DOI:
10.1039/C5RA04129E
(Paper)
RSC Adv., 2015,
5, 31311-31317
Pd/mannose promoted tandem cross coupling-nitro reduction: expedient synthesis of aminobiphenyls and aminostilbenes†
Received
9th March 2015
, Accepted 25th March 2015
First published on 25th March 2015
Abstract
The dual role of D-mannose as a ligand for Pd catalyzed cross-coupling, and as a hydrogen source for nitro reduction is demonstrated in a modular cross coupling-nitro reduction sequence. The synthetic utility and generality of this green protocol has been illustrated by the synthesis of 20 aminobiphenyl and 10 aminostilbene derivatives in high yields through a one-pot Suzuki coupling-nitro reduction and a Heck coupling-nitro reduction, respectively, starting from halonitroarenes as substrates.
1. Introduction
Aminobiphenyls and aminostilbenes are an important class of compounds and form the core structural unit of a variety of hair dyes (I),1 antiplatelet agents (II),2 fluorescent agents (III),3 chemosensors (IV)4 and chemotherapeutic agents (V)5 (Fig. 1).
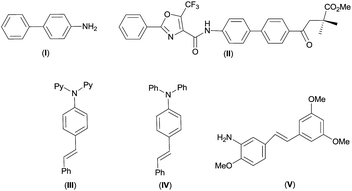 |
| Fig. 1 Selected examples of molecules containing aminobiphenyl and aminostilbene units. | |
Their synthesis, in general involves two common steps: namely, (a) Pd/ligand catalyzed cross-coupling of nitro aryl halides with boronic acids/styrenes, and (b) reduction of aromatic nitro group to yield aminobiphenyls/aminostilbenes (Scheme 1).6 A one-pot Suzuki cross-coupling followed by reductive amination of the nitro functionality has been used previously for the synthesis of secondary biaryl amines.7 Suzuki coupling starting from amino aryl halides is not as popular; since electron-donating substituents on the aryl group make the oxidative addition difficult and, that is why electron poor aryl halides are often referred to as ‘activated’, and electron-rich aryl groups as ‘deactivated’, since they give low yield of the products.8 A variety of phosphine ligands such as BINAP,9 PHOX,10 triphenyl phosphine11 etc. are used in Pd catalyzed cross-couplings as they help in stabilizing Pd in its various oxidation states in the catalytic cycle. However, most of these ligands are air and moisture sensitive, toxic, and reasonably expensive which makes it highly desirable to look for alternate cheaper and greener sources.
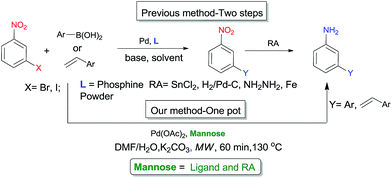 |
| Scheme 1 Synthesis of aminobiphenyls and aminostilbenes. | |
In this regard, ligands such as N-heterocyclic carbenes,12 oxazolines,13 Schiff bases,14 tetrazoles,15 amino acids,16 1,3-dicarbonyl compounds17 etc. have been developed. Further, reduction of nitro group is usually effected by catalytic hydrogenation using potentially flammable hydrogen gas (H2),18 or reducing agents such as hydrazine hydrate,19 silanes,20 sodium hydrosulphite,21 formates,22 decarborane23 etc. as the hydrogen source. In a recent study, glucose has also been used for the reduction of nitroarenes to the corresponding amines.24
Since, carbohydrates are the most abundant, eco-friendly and inexpensive biomolecules available in nature, their applications find immense attraction.25 They serve as precursors to many natural products and are used as chiral auxiliaries in asymmetric induction.26 In our endeavour towards developing greener and economical chemical protocols, we have recently reported D-glucosamine as an efficient alternative to phosphines in Pd-catalyzed Heck coupling of aryl and heteroaryl halides under microwave conditions.27 In continuation of these ongoing efforts, we explored the possibility of designing a one-pot cross coupling-reduction sequence using simple sugars. Multistep one-pot processes, so-called tandem processes, have the potential to impact the manufacturing of fine chemicals and pharmaceutical intermediates.28 Compared with stepwise synthesis, the combination of multiple catalytic reactions into one synthetic operation reduces the number of purification steps, provides better substrate scope, and enhances reactivity and selectivity by allowing equilibrium reactions to proceed to nearly full conversion, thus contributing to an improved process economy as well as to more sustainable synthetic routes. Herein, we demonstrate D-mannose for the first time as an efficient ligand for Pd catalyzed cross-coupling, as well as hydrogen source for nitro reduction. Both the properties have been utilized in developing an efficient one-pot tandem protocol for aminobiphenyl and aminostilbene synthesis starting from halonitroarenes.
