DOI:
10.1039/C5RA04445F
(Paper)
RSC Adv., 2015,
5, 44204-44210
Block copolymer as a novel functional phase in an aqueous biphasic system for species selective iodine extraction†
Received
13th March 2015
, Accepted 11th May 2015
First published on 11th May 2015
Abstract
This article describes a newly designed block copolymer based aqueous biphasic system (ABS, composed of two immiscible aqueous phases) which is further utilized to study extraction behavior of three iodine species viz., I2, I−, and IO4−. Phase diagram and tie line data reveals the potential working range of this new biphasic system. Fluorescence, dynamic light scattering, transmission electron microscopy (TEM) and conductance data establish the micellar arrangement in solutions of this particular block copolymer. Elemental iodine is easily soluble in copolymer solution due to charge transfer complexation. Polymer phase of the ABS is found to have species dependent selectivity of extraction for the three different iodine species. Two non toxic, non flammable dyes 3,6-diamino-10-phenylphenazinium chloride (DPC) and haematoxylin are introduced for the first time to detect iodide and periodate species respectively in the copolymer medium.
Introduction
Aqueous biphasic systems (ABSs) are currently in the forefront of safer extraction alternatives as they provide multitude of mechanisms in the separation processes.1,2 As both phases in an ABS are strictly made up of water, it is regarded as environmentally benign alternative for conventional liquid/liquid (l/l) extractions. Practical applications of ABSs include separation and preconcentration of different solutes like cell membranes, micro organelles, proteins, DNA, dye molecules, ions etc.2–4 When a solute is added to the ABS, it may distribute unequally between the two phases or selectively retain in one phase. Thus, phase preference of a solute in an ABS depends on various factors for example ΔGhyd value, hydration environment, ionic radius, temperature, pH, etc.5 For practical purposes, it is essential to obtain the phase diagram data which define the potential operation range of such systems. Generally phase diagram represents a binodal curve, which divides a region of compositions that will form two immiscible aqueous phases from those that will form one phase. It also describes the unique property of a particular aqueous biphasic system under specific conditions of pH and temperature.6 ABSs made up of polymers have been widely studied7–9 though literature reported a small number of equilibrium data for tri block copolymer based ABSs.10–12 However, copolymers are attractive for a variety of reasons. Large solubility difference between hydrophobic and hydrophilic units in co polymeric solution helps to assemble novel core–shell type macroscopic aggregates where hydrophobic moieties are segregated from the aqueous exterior to form an inner core surrounded by the hydrophilic part.13 Amphiphilic character and low viscosity make copolymers more proficient matrices for separation and extraction studies.
Iodine plays a crucial role in living systems which is also largely dependent on its speciation. Iodine is an important ingredient in the generation of thyroid hormones, which regulates several biochemical reactions associated with growth and developments of reproductive systems, immune defense systems and many more biological processes.14 Additionally, being a disinfectant, iodine has been extensively used in antibacterial treatment, water processing, dairy industry and even in space flights.15–17 Different iodine species are responsible for their varied applications. Therefore, it is a difficult task to identify each iodine species, as iodine itself is a redox sensitive element in aqueous solutions. Literature survey reveals that speciation of iodine was studied using radiotracers 125I/129I.18,19 Extraction of different iodine species was also observed in ABS by earlier researchers.18,20 However, use of the phase forming component of ABS itself in speciation is still unexplored.
The present article is the first report on the formation of ABS by poly(propylene glycol)-block-poly(ethylene glycol)-block-poly(propylene glycol) (PPG-PEG-PPG) copolymer with sodium tartrate at room temperature. Stability of this ABS was found to be exceptionally high in a wide range of pH conditions. The binodal and the ternary phase diagrams were constructed for the ABS. Species dependent extraction and sensing of I2, I− and IO4− was achieved in the developed system. The sensing of I− was done with the aid of a non toxic, non flammable dye 3,6-diamino-10-phenylphenazinium chloride (DPC). DPC has reported complexation with various metal ions e.g. palladium, tin, mercury, silver, gold etc.21 Moreover, DPC was used to detect trace amount of DNA as a luminescent phosphorescence probe.22 It has never been used for I− detection. Haematoxylin is another compound occurring in logwood tree and is widely used to stain cell nuclei. It is a basic compound and binds with compounds containing negative charges like DNA and RNA.23 Haematoxylin was found sensitive towards periodate and it gives a characteristic absorbance peak suitable for qualitative and quantitative detection of periodate when treated with it. Here, we present our investigation on the extraction of different iodine species using the newly designed ABS.
