DOI:
10.1039/C5RA04270D
(Paper)
RSC Adv., 2015,
5, 40700-40707
Development of a novel drug-eluting stent consisting of an abluminal and luminal coating layer dual therapy system
Received
11th March 2015
, Accepted 20th April 2015
First published on 20th April 2015
Abstract
The aim of this study was to develop a dual drug-coated stent using a bi-directional coating system. Sirolimus (SRL) was coated onto the abluminal area of the stent to prevent restenosis, and WKYMVm, a peptide for endothelial homing, was coated onto the luminal area of the stent to enhance endothelialization. To verify the bidirectional coating of materials, various morphological analysis was carried out by using optical microscopy, scanning electron microscopy, and fluorescence microscopy. The release velocities of the drugs coated onto the luminal and abluminal surfaces of the stent were investigated by using instruments that mimic the body’s circulation system. The proliferation of smooth muscle cells was inhibited by SRL, whereas the proliferation of human umbilical vein endothelial cells was enhanced by WKYMVm. This study demonstrated that it is feasible to separate coating layers of the stent strut with new coating technology for the bi-directional function of drugs.
1. Introduction
Cardiovascular disease is a leading cause of death globally and the ratio increases each year in a dramatic way.1 Coronary artery stenosis can be treated with coronary artery bypass surgery or angioplasty.2 But, treatment using angioplasty can cause restenosis (recurrence of stenosis) within 6 months after the procedure.3 Restenosis is believed to include vascular smooth muscle cells. Over the last decade, coronary stents have revolutionized the treatment of cardiovascular disease.4 Stents have been commonly used to prevent restenosis.5 Stents generally have open tubular structures and can be either balloon-expandable or self-expandable.
Stents have become increasingly important to restore the function of the cardiovascular system. Stent-based local drug release at the site of cardiovascular injury via a polymer coated stent is an attractive therapeutic method to achieve an effective local concentration of the drug for a desired period of time.6 However, stents may stimulate foreign body reactions that result in thrombosis or restenosis. Residues of the coated polymers left after the complete elution of a drug can trigger significant thrombogenic risk and are accountable for the subsequent hypersensitivity and inflammation.7 To avoid these complications, a variety of coating strategies were applied on stents, such as different coating methods and variations in coating materials like with drugs, proteins, antibodies, growth factors etc. Such a method has been proposed in this study to overcome the drawbacks associated with stent coating.
Instead of completely disqualifying the use of polymeric coatings on stents, the best way to increase the overall hemo-compatibility of the stent is by enriching the stent surface by coating it with inert or bioactive components. Non-thrombogenic coatings that may aid re-endothelialization were an encouraging alternative to conventional drug-eluting stents. Stent thrombus formation is mostly caused by stent surface hydrophobicity, morphology, roughness, and electrical charge which are triggered by the adsorption of plasma proteins.8 Low-molecular weight heparin (LMWH) immobilized stent surfaces were coated with sirolimus for improved thrombo-resistance. A drug-eluting stent (DES) with a LMWH layer showed a 90% reduction in platelet deposition during an in vitro test.9
The first generation anti-proliferative coated stents, paclitaxel and sirolimus (SRL), inhibited the vascular smooth muscle cell (SMC) proliferation via cell cycle targeting.10 SRL arrests the cell cycle at the G1 phase by mTOR transduction disruption. Sirolimus was found to be a potent and immunosuppressive agent against SMCs, and blocks cell division that can lead to an arrest in the G1 interface. The antiproliferative effect of SRL has been used to prevent restenosis in DESs.11,12
Phosphorylcholine (PC), a naturally occurring lipid group for biocompatibility in blood, is a part of the coating used for zotarolimus-eluting Endeavor® stents. However, PC-coated stents have not shown promising results despite their favorable characteristics such as biocompatibility, non-allergenicity, as well as a lower inflammatory response. In fact, except stents coated with anti-proliferative drugs, there has been no significant clinical success in the area of favorable stent coatings.13 In a study, anti-human CD133-antibody and glycoprotein expressed on endothelial-regeneration cells, were covalently coated onto a stent surface. This stent just achieved effective binding of CD133-positive cells in vitro.14 In fact, performances of DESs need to increase the direct cellular effect in re-endothelialization and vessel wall healing.
