DOI:
10.1039/C5RA03931B
(Paper)
RSC Adv., 2015,
5, 27069-27074
β-Substituted triarylborane appended porphyrins: photophysical properties and anion sensing†
Received
5th March 2015
, Accepted 10th March 2015
First published on 10th March 2015
Abstract
β-Substituted triarylