 (w), 1720 (s), 1608 (w), 1589 (w), 1578 (w), 1558 (w), 1476 (w), 1449 (w), 1430 (s), 1418 (w), 1364 (w), 1317 (s), 1288 (s), 1273 (s), 1240 (s), 1190 (m), 1130 (m), 1124 (m), 1104 (s), 1033 (w), 1018 (w), 1001 (w), 950 (m), 875 (w), 851 (m), 828 (w), 799 (w), 767 (s), 701 (m), 683 (w), 644 (w), 628 (w), 522 (w), 521 (w), 486 (w), 411 (w) cm−1.
Step 2. Transesterification product ethyl 4-(6-(hydroxymethyl)pyridin-3-yl)benzoate was formed as a white solid in 70% yield. 1H NMR (400 MHz, CDCl3): δ 8.81 (s, 1H), 8.16 (s, 1H), 8.14 (s, 1H), 7.92 (dd, J = 8.1, 2.1 Hz, 1H), 7.66 (s, 1H), 7.64 (s, 1H), 7.37 (d, J = 8.1 Hz, 1H), 4.83 (s, 2H), 4.41 (q, J = 7.1 Hz, 2H), 3.75 (s, 1H), 1.42 (t, J = 7.1 Hz, 3H) ppm. 13C NMR (101 MHz, CDCl3): δ 166.6, 159.2, 147.5, 142.3, 135.7, 134.9, 130.7, 130.6, 127.4, 120.9, 64.6, 61.5, 14.7 ppm. MS (ESI, MeOH, 150 °C): 258.16 m/z: [M + H]+. Anal. calcd for C15H15NO3: C 70.02, H 5.88, N 5.44; found: C 69.70, H 5.16, N 5.29. IR (KBr pellet): 3413 (w), 3184 (m), 3059 (w), 3029 (w), 3007 (w), 2953 (w), 2896 (w), 2838 (w), 2708 (w), 2658 (w), 1717 (s), 1607 (m), 1576 (w), 1514 (w), 1481 (w), 1433 (m), 1418 (w), 1377 (m), 1364 (m), 1319 (w), 1290 (s), 1276 (s), 1210 (w), 1187 (m), 1103 (s), 1073 (s), 1023 (w), 1007 (s), 979 (w), 965 (w), 874 (w), 840 (m), 770 (s), 701 (s), 652 (w), 630 (w), 552 (w), 476 (w), 424 (w), 410 (w) cm−1.
Synthesis of trans-[Zn(iso-hmnH)2(H2O)2] (1)
Mixture of Zn(NO3)2·6H2O (30 mg, 0.1 mmol) and iso-ehmnH (36 mg, 0.2 mmol) were dissolved in DMF/H2O (6 mL, v/v = 1
:
1) and the mixture heated at 95 °C for 72 h to give a colorless solution which was filtered. Slow evaporation of the solution yielded colorless crystals of 1 which were collected, washed with H2O and drying under vacuum. Yield: 25 mg, 62% based on Zn. MS (ESI, MeOH and trace DMF/H2O, 150 °C): 369.7 m/z: [M − 2H2O + H]+. Anal. calcd (%) for C14H16N2O8Zn: C 41.45, H 3.98, N 6.91; found: C 41.78, H 3.74, N 7.01. IR (KBr pellet): 3347 (m), 3054 (m), 2914 (m), 2841 (m), 2513 (m), 1956 (w), 1683 (s), 1602 (s), 1549 (s), 1454 (s), 1386 (s), 1289 (m), 1258 (m), 1218 (m), 1125 (m), 1103 (m), 1074 (s), 1027 (m), 1008 (w), 898 (m), 867 (m), 779 (s), 717 (m), 699 (s), 554 (w), 499 (w), 427 (w) cm−1.
Synthesis of cis-[Zn(hmnH)2(H2O)2]·2H2O (2)
Complex 2 was synthesized from Zn(NO3)2·6H2O and ehmnH using the same reaction conditions described for the synthesis of 1. Yield: 29 mg, 66% based on Zn. MS (ESI, MeOH and trace DMF/H2O, 150 °C): 369.8 m/z: [M − 2H2O + H]+. Anal. calcd (%) for C14H20N2O10Zn·2H2O: C 41.45, H 3.98, N 6.91; found: C 41.33, H 4.05, N 6.89. IR (KBr pellet): 3403 (s), 3116 (s), 3077 (s), 2934 (m), 2857 (w), 2703 (w), 2444 (m), 2222 (m), 2004 (w), 1892 (w), 1778 (m), 1713 (w), 1614 (s), 1589 (s), 1558 (s), 1490 (s), 1446 (s), 1369 (s), 1340 (s), 1276 (s), 1259 (m), 1223 (m), 1154 (s), 1128 (m), 1078 (s), 1038 (s), 1004 (w), 939 (s), 877 (s), 866 (s), 848 (w), 800 (s), 772 (s), 719 (w), 687 (s), 653 (s), 544 (s), 496 (w), 477 (w), 444 (m), 421 (s) cm−1.