2. Results and discussion
We began our investigation by heating 3-nitrobromobenzene (1a, 1.0 equiv.) with phenyl boronic acid (2a, 1.0 equiv.) in DMF/H2O (3
:
1) using K2CO3 (3.0 equiv.), glucose (3.0 equiv.) and Pd(OAc)2 (5 mol%) as catalyst at 130 °C. After 14 hours of reaction, the desired product biaryl amine (3a) was isolated in 65% yield. Pleased with the one-pot cross-coupling and nitro reduction, we explored if the same reaction could be carried out under microwave conditions with an intention of reducing the reaction time, side reactions and increasing the product yield. We were quite delighted to find that changing from conventional to MW heating drastically reduced the reaction time to 1 hour, and 3a was isolated in 67% yield. The results indicated that as anticipated, the reaction followed a tandem cross-coupling nitro-reduction sequence mediated by Pd–glucose system. Further, we found that lowering the Pd loading to 3 mol% did not affect the product yield. However, decreasing it further to 2 mol% and 1 mol% led to substantial reduction in the yield of reduced product 3a. Therefore, it was decided to carry out further optimization studies with 3 mol% Pd(OAc)2. To establish the role of sugars in promoting the reaction, a variety of sugars such as galactose, glucosamine, arabinose, ribose, fructose, sucrose, mannose and 1,2-protected glucose (L1–9) were examined. The results as shown in Table 1 indicated D-mannose to be the most potent of all the sugars in effecting the cross-coupling cum reduction. No prior report on use of D-mannose as a ligand and reducing agent exists in literature. With the non-reducing sugar sucrose (L7), the reduced product 3a was not obtained at all and the reaction stopped after cross-coupling to yield 3-nitrobiphenyl (7b) in 90% yield. Optimization of reaction with respect to solvent, temperature, and base was carried out; and the results are summarized in Table 2. A series of organic solvents such as DMF, CH3CN and DCE (Table 2; entry 1–3) were screened. It was found that reaction did not take place in CH3CN and DCE while 20% product was formed in DMF. In water alone as solvent, 3a was obtained in 30%. The stalling of reaction in organic medium suggested the necessity of water as a co-solvent to solubilize sugar required for the reaction. Based on this observation, mixtures of organic solvent and water in the ratio 3
:
1 were examined. Amongst DMSO/H2O, CH3CN/H2O, dioxane/H2O and DMF/H2O combinations (Table 2; entry 5–8), highest yield of 3a (88%) was obtained in DMF/H2O. Changing the ratio of DMF/H2O to 1
:
1, 2
:
1 and 4
:
1 resulted in reduced product yield 50–71% (Table 2, entry 9). Further, variation of temperature in the range 80–140 °C revealed that the optimum temperature for the reaction was 130 °C. Raising the temperature to 140 °C or lowering to 100 °C reduced the yield of 3a (Table 2; entry 10–13). Furthermore, effect of base on the reaction was investigated. Different bases such as KOH, Et3N, Cs2CO3 and K2CO3 were tested (Table 2; entry 8, 14–16), and highest yield was obtained with K2CO3. Lowering the equivalents of K2CO3 resulted in reduced yield of the product while increasing it to 4 equivalents did not bring about much change (Table 2; entry 17).