WKYMVm (Trp–Lys–Met–Val–D-Met–NH2) (WPP) is a synthetic peptide that stimulates angiogenesis of endothelial cells, proliferation, migration, and formation of active healing processes. To overcome the cardiovascular system, therapeutic compounds such as SRL and WPP must be efficiently delivered to the cardiovascular system. The safety and efficacy of such an approach critically depends on the delicate combination of drug, polymer, and the kinetics of release.15
In this study, we introduced stents with abluminal and luminal surfaces coated with different coating materials containing drugs to avoid restenosis and enhance re-endothelialization (Fig. 1). Here, we present a novel ultrasonic spraying dual coating system (fabricated by own system), in which two different drug solutions are used for making the abluminal and luminal surface coated stent, using two spray nozzles with separate drug solution syringe pumps. Our set-up is different than the reported manual ultrasonic spray system set-ups because we use a robot-controlled movable multi-armed system. We evaluated the present set-up by checking the changed surface of the coated stents and their effect (dual coated stent) on the cumulative amount of drug release.
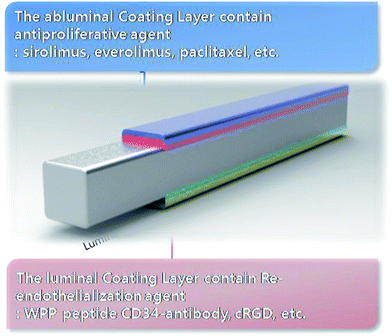 |
| Fig. 1 Schematic design of abluminal and luminal coated stents: the abluminal surface of the stent elutes the antiproliferative agent and the luminal surface of the stent elutes the re-endothelialization agent. | |
2. Results and discussion
2.1. Identification of coating method on the abluminal and luminal surface of the stent strut
We prepared a DES using an ultrasonic spray coating method with the newly developed device shown in our schematic coating design (Fig. 1). The OM images show that the luminal stent coating has a bright violet color on the luminal surface of the stent (Fig. 2(a-1) and (a-2)), while the abluminal surface was not a bright color. On the contrary, the abluminal coating stent was a bright color on the abluminal surface of the stent without the luminal coating layers (Fig. 2(b-1) and (b-2)). The exchangeable drug positioning of the polymer and drug and simultaneous coating to the luminal and abluminal surfaces were investigated for DESs with an abluminal coating (DAC), DESs with a luminal coating (DLC), and DESs with an abluminal and luminal coating (DALC), respectively. As shown in Fig. 3, the conventional morphologies of the metal and polymer were observed in the abluminal and luminal areas of the stent, respectively. It showed that the coating layer was separated between the luminal and abluminal areas on the stent surface according to the process. Fig. 4 shows the fluorescence microscopy (FM) images of the abluminal or luminal coated layers. The images also showed the uniform dispersion of FITC-labeled fluorescent WPP. These results confirmed that the stent had been coated only on the luminal surface and not on the abluminal surface of the stent. On the contrary, the abluminal coated stent showed fluorescence on the abluminal surface of the stent compared to the luminal surface of stent.