Synthesis of trans-[Co(iso-hmnH)2(H2O)2] (3)
Co(NO3)2·6H2O (29 mg, 0.1 mmol) and iso-hmnH2 (30 mg, 0.2 mmol) were dissolved in DMF/H2O/MeCN (6 mL, v/v/v = 1
:
1
:
1) and 5 drops (ca. 0.25 mL) of 68% HNO3 added. The mixture was kept at 100 °C for 36 h to give a large amount of orange crystals which were collected and dried under vacuum. Yield: 34 mg, 85% based on Co. Anal. calcd (%) for C14H16CoN2O8: C 42.12, H 4.04, N 7.02; found: C 42.23, H 4.02, N 6.82. IR (KBr pellet): 3296 (s), 3069 (sh), 3052 (sh), 2841 (w), 2498 (s, br), 1669 (sh), 1598 (s), 1564 (s), 1456 (m), 1420 (s), 1387 (s), 1342 (m), 1289 (m), 1256 (m), 1218 (w), 1124 (w), 1103 (w), 1068 (s), 1023 (w), 1009 (w), 910 (w), 896 (m), 867 (w), 778 (s), 718 (m), 699 (s), 545 (w), 503 (w), 428 (m) cm−1.
Synthesis of trans-[Ni(iso-hmnH)2(H2O)2] (4)
Complex 4 was synthesized from Ni(NO3)2·6H2O and iso-hmnH2 using the same reaction conditions described for the synthesis of 3. Yield: 78% based on Ni. Anal. calcd (%) for C14H16N2NiO8: C 42.15, H 4.04, N 7.02; found: C 42.45, H 3.88, N 7.03. IR (KBr pellet): 3303 (s), 3086 (sh), 3055 (sh), 2848 (w), 2507 (s, br), 1953 (w), 1794 (sh), 1599 (s), 1542 (s), 1456 (m), 1421 (s), 1387 (s), 1342 (m), 1289 (m), 1258 (m), 1220 (w), 1126 (w), 1105 (w), 1066 (s), 1027 (w), 1007 (w), 897 (w), 866 (w), 779 (s), 719 (m), 701 (s), 602 (w), 547 (w), 506 (w), 429 (m) cm−1.
Synthesis of cis-[Ni(hmnH)2(H2O)2]·2H2O (5)
Complex 5 was synthesized from Ni(NO3)2·6H2O and hmnH2 using the same reaction conditions described for the synthesis of 3. Yield: 28 mg, 64% based on Ni. Anal. calcd (%) for C14H20N2NiO10: C 39.47, H 4.50, N 6.58; found: C 39.47, H 4.82, N 6.45, corresponding to 5 – 0.5H2O. IR (KBr pellet): 3538 (s), 3255 (s), 3077 (s), 3049 (s), 2919 (m), 2844 (w), 2510 (w), 2010 (w), 1903 (w), 1687 (w), 1620 (s), 1596 (s), 1558 (s), 1509 (w), 1482 (w), 1456 (w), 1396 (m), 1367 (s), 1338 (s), 1280 (s), 1255 (w), 1156 (m), 1136 (m), 1036 (s), 1006 (w), 952 (w), 870 (w), 867 (w), 779 (m), 718 (w), 688 (w), 658 (w), 632 (w), 546 (w), 474 (w), 426 (w) cm−1.
Synthesis of trans-[Cu(iso-hmnH2)2(NO3)2]·2H2O (6)
Cu(NO3)2·3H2O (48 mg, 0.20 mmol) and iso-hmnH2 (61 mg, 0.4 mmol) were dissolved in MeOH/MeCN (6 mL, v/v = 2
:
1). The mixture was briefly stirred at r.t. and the small amount of precipitate was filtered off. The filtrate was allowed to slowly evaporate to give green crystals of 6. Yield: 42 mg, 40% based on Cu. MS (ESI, MeOH, 150 °C): 278.0 m/z: [Cu(iso-hmnH2)(NO3)]+, 368.0 m/z: [Cu(iso-hmnH2)2–H]+. Anal. calcd (%) for C14H18CuN4O14: C 31.73, H 3.42, N 10.57; found: C 37.07, H 3.91, N 8.97, roughly corresponding to trans-[Cu(iso-hmnH2)2(NO3)2]·2H2O − 2H2O + 3MeOH (C 34.61, H 4.44, N 9.50). IR (KBr pellet): 3434 (br, s), 2839 (w), 2782 (w), 2642 (w), 2505 (w), 2422 (w), 1952 (w), 1731 (m), 1638 (s), 1615 (s), 1561 (m), 1476 (w), 1384 (s), 1281 (m), 1258 (m), 1211 (w), 1181 (w), 1123 (w), 1097 (w), 1076 (w), 1036 (w), 988 (w), 902 (w), 871 (w), 808 (w), 761 (m), 721 (m), 687 (m), 575 (w), 532 (w), 472 (w), 426 (w) cm−1.
Synthesis of trans-[Cu(hmnH2)2(H2O)2][NO3]2 (7)
Complex 7 was synthesized from Cu(NO3)2·3H2O and hmnH2 using the same reaction conditions described for the synthesis of 6. Yield: 15% based on Cu. MS (ESI, MeOH, 150 °C): 368.01 m/z [Cu(hmnH2)2–H]+. Anal. calcd (%) for C14H18CuN4O14: C 31.73, H 3.42, N 10.57; found: C 34.23, H 3.24, N 10.41, corresponding to trans-[Cu(hmnH2)2(H2O)2][NO3]2–2H2O (C 34.05, H 2.86, N 11.35). IR (KBr pellet): 3424 (sh), 3182 (s), 3058 (s), 2932 (sh), 2579 (w), 2426 (w), 2008 (w), 1874 (w), 1763 (w), 1725 (s), 1714 (s), 1619 (s), 1558 (w), 1498 (m), 1435 (sh), 1385 (s), 1280 (m), 1257 (m), 1226 (s), 1210 (s), 1129 (s), 1046 (s), 1034 (s), 980 (m), 941 (w), 870 (m), 844 (w), 825 (m), 775 (s), 761 (s), 696 (sw), 643 (m), 535 (w), 499 (w), 429 (w) cm−1.