Experimental
Materials
Polypropylene glycol-polyethylene glycol-polypropylene glycol copolymer (PPG-PEG-PPG #2000) (Sigma-Aldrich), iodine resublimed (Merck), sodium iodide (Merck), sodium meta periodate (Merck), 3,6-diamino-10-phenylphenazinium chloride (DPC) (George T. Gurr Ltd.), haematoxylin(7,11b-dihydroindeno[2,1-c]chromene-3,4,6a,9,10(6H)-pentol) (BDH) sodium tartrate and all other chemicals were of analytical grade and used as received.
Apparatus
Luminescence spectrometer LS-55B (Perkin-Elmer, USA) was used for fluorescence intensity measurement. Conductivity meter (JENWAY-4520) was used to measure the conductances. The UV visible spectra were recorded using U-3501 Hitachi spectrophotometer. Mettler Toledo seven compact pH/Ion meter S220 was used to measure and adjust the pH of different solutions. Hermle microprocessor controlled universal refrigerated high speed table top centrifuge (model Z 36 K) with an adjustable speed range of 200 to 30
000 rpm was used. A BOD incubator shaker NOVA model: SHCI 10(D) was used to maintain a constant temperature at 296 ± 0.5 K. A JEM 2100 transmitted electron microscopy (TEM) was used to get the image of the nanocomposite. Dynamic light scattering studies were performed using Malvern Instrument zetasizer nano.
Construction of the phase diagram
Phase diagram of this new copolymer-based ABS was constructed by the simple turbidimetric titration method.24,25 Aqueous solutions of PPG-PEG-PPG #2000 (30%) and Na-tartrate (1.2 M) were taken as the stock solutions to obtain the binodal curve. The detailed Experimental method is described as per our earlier reports.24,26 By convention, the component predominantly in the bottom phase was plotted in the abscissa (Na-tartrate for our system), and the component predominantly in the top phase was plotted as the ordinate (PPG-PEG-PPG #2000 for our system).
Tie-line determination
The experimental binodal curve was fitted in the following equation proposed by Merchuk et al. |
Y = A exp[(BX0.5) − (CX3)]
| (1) |
where, Y and X are the mass fraction of PPG-PEG-PPG and Na-tartrate respectively. A, B, and C are constants obtained by the regression of the experimental binodal data.
The tie-lines associated with the phase diagram were determined by gravimetric method.25 Different sets with ternary mixtures composed of PPG-PEG-PPG + Na-tartrate + water at the biphasic region were gravimetrically prepared and continuously agitated in the BOD shaker for complete distribution. They were centrifuged for 5 min at 4500 rpm at (296 ± 0.5) K, for a complete separation of the coexisting phases. Both phases were then carefully separated and weighed individually.
Tie lines were determined using the relationship between the top phase composition and the overall system composition by solving the four equations (eqn (2)–(5)) with four unknown values ([Poly]poly, [Poly]salt, [Salt]poly, and [Salt]salt):25
|
[Poly]poly = A exp[(B × [Salt]poly0.5) − (C × [Salt]poly3)]
| (2) |
|
[Poly]salt = A exp[(B × [Salt]salt0.5) − (C × [Salt]salt3)]
| (3) |
|
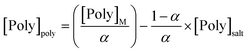 | (4) |
|
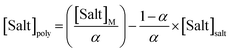 | (5) |
The subscripts “poly”, “salt”, and “M” designate the copolymer-rich phase, the salt-rich phase, and the mixture, respectively. [Poly] and [Salt] indicate respectively the weight fractions of PPG-PEG-PPG and sodium tartrate, and
α is the ratio between the mass of the top phase and the total mass of the mixture.
