DOI:
10.1039/C5RA05155J
(Communication)
RSC Adv., 2015,
5, 39539-39543
Aerobic photooxidative direct asymmetric aldol reactions of benzyl alcohols using water as the solvent†
Received
24th March 2015
, Accepted 16th April 2015
First published on 16th April 2015
Abstract
We report an aerobic photooxidative direct asymmetric aldol reaction using water as the solvent. In this reaction, primary benzyl alcohols are oxidized into benzaldehydes under an oxygen atmosphere using anthraquinone-2-sodium sulfonate monohydrate as an organophotocatalyst. Stereoselective aldol reactions then proceed using a proline-type organocatalyst.
The aldol reaction is a fundamental and reliable method for forming carbon–carbon bonds. The products of this reaction, β-hydroxy carbonyl compounds, are contained in many natural products and are used in many medicines.1 Direct asymmetric aldol (DAA) reactions catalyzed by organocatalysts have been actively developed in recent decades because such reactions offer advantages, such as stability in the presence of air and water and reactants with low toxicities, over alternative reactions.2 A great deal of attention has been paid to the use of water as a solvent for these reactions because it is a safe, inexpensive, and environmentally benign solvent.3 Various organocatalytic DAA reactions using water as the solvent have been reported.4
Catalytic tandem reactions, in which a series of multistep reactions are performed in a single vessel, can be powerful tools from an environmental viewpoint because they save energy and waste.5,6 Alcohols are readily accessible, low cost, and easy to handle, so some researchers have reported the reactions in which alcohols are used as starting materials to produce aldehydes in situ.7,8 To the best of our knowledge, no oxidative DAA reactions using alcohol have yet been developed. There are some difficulties in performing such a reaction: (1) the alcohol has to be selectively oxidized to the aldehyde and (2) the aldehyde that is generated, the ketone reactant, the asymmetric organocatalyst (such as a proline derivative), and the final product have to be able to survive the oxidative reaction conditions. Recently, Kudo and coworkers described highly stereoselective sequential oxidation/asymmetric aldol reactions of several benzyl alcohols using resin-supported catalysts, but the sequential addition of the ketone of interest was required after the oxidation reaction had been performed because the ketones used could not tolerate the oxidative conditions.9
We have been investigating mild oxidation reactions using molecular oxygen and irradiation with fluorescent lamps.10 Recently, we reported oxidation reactions catalyzed by anthraquinones, which acted as organophotocatalysts.11 Furthermore, we have also been studying organocatalyzed asymmetric aldol reactions using water or brine as the reaction solvent.12 It occurred to us that using water as the solvent would allow the selective oxidation of a benzyl alcohols (which would be relatively soluble in water) to be achieved without the other compounds in the reaction mixture (such as aldehydes, ketones, organocatalysts, and products) being oxidized and react with enamine generated by ketone with organocatalyst to give oxidative DAA reactions (Scheme 1). Here, we describe a detailed study of an oxidative DAA reaction using water as the solvent.
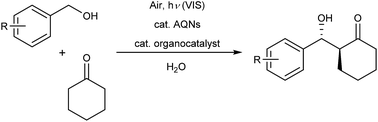 |
| Scheme 1 Oxidative direct asymmetric aldol reaction from benzyl alcohol. | |
First, we examined the asymmetric organocatalyzed reaction of 4-bromobenzyl alcohol (1a) and cyclohexanone (2a) in the presence of anthraquinone-2-sodium sulfonate monohydrate (AQN-2-SO3Na·H2O) using water under an air atmosphere (i.e., open) with irradiation from a fluorescent lamp for 12 h. Among the proline derivatives (4a–d) that were used as organocatalysts, only trans-4-tert-butyldiphenylsiloxy L-proline (4a) gave the β-hydroxy ketone 3a, and it gave a 28% yield with high stereoselectivity (Table 1).4c
Table 1 Study of organocatalyst
The results of the tests using different solvents and organophotocatalysts are shown in Table 2. We found that a combination of H2O and AQN-2-SO3Na·H2O that is soluble in water gave the photooxidative DAA reaction product 3a. General organic solvents gave none of the desired product 3a because AQN-2-SO3Na·H2O is insoluble in these solvents.
Table 2 Study of AQN derivatives and solvents
We then conducted further experiments to optimize the reaction conditions (Table 3). After several investigations, a prolonged reaction time (48 h) and the addition of AcOH (entry 10) which may accelerate the formation of enamine as an active species derived from 2a and 4a gave the best result.