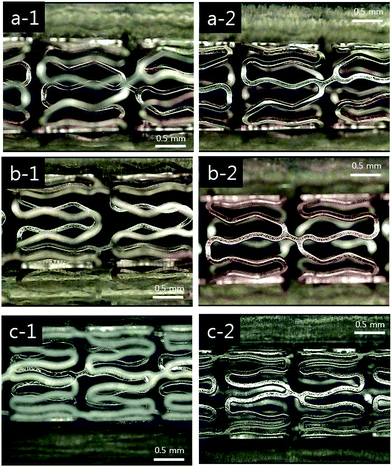 |
| Fig. 2 Optical microscopy images of (a-1) & (a-2): a luminal side coated stent ((a-1) luminal side focused and (a-2) abluminal side focused respectively), (b-1) & (b-2): an abluminal side coated stent ((b-1) luminal side focused and (b-2) abluminal side focused respectively), (c-1) & (c-2): an abluminal and luminal side coated stent ((c-1) luminal side focused and (c-2) abluminal side focused respectively). The microscope has a magnification of 5×. | |
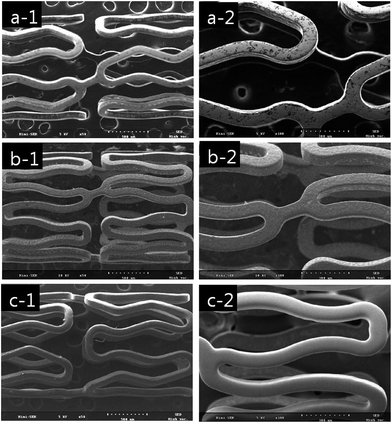 |
| Fig. 3 Scanning electron microscopy analyses of a luminal side coated stent ((a-1) & (a-2)), an abluminal side coated stent ((b-1) & (b-2)), an abluminal and luminal side coated stent ((c-1) & (c-2)). The microscope has a magnification of 50× (a-1, b-1 & c-1) and 100× (a-2, b-2 & c-2). | |
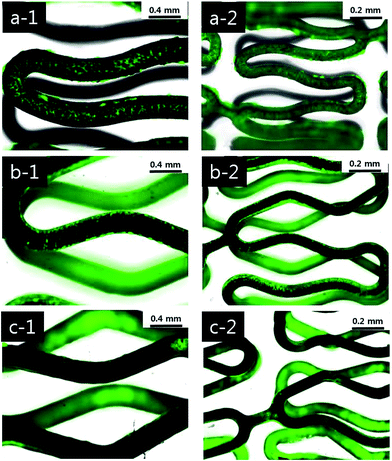 |
| Fig. 4 Fluorescence microscopy images of a luminal side coated stent ((a-1) & (a-2)), an abluminal side coated stent ((b-1) & (b-2)), an abluminal and luminal side coating stent ((c-1) & (c-2)). The microscope has a magnification of 10× (a-2, b-2 & c-2) and 20× (a-1, b-1 & c-1). | |
General DESs with a circumferential coating have been shown to delay re-endothelialization and induce in-stent thrombosis during arterial repair.16 To overcome this limitation, control over the positioning of the drug coating to the luminal or abluminal areas is required. According to this study, in-stent restenosis and thrombosis may be prevented by coating the abluminal surface of the stent strut with a drug to prevent restenosis and coating the luminal surface of the stent strut with an endothelialization accelerating drug. Fig. 5a shows that the coating thickness of the abluminal coating layer (9.5 ± 0.95 μm) and the coating thickness of the luminal coating layer (4.4 ± 0.82 μm). The concentration of the drug and thickness were higher in the abluminal area when compared to the luminal area. This is because of the different coating methods along with the coating position of the drug. For the abluminal coating, a top-to-bottom coating system was employed. Whereas, for the luminal coating, the nozzle was located beside the stent and the drug was sprayed through a reflecting plate. Therefore, more drug loss to the inner cavity side took place when coating the luminal surface. Fig. 5(b-1) shows the atomic force microscopy (AFM) image of the surface roughness on the luminal layer (Ra: 6593 nm) and Fig. 5(b-2) shows the AFM image of the surface roughness on the abluminal layer (Ra: 15.888 nm). The value of the abluminal surface roughness was lower than that of the luminal surface.
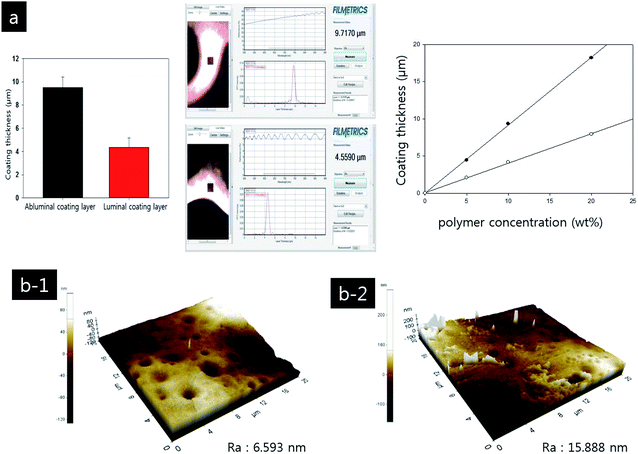 |
| Fig. 5 Coating thickness analyses (a) and AFM tapping mode images (b) of DALC (abluminal coating layer (●) luminal coating layer (○)). | |
2.2. Determination of drug loading and in vitro release
Referring to the stent surface, the SRL loading per mm2 stent surface was 1.4 ± 0.03 μg in the abluminal coating layer, and the WPP loading per mm2 stent surface was 1.0 ± 0.05 μg in the luminal coating layer. The in vitro drug release kinetics were also determined by HPLC for 32 days at regular intervals.