Each tie-line length (TLL) and slope of the tie-lines (STL) could be obtained using the following equation:26
|
 | (6) |
|
STL = (YT − YB)/(XT − XB)
| (7) |
where T, B, and M, represent the top phase, the bottom phase and the mixture, respectively; and
X and
Y represent the equilibrium weight fraction percentages of the phase forming components.
Characterisation of the micellar medium in block copolymer solution
Varying concentrations of PPG-PEG-PPG solution were prepared and their fluorescence emissions were recorded after excitation at 300 nm (which is also the λmax in its absorption spectrum). Conductance measurement of all these solutions was performed to find the critical micellar concentration. Platinum electrode was dipped into each solution and conductance values were measured. Dynamic light scattering experiment was performed using a 30% (v/v) solution of the block copolymer which helped to measure the hydrodynamic radius of the micelles and their size distribution in solution state. A small amount of the liquid sample was placed on a copper grid, dried under an IR lamp and taken for transmission electron microscopy for visualizing the micellar aggregates.
Detection and spectral analysis of I2, I− and IO4− species
Iodine granules were dissolved in PPG-PEG-PPG by using an ultrasonicator to make a 1 mM solution. For spectral detection of iodide, we have chosen DPC dye which itself has a purplish red color both in water and copolymer medium. DPC on interaction with I− turns orangish red which intensifies with progress of time. So, kinetic spectrophotometric studies were performed for detection of I− ions. Periodate was detected using the characteristic absorption peak at 450 nm in 5 mM haematoxylin solution prepared in 30% copolymer medium. From a 0.05 mM sodium periodate stock solution, increasing concentrations were added to the haematoxylin to find a suitable calibration in the range 1–6 μM of periodate. This calibration was used to analyse the copolymer rich phase of the ABS for periodate after extraction.
The kinetic spectrophotometric studies
For kinetic spectrophotometric studies with I− ions, 1 mM DPC solution was prepared in 30% (v/v) PPG-PEG-PPG medium. When NaI solution of varying concentrations were added to this solution, increasing absorption intensity at 360 nm were observed over a short interval of time. Increase in the absorbance was recorded in the time scan mode to measure the values at regular time intervals. Several such kinetic runs have been carried out at a fixed concentration of DPC with varied concentrations of iodide. From time-scan data, we are able to know the rate of the reaction with respect to concentration of iodide present in unknown sample solution.
Aqueous biphasic extraction studies with I2/I−/IO4− species
For aqueous biphasic extraction of I2, I− and IO4− species, the ABS was prepared by mixing 3 mL of PPG-PEG-PPG #2000 (30% v/v solution) and 3 mL of sodium tartrate (1.2 M) in 15 mL graduated test tubes. Solutions were then shaken for 10–15 minutes. After centrifugation for 10 minutes at 4500 rpm, this copolymer/salt system was found to form equal volume phase separation at room temperature. This solution was used for biphasic extraction studies using different pH of sodium tartrate to find the optimum condition. To study the pH dependent extraction of I2, 3 mL 30% (v/v) solution of PPG-PEG-PPG containing 1 mM iodine was shaken with the same sodium tartrate solution adjusted to different pH. For the extraction of I− and IO4−, 0.2 mL each of 1 mM sodium iodide and 1 mM sodium periodate were added to the ABSs prepared under varying pH conditions. For analyzing the I− content of the polymer rich phase, it was treated with DPC as discussed earlier and the absorption kinetics of the complex was measured at 530 nm. For estimating the IO4−, the polymer rich phase was treated with haematoxylin and the extraction profiles were monitored spectrophotometrically using the complexation at 450 nm.