Synthesis of {[Cu(hmnH)2]·DMF·H2O}n (8)
Cu(NO3)2·3H2O (24 mg, 0.1 mmol) and hmnH2 (30 mg, 0.2 mmol) were dissolved in DMF/H2O/EtOH (6 mL, v/v/v = 1
:
1
:
1) and 5 drops of 68% HNO3 added (ca. 0.25 mL). The mixture was kept at 100 °C for 36 h to give a light blue solution. Slow evaporation of the solution yielded light blue crystals of 8, which were collected and dried under vacuum. Yield: 15 mg, 33% based on Cu. Anal. calcd (%) for C17H21CuN3O8: C 44.49, H 4.61, N 9.16; found: C 44.60, H 3.57, N 8.32, corresponding to [Cu(hmnH)2·0.5DMF·0.5H2O]n (C15.5H17CuN2.5O7: C 44.98, H 4.14, N 8.46). IR (KBr pellet): 3469 (m), 3077 (m), 3048 (m), 2949 (w), 2907 (w), 2805 (w), 2661 (w), 2514 (w), 2431 (w), 1891 (w), 1714 (m), 1671 (s), 1618 (s), 1602 (s), 1557 (s), 1498 (w), 1439 (w), 1391 (s), 1347 (s), 1282 (s), 1255 (w), 1235 (w), 1164 (m), 1135 (w), 1099 (w), 1063 (m), 1045 (s), 986 (w), 922 (w), 868 (m), 854 (m), 799 (m), 733 (s), 726 (w), 686 (w), 671 (w), 657 (m), 573 (w), 520 (m), 475 (w), 434 (m) cm−1.
Synthesis of [CuCl (iso-hmnH2)(iso-hmnH)]·H2O (9)
CuCl2·2H2O (17 mg, 0.1 mmol) and iso-hmnH2 (30 mg, 0.2 mmol) were dissolved in MeOH/MeCN (12 mL, v/v = 2
:
1) and the solution was slowly evaporated at r.t. to give blue crystals of 9 which were collected and dried under vacuum. Yield: 35 mg, 82% based on Cu. Anal. calcd (%) for C14H15ClCuN2O7: C 39.82, H 3.58, N 6.63; found: C 39.82, H 4.32, N 6.57. IR (KBr pellet): 3520 (s), 3420 (s), 3095 (m), 3051 (m), 2971 (m), 2892 (m), 2779 (m), 2695 (m), 2632 (m), 2585 (m), 2541 (m), 1947 (w), 1921 (w), 1718 (m), 1621 (s), 1590 (s), 1568 (s), 1552 (s), 1491 (w), 1445 (m), 1382 (s), 1278 (s), 1248 (s), 1204 (s), 1116 (w), 1100 (w), 1063 (s), 1035 (m), 909 (w), 893 (w), 863 (w), 784 (m), 765 (s), 714 (w), 700 (s), 683 (s), 652 (w), 590 (w), 539 (w), 500 (w), 472 (m), 432 (m) cm−1.
Synthesis of trans-Cu(iso-hmnH)2(H2O)2 (10)
CuCl2·2H2O (9 mg, 0.1 mmol) and iso-hmnH2 (15 mg, 0.2 mmol) were dissolved in DMF/H2O (6 mL, v/v = 1
:
1) and 5 drops of 68% HNO3 (ca. 0.25 mL) added. The mixture was kept at 85 °C for 72 h to give olive green crystals of 10 which was collected and dried under vacuum. Yield: 22 mg, 55% based on Cu. Anal. calcd (%) for C14H16CuN2O8: C 41.64, H 3.99, N 6.94; found: C 41.10, H 3.65, N 7.00. IR (KBr pellet): 3385 (s), 3294 (s), 3130 (s), 3088 (s), 3050 (s), 2905 (m), 2838 (m), 2721 (m), 1952 (w), 1618 (s), 1554 (s), 1494 (w), 1451 (m), 1384 (s), 1296 (m), 1278 (m), 1259 (m), 1213 (w), 1125 (w), 1100 (w), 1076 (m), 1035 (w), 1006 (w), 972 (w), 911 (w), 898 (w), 866 (w), 790 (s), 782 (s), 699 (s), 661 (w), 581 (w), 544 (w), 503 (w), 487 (w), 434 (w) cm−1.
Synthesis of CuCl(hmnH2)(hmnH) (11)
CuCl2·2H2O (17 mg, 0.1 mmol) and hmnH2 (30 mg, 0.2 mmol) were dissolved in MeOH/MeCN (12 mL, v/v = 2
:
1) and the solution was slowly evaporated at r.t. to give blue crystals of 11 which were collected and dried under vacuum. Yield: 25 mg, 62% based on Cu. Anal. calcd (%) for C14H13ClN2O6Cu: C 41.59, H 3.24, N 6.93; found: C 40.73, H 3.15, N 6.78. IR (KBr pellet): 3185 (m), 3062 (m), 2921 (m), 2770 (m), 2607 (m), 2533 (m), 2500 (m), 2004 (w), 1886 (w), 1708 (s), 1616 (s), 1586 (s), 1557 (s), 1499 (w), 1388 (s), 1375 (s), 1351 (w), 1331 (w), 1259 (s), 1229 (s), 1211 (m), 1153 (w), 1136 (s), 1046 (s), 1031 (s), 996 (w), 983 (w), 945 (w), 884 (w), 872 (m), 862 (m), 802 (s), 778 (s), 755 (m), 706 (w), 682 (w), 652 (w), 539 (m), 514 (w), 502 (w), 471 (w), 425 (w) cm−1.