Table 3 Study of further experiments to optimize the reaction conditions
We examined the scope and limitations of the reaction using the optimized conditions. As is shown in Table 4, the corresponding aldol adducts were obtained in moderate to good yields with strong enantioselectivities (entries 1–7). Reactions in which para- or meta-nitrobenzyl alcohols were used were conducted under an O2 atmosphere to promote the oxidation of alcohols (entries 8 and 9). However, substrates with an ortho-substituted or without electron-withdrawing groups on the benzene rings or heterocyclic rings gave poor results (entries 10–15). It seems that electron-withdrawing group on the benzene ring promote the aldol reaction by making electron poor at the carbonyl carbon of the corresponding benzaldehyde. We changed the ring size of cycloalkanone and found that cycloheptanone is not applicable to the reaction, even though cyclopentanone is (entries 16–21). Unfortunately, though we investigated the reactions with acyclic ketones, we could just get corresponding aldol adduct in low yields (entries 22 and 23).
Table 4 Scope and limitation
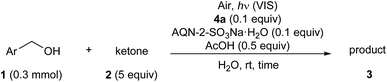
|
Entry |
|
Product |
H2O (mL) |
Time (h) |
Yielda (%) |
anti : synb |
% eec |
Isolated yields. Numbers in parentheses are yields determined by 1H NMR spectroscopic analysis of crude. Determined by 1H NMR spectroscopic analysis. Determined by HPLC. Without AcOH. 10 equiv. of ketone was used. Reactions were carried out under oxygen atmosphere. 27 equiv. of ketone was used. |
1 |
Ar1 = |
4-BrC6H4 (3a) |
 |
3 |
48 |
71 |
13 : 1 |
94 |
2 |
|
3-BrC6H4 (3b) |
3 |
48 |
60 |
16 : 1 |
>99 |
3d |
|
4-CIC6H4 (3c) |
2 |
48 |
63 |
14 : 1 |
95 |
4 |
|
3-CIC6H4 (3d) |
3 |
48 |
65 |
17 : 1 |
97 |
5 |
|
4-CF3C6H4 (3e) |
3 |
60 |
62 |
12 : 1 |
98 |
6 |
|
4-CNC6H4 (3f) |
3 |
60 |
58 |
7.4 : 1 |
>99 |
7d,e |
|
4-CO2Me6H4 (3g) |
2 |
48 |
73 |
15 : 1 |
>99 |
8d,f |
|
4-NO2C6H4 (3h) |
0.5 |
120 |
61 |
9.7 : 1 |
94 |
9d,f |
|
3-NO2C6H4 (3i) |
0.5 |
120 |
54 |
8.5 : 1 |
>99 |
10d,f |
|
2-NO2C6H4 (3j) |
1 |
120 |
(14) |
— |
— |
11d,f |
|
C6F5 (3k) |
0.5 |
120 |
(0) |
— |
— |
12f |
|
4-OMeC6H4 (3l) |
0.5 |
120 |
(7) |
— |
— |
13 |
|
2-Naphthyl (3m) |
0.5 |
48 |
(4) |
— |
— |
14 |
|
4-Pyridyl (3n) |
3 |
48 |
(7) |
— |
— |
15 |
|
2-Furyl (3o) |
3 |
48 |
(0) |
— |
— |
16d |
Ar2 = |
4-CIC6H4 (3p) |
 |
2 |
48 |
50 |
2.4 : 1 |
>99 |
17 |
|
4-CNC6H4 (3q) |
3 |
60 |
53 |
2.2 : 1 |
>99 |
18e,f |
|
4-NO2C6H4 (3q) |
0.2 |
120 |
55 |
3.2 : 1 |
>99 |
19d |
Ar3 = |
4-CIC6H4 (3s) |
 |
2 |
48 |
(2) |
— |
— |
20 |
|
4-CNC6H4 (3t) |
3 |
60 |
(7) |
— |
— |
21e,f |
|
4-NO2C6H4 (3u) |
0.5 |
120 |
(8) |
— |
— |
22d,f,g |
|
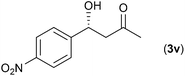 |
0.5 |
120 |
(5) |
— |
— |
23d,f,g |
|
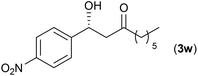 |
0.5 |
120 |
(Trace) |
— |
— |
Next, we performed some experiments to reveal the reaction mechanism (Scheme 2). When we conducted the reaction under Ar, the yield of the desired product is very low (eqn (1)). If there is no irradiation from fluorescent lamp, the reaction is suppressed perfectly (eqn (2)). In eqn (3), we added sodium L-ascorbate as a radical scavenger, the photooxidation of the alcohol is suppressed to a certain extent. Thus, we suppose that the oxidation step involves radical steps.