Results and discussion
The block copolymer forms micellar arrangement in aqueous solution which is supported by its fluorescence intensity, dynamic light scattering experiments and TEM images. Conductance measurements were performed to find the critical micellar concentration.27 Fig. 1 shows the emission spectra of copolymer solutions which show a gradual increase in intensity with increasing concentrations. This is because of increased micellar concentration which results in increased electron density and hence increased fluorescence generation sites.28,29 Fig. 2 shows the conductance values of copolymer solutions at 25 ± 0.5 °C. A break near the concentration 1.5% (v/v) indicates the CMC of the solution. The observed conductance values are very low (in the μS range) as the conductance arises due to polar head groups and not by the actual ions.27 Micellar aggregation results in the formation of electron dense regions in solution which accounts for the observed increase in conductance. Dynamic light scattering experiment reveals that the hydrodynamic radius of the micelles lies in 1–2 nm range (Fig. 3). TEM image of a drop of this solution further strengthens this fact as small dark spots (∼1–2 nm) can be seen in the polymeric matrix arising out of the micellar distribution (Fig. 4).
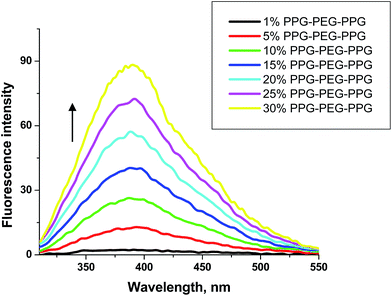 |
| Fig. 1 Room temperature fluorescence emission spectra of copolymer solution with increasing concentrations (λex = 300 nm). | |
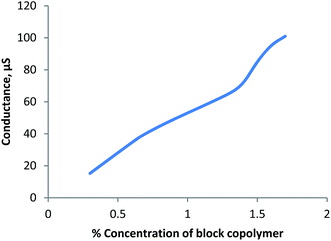 |
| Fig. 2 Conductance vs. concentration plot for copolymer solution at 25 ± 0.5 °C. | |
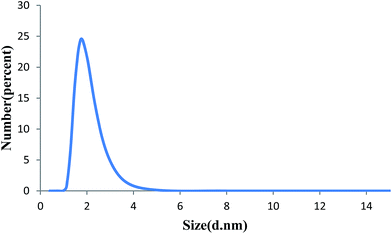 |
| Fig. 3 Micellar size distribution as observed in dynamic light scattering experiment. | |
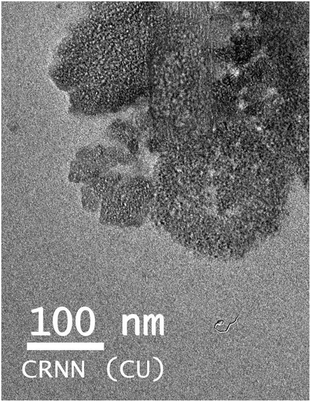 |
| Fig. 4 Transmission electron microscopic image of 3% PPG-PEG-PPG (v/v) block copolymer solution showing ∼1 nm sized micellar distribution. | |
The phase diagram and the tie lines
The phase behavior of the newly designed ABS comprising of PPG-PEG-PPG and sodium tartrate system was established with the help of phase diagram and tie line data. The phase diagram of PPG-PEG-PPG was obtained at 296 ± 0.5 K (Fig. 5) and the details of system compositions and final compositions are tabulated in Table 1. The area above the binodal curve indicates the existence of two distinct phases whereas the area on and below the curve indicates a homogenous single phase. The ternary phase diagrams composed by PPG-PEG-PPG + water + Na-tartrate along with the respective tie lines are shown in Fig. 6.