Table 1 Optimization of sugars for Suzuki coupling-nitro reduction and Heck coupling-nitro reductiona
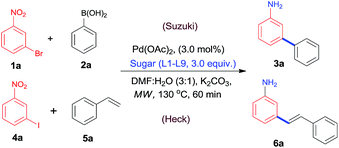
|
Reaction conditions: for Suzuki, 1a (1.0 equiv., 0.1 mmol), 2a (1.0 equiv., 0.1 mmol); for Heck, 4a (1.0 equiv., 0.08 mmol), 5a (1.0 equiv., 0.08 mmol), sugar (3.0 equiv., 0.3 mmol), Pd(OAc)2 (0.03 equiv., 3 mol%), K2CO3 (3.0 equiv., 0.3 mmol), DMF/H2O (3 : 1), 60 min (MW), 130 °C, power 20 watts. HPLC yield of 3a. HPLC yield of 6a. Suzuki coupling with 2.0 equiv. of mannose. Suzuki coupling with 1.0 equiv. of mannose. |
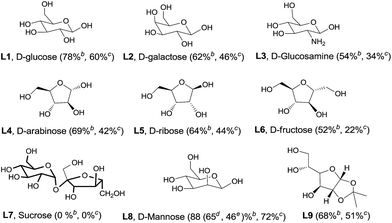 |
Table 2 Optimization of reaction conditions for tandem Suzuki coupling-nitro reduction and Heck coupling-nitro reduction
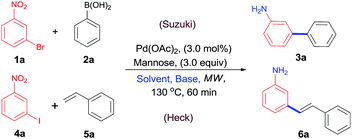
|
Entrya |
Solvent |
Temp (°C) |
Base |
Yieldb (%) |
Yieldc (%) |
Reaction conditions: for Suzuki, 1a (1.0 equiv., 0.1 mmol), 2a (1.0 equiv., 0.1 mmol); for Heck, 4a (1.0 equiv., 0.1 mmol), 5a (1.2 equiv., 0.12 mmol), mannose (3.0 equiv., 0.3 mmol), Pd(OAc)2 (0.03 equiv., 3 mol%), base (3.0 equiv.), MW, 20 W, 60 min, 130 °C; binary solvent (3 : 1). HPLC yield for Suzuki. HPLC yield for Heck. Reaction time 50 minutes. Reaction time 40 minutes. Solvent in 1 : 1 ratio. Solvent in 2 : 1 ratio. Solvent in 4 : 1 ratio. With 1.0 equiv. K2CO3. With 2.0 equiv. K2CO3. With 4.0 equiv. K2CO3. Under conventional heating at 130 °C for 12 h. |
1 |
DMF |
130 |
K2CO3 |
20 |
10 |
2 |
CH3CN |
130 |
K2CO3 |
0 |
0 |
3 |
DCE |
130 |
K2CO3 |
0 |
0 |
4 |
H2O |
130 |
K2CO3 |
30 |
15 |
5 |
DMSO/H2O |
130 |
K2CO3 |
55 |
52 |
6 |
CH3CN/H2O |
130 |
K2CO3 |
5 |
5 |
7 |
Dioxane/H2O |
130 |
K2CO3 |
20 |
20 |
8 |
DMF/H2O (3 : 1) |
130 |
K2CO3 |
88, 81d, 73e |
72, 56d, 43e |
9 |
DMF/H2O |
130 |
K2CO3 |
50f, 60g, 71h |
54f, 59g, 65h |
10 |
DMF/H2O |
120 |
K2CO3 |
82 |
64 |
11 |
DMF/H2O |
110 |
K2CO3 |
63 |
59 |
12 |
DMF/H2O |
100 |
K2CO3 |
41 |
42 |
13 |
DMF/H2O |
140 |
K2CO3 |
79 |
68 |
14 |
DMF/H2O |
130 |
KOH |
76 |
54 |
15 |
DMF/H2O |
130 |
Cs2CO3 |
45 |
43 |
16 |
DMF/H2O |
130 |
Et3N |
0 |
0 |
17 |
DMF/H2O |
130 |
K2CO3 |
42i, 68j, 84k |
30i, 50j, 67k |
18 |
DMF/H2O |
130 |
K2CO3 |
70l |
58l |
The same protocol was extended to investigate the feasibility of a one-pot Heck coupling-nitro reduction. The reaction of 3-iodonitrobenzene (4a, 1.0 equiv.) with styrene (5a, 1.0 equiv.) in presence of Pd(OAc)2 (3 mol%), mannose (3 equiv.) and K2CO3 (3.0 equiv.) in DMF/H2O (3
:
1) for 60 minutes yielded 3-amino trans-stilbene (6a) in 72% yield. Excited with the generality of the protocol towards cross-coupling nitro-reduction sequences, we optimized the conditions again for this transformation. Screening of various sugars for Heck coupling-nitro reduction is given in Table 1, and showed D-mannose as the most efficient ligand/reducing agent for this reaction as well. Optimization with respect to solvent, base and temperature was carried out in the same way as for Suzuki coupling-reduction and the results are summarized in Table 2. The data indicated that the trend observed for various reaction parameters in this case was identical to the previous cross coupling-reduction sequence discussed. It was concluded from this study that the best optimized conditions for 1.0 equiv. of substrates in DMF
:
H2O (3
:
1) were 3 mol% Pd(OAc)2, 3.0 equiv. mannose, 3.0 equiv. K2CO3, and heating at 130 °C in MW for 60 minutes.