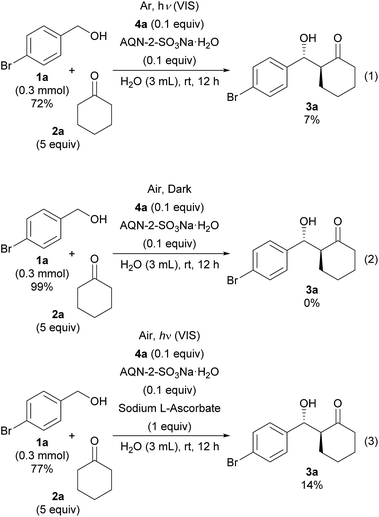 |
| Scheme 2 Study of reaction mechanism: yields are determined by 1H NMR spectroscopic analysis of the crude. | |
According to the results mentioned above, we depicted the plausible path of this reaction (Scheme 3). To begin with AQN-2-SO3− is excited by the irradiation of fluorescent lamp and H atom at the benzylic position of alcohol 1 is abstracted to produce benzyl radical. Then, this intermediate traps molecular oxygen to generate aldehyde via forming peroxy radical and hydroperoxide. Finally, the aldehyde react with ketone 2 assisted by proline-type catalyst and desired aldol adduct 3 is obtained.
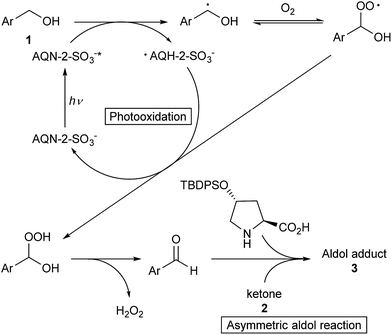 |
| Scheme 3 Plausible path. | |
Conclusions
In conclusion, we have described a photooxidative stereoselective aldol reaction starting from an alcohol substrate. This method uses molecular oxygen and visible light with AQN-2-SO3Na·H2O to oxidize the alcohol. We found that this photooxidation is not what interrupts the asymmetric aldol reaction because high enantioselectivity is preserved. The reaction can be performed using water as the solvent. We believe that this method should be applicable to other reactions starting from aldehydes. Further investigations into photooxidative reactions are now in progress in our laboratory.
Notes and references
- R. Mahrwald, in Modern Aldol Reactions, Wiley-VCH, Weinheim, Germany, 2004, vol. 1 and 2 Search PubMed.
- For selected reviews on organocatalysts, see:
(a) P. I. Dalko and L. Moisan, Angew. Chem., Int. Ed., 2004, 43, 5138 CrossRef CAS PubMed;
(b) H. Pellisier, Tetrahedron, 2007, 63, 9267 CrossRef PubMed;
(c) S. Mukherjee, J. W. Yang, S. Hoffmann and B. List, Chem. Rev., 2007, 107, 5471 CrossRef CAS PubMed;
(d) A. Dondoni and A. Massi, Angew. Chem., Int. Ed., 2008, 47, 4638 CrossRef CAS PubMed;
(e) A. Lattanzi, Chem. Commun., 2009, 1452 RSC;
(f) X. Liu, L. Lin and X. Feng, Chem. Commun., 2009, 6145 RSC;
(g) X.-H. Chen, J. Yu and L.-Z. Gong, Chem. Commun., 2010, 46, 6437 RSC;
(h) B. Bhanushali and C.-G. Zhao, Synthesis, 2011, 1815 Search PubMed.
- For reviews on organic synthesis in water, see:
(a) P. A. Grieco, in Organic Synthesis in Water, Blackie A&P, London, 1998 Search PubMed;
(b) S. Kobayashi and K. Manabe, Acc. Chem. Res., 2002, 35, 209 CrossRef CAS PubMed;
(c) U. M. Lindstrom, Chem. Rev., 2002, 102, 2751 CrossRef PubMed;
(d) C. J. Li, Chem. Rev., 2005, 105, 3095 CrossRef CAS PubMed;
(e) M. Raj and V. K. Singh, Chem. Commun., 2009, 6687 RSC;
(f) J. Paradowska, M. Stodulski and J. Mlynarski, Angew. Chem., Int. Ed., 2009, 48, 4228 CrossRef PubMed;
(g) M. Gruttadauria, F. Giacalone and R. Noto, Adv. Synth. Catal., 2009, 351, 33 CrossRef CAS PubMed;
(h) N. Mase and C. F. Barbas III, Org. Biomol. Chem., 2010, 8, 4043 RSC.