Synthesis of cis-Zn(4,2,4-hpbH)2(H2O)2 (12)
Zn(NO3)2·6H2O (11.2 mg, 0.038 mmol) and 4,2,4-ehpbH (19.3 mg, 0.075 mmol) were dissolved in DEF/EtOH/H2O (5 mL, v/v/v = 2
:
2
:
1) and transferred to a glass bottle with screw cap. The bottle was heated in a programmable oven smoothly from 25 °C to 110 °C within 4 h and at 110 °C for 3 days, and then cooled to r.t. The formed colorless crystals of 12 were collected by filtration, wash with DMF and dry under vacuum. Yield: 15 mg, 72% based on Zn. MS (ESI, MeOH and trace DEF, 150 °C): 325.61 m/z: [Zn(4,2,4-hpbH) + MeOH]+. Anal. calcd (%) for C26H24N2O8Zn: C 55.98, H 4.34, N 5.02; found: C 55.76, H 4.02, N 4.94. IR (KBr pellet): 3285 (w), 2974 (w), 2923 (w), 2831 (w), 2607 (w), 2567 (w), 1930 (w), 1676 (w), 1619 (s), 1601 (s), 1561 (s), 1542 (sh), 1509 (w), 1470 (w), 1410 (w), 1364 (s), 1334 (m), 1282 (m), 1250 (w), 1193 (w), 1176 (w), 1134 (w), 1097 (w), 1087 (m), 1049 (m), 1017 (w), 965 (w), 885 (w), 868 (w), 838 (m), 787 (s), 734 (w), 706 (w), 645 (w), 595 (w), 538 (w), 513 (w), 488 (w), 439 (w), 413 (w) cm−1.
Synthesis of trans-Zn(3,2,4-hpbH)2(H2O)2 (13)
Complex 13 was synthesized following the same route as described for 12. Yield 47% (based on Zn) as colorless crystals. MS (ESI, MeOH and trace DEF, 150 °C): 325.56 m/z: [Zn(3,2,4-hpbH) + MeOH]+. Anal. calcd (%) for C26H24N2O8Zn: C 55.98, H 4.34, N 5.02; found: C 55.41, H 4.23, N 4.99. IR (KBr pellet): 3266 (br), 3059 (w), 2931 (w), 2641 (w), 2503 (w), 1620 (s), 1591 (s), 1546 (vs), 1471 (w), 1447 (m), 1380 (vs), 1313 (w), 1274 (m), 1197 (w), 1167 (w), 1123 (w), 1077 (m), 1052 (s), 1025 (m), 961 (w), 923 (w), 904 (w), 880 (w), 839 (m), 819 (w), 769 (s), 754 (m), 728 (w), 711 (w), 689 (w), 678 (s), 630 (m), 546 (w), 501 (m), 478 (w), 437 (w), 415 (w) cm−1.
Synthesis of cis-Zn(4,6,3-hpbH)2(H2O)2 (14)
Complex 14 was synthesized following the same route as described for 12. Yield 62% (based on Zn) as colorless crystals. MS (ESI, MeOH and trace DEF, 150 °C): 325.60 m/z: [Zn(4,6,3-hpbH) + MeOH]+, 404.89 m/z: [Zn(4,6,3-hpbH) + NaCl + 3H2O]+. Anal. calcd (%) for C26H26N2O9Zn: C 54.23, H 4.55, N 4.86; found: C 53.68, H 4.23, N 4.78. IR (KBr pellet): 3436 (br), 3103 (w), 3071 (w), 3037 (w), 2932 (w), 2906 (w), 2865 (w), 2725 (w), 2426 (w), 1950 (w), 1630 (w), 1609 (w), 1588 (m), 1566 (w), 1533 (m), 1489 (w), 1447 (w), 1415 (m), 1385 (s), 1369 (sh), 1317 (w), 1301 (w), 1243 (w), 1215 (w), 1183 (w), 1138 (w), 1108 (w), 1062 (w), 1046 (m), 1025 (w), 1010 (w), 990 (w), 948 (w), 871 (w), 832 (w), 819 (w), 790 (s), 744 (w), 731 (w), 720 (w), 706 (w), 668 (w), 649 (w), 566 (w), 551 (w), 525 (w), 496 (w), 448 (w), 438 (w), 418 (w) cm−1.