To establish the versatility of this methodology, a variety of nitro aryl halides (1a–c) and aryl boronic acids (2a–n) were reacted on a 0.5 mmol scale (∼100 mg) under the optimized conditions, and substituted aminobiphenyls (3a–t) were obtained in high yields (Table 3). In general, it was found that electron donating groups (methyl, ethyl, methoxy, amino) on the aryl boronic acid gave slightly higher yield of the products compared to aryl boronic acids substituted with electron withdrawing groups (F, CN). The reduction was chemoselective for nitro group as the acetanilide, nitrile, methyl and methoxy groups remained intact under the employed conditions (Table 3). Further, unlike previous reported methods such as catalytic hydrogenation,29 Pd(OAc)2/PMHS30 or S8/mild base,31 where dehalogenation of halogen substituted nitro compounds takes place, in this case, the coupling and nitro reduction proceeded without dehalogenation (3o, 3p). Furthermore, dinitrobiphenyls produced by cross-coupling of bromonitrobenzene with nitrophenyl boronic acid underwent complete reduction of both the nitro groups yielding diaryldiamines (3m, 3n) in high yield. Indole-5-boronic acid could also be easily converted to the corresponding arylheteroaryl amine without affecting the heterocyclic ring (3t). When the reaction was performed on gram scale with 1 g of the substrate 1a under MW conditions, 700 mg of the product 3a was isolated (85%) demonstrating the practical utility of this method. The generality was further established by synthesis of an array of aminostilbenes. The synthesis was achieved by reacting ortho- and meta- substituted nitro aryl iodides (4a–c) with various substituted styrenes (5a–f) and the products (6a–j) were isolated in 57–81% yield. Yields with electron rich styrenes such as naphthyl styrene and methoxy styrene were little higher than with unsubstituted styrene or having electron withdrawing substituents on benzene ring (Table 4). The double bond which is highly labile under reducing conditions32 was essentially inert with the employed reduction protocol. Reaction of 4-iodonitrobenzene (4c) with internal olefin 2,3-dihydrofuran was carried out under the same reaction conditions to explore any enantioselectivity that might be offered by use of mannose as a ligand. Unfortunately, however, it was found that the reaction stopped after the cross-coupling step itself yielding a racemic mixture of 2-(4-nitrophenyl)-2,3-dihydrofuran (6′) in 61% yield along with unidentified side products. Increasing the reaction time for another 30 minutes did not change the product distribution and no traces of the reduced amine were obtained. To understand the course of action in this one-pot coupling-reduction sequence, we decided to conduct a time-dependent monitoring of the reaction. It was found that the Pd catalyzed cross-coupling of 1a and 2a was completed in 5 minutes giving 89% yield of the coupled nitrobiphenyl (7a). However, no traces of the final product 3a or the reduced amine ortho-bromoaniline were seen.
Table 3 Tandem Suzuki coupling-nitro reduction for synthesis of aminobiphenylsa
Table 4 Tandem Heck coupling-nitro reduction for synthesis of aminostilbenesa
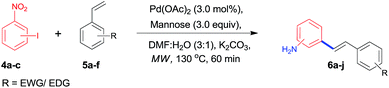
|
Reaction conditions: 4a–b (1.0 equiv.), 5a–f (1.2 equiv.), D-mannose (3.0 equiv.), Pd(OAc)2 (3 mol%), K2CO3 (3.0 equiv.), DMF/H2O (3 : 1), 130 °C, MW, 20 W, 60 min, 130 °C; yields of isolated product. |
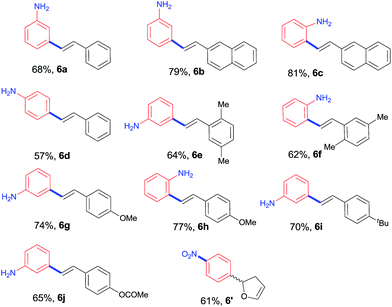 |
From these observations, it was evident that the reaction followed a tandem cross coupling-reduction sequence. It initiated by a fast cross-coupling completed in approximately 5–15 minutes for various substrates, followed by complete reduction of the nitro group to amine in the next 45 minutes under MW irradiation to yield the aminobiphenyls/aminostilbenes. Based on this observation, by arresting the reaction within 5–15 minutes, nitrobiaryl derivatives were isolated in high yields using Pd/mannose system (Table 5). Both the Heck and Suzuki coupling did not take place in the absence of mannose under the employed reaction conditions, and only the homo coupled product was obtained as the major product. This suggested the probable role of D-mannose as a ligand in stabilizing the Pd species, and facilitating a fast cross-coupling reaction.