The release profile of WPP and SRL from the DLC (Fig. 6a), DAC (Fig. 6b), and DALC (Fig. 6c) are shown in Fig. 6. The drug release was faster in the luminal area compared to the abluminal area. An initial burst release of WPP of more than 30% was observed within the first day in the luminal coating layer, whereas no apparent burst release of SRL was observed. Only a slow release was observed within the first day from the abluminal coating layer of the DESs. After 8 days, the cumulative release percentage of WPP release from the stent was approximately 80% and was continuous for 32 days. In contrast, the cumulative percentage of SRL released from the stent was significantly slower. In vitro drug release from the DESs broadly exhibited a sustained release during the test period.
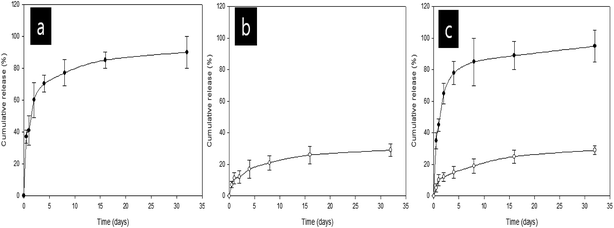 |
| Fig. 6 Cumulative amount of released sirolimus (○) and WPP (●) from the DLC (a), DAC (b), and DALC (c). | |
The hydrophilic peptide of WPP is readily distributed and conveyed in tissues, but is rapidly cleared in the absence of tissue. Hydrophobic SRL, on the other hand, is more often subject to association or dissociation resulting in sustained localization close to the delivery source. This drug binding slowed transport, leading to high concentrations of drug localized near the site of application. This excessive tissue SRL concentration, in fact, exceeds the driving concentration. In contrast, a hydrophilic drug such as WPP would have lower tissue levels than those of the driving medium, resulting in a low volume of arterial distribution. Hydrophobic drugs displayed a more heterogeneous local tissue concentration than hydrophilic drugs, but achieved higher mean concentrations. Hydrophobic drugs also tended to remain closer to the intima following luminal release, while hydrophilic drugs achieved higher concentrations in deeper layers.17
The commercially available sirolimus-eluting stent releases its drug in a similar pattern over several weeks and the stent is completely free from sirolimus within 45–60 days.11 For the DLC, DAC, and DALC, the drug was released slowly for 32 days. The release velocity of SRL and WPP in the DLC was similar to the DALC. These results suggest that the coating method for distinguishing the luminal and abluminal areas does not affect the drug release and the coating thickness of the other area. The drug and polymer were mixed simply with identical solvents and coated the surface. Thus, as the coating thickness increased gradually, so the amount of drug and polymer was also increased. In addition, the drug release velocity was also delayed with increasing drug amounts (Fig. 5a).
2.3. Assay of smooth muscle cell and endothelial cell proliferation
The results showed that HUVEC viability after 7 days was 80%, 170% and 153% for the DAC, DLC and DALC, respectively. The groups with WPP increased cell proliferation (Fig. 7). During the treatment of the DALC in SMCs, the cell viability showed no change when compared to the DLC treatment group. WPP had absolutely no effect on the SMC viability. The in vitro experiments indicated that SRL inhibited SMC proliferation in a concentration-dependent manner, SRL (<0.1 mM). WPP did not affect the SMC proliferation compared with the control, but WPP affected the HUVEC proliferation. WPP was also indicated as a potent and highly efficacious agent for the HUVECs. The DALC showed inhibition towards SMC proliferation and proliferation of the HUVECs. The results would be beneficial in decreasing restenosis and improving the healing of the stent covered endothelial cells in the process of vascular wall healing.