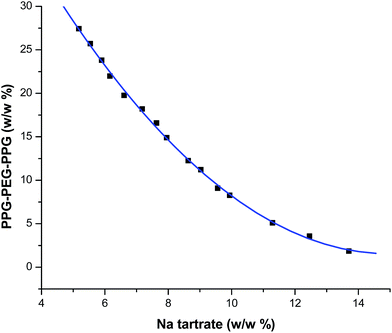 |
| Fig. 5 Phase diagram of PPG-PEG-PPG and sodium tartrate aqueous biphasic system drawn at 296 ± 0.5 K. | |
Table 1 Experimental (liquid + liquid) equilibrium mass fractions (binodal curve data) for the system sodium tartrate (x1) + PPG-PEG-PPG (x2) in water at temperature T = 296 ± 0.5 K, pressure p = 0.1 MPa
System composition |
Final composition |
x1 |
x2 |
x1 |
x2 |
5.52 |
28 |
5.18 |
27.44 |
5.80 |
27 |
5.54 |
25.71 |
6.07 |
25 |
5.90 |
23.81 |
6.44 |
23 |
6.16 |
21.98 |
7.36 |
22 |
6.61 |
19.76 |
8.28 |
21 |
7.18 |
18.20 |
9.20 |
20 |
7.63 |
16.58 |
10.12 |
19 |
7.95 |
14.90 |
11.96 |
17 |
8.63 |
12.26 |
12.89 |
16 |
9.03 |
11.22 |
14.72 |
14 |
9.56 |
9.09 |
15.65 |
13 |
9.94 |
8.26 |
16.57 |
12 |
11.29 |
5.10 |
17.49 |
11 |
12.46 |
3.57 |
18.41 |
9 |
13.70 |
1.84 |
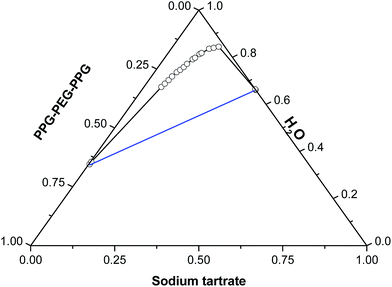 |
| Fig. 6 Ternary phase diagram of water–PPG-PEG-PPG–Na-tartrate system drawn at 296 ± 0.5 K. Weight fractions of Na-tartrate, water and PPG-PEG-PPG are shown in the three coordinates. | |
We get tie-lines by connecting two nodes on the binodal curve and each point on the tie line defines the final concentrations of phase components in the top and bottom phases. As the tie-line length increases, the top phase becomes more concentrated with the copolymer PPG-PEG-PPG and the bottom phase becomes richer with the salt. Table S1† represents the compositions of PPG-PEG-PPG and Na-tartrate in the top and the bottom phases respectively along with the tie-line lengths (TLL) and slope of tie lines (STL). The fitting parameters of the binodal curve are tabulated in Table S2.†
Detection and spectral analysis of the three iodine species
I2: Iodine shows brown color after dissolution in PPG-PEG-PPG. Absorbance of copolymer dissolved I2 solutions were measured for calibration by UV-visible spectrometer and were found to have three λmax values at 227 nm, 290 nm and 370 nm (Fig. S1†). This brown color indicates charge transfer complex formation between iodine and the polymeric entity which is also in agreement with earlier observations.27 As iodine is an electron acceptor, it can easily accept lone pair of electrons from oxygen atoms of the copolymer resulting in complexation; this type of complexation was used to determine CMC value of nonionic surfactants by several researchers.30–32 In fact, the tendency of the otherwise water insoluble I2 to dissolve in PPG-PEG-PPG is partially attributed to this aforesaid complexation.