- For examples of organocatalyzed aldol reactions in water without any organic solvent using pyrrolidine derivatives, see:
(a) S. S. Chimni, D. Mahajan and V. V. S. Babu, Tetrahedron Lett., 2005, 46, 5617 CrossRef CAS PubMed;
(b) N. Mase, Y. Nakai, N. Ohara, H. Yoda, K. Takabe, F. Tanaka and C. F. Barbas III, J. Am. Chem. Soc., 2006, 128, 734 CrossRef CAS PubMed;
(c) Y. Hayashi, T. Sumiya, J. Takahashi, H. Gotoh, T. Urushima and M. Shoji, Angew. Chem., Int. Ed., 2006, 45, 958 CrossRef CAS PubMed;
(d) Y. Hayashi, S. Aratake, T. Okano, J. Takahashi, T. Sumiya and M. Shoji, Angew. Chem., Int. Ed., 2006, 45, 5527 CrossRef CAS PubMed;
(e) Y. Wu, Y. Zhang, M. Yu, G. Whao and S. Wang, Org. Lett., 2006, 8, 4417 CrossRef CAS PubMed;
(f) D. Font, C. Jimeno and M. A. Pericàs, Org. Lett., 2006, 8, 4653 CrossRef CAS PubMed;
(g) W.-P. Huang, J.-R. Chen, X.-Y. Li, Y.-J. Cao and W.-J. Xiao, Can. J. Chem., 2007, 85, 208 CrossRef CAS;
(h) F. Giacalone, M. Gruttadauria, A. M. Marculescu and R. Noto, Tetrahedron Lett., 2007, 48, 255 CrossRef CAS PubMed;
(i) S. Aratake, T. Itoh, T. Okano, T. Usui, M. Shoji and Y. Hayashi, Chem. Commun., 2007, 2524 RSC;
(j) V. Maya, M. Raj and V. K. Singh, Org. Lett., 2007, 9, 2593 CrossRef CAS PubMed;
(k) M. Gruttadauria, F. Giacalone, A. M. Marculescu, P. L. Meo, S. Riela and R. Noto, Eur. J. Org. Chem., 2007, 4688 CrossRef CAS PubMed;
(l) D. Font, S. Sayalero, A. Bastero, C. Jimeno and M. A. Pericàs, Org. Lett., 2008, 10, 337 CrossRef CAS PubMed;
(m) L. Zu, H. Xie, H. Li, J. Wang and W. Wang, Org. Lett., 2008, 10, 1211 CrossRef CAS PubMed;
(n) M. Lombardo, F. Pasi, S. Easwar and C. Trombini, Synlett, 2008, 2471 CrossRef CAS PubMed;
(o) S. S. Chimni, S. Singh and D. Mahajan, Tetrahedron: Asymmetry, 2008, 19, 2276 CrossRef CAS PubMed;
(p) S. S. Chimni, S. Singh and A. Kumar, Tetrahedron: Asymmetry, 2009, 20, 1772 CrossRef PubMed;
(q) S.-P. Zhang, X.-K. Fu and S.-D. Fu, Tetrahedron Lett., 2009, 50, 1173 CrossRef CAS PubMed;
(r) S.-D. Fu, X.-K. Fu, S.-P. Zhang, X.-C. Zou and X.-J. Wu, Tetrahedron: Asymmetry, 2009, 20, 2390 CrossRef CAS PubMed;
(s) M. R. Vishnumaya and V. K. Singh, J. Org. Chem., 2009, 74, 4289 CrossRef CAS PubMed;
(t) M. D. Nisco, S. Pedatella, H. Ullah, J. H. Zaidi, D. Naviglio, O. Ozdamar and R. Caputo, J. Org. Chem., 2009, 74, 9562 CrossRef PubMed;
(u) N. Mase, N. Noshiro, A. Mokuya and K. Takabe, Adv. Synth. Catal., 2009, 351, 2791 CrossRef CAS PubMed;
(v) Y.-N. Jia, F.-C. Wu, X. Ma, G.-J. Zhu and C.-S. Da, Tetrahedron Lett., 2009, 50, 3059 CrossRef CAS PubMed;
(w) Y.-J. An, Y.-X. Zhang, Y. Wu, Z.-M. Liu, C. Pi and J.-C. Tao, Tetrahedron: Asymmetry, 2010, 21, 688 CrossRef CAS PubMed;
(x) R. Pedrosa, J.-M. Andrés, R. Manzano, D. Román and S. Téllez, Org. Biomol. Chem., 2011, 9, 935 RSC.