Table 5 Synthesis of nitrobiaryl derivativesa
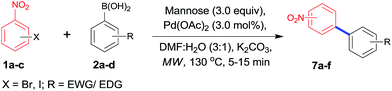
|
Reaction conditions: 1a–c (1.0 equiv.), 2a–d (1.0 equiv.), sugar (3.0 equiv.), Pd(OAc)2 (0.03 equiv., 3.0 mol%), K2CO3 (3.0 equiv.), DMF/H2O (3 : 1), MW, 20 W, 5–15 min, 130 °C, yield is isolated yield. |
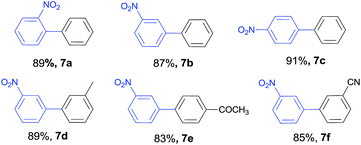 |
The mechanism of the reaction has been illustrated in Scheme 2. The reaction is believed to be initiated by reduction of palladium acetate to Pd(0) by mannose (PXRD, see ESI, Fig. 2†), and stabilization of Pd(0) in form of a chelate complex (A) through coordination with the hydroxyl groups of mannose through 1,2 or 3,4 sites.33 This is followed by oxidative addition of aryl halide to form the organopalladium species (B). For the Suzuki coupling cycle, reaction of B with K2CO3 gives an intermediate (C) which via transmetallation with the boronate complex (D) forms the organopalladium species (E). Reductive elimination of E yields the desired biphenyl with the regeneration of the active Pd(0) species for the next catalytic cycle. In the Heck pathway, π-complexation of the olefin with B gives an intermediate (F). This is followed by insertion of styrene into the Pd–C bond to yield an intermediate (G) which undergoes hydride elimination with the formation of a new Pd–olefin complex (H). Dissociation of H yields the desired trans-stilbene along with formation of Pd(II) compound (I). Pd(0) is regenerated by reductive elimination of I by K2CO3 in the final step. The reduction of nitro group to amino group in the next step is realized by an in situ generation of hydrogen along with lactate, acetate, formate and glycolate through hot alkaline degradation of mannose.34
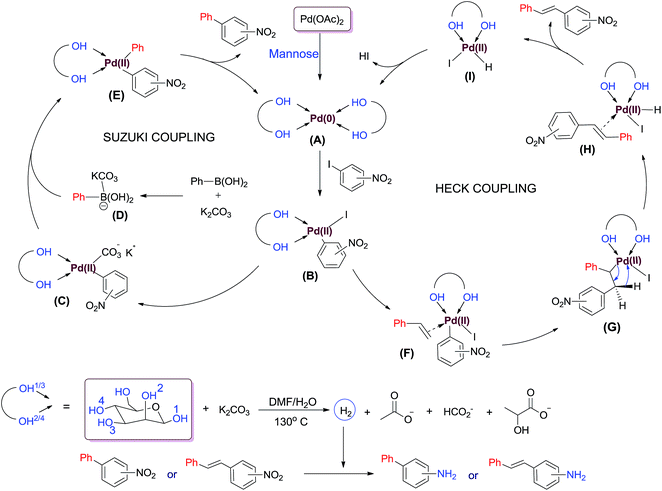 |
| Scheme 2 Mechanism of tandem cross coupling-reduction. | |
3. Experimental section
General remarks
All the reactions were carried out under Microwave irradiation using CEM Discover system. All reagents and solvents were of pure analytical grade. Thin layer chromatography (TLC) was performed on 60 F254 silica gel, pre-coated on aluminium plates and revealed with either a UV lamp (λmax = 254 nm) or iodine vapours. The products were purified by column chromatography on silica gel 230–400 mesh. 1H and 13C NMR spectra were recorded on a Bruker DPX-300 MHz spectrometer (1H 300 MHz, 13C 75 MHz) using CDCl3 as the solvent with tetramethylsilane (TMS) as the internal standard at room temperature. Chemical shifts are in δ (ppm) relative to TMS. The coupling constants (J) in Hz. The ESI-MS was performed on MICROTOF II mass spectrometer. Powder XRD was taken on powder X-ray diffractometer (Bruker). Achiral HPLC analysis was performed on Hitachi HPLC system (Waters, Milliford), using Phenomenex C18 HPLC column (5.0 μm, 4.6 mm × 250 mm) and acetonitrile and ammonium acetate buffer (pH 6.70; 0.01 M) as gradient eluting solvent system. Chiral HPLC was performed on above mentioned system using Column-Chiralpak IA (5.0 μm, 4.6 mm × 250 mm) at isocratic elution using 0.1% TFA in ethanol 1% and 0.1% TFA in n-hexane 99% of 15 min run time.
General procedure for synthesis of aminobiphenyls (3a–t)
To a solution of nitroarylbromide (0.49 mmol, 1.0 equiv.) in DMF
:
H2O (3
:
1) in a microwave tube, phenyl boronic acid (0.49 mmol, 1.0 equiv.), D-mannose (1.47 mmol, 3.0 equiv.), K2CO3 (1.47 mmol, 3.0 equiv.) and palladium acetate (3 mol%) were added. The contents were irradiated in microwave for 60 min at 130 °C. After completion of the reaction, the combined organic layer was extracted with ethyl acetate, washed with water, dried over Na2SO4 and concentrated under reduced pressure. The residue was purified by column chromatography to afford the aminobiphenyl product.