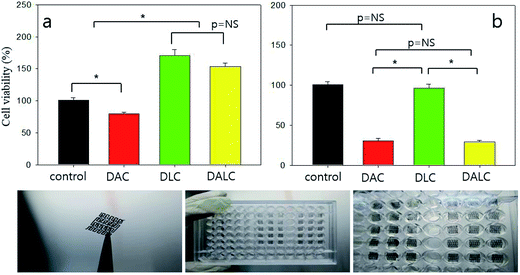 |
| Fig. 7 Cell viability against HUVECs with (a) A10 cells, (b) an abluminal side coated stent with sirolimus (DAC), a luminal side coated stent with WPP (DLC), and an abluminal and luminal coated stent with sirolimus and WPP (DALC) after 3 days. Five experiments (N = 5) were performed for each concentration per drug. | |
2.4. In vivo study
Fig. 8 shows the lumen surface of a rabbit iliac artery at 14 days and the results showed that the DLC, DAC and DALC covered the surface of stent. An enhancement of strut coverage ratio in the DALC group (77.5%) was higher than that of DAC group (50.5%). However, the coverage ratio of DALC group is quite similar to DLC group (78.1%). It doesn’t show fibrin deposition on the stent for all groups without thrombosis. This results might suggest that the luminal surface with WPP accelerates the healing process for the artery. When compared with other stents, the stent coverage ratio was reported to be 53.5% for a bare metal stent (BMS) in the rabbit iliac artery after 28 days.18 Percutaneous transluminal coronary angioplasty (PTCA)-containing DESs for maintaining an expanded vessel wall and continuous drug elution to suppress the proliferation of neointima layers were done more effectively by suppressing proliferation and movement of smooth muscle cells. However, for arterial healing, a surgery using a DES may induce more serious disorders than the BMS. Furthermore, since endothelialization occurs slowly, the DES induces in-stent thrombosis more frequently than the BMS.10 According to the results, arterial healing showed an obvious difference on the luminal surface.
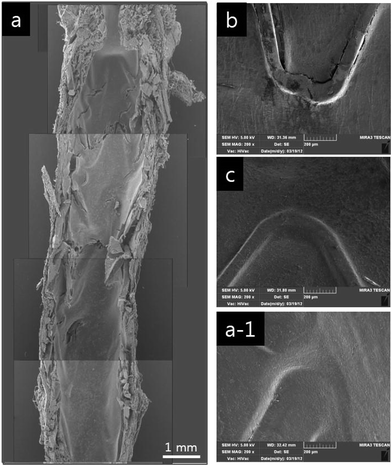 |
| Fig. 8 Representative SEM images of harvested arteries on stents for 14 days post-implantation ((a), (a-1): DALC, (b): DAC, and (c): DLC). | |
3. Experimental
3.1. Materials
Poly[d,L-lactide-co-glycolide] (PLGA, 50
:
50, Mw = 48 kDa) was purchased from EVONIK (UK), Sirolimus was purchased from LC Laboratories (USA) and the WKYMVm peptide (WPP) was purchased from Anygen (Korea). Nile red, phosphate-buffer saline (PBS), 3-(4,5-dimethyl-2-thiazolyl)-2,5-diphenyl-2H-tetrazolium bromide (MTT) and molecular porous dialysis membrane bags (MWCO: 3500 Da) were from Sigma-Aldrich (St. Louise, Mo, USA). All other chemicals and solvents were of analytical or reagent grade and used without further purification.
3.2. Novel ultrasonic coating system development
A novel ultrasonic coating system was set up using the design shown in Fig. 9. It was composed of two separate ultrasonic nozzles with diameters of 0.51 mm (CERATORQ Corp. Korea), two 10 mL plastic syringes, and a robotic system that moves laterally, controlled by a LabVIEW 2013 program (National Instruments, USA). The whole setup was placed in a sealed chamber with an automatic exhaust system, dehumidifier and heating system.