Synthesis of trans-Co(3,2,4-hpbH)2(H2O)2 (15)
Co(NO3)2·6H2O (3.6 mg, 0.013 mmol) and 3,2,4-mhpbH (6.0 mg, 0.025 mmol) were dissolved in DMF/EtOH/H2O (9 mL, v/v/v = 4
:
4
:
1) and transferred to a 50 mL glass reactor with screw cap. The bottle was transferred to a programmable oven and heated smoothly from 25 °C to 110 °C within 4 h and at 110 °C for 3 days before cooling to r.t. within 1.5 days to provide light orange crystals of 15. Yield 6 mg, 51% (based on Co). Anal. calcd (%) for C26H24CoN2O8·0.5H2O: C 55.72, H 4.50, N 5.00; found: C 55.40, H 4.32, N 5.00. IR (KBr pellet): 3234 (br), 3062 (s), 2925 (m), 2852 (m), 2641 (m), 2597 (m), 2509 (br), 1958 (w), 1921 (w), 1619 (vs), 1591 (vs), 1565 (vs), 1545 (vs), 1474 (w), 1450 (m), 1398 (vs), 1378 (vs), 1312 (m), 1286 (m), 1273 (m), 1200 (w), 1169 (w), 1122 (w), 1087 (w), 1073 (w), 1050 (vs), 1022 (m), 961 (w), 922 (w), 880 (m), 839 (m), 820 (m), 770 (vs), 754 (s), 732 (w), 690 (m), 679 (s), 629 (m), 592 (w), 549 (w), 502 (w), 484 (w), 437 (w), 418 (w) cm−1.
Synthesis of trans-Ni(3,2,4-hpbH)2(H2O)2 (16)
Complex 16 was synthesized following the same route as described for 15 from Ni(NO3)2·6H2O and 3,2,4-mhpbH. Yield 41.6% based on Ni. Anal. calcd (%) for C26H24N2NiO8·H2O: C 54.86, H 4.60, N 4.92; found: C 54.78, H 4.36, N 4.81. IR (KBr pellet): 3275 (br), 3059 (w), 2924 (m), 2852 (w), 2503 (br), 1745 (w), 1621 (s), 1591 (m), 1565 (m), 1542 (vs), 1504 (w), 1476 (w), 1448 (m), 1440 (s), 1380 (vs), 1338 (w), 1313 (w), 1286 (w), 1274 (w), 1202 (w), 1167 (w), 1123 (w), 1087 (w), 1049 (s), 1026 (m), 963 (w), 921 (w), 901 (w), 880 (w), 840 (w), 820 (w), 770 (s), 755 (m), 730 (w), 689 (m), 679 (m), 631 (m), 549 (w), 503 (w), 481 (w), 459 (w), 439 (w) cm−1.
Synthesis of [Mn(4,6,3-hpbH)2]n (17)
Mn(NO3)2·4H2O (2.51 mg, 0.01 mmol) and 4,6,3-ehpbH (5.14 mg, 0.02 mmol) were dissolved in DMF/EtOH/H2O (4 mL, v/v/v = 2
:
1
:
1). The solution was then placed in a 50 mL glass reactor with screw cap in a programmable oven and heated smoothly from r.t. to 110 °C and kept at 110 °C for 3 days before cooling to r.t. during one and half days to provide colorless crystals of 17. Yield: 2 mg, 39.1% based on Mn. Anal. calcd (%) for C26H20MnN2O6·H2O: C 58.99, H 4.19, N 5.29; found: C 58.45, H 4.07, N 5.48. IR (KBr pellet): 3430 (br), 3057 (w), 2927 (w), 2887 (w), 2825 (w), 2821 (w), 2579 (br), 1606 (vs), 1593 (vs), 1570 (s), 1548 (s), 1519 (m), 1455 (s), 1388 (vs), 1308 (w), 1279 (w), 1235 (w), 1224 (w), 1183 (w), 1141 (w), 1105 (w), 1066 (s), 1042 (m), 1009 (m), 969 (w), 941 (w), 930 (w), 872 (w), 833 (w), 814 (w), 781 (s), 738 (w), 719 (w), 705 (m), 656 (m), 548 (m), 527 (m), 485 (w), 429 (w), 417 (w) cm−1.
X-ray crystallography
Crystallographic measurements (except 12) were made on a Bruker AXS APEX II diffractometer by using graphite-monochromated Mo Kα (λ = 0.71073 Å) in the Institute of Materials Research and Engineering. Complex 12 was measured on an Agilent Technologies SuperNova Dual diffractometer with Cu Kα (λ = 1.54178 Å) X-ray Source in University of Malaya. The data were subjected for empirical absorption correction using SADABS8 (except 12) or spherical harmonics implemented in SCALE3 ABSPACK scaling algorithm (12). All crystal structures were solved by direct methods and refined on F2 by full-matrix least-squares techniques with SHELXTL-97 program.9
For 8, the DMF solvate adopts a symmetry induced disorder and the symmetry was suppressed using PART-1 instruction with the occupancy factors further fixed at 0.50. For 14, the dissociated water molecule is disordered by symmetry and PART-1 was used to suppress the symmetry and the occupancy factors were further fixed at 0.50. All the H atoms on the OH, H2O and/or –COOH groups were found for the difference Fourier map with O–H distances restrained to 0.83 Å while their thermal parameter fixed to Uiso(H) = 1.2Ueq(O). A summary of the key crystallographic data for 1–17 are listed in Table 1.