-
(a) G. H. Posner, Chem. Rev., 1986, 86, 831 CrossRef CAS;
(b) T. L. Ho, in Tandem Organic Reactions, Wiley-Interscience, New York, 1992 Search PubMed;
(c) N. Hall, Science, 1994, 266, 32 CAS;
(d) J.-C. Wasilke, S. J. Obrey, T. Baker and G. C. Bazan, Chem. Rev., 2005, 105, 1001 CrossRef CAS PubMed.
-
(a) M. J. Burk, J. R. Lee and J. P. Martinez, J. Am. Chem. Soc., 1994, 116, 10847 CrossRef CAS;
(b) V. P. Balema and E. Hey-Hawkins, Z. Anorg. Allg. Chem., 1986, 622, 2053 CrossRef PubMed;
(c) D. E. Fogg and E. N. dos Santos, Coord. Chem. Rev., 2004, 248, 2365 CrossRef CAS PubMed.
- For reviews on the electrophilic reactions using alcohols as substrates, see:
(a) R. J. K. Taylor, M. Reid, J. Foot and S. A. Raw, Acc. Chem. Res., 2005, 38, 851 CrossRef CAS PubMed;
(b) G. Guillena, D. J. Ramón and M. Yus, Angew. Chem., Int. Ed., 2007, 46, 2358 CrossRef CAS PubMed; for recent examples of the sequential reactions including alcohols oxidations, see:
(c) X.-Q. Li, W.-K. Wang and C. Zhang, Adv. Synth. Catal., 2009, 351, 2342 CrossRef CAS PubMed;
(d) H. Sun, F.-Z. Su, J. Ni, Y. Cao, H.-Y. He and K.-N. Fan, Angew. Chem., Int. Ed., 2009, 48, 4390 CrossRef CAS PubMed;
(e) M. Davi and H. Lebel, Org. Lett., 2009, 11, 41 CrossRef CAS PubMed;
(f) A. J. Blacker, M. M. Farah, M. I. Hall, S. P. Marsden, O. Saidi and J. M. J. Williams, Org. Lett., 2009, 11, 2039 CrossRef CAS PubMed;
(g) R. Grigg, S. Whitney, V. Sridharan, A. Keep and A. Derrick, Tetrahedron, 2009, 65, 4375 CrossRef CAS PubMed;
(h) J. Brioche, G. Masson and J. Zhu, Org. Lett., 2010, 12, 1432 CrossRef CAS PubMed; for examples of direct amide synthesis form alcohols, see:
(i) C. Gunanathan, Y. Ben-David and D. Milstein, Science, 2007, 317, 790 CrossRef CAS PubMed;
(j) K. R. Reddy, C. U. Maheswari, M. Venkateshwar and M. L. Kantam, Eur. J. Org. Chem., 2008, 3619 CrossRef CAS PubMed;
(k) L. U. Nordstrøm, H. Vogt and R. Madsen, J. Am. Chem. Soc., 2008, 130, 17672 CrossRef PubMed;
(l) S. C. Ghosh, S. Muthaiah, Y. Zhang, X. Xu and S. H. Hong, Adv. Synth. Catal., 2009, 351, 2643 CrossRef CAS PubMed;
(m) T. Zweifel, J.-V. Naubron and H. Grützmacher, Angew. Chem., Int. Ed., 2009, 48, 559 CrossRef CAS PubMed;
(n) K. Shimizu, K. Oshima and A. Satsuma, Chem.–Eur. J., 2009, 15, 9977 CrossRef CAS PubMed;
(o) B. E. Maki and K. A. Scheidt, Org. Lett., 2009, 11, 1651 CrossRef CAS PubMed;
(p) A. J. A. Watson, A. C. Maxwell and J. M. J. Williams, Org. Lett., 2009, 11, 2667 CrossRef CAS PubMed;
(q) J. H. Dam, G. Osztrovszky, L. U. Nordstrøm and R. Madsen, Chem.–Eur. J., 2010, 16, 6820 CrossRef CAS PubMed;
(r) S. Muthaiah, S. C. Ghosh, J.-E. Jee, C. Chen, J. Zhang and S. H. Hong, J. Org. Chem., 2010, 75, 3002 CrossRef CAS PubMed.