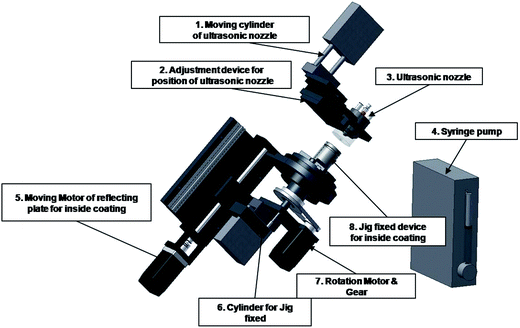 |
| Fig. 9 3D schematic representation of the novel dual ultrasonic spray coating system. (1) Moving cylinder for the ultrasonic nozzle position movement. (2) Adjustment device that is able to change the position of the ultrasonic nozzle. (3) Ultrasonic nozzle: the device for spraying a coating solution onto the surface of the stent. (4) Syringe pump: a pump for supplying a coating solution to the ultrasonic nozzle. (5) Moving motor of the reflecting plate to move the reflecting plate to coat the inside of stent. (6) Cylinder for jig fixed: on/off control of the jig fixed device. (7) Rotation motor & gear: motor & gear that bring a rotary motion to the jig to ensure uniform coating on the stent surface. (8) Jig fixed device for inside coating: locking device of the jig for internal coating. | |
The ultrasonic coating was carried out at 24 °C and 35% humidity with a closed chamber. The most important feature of our setup is the ultrasonic spray for coating the inside of the stent (Fig. 10), which can easily be controlled via a robotic system. A robotic system has a linear servo motor (TPC for one axis linear motor) to enable micro step control. The robot can be controlled through a computer PCI bus controller (National Instruments PCI-7390) using the LabVIEW 2013 program interface. We can precisely control the robot’s acceleration, speed and stroke distance. The system consists of a noise filter in front of the power supply for stability. To control the stroke position, we provided the robot with a limit photo sensor. Furthermore, we developed a terminal block for easy connection of the robot to the PCI-7390 board (National Instruments, USA).
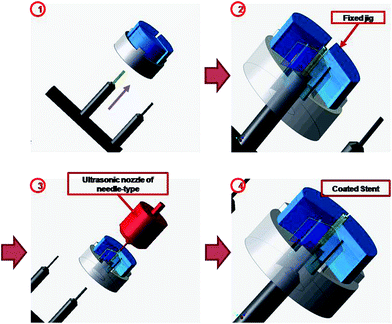 |
| Fig. 10 The working procedure for coating the inside of the stent. (1) Changed to fixed coating jig after pin inserted with stent. (2) After being inserted into the fixture, the supply pin is removed. (3) The ultrasonic nozzle, which is a type of needle, is moved to the outside of the stent, after coating the inside of the stent. (4) Final coated stent product. | |
3.3. Novel method for coating the abluminal and luminal surfaces of the stent strut
To identify the coating surface, we prepared a coating solution with Nile red. Briefly, the coating method is as follows. PLGA (20 mg) was dissolved in 5 mL tetrahydrofuran (THF) and Nile red solution was added (1 mg) and stirred for 1 hour. The bare metal stent (BMS, Co–Cr L605 alloy) was coated by the ultrasonic spray method using our coating machine (Model, Korea). The coating solution was applied to operate for a flow rate of 50 μL min−1 while BMS was placed on a mandrel that was attached to a rotating shaft. To remove any residual solvent in the stent, the coated stent was vacuum-dried for 24 hours. The whole procedure of stent coating is explained in Fig. 10. First change to fixed coating jig after the pin is inserted with the stent. After being inserted into the fixture, the supply pin is removed. Then the needle type ultrasonic nozzle is moved to the outside of the stent, after coating the inside of the stent.
3.4. Identification of coating method on the abluminal and luminal surfaces of the stent strut
The surface morphology was observed by optical microscopy (OM, Xi-CAM, Bestec Vision, Korea), scanning electron microscopy (SEM, SNE-1500M, SEC Co., Ltd, Korea), and fluorescence microscopy (FM, JULI, NanoEnTek, korea). The SEM sample was sputter coated with gold for 1.5 min to achieve a coating of approximately 15 nm. FM was used to investigate the homogeneity and identification of the coating on the abluminal and luminal surfaces. The distribution of the coating layer was detected with the fluorescent marker Nile red using an excitation wavelength of 488 nm. The green Nile red was identified with 530 nm and 10× and 20× objectives. The overlay of the fluorescence and the transmission light was recorded.
3.5. Preparation of the drug-eluting stent with an abluminal and luminal coating (DALC)
A schematic representation of the coating system is proposed in Fig. 1. The coating solution on the luminal surface of the stent strut was prepared with 2.0 wt% PC and 0.5 wt% WPP in methanol. The BMS, with a length of 18 mm and a diameter of 1.6 mm, was fix to a mandrel and the solution was sprayed on to the rotating stent at a distance of 0.5 cm. After coating, the residual methanol was removed by drying under a vacuum for 24 hours.