Table 1 Summary of crystallographic data for 1–17
Compounds |
1 |
2 |
3 |
4 |
R1 = ∑‖Fo| − |Fc‖/∑|Fo|. wR2 = {∑[w(Fo2 − Fc2)2]/∑[w(Fo2)2]}1/2. GOF = {∑[w(Fo2 − Fc2)2]/(n − p)}1/2, where n is the number of reflections and p is total number of parameters refined. |
Formula |
C14H16N2O8Zn |
C14H20N2O10Zn |
C14H16CoN2O8 |
C14H16N2NiO8 |
FW |
405.66 |
441.69 |
399.22 |
399.00 |
Crystal system |
Orthorhombic |
Monoclinic |
Orthorhombic |
Orthorhombic |
Space group |
Pbca |
C2/c |
Pbca |
Pbca |
a (Å) |
7.0534(4) |
13.6211(6) |
7.1867(3) |
7.1353(4) |
b (Å) |
13.3638(8) |
7.7315(4) |
13.3223(6) |
13.2866(8) |
c (Å) |
16.8646(10) |
16.8791(8) |
16.8797(7) |
16.8095(10) |
α (°) |
90.00 |
90.00 |
90.00 |
90.00 |
β (°) |
90.00 |
103.7790(10) |
90.00 |
90.00 |
γ (°) |
90.00 |
90.00 |
90.00 |
90.00 |
V (Å3) |
1589.66(16) |
1726.41(14) |
1616.12(12) |
1593.61(16) |
Z |
4 |
4 |
4 |
4 |
ρcalc (g cm−3) |
1.695 |
1.699 |
1.641 |
1.663 |
F(000) |
832 |
912 |
820 |
824 |
μ (mm−1) |
1.593 |
1.482 |
1.109 |
1.265 |
Total reflns |
20 767 |
15 177 |
33 694 |
45 407 |
Uniq. reflns |
1828 |
2152 |
4334 |
5008 |
Obsd reflns |
1528 |
1944 |
3448 |
3552 |
Rint |
0.0336 |
0.0211 |
0.0231 |
0.0309 |
Variables |
124 |
138 |
125 |
124 |
R1a |
0.0233 |
0.0234 |
0.0358 |
0.0323 |
wR2b |
0.0659 |
0.0601 |
0.1059 |
0.0951 |
GOFc |
1.087 |
1.085 |
1.114 |
1.045 |
ρmax/ρmin (e Å−3) |
0.374/−0.301 |
0.376/−0.255 |
0.588/−0.533 |
0.603/−0.496 |
5 |
6 |
7 |
8 |
9 |
C14H20N2NiO10 |
C14H18CuN4O14 |
C14H18CuN4O14 |
C17H21CuN3O8 |
C14H15ClCuN2O7 |
435.03 |
529.86 |
529.86 |
458.91 |
422.27 |
Monoclinic |
Monoclinic |
Triclinic |
Monoclinic |
Triclinic |
C2/c |
P21/n |
P![[1 with combining macron]](https://www.rsc.org/images/entities/char_0031_0304.gif) |
P21/n |
P![[1 with combining macron]](https://www.rsc.org/images/entities/char_0031_0304.gif) |
13.3910(4) |
8.5580(4) |
7.256(6) |
7.451(2) |
7.7474(7) |
7.7123(2) |
10.3042(5) |
7.425(6) |
10.916(3) |
9.0283(8) |
16.9892(5) |
12.2558(6) |
9.897 |
12.454(4) |
11.6264(10) |
90.00 |
90.00 |
89.716(17) |
90.00 |
81.603(2) |
104.4560(10) |
108.0080(10) |
77.622(17) |
106.955(6) |
80.274(2) |
90.00 |
90.00 |
86.150(19) |
90.00 |
81.512(2) |
1699.02(8) |
1027.81(9) |
519.6(7) |
968.9(5) |
786.61(12) |
4 |
2 |
1 |
2 |
2 |
1.701 |
1.712 |
1.693 |
1.573 |
1.783 |
904 |
542 |
271 |
474 |
430 |
1.203 |
1.145 |
1.133 |
1.178 |
1.601 |
22 722 |
14 171 |
12 033 |
15 551 |
11 032 |
3736 |
2456 |
2115 |
2231 |
2852 |
3266 |
2262 |
1954 |
1435 |
2564 |
0.0246 |
0.0182 |
0.0625 |
0.0983 |
0.0278 |
138 |
163 |
163 |
163 |
241 |
0.0277 |
0.0527 |
0.0350 |
0.0719 |
0.0279 |
0.0746 |
0.1470 |
0.0946 |
0.2294 |
0.0758 |
1.046 |
1.055 |
1.136 |
1.089 |
1.063 |
0.484/−0.269 |
2.473/−0.786 |
0.518/−0.759 |
1.705/−1.186 |
0.538/−0.514 |
10 |
11 |
12 |
13 |
C14H16CuN2O8 |
C14H13ClCuN2O6 |
C26H24N2O8Zn |
C26H24N2O8Zn |
403.83 |
404.25 |
557.84 |
557.84 |
Orthorhombic |
Triclinic |
Monoclinic |
Monoclinic |
Pbca |
P![[1 with combining macron]](https://www.rsc.org/images/entities/char_0031_0304.gif) |
C2/c |
C2/c |
6.9918(5) |
7.5935(3) |
28.325(2) |
25.0244(9) |
13.1602(9) |
7.7855(4) |
7.1216(6) |
7.5228(3) |
17.1546(12) |
14.6720(7) |
12.6168(13) |
12.9729(5) |
90.00 |
83.0350(10) |
90.00 |
90.00 |
90.00 |
86.3830(10) |
114.533(14) |
107.7859(7) |
90.00 |
61.4210(10) |
90.00 |
90.00 |
1578.45(19) |
756.08(6) |