I−: DPC is freely soluble in water as well as in PPG-PEG-PPG. For spectral detection of iodide, we have chosen DPC dye which itself shows absorption peak at 530 nm in both water and copolymer medium. However no spectral evidence of DPC–I− interaction was observed in water medium. In the micellar medium of the block copolymer, DPC interacts with the large iodide ions. As a result a new peak appears at 360 nm (Fig. S2†). The negative charge of I− spreads all over the micellar entity. This results in reduced charge density and increased polarizability of the complex species having van der Waals forces of attraction.33 A steady increase in the absorbance value at 360 nm is observed with time for a particular concentration range of iodide which corresponds to the π → π* transition arising out of a large π electron density in the complex entity that increases with time as association progresses. The increasing absorbance of the of DPC–I− complex prepared in copolymer solution with different I− concentrations give different slopes (Fig. 7). Using the kinetic spectrophotometric methods, a plot of log
V vs. log C would give the rate constant and the order of the reaction following two eqn (8) and (9) (ref. 3)
where
V is the reaction rate (slope),
k is the rate constant,
C is the concentration of iodide and
n is the order of the reaction. The logarithmic form of equation may be written as:
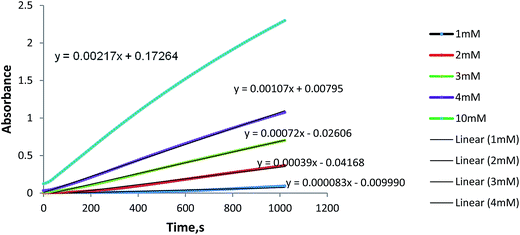 |
| Fig. 7 Absorbance vs. time plot for the reaction of different concentrations of iodide with DPC in PPG-PEG-PPG medium. | |
A plot of log
V vs. log
C gives a slope (Fig. 8) which is the order of the reaction and is 1.412 (∼1) and the rate constant is 1.173 s−1. This value would help to find the unknown concentration of iodide, when treated with DPC in polymeric solution.
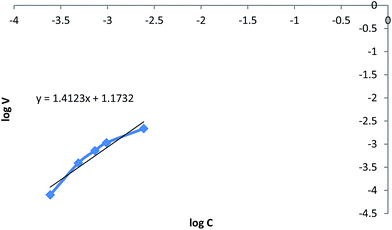 |
| Fig. 8 log V vs. log C plot to obtain the value of rate constant and order of the DPC–I− reaction. | |
IO4−: The haematoxylin dye shows an absorption peak at a λmax of 450 nm. Upon treatment with increasing concentrations of IO4− the intensity of this particular peak increases gradually in block copolymer medium (Fig. S3†). This is because of probable interaction between the two moieties that increases the electron density responsible for visible light absorption. A calibration plot was obtained for periodate in the concentration range 1–7 μM and used for obtaining the concentration of unknown periodate after extraction in ABS.
Aqueous biphasic extraction studies with I2/I−/IO4− species
The extraction of the three species of iodine viz., I2, I− and IO4− in ABS were monitored at varying pH conditions of the salt rich phase. The unknown concentrations of these iodine species in the polymer rich phase of the ABS could be easily obtained for I2 and IO4− directly from the calibration plot. The concentration of iodide extracted in polymer rich phase of ABS was calculated from the absorption kinetics of the solution (Fig. 9) using the log
V vs. log
C plot. Elemental iodine was 100% extracted in the PPG-PEG-PPG phase of ABS. Even from different pH of salt rich phase (pH 4.5 to 9.5) the results of extraction were same. Retention of iodine in polymer phase is due to charge transfer complexation between iodine and oxygen atom which is already mentioned earlier. Fig. 10 represents overall extraction pattern of all the three iodine species in one plot. It is clearly observed that I2 species is extractable at all pH conditions of the developed ABS. I− is extracted to certain extent (60%) from pH 4.5 of salt rich phase. Sodium tartrate forms a slightly basic solution upon dissolution in water. At lower pH (∼4) there is change in the ionic concentrations of tartrate as there is more of tartaric acid in the medium resulting in a change in the electronic and hydration environment of I−. This causes a portion of the iodide ions to get partitioned in the polymer rich phase at lower pH. IO4− has the tendency to remain in the salt rich phase at all the pH conditions studied. Periodate being an oxy anion and a strong oxidizing agent is liable to cause destruction in the micellar structure and prefers to remain in the unorganized salt rich phase and consequently its extraction is in the polymer phase is nullified.