The coating solutions of the abluminal surface of the stent strut were prepared with 5.0 wt% PLGA and 0.5 wt% sirolimus in THF. The BMS, with a length of 18 mm and a diameter of 1.6 mm, was fix to a mandrel and the solution was sprayed on to the rotating stent at a distance of 3 cm. After coating, the residual THF was removed by drying under a vacuum for 24 hours.
3.6. Coating evaluation
The surface morphologies of the coated stents were observed by OM and SEM. Samples for the SEM were sputter coated with gold for 1.5 min to achieve a coating of approximately 15 nm. Coated stents were also inspected by FM. Coating thickness was measured by reflection spectrometry (RS, F40, Filmetrics, Inc., USA). The topography and the roughness of the surfaces were measured using atomic force microscopy (AFM, XE-100, Park System, Korea) under a tapping mode at room temperature.
3.7. Determination of drug loading and in vitro release
To determine the drug loading of the coated stents, a standard curve was obtained from various concentrations of SRL and WPP by high pressure liquid chromatography (HPLC, Nanospace SI-2, Shiseido, Japan). The coating stent was expanded into a silicon tube by balloon (3.0 × 20 mm) with 10 mmHg of pressure, and then both ends of the silicon tube were dipped in 5 mL of 10% ACN/PBS pH 4.5 solution at 37 °C.
Release measurements were performed using a peristaltic pump at 37 °C for 32 days in a setup similar to the body’s blood circulation system. The solution after drug release was detected at 278 nm (SRL) and 330 nm (WPP) using HPLC. The chromatographic separation of SRL and WPP was accomplished using a 50 × 4.6 mm, Phenomenex Luna C18 – 3 μm reverse phase analytical column. The mobile phase consisted of methanol, water, and formic acid at a ratio of 90
:
10
:
0.02 v/v%. The measurements were carried out in triplicate.
3.8. Assay of smooth muscle cell and endothelial cell proliferation
Smooth muscle cells (SMCs) and human umbilical vein endothelial cells (HUVECs) were purchased from the American Type Culture collection and grown in DMEM and EGM-2 supplemented with 10% FBS and 1% penicillin/streptomycin sulfate. Cells were maintained at 37 °C, 5% CO2 up to passage 3 and used at a predetermined concentration. The combination effect of SRL and WPP on cell proliferation was determined using a MTT cell proliferation assay. Cells were seeded at a density of 5 × 104 per well in 96-well plates using 100 μL DMEM supplemented in a CO2 incubator for 24 hours. After that, the coating stent containing sirolimus and WPP were added. After 7 days of incubation, MTT was added to the 96 wells. The absorbance was measured at 560 nm using a microtiter plate reader (Thermomax microplate reader, Molecular Devices).
3.9. In vivo study
The study was performed with the approval of the ethics committee of Chonam National University Hospital for the research. Since implantation of the stent was performed by expansion of a balloon, the stent was located to the intima of the artery. The animal experiment on New Zealand white rabbits has been described previously.18 The DLC, DAC and DALC were implanted in the left or right iliac arteries for 14 days. The stent was deployed by inflating the balloon catheter to nominal pressure (artery ratio: 1.1–1.3). The animals were sacrificed with 5 mL of potassium chloride in the intracarotid artery. Iliac arteries on the stent were removed carefully and fixed in 10% neutral buffer overnight. The arteries were step-sectioned and were processed for SEM.
3.10. Statistical analysis
Statistical analysis was performed with the aid of a commercially available software (SPSS Version 11.0, Chicago, IL). The results are expressed as the mean ± standard deviation (SD) from three experiments. A value of *p < 0.05 was considered statistically significant.
4. Conclusion
In summary, this study demonstrated that it is feasible to have separate coating layers of stent struts with new coating technology designed to increase stent endothelialization. This technology may promote vascular healing using PTCA. We showed that the drug release patterns from the abluminal and luminal coating layers result in inhibition of SMC proliferation and proliferation of endothelial cells. In future, we are planning to carry out animal experiments with the BMS, DLC, DAC, DLAC, and circumferential DESs to evaluate the preclinical evaluation.
Notes
The authors declare no competing financial interest.
Acknowledgements
This research was supported by a grant from the Technology Innovation Development Project funded by the Korean Small and Medium Business Administration (Project no. S2083465).