2315.3(4) |
2325.47(15) |
4 |
2 |
4 |
4 |
1.699 |
1.776 |
1.600 |
1.593 |
828 |
410 |
1152 |
1152 |
1.432 |
1.656 |
1.973 |
1.113 |
15 498 |
33 421 |
7792 |
10 557 |
1805 |
4605 |
2402 |
2666 |
1501 |
4255 |
1991 |
2433 |
0.0296 |
0.0204 |
0.0929 |
0.0181 |
124 |
226 |
180 |
178 |
0.0247 |
0.0242 |
0.0572 |
0.0289 |
0.0651 |
0.0663 |
0.1594 |
0.0790 |
1.019 |
1.045 |
1.060 |
1.047 |
0.404/−0.336 |
0.449/−0.370 |
0.909/−0.959 |
0.483/−0.419 |
14 |
15 |
16 |
17 |
C26H26N2O9Zn |
C26H24CoN2O8 |
C26H24N2NiO8 |
C26H20MnN2O6 |
575.86 |
551.40 |
551.18 |
511.38 |
Monoclinic |
Monoclinic |
Monoclinic |
Triclinic |
C2/c |
C2/c |
C2/c |
P![[1 with combining macron]](https://www.rsc.org/images/entities/char_0031_0304.gif) |
26.264(2) |
25.051(11) |
24.917(4) |
9.904(2) |
8.4871(8) |
7.425(3) |
7.3870(11) |
10.165(2) |
12.0334(11) |
12.951(6) |
12.944(2) |
2.286(3) |
90.00 |
90.00 |
90.00 |
77.470(3) |
114.801(2) |
107.721(8) |
107.482(4) |
68.167(3) |
90.00 |
90.00 |
90.00 |
85.302(3) |
2434.9(4) |
2294.8(16) |
2272.6(6) |
1120.9(4) |
4 |
4 |
4 |
2 |
1.571 |
1.596 |
1.611 |
1.515 |
1192 |
1140 |
1144 |
526 |
1.069 |
0.806 |
0.912 |
0.636 |
7396 |
15 227 |
11 008 |
20 302 |
2777 |
2637 |
2071 |
4246 |
2542 |
1495 |
1239 |
3197 |
0.0143 |
0.1697 |
0.1516 |
0.0544 |
192 |
178 |
178 |
322 |
0.0283 |
0.0575 |
0.0563 |
0.0410 |
0.0857 |
0.1167 |
0.1290 |
0.1069 |
1.043 |
0.988 |
1.009 |
1.017 |
0.414/−0.412 |
0.408−0.437 |
0.482/−0.446 |
0.352/−0.377 |
Acknowledgements
This work was supported by IMRE assured funding 13-1C0203, National Natural Science Foundation of China (no. 21401134), Science and Technology Department of Jiangsu Province (BK20140307) and Department of Education of Jiangsu Province (14KJB150023). S.W.N. thanks the University of Malaya (UM.C/625/1/HIR/247) for supporting this study.
Notes and references
-
(a) R. Ramirez-Contreras, N. Bhuvanesh, J. Zhou and O. V. Ozerov, Angew. Chem., Int. Ed., 2013, 52, 10313–10315 CrossRef CAS PubMed;
(b) J. Plešek, B. Grüner, V. Šícha, V. Bőhmer and I. Císařová, Organometallics, 2012, 31, 1703–1715 CrossRef;
(c) J. W. Dube, C. L. B. Macdonald and P. J. Ragogna, Angew. Chem., Int. Ed., 2012, 51, 13026–13030 CrossRef CAS PubMed;
(d) T. T. Ong, P. Kavuru, T. Nguyen, R. Cantwell, Ł. Wojtas and M. J. Zaworotko, J. Am. Chem. Soc., 2011, 133, 9224–9227 CrossRef CAS PubMed;
(e) A. L. Cresswell, M.-O. M. Piepenbrock and J. W. Steed, Chem. Commun., 2010, 46, 2787–2789 RSC;
(f) H. Li, A. J. A. Aquino, D. B. Cordes, F. Hung-Low, W. L. Hase and C. Krempner, J. Am. Chem. Soc., 2013, 135, 16066–16069 CrossRef CAS PubMed.
- A. Vogler and H. Kunkely, Coord. Chem. Rev., 2007, 251, 577–583 CrossRef CAS PubMed.
-
(a) M. Stradiotto, K. D. Hesp and R. J. Lundgren, Angew. Chem., Int. Ed., 2010, 49, 494–512 CrossRef CAS PubMed;
(b) W. Jiang, Y. Gao, Y. Sun, F. Ding, Y. Xu, Z. Bian, F. Li, J. Bian and C. Huang, Inorg. Chem., 2010, 49, 3252–3260 CrossRef CAS PubMed.
-
(a) Q.-X. Yao, Z.-F. Ju, W. Li, W. Wu, S.-T. Zheng and J. Zhang, CrystEngComm, 2008, 10, 1299–1301 RSC;
(b) X.-H. Jin, J.-K. Sun, X.-M. Xu, Z.-H. Li and J. Zhang, Chem. Commun., 2010, 46, 4695–4697 RSC;
(c) M. Higuchi, K. Nakamura, S. Horike, Y. Hijikata, N. Yanai, T. Fukushima, J. Kim, K. Kato, M. Takata, D. Watanabe, S. Oshima and S. Kitagawa, Angew. Chem., Int. Ed., 2012, 51, 8369–8372 CrossRef CAS PubMed.