General procedure for synthesis of aminostilbenes (6a–j)
To a solution of nitroaryliodide (0.49 mmol, 1.0 equiv.) in DMF
:
H2O (3
:
1) in a microwave tube, styrene (0.59 mmol, 1.2 equiv.), D-mannose (1.47 mmol, 3.0 equiv.), K2CO3 (1.47 mmol, 3.0 equiv.) and palladium acetate (1 mol%) were added. The contents were irradiated in microwave for 60 min at 130 °C. After completion of the reaction, the combined organic layer was extracted with ethyl acetate, washed with water, dried over Na2SO4 and concentrated under reduced pressure. The residue was purified by column chromatography to afford the aminostilbene product.
4. Conclusion
Through this work, we demonstrate that D-mannose is an efficient, green and economical replacement of phosphine ligands in Pd-catalyzed Suzuki and Heck reactions, and also an effective reducing agent for chemoselective reduction of nitro group in the presence of other functional groups such as CN, C
C and CONH2. Both these features have been utilized in developing one-pot cross coupling-nitro reduction sequence. The protocol is very simple and versatile, and has been used for the synthesis of an array of aminobiphenyls and aminostilbenes in high yields. The use of MW provides high yield of products in short times. The developed method is an environmentally friendly alternative to the existing two-step procedure, since it eliminates the use of toxic phosphines and reducing agents, and provides scalable, atom economic route for practical synthesis of aminobiphenyls and aminostilbenes.
Acknowledgements
The authors thank Department of Science and Technology (DST), India (RP02555) for financially supporting this work, and DST-FIST for funding the ESI-HRMS facility at IIT Delhi. SR thank CSIR for his graduate fellowship.
Notes and references
-
(a) R. J. Turesky, J. P. Freeman, R. D. Holland, D. M. Nestorick, D. W. Miller, D. L. Ratnasinghe and F. F. Kadlubar, Chem. Res. Toxicol., 2003, 16, 1162–1163 CrossRef CAS PubMed;
(b) F. Cioni, G. Bartolucci, G. Pieraccini, S. Meloni and G. Moneti, Rapid Commun. Mass Spectrom., 1999, 13, 1833–1837 CrossRef CAS;
(c) S. W. Oh, M. N. Kang, C. W. Cho and M. W. Lee, Dyes Pigm., 1997, 33, 119–135 CrossRef CAS.
- D. R. Bolin, A. W. H. Cheung, F. Firooznia, M. M. Hamilton, L. A. McDermott, Y. Qian, J. Tan and W. Yun, US Pat., Appl. Publ. 20090093497, 2009.
-
(a) A. Kamal, G. B. Kumar, S. Polepalli, A. B. Shaik, V. S. Reddy, M. K. Reddy, C. R. Reddy, R. Mahesh, J. S. Kapure and N. Jain, Chem. Med. Chem., 2014, 11, 2565–2579 CrossRef PubMed;
(b) A. P. De Silva, H. Q. N. Gunaratne, T. Gunnlaugsson, A. J. M. Huxley, C. P. McCoy, J. T. Rademacher and T. E. Rice, Chem. Rev., 1997, 97, 1515–1566 CrossRef CAS PubMed;
(c) B. Valeur and I. Leray, Coord. Chem. Rev., 2000, 205, 3–40 CrossRef CAS;
(d) B. Wang and M. R. Wasielewski, J. Am. Chem. Soc., 1997, 119, 12–21 CrossRef CAS;
(e) G. K. Walkup, S. C. Burdette, S. J. Lippard and R. Y. Tsien, J. Am. Chem. Soc., 2000, 122, 5644–5645 CrossRef CAS.
-
(a) J. S. Yang, Y. H. Lin and C. S. Yang, Org. Lett., 2002, 5, 777–780 CrossRef PubMed;
(b) T. Hirano, K. Kikuchi, Y. Urano, T. Higuchi and T. Nagano, J. Am. Chem. Soc., 2000, 122, 12399–12400 CrossRef CAS;
(c) S. C. Burdette, G. K. Walkup, B. Spingler, R. Y. Tsien and S. J. Lippard, J. Am. Chem. Soc., 2001, 123, 7831–7841 CrossRef CAS PubMed.