References
- A. N. DeMaria, J. Am. Coll. Cardiol., 2013, 61, 1205 CrossRef PubMed.
- D. W. Park, K. B. Seung, Y. H. Kim, J. Y. Lee, W. J. Kim, S. J. Kang, S. W. Lee, C. W. Lee, S. W. Park, S. C. Yun, H. C. Gwon, M. H. Jeong, Y. S. Jang, H. S. Kim, P. J. Kim, I. W. Seong, H. S. Park, T. Ahn, I. H. Chae, S. J. Tahk, W. S. Chung and S. J. Park, J. Am. Coll. Cardiol., 2010, 56, 117 CrossRef PubMed.
- C. Bauters, J. M. Lablanche, F. Leroy and M. E. Bertrand, Arch. Mal. Coeur Vaiss., 1992, 85, 1515 CAS.
- M. S. R. Frombach and W. Seger, Gesundheitswesen, 1997, 59, 447 Search PubMed.
- J. R. Sindermann, V. Verin, J. W. Hopewell, H. P. Rodemann and J. H. Hendry, Cardiovasc. Res., 2004, 63, 22 CrossRef CAS PubMed.
- S. M. S. McGinty, C. McCormick and M. Wheel, Math. Med. Biol., 2014, 1–24, DOI:10.1093/imammb/dqt025.
- T. F. Luscher, J. Steffel, F. R. Eberli, M. Joner, G. Nakazawa, F. C. Tanner and R. Virmani, Circulation, 2007, 115, 1051 CrossRef PubMed.
- G. Sydow-Plum and M. Tabrizian, Mater. Sci. Technol., 2008, 24, 1127 CrossRef CAS PubMed.
- J. N. Zhao, R. Falotico, T. Nguyen, Y. Cheng, T. Parker, V. Dave, C. Rogers and J. Riesenfeld, J. Biomed. Mater. Res., Part B, 2012, 100, 1274 CrossRef PubMed.
- E. C. Perin, Rev. Cardiovasc. Med., 2005, 1(suppl. 6), S13 Search PubMed.
- A. S. Puranik, E. R. Dawson and N. A. Peppas, Int. J. Pharm., 2013, 441, 665 CrossRef CAS PubMed.
-
(a) S. N. Sehgal, Clin. Biochem., 1998, 31, 335 CrossRef CAS;
(b) W. Khan, S. Farah, A. Nyska, A. M. S. Lee, S. A. Yoo, C. S. Cho, P. G. Suh, W. U. Kim and S. H. Ryu, J. Immunol., 2006, 177, 5585 CrossRef.
- M. N. Babapulle and M. J. Eisenberg, Circulation, 2002, 106, 2734 CrossRef.
- A. Sedaghat, J. M. Sinning, K. Paul, G. Kirfel, G. Nickenig and N. Werner, Clin. Res. Cardiol., 2013, 102, 413 CrossRef CAS PubMed.
- J. Domb, J. Controlled Release, 2013, 168, 70 CrossRef PubMed.
-
(a) A. T. O. S. Vaina and P. W. Serruys, Minerva Cardioangiol., 2005, 53, 341 Search PubMed;
(b) J. Zhang, Int. Heart J., 2014, 55, 213 CrossRef PubMed.
-
(a) K. H. Min, K. Park, Y. S. Kim, S. M. Bae, S. Lee, H. G. Jo, R. W. Park, I. S. Kim, S. Y. Jeong, K. Kim and I. C. Kwon, J. Controlled Release, 2008, 127, 208 CrossRef CAS PubMed;
(b) C. J. Creel, M. A. Lovich and E. R. Edelman, Circ. Res., 2000, 86, 879 CrossRef CAS;
(c) C. W. Hwang, D. Wu and E. R. Edelman, Circulation, 2001, 104, 600 CrossRef CAS.
-
(a) I. H. Bae, I. K. Park, D. S. Park, H. S. Lee and M. H. Jeong, J. Mater. Sci.: Mater. Med., 2012, 23, 1259 CrossRef CAS PubMed;
(b) Y. Z. Wu, L. Shen, Q. B. Wang, X. Hu, J. Xi, J. Y. Qian and J. B. Ge, Chin. Med. J., 2012, 125, 983 CAS.
|
This journal is © The Royal Society of Chemistry 2015 |