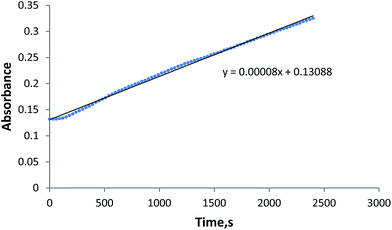 |
| Fig. 9 Absorbance vs. time curves for the reaction of extracted iodide (in PPG-PEG-PPG phase at pH ∼ 4) with PSF. | |
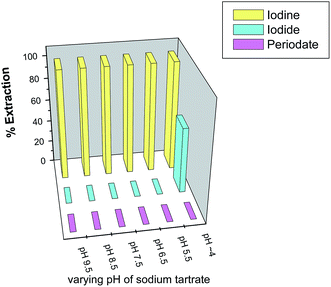 |
| Fig. 10 Extraction of elemental iodine, iodate and periodate in PPG-PEG-PPG rich phase from varying pH of sodium tartrate phases. | |
Conclusion
PPG-PEG-PPG block copolymer was found to form an ABS with sodium tartrate whose phase diagram has been constructed at 296 ± 0.5 K. This copolymer based ABS is an environmentally benign and simple technique and has unique applications in extraction studies. It can efficiently extract iodine and iodide in polymer phase but not periodate. Therefore polymeric phase of this new ABS can also be a novel matrix towards species selectivity of iodine.
Acknowledgements
The authors gratefully acknowledge the generous gift of the dyes used for detection by Prof. S. K. Kar, (Department of Chemistry, University of Calcutta). P. S. expresses sincere thanks to the UGC, India [Memo no. UGC/1228C/Major Research (SC) 2012] for providing necessary fellowship. A. C. gratefully thanks DST Fast Track (Fast Track Sanction no. SR/FT/CS-105/2011) for funding. We are thankful to Dr Abhijit Saha, UGC-DAE Consortium for Scientific Research, Kolkata, India for DLS experiment. We also thank Ms. Urmila Goswami, Centre for Research in Nanoscience and Nanotechnology, University of Calcutta, India, for obtaining TEM images.
References
- M. Mirsiaghi, G. Pazuki, M. Vossoughi and I. Alemzadeh, J. Chem. Eng. Data, 2010, 55, 3005–3009 CrossRef CAS.
- A. Frerix, M. Schonewald, P. Geilenkirchen, M. Muller, M. R. Kula and J. Hubbuch, Langmuir, 2006, 22, 4282–4290 CrossRef CAS PubMed.
- P. Samaddar and K. Sen, RSC Adv., 2013, 3, 20750–20757 RSC.
- A. B. Mageste, L. R. de Lemos, G. M. D. Ferreira, M. C. H. da Silva, L. H. M. da Silva, R. C. F. Bonomo and L. A. Minim, J. Chromatogr. A, 2009, 1216, 7623–7629 CrossRef CAS PubMed.
- I. Regupathi, S. Murugesan, R. Govindarajan, S. P. Amaresh and M. Thanapalan, J. Chem. Eng. Data, 2009, 54, 1094–1097 CrossRef CAS.
- J. Han, Y. Wang, Y. F. Li, C. L. Yu and Y. S. Yan, J. Chem. Eng. Data, 2011, 56, 3679 CrossRef CAS.
- R. S. Diniz, E. C. Souza Jr, J. S. R. Coimbra, E. B. de Oliveira and A. R. da Costa, J. Chem. Eng. Data, 2012, 57, 280–283 CrossRef CAS.
- S. C. Silveério, A. Wegrzyn, E. Lladosa, O. Rodríguez and E. A. Macedo, J. Chem. Eng. Data, 2012, 57, 1203–1208 CrossRef.
- J. G. Huddleston, H. D. Willauer and R. D. Rogers, J. Chem. Eng. Data, 2003, 48, 1230–1236 CrossRef CAS.
- L. S. Virtuoso, K. A. S. F. Vello, A. A. de Oliveira, C. M. Junqueira, A. F. Mesquita, N. H. T. Lemes, R. M. M. de Carvalho, M. C. H. da Silva and L. H. M. da Silva, J. Chem. Eng. Data, 2012, 57, 462–468 CrossRef CAS.
- L. R. de Lemos, I. J. B. Santos, G. D. Rodrigues, G. M. D. Ferreira, L. H. M. da Silva, M. D. C. H. da Silva and R. M. M. de Carvalho, J. Chem. Eng. Data, 2010, 55, 1193–1199 CrossRef.