-
(a) M. Roberti, D. Pizzirani, D. Simoni, R. Rondanin, R. Baruchello, C. Bonora, F. Buscemi, S. Grimaudo and M. Tolomeo, J. Med. Chem., 2003, 46, 3546–3554 CrossRef CAS PubMed;
(b) J. Burns, T. Yokota, H. Ashihara, M. E. J. Lean and A. Crozier, J. Agric. Food Chem., 2002, 50, 3337–3340 CrossRef CAS PubMed.
-
(a) A. Suzuki, J. Organomet. Chem., 1999, 576, 147–168 CrossRef CAS;
(b) R. F. Heck, J. Am. Chem. Soc., 1969, 91, 6707–6714 CrossRef CAS;
(c) S. L. Buchwald, J. Am. Chem. Soc., 2005, 127, 4685–4696 CrossRef PubMed;
(d) J. P. Wolfe, R. A. Singer, B. H. Yang and S. L. Buchwald, J. Am. Chem. Soc., 1999, 41, 9550–9561 CrossRef;
(e) M. M. Pohl, H. Junge, K. Junge and M. Beller, Chem. Commun., 2011, 47, 10972–10974 RSC;
(f) D. Balcom and A. Furst, J. Am. Chem. Soc., 1953, 17, 4332–4334 Search PubMed;
(g) D. K. Bates and K. Li, J. Org. Chem., 2002, 67, 8662–8665 CrossRef CAS PubMed.
-
(a) S. Å. Lunde and M. O. Sydnes, Synlett, 2013, 2340–2344 CAS;
(b) L. Pedersen, M. F. Mady and M. O. Sydnes, Tetrahedron Lett., 2013, 54, 4772–4775 CrossRef CAS PubMed.
- N. J. Whitecomb, K. K. Hii and S. E. Gibson, Tetrahedron, 2001, 57, 7449–7476 CrossRef.
-
(a) M. S. Shibasaki, J. Org. Chem., 1989, 54, 4738–4739 CrossRef;
(b) L. E. Overman, J. Am. Chem. Soc., 1998, 120, 6477–6487 CrossRef.
- G. Helmchen and A. Pfaltz, Acc. Chem. Res., 2000, 33, 336–345 CrossRef CAS PubMed.
-
(a) M. Mori and K. Ban, Tetrahedron, 1977, 12, 1037–1040 CrossRef;
(b) L. E. Overman, J. Am. Chem. Soc., 1990, 112, 6959–6964 CrossRef.
-
(a) C. W. K. Gstottmayr, V. P. W. Bohm, E. Herdtweck, M. Grosche and W. A. Herrmann, Angew. Chem., Int. Ed., 2002, 41, 1363–1365 CrossRef CAS;
(b) T. T. Gao, A. P. Jin and I. X. Shao, Beilstein J. Org. Chem., 2012, 8, 1916–1919 CrossRef CAS PubMed;
(c) G. C. Fortman and S. P. Nolan, Chem. Soc. Rev., 2011, 40, 5151–5169 RSC.
-
(a) S. Lee, J. Organomet. Chem., 2006, 691, 1347–1355 CrossRef CAS PubMed;
(b) S. M. Hussain, M. B. Ibrahim, A. Fazal, R. Suleiman, M. Fettouhi and B. El Ali, Polyhedron, 2014, 70, 39–46 CrossRef PubMed.
-
(a) G. A. Grasa, A. C. Hillier and S. P. Nolan, Org. Lett., 2001, 3, 1077–1080 CrossRef CAS PubMed;
(b) M. Solinas, B. Sechi, G. Chelucci, S. Baldino, J. R. Pedro and G. Blay, J. Mol. Catal. A: Chem., 2014, 385, 73–77 CrossRef CAS PubMed.
- A. K. Gupta, C. H. Song and C. H. Oh, Tetrahedron Lett., 2004, 45, 4113–4116 CrossRef CAS PubMed.
- X. Cong, H. Tang, C. Wu and X. Zeng, Organometallics, 2013, 32, 6565–6575 CrossRef CAS.
- X. Cui, J. Li, L. Lui and Q. X. Guo, Chin. Chem. Lett., 2007, 18, 625–628 CrossRef CAS PubMed.
-
(a) H. U. Blaser, Science, 2006, 313, 312–313 CrossRef CAS PubMed;
(b) A. Corma, P. Serna, P. Concepcion and J. Calvino, J. Am. Chem. Soc., 2008, 130, 8748–8753 CrossRef CAS PubMed;
(c) H. U. Blaser, U. Siegrist, H. Steiner and M. Studer, Fine Chemicals through Heterogeneous Catalysis, ed. R. A. Sheldon and H. V. Bekkum, Wiley-VCH, Weinheim, 2001, pp. 389–406 Search PubMed.