- The aldol-type reactions using alcohols as starting materials have been achieved, though the products resulted in achiral or racemic:
(a) H. Shabany, E. Abel, J. P. Evans, I. M. McRobbie and G. W. Gokel, Tetrahedron Lett., 2000, 41, 6705 CrossRef CAS;
(b) K. Tanaka, S. Motomatsu, K. Koyama and K. Fukase, Tetrahedron Lett., 2008, 49, 2010 CrossRef CAS PubMed;
(c) K. Shimizu, R. Sato and A. Satsuma, Angew. Chem., Int. Ed., 2009, 48, 3982 CrossRef CAS PubMed;
(d) S. Kim, S. W. Bae, J. S. Lee and J. Park, Tetrahedron, 2009, 65, 1461 CrossRef CAS PubMed;
(e) A. Denichoux, T. Fukuyama, T. Doi, J. Horiguchi and I. Ryu, Org. Lett., 2010, 12, 1 CrossRef CAS PubMed;
(f) O. Kose and S. Saito, Org. Biomol. Chem., 2010, 8, 896 RSC;
(g) H. Fan, Y. Yang, J. Song, G. Ding, C. Wu, G. Yang and B. Han, Green Chem., 2014, 16, 600 RSC.
- K. Akagawa, S. Takigawa, E. Mano and K. Kudo, Tetrahedron Lett., 2011, 52, 770 CrossRef CAS PubMed.
-
(a) A. Itoh, S. Hashimoto, T. Kodama and Y. Masaki, Synlett, 2005, 2107 CrossRef CAS PubMed;
(b) A. Itoh, S. Hashimoto and Y. Masaki, Synlett, 2005, 2639 CrossRef CAS PubMed;
(c) S. Hirashima and A. Itoh, Synthesis, 2006, 1757 CAS;
(d) K. Kuwabara and A. Itoh, Synthesis, 2006, 1949 CAS;
(e) S. Hirashima, S. Hashimoto, Y. Masaki and A. Itoh, Tetrahedron, 2006, 62, 7887 CrossRef CAS PubMed;
(f) T. Sugai and A. Itoh, Tetrahedron Lett., 2007, 48, 2931 CrossRef CAS PubMed;
(g) T. Sugai and A. Itoh, Tetrahedron Lett., 2007, 48, 9096 CrossRef CAS PubMed;
(h) S. Hirashima and A. Itoh, Photochem. Photobiol. Sci., 2007, 6, 521 RSC;
(i) S. Hirashima and A. Itoh, Green Chem., 2007, 9, 318 RSC.
-
(a) L. Cui, N. Tada, H. Okubo, T. Miura and A. Itoh, Green Chem., 2011, 13, 2347 RSC;
(b) L. Cui, S. Furuhashi, Y. Tachikawa, N. Tada, T. Miura and A. Itoh, Tetrahedron Lett., 2013, 54, 162 CrossRef CAS PubMed;
(c) L. Cui, Y. Matsusaki, N. Tada, T. Miura, B. Uno and A. Itoh, Adv. Synth. Catal., 2013, 355, 2203 CrossRef CAS PubMed;
(d) Y. Shimada, K. Hattori, N. Tada, T. Miura and A. Itoh, Synthesis, 2013, 2684 CAS.
-
(a) T. Miura, Y. Yasaku, N. Koyata, Y. Murakami and N. Imai, Tetrahedron Lett., 2009, 50, 2632 CrossRef CAS PubMed;
(b) T. Miura, M. Ina, K. Imai, K. Nakashima, Y. Yasaku, N. Koyata, Y. Murakami, N. Imai, N. Tada and A. Itoh, Tetrahedron: Asymmetry, 2011, 22, 1028 CrossRef CAS PubMed;
(c) T. Miura, H. Kasuga, K. Imai, M. Ina, N. Tada, N. Imai and A. Itoh, Org. Biomol. Chem., 2012, 10, 2209 RSC.
Footnote |
† Electronic supplementary information (ESI) available: See DOI: 10.1039/c5ra05155j |
|
This journal is © The Royal Society of Chemistry 2015 |