-
(a) H. Wang, K. Yao, Z. Zhang, J. Jagiello, Q. Gong, Y. Han and J. Li, Chem. Sci., 2014, 5, 620–624 RSC;
(b) M. Li and M. Dincă, Chem. Sci., 2014, 5, 107–111 RSC;
(c) A. P. Katsoulidis, K. S. Park, D. Antypov, C. Marti-Gastaldo, G. J. Miller, J. E. Warren, C. M. Robertson, F. Blanc, G. R. Darling, N. G. Berry, J. A. Purton, D. J. Adams and M. J. Rosseinsky, Angew. Chem., Int. Ed., 2014, 53, 193–198 CrossRef CAS PubMed;
(d) S. Yang, L. Liu, J. Sun, K. M. Thomas, A. J. Davies, M. W. George, A. J. Blake, A. H. Hill, A. N. Fitch, C. C. Tang and M. Schröder, J. Am. Chem. Soc., 2013, 135, 4954–4957 CrossRef CAS PubMed;
(e) T. Yamada, K. Otsubo, R. Makiura and H. Kitagawa, Chem. Soc. Rev., 2013, 42, 6655–6669 RSC;
(f) W. Xuan, C. Ye, M. Zhang, Z. Chen and Y. Cui, Chem. Sci., 2013, 4, 3154–3159 RSC;
(g) Y.-S. Wei, K.-J. Chen, P.-Q. Liao, B.-Y. Zhu, R.-B. Lin, H.-L. Zhou, B.-Y. Wang, W. Xue, J.-P. Zhang and X.-M. Chen, Chem. Sci., 2013, 4, 1539–1546 RSC;
(h) D. Liu, J.-P. Lang and B. F. Abrahams, J. Am. Chem. Soc., 2011, 133, 11042–11045 CrossRef CAS PubMed;
(i) C. Wang, D. Liu and W. Lin, J. Am. Chem. Soc., 2013, 135, 13222–13234 CrossRef CAS PubMed;
(j) J. A. Mason, M. Veenstra and J. R. Long, Chem. Sci., 2014, 5, 32–51 RSC;
(k) S. Pullen, H. Fei, A. Orthaber, S. M. Cohen and S. Ott, J. Am. Chem. Soc., 2013, 135, 16997–17003 CrossRef CAS PubMed;
(l) V. Guillerm, F. Ragon, M. Dan-Hardi, T. Devic, M. Vishnuvarthan, B. Campo, A. Vimont, G. Clet, Q. Yang, G. Maurin, G. Férey, A. Vittadini, S. Gross and C. Serre, Angew. Chem., Int. Ed., 2012, 51, 9267–9271 CrossRef CAS PubMed;
(m) A. McKinlay, R. Morris, P. Horcajada, G. Férey, R. Gref, P. Couvreur and C. Serre, Angew. Chem., Int. Ed., 2010, 49, 6260–6266 CrossRef CAS PubMed;
(n) S. Chavan, J. G. Vitillo, M. J. Uddin, F. Bonino, C. Lamberti, E. Groppo, K.-P. Lillerud and S. Bordiga, Chem. Mater., 2010, 22, 4602–4611 CrossRef CAS;
(o) H.-L. Zhou, R.-B. Lin, C.-T. He, Y.-B. Zhang, N. Feng, Q. Wang, F. Deng, J.-P. Zhang and X.-M. Chen, Nat. Commun., 2013, 4 DOI:10.1038/ncomms3534.
-
(a) W.-H. Zhang, N. B. Sulaiman, P. X. S. Tio and T. S. A. Hor, CrystEngComm, 2011, 13, 2915–2922 RSC;
(b) E. Y. Tsui, J. S. Kanady and T. Agapie, Inorg. Chem., 2013, 52, 13833–13848 CrossRef CAS PubMed;
(c) J. S. Kanady, T. Rosalie, J. A. Stull, L. Lu, T. A. Stich, M. W. Day, J. Yano, R. D. Britt and T. Agapie, Chem. Sci., 2013, 4, 3986–3996 RSC;
(d) E. Y. Tsui, M. W. Day and T. Agapie, Angew. Chem., Int. Ed., 2011, 50, 1668–1672 CrossRef CAS PubMed;
(e) T. C. Stamatatos, K. M. Poole, K. A. Abboud, W. Wernsdorfer, T. A. O'Brien and G. Christou, Inorg. Chem., 2008, 47, 5006–5021 CrossRef CAS PubMed;
(f) P. L. Feng, C. C. Beedle, C. Koo, W. Wernsdorfer, M. Nakano, S. Hill and D. N. Hendrickson, Inorg. Chem., 2008, 47, 3188–3204 CrossRef CAS PubMed;
(g) H. Hiraga, H. Miyasaka, K. Nakata, T. Kajiwara, S. Takaishi, Y. Oshima, H. Nojiri and M. Yamashita, Inorg. Chem., 2007, 46, 9661–9671 CrossRef CAS PubMed.
- M. Armaghan, et al., 2015, unpublished results.
- G. M. Sheldrick, SADABS. Program for empirical absorption correction of area detector data, University of Göttingen, Germany, 1996 Search PubMed.
- G. M. Sheldrick, SHELXS-97 and SHELXL-97. Programs for crystal structure solution and refinement, University of Göttingen, Germany, 1997 Search PubMed.
Footnote |
† Electronic supplementary information (ESI) available. CCDC 984612, 984613 and 1013140–1013154. Crystal structures of 3–5, 15 and 16, crystal packing diagram of 1, 2, 12–14 and 17, simulated and experimental PXRD patterns for 8, TGA curves for 8. For ESI and crystallographic data in CIF or other electronic format see DOI: 10.1039/c5ra05564d |
|
This journal is © The Royal Society of Chemistry 2015 |