- G. D. Rodrigues, M. D. C. H. da Silva, L. H. M. da Silva, L. da Silva Teixeira and V. M. de Andrade, J. Chem. Eng. Data, 2009, 54, 1894–1898 CrossRef.
- K. Kataokaa, A. Haradaa and Y. Nagasaki, Adv. Drug Delivery Rev., 2001, 47, 113–131 CrossRef.
- A. M. Pineiro, V. R. Hortas and P. B. Barrera, J. Anal. At. Spectrom., 2011, 26, 2107–2152 RSC.
- B. S. Hetzel and J. T. Dunn, Annu. Rev. Nutr., 1989, 9, 21–38 CrossRef CAS PubMed.
- K. L. Cargill, B. H. Pyle, R. L. Sauer and G. A. McFeters, Can. J. Microbiol., 1992, 38, 423–429 CrossRef CAS.
- S. He, B. Wang, H. Chen, C. Tang and Y. Feng, ACS Appl. Mater. Interfaces, 2012, 4, 2116–2123 CAS.
- S. T. Griffin, S. K. Spear and R. D. Rogers, J. Chromatogr. B: Anal. Technol. Biomed. Life Sci., 2004, 807, 151–156 CrossRef CAS PubMed.
- X. Houa, V. Hansena, A. Aldahanb, G. Possnertc, O. C. Lindd and G. Lujanienee, Anal. Chim. Acta, 2009, 632, 181–196 CrossRef PubMed.
- T. A. Graber, H. Medina, H. R. Galleguillos and M. E. Taboada, J. Chem. Eng. Data, 2007, 52, 1262–1267 CrossRef CAS.
- K. M. M. Krishna Prasad and K. P. Subhashchandran, Asian J. Chem., 1992, 4, 715–725 Search PubMed.
- J. M. Liu, X. M. Huang, Z. B. Liu, F. M. Li, L. P. Lin, X. X. Wang, C. Q. Lin, Y. H. Huang, Z. M. Li and S. Q. Lin, J. Fluoresc., 2011, 21, 195–202 CrossRef CAS PubMed.
- D. P. Jackson, S. Bell, J. Payne, F. A. Lewis, J. Sutton, G. R. Taylor and P. Quirke, Nucleic Acids Res., 1989, 17, 10134 CrossRef CAS PubMed.
- A. Chakraborty and K. Sen, J. Chem. Eng. Data, 2014, 59, 1288–1294 CrossRef CAS.
- R. Hatti-Kaul, Methods in Biotechnology: Aqueous Two Phase Systems, Methods and Protocol, Humana Press, Totowa, NJ, 2000, p. 11 Search PubMed.
- A. Chakraborty and K. Sen, RSC Adv., 2014, 4, 64328–64335 RSC.
- S. P. Paik, S. K. Ghatak, D. Dey and K. Sen, Anal. Chem., 2012, 84, 7555–7561 CrossRef CAS PubMed.
- K. Sen and P. Samaddar, J. Mol. Liq., 2014, 200, 369–373 CrossRef CAS PubMed.
- M. Schuch, G. A. Gross and J. M. Köhler, Sensors, 2007, 7, 2499–2509 CrossRef CAS PubMed.
- L. Dai, J. Phys. Chem., 1992, 96, 6469–6471 CrossRef CAS.
- S. K. Hait and S. P. Moulik, J. Surfactants Deterg., 2001, 4, 303–309 CrossRef CAS PubMed.
- M. J. Schick, Nonionic Surfactants: Physical Chemistry, Marcel Dekker, New York, 1987 Search PubMed.
- D. S. Pellosi, B. M. Estevão, J. Semensato, D. Severino, M. S. Baptista, M. J. Politi, N. Hioka and W. Caetano, J. Photochem. Photobiol., A, 2012, 247, 8–15 CrossRef CAS PubMed.
Footnote |
† Electronic supplementary information (ESI) available. See DOI: 10.1039/c5ra04445f |
|
This journal is © The Royal Society of Chemistry 2015 |
Click here to see how this site uses Cookies. View our privacy policy here.