-
(a) U. Sharma, P. Kumar, N. Kumar, V. Kumar and B. Singh, Adv. Synth. Catal., 2010, 354, 1834–1840 CrossRef;
(b) U. Sharma, P. K. Verma, N. Kumar, V. Kumar, M. Bala and B. Singh, Chem.–Eur. J., 2011, 17, 5903–5907 CrossRef CAS PubMed.
-
(a) K. Junge, B. Wendt, N. Shaikh and M. Beller, Chem. Commun., 2010, 46, 1769–1771 RSC;
(b) R. J. Rahaim and R. E. Maleczka, Org. Lett., 2005, 7, 5087–5090 CrossRef CAS PubMed.
- C. T. Redemann and C. E. Redemann, Org. Synth., 1955, 3, 69 Search PubMed.
-
(a) H. Imai, T. Nishiguchi and K. Fukuzumi, Chem. Lett., 1976, 655–656 CrossRef CAS;
(b) S. Gowda, K. Abiraj and D. C. Gowda, Tetrahedron Lett., 2002, 43, 1329–1331 CrossRef CAS;
(c) A. K. Srinivasa, R. Gejjalagere and D. C. Gowda, Can. J. Chem., 2005, 83, 517–520 CrossRef.
- J. W. Bae, Y. J. Cho, S. H. Lee, C. O. M. Yoon and C. M. Yoon, Chem. Commun., 2000, 1857–1858 RSC.
- M. Kumar, U. Sharma, S. Sharma, V. Kumar, B. Singh and N. Kumar, RSC Adv., 2013, 3, 4894–4898 RSC.
-
(a) R. E. Ireland and J. P. Vevert, J. Org. Chem., 1980, 45, 4259–4260 CrossRef CAS;
(b) N. Xavier and A. Rauter, Curr. Top. Med. Chem., 2014, 14, 1235–1243 CrossRef CAS.
-
(a) Y. Mata, M. Dieguez, O. Pamies and C. Claver, Org. Lett., 2005, 7, 5597–5599 CrossRef CAS PubMed;
(b) P. Cui, H. Liu, X. Guo, D. Zhang, Y. Wang and C. Wang, J. Org. Chem., 2013, 33, 422–436 Search PubMed;
(c) M. Dieguez and O. Pamies, in Carbohydrates-Tool for Stereoselective Synthesis, ed. M. K. Boysen, Wiley-VCH, Weinheim, 2013, pp. 225–245 Search PubMed.
- A. K. Jha, R. K. Sahani and N. Jain, Synlett, 2015, 259–264 CAS.
-
(a) D. E. Fogg and E. N. dos Santos, Coord. Chem. Rev., 2004, 248, 2365–2379 CrossRef CAS PubMed;
(b) A. Ajamian and J. L. Gleason, Angew. Chem., Int. Ed., 2004, 43, 3754–3760 CrossRef CAS PubMed;
(c) J. C. Wasilke, S. J. Obrey, R. T. Baker and G. C. Bazan, Chem. Rev., 2005, 105, 1001–1020 CrossRef CAS PubMed;
(d) M. M. Hussain and P. J. Walsh, Acc. Chem. Res., 2008, 41, 883–893 CrossRef CAS PubMed;
(e) C. A. Denard, J. F. Hartwig and H. Zhao, ACS Catal., 2013, 3, 2856–2864 CrossRef CAS.
- R. S. Dowing, P. J. Kunkeler and H. V. Bekkum, Catal. Today, 1997, 37, 121–136 CrossRef.
- R. J. Rahaim and R. E. Maleczka, Org. Lett., 2005, 7, 5087–5090 CrossRef CAS PubMed.
- M. A. McLaughlin and D. M. Barnes, Tetrahedron Lett., 2006, 47, 9095–9097 CrossRef CAS PubMed.
-
(a) H. U. Blaser, H. Steiner and M. Studer, ChemCatChem, 2009, 1, 210–221 CrossRef CAS;
(b) L. Pehlivan, E. Metay, S. Laval, W. Dayoub, P. Demonchaux, G. Mignani and M. Lemaire, Tetrahedron, 2011, 67, 1971–1976 CrossRef CAS PubMed.
- P. Klufers and T. Kunte, Angew. Chem., Int. Ed., 2001, 22, 4210–4212 CrossRef.
- A. V. Ellis and M. A. Wilson, J. Org. Chem., 2002, 67, 8469–8474 CrossRef CAS PubMed.
Footnote |
† Electronic supplementary information (ESI) available. See DOI: 10.1039/c5ra04129e |
|
This journal is © The Royal Society of Chemistry 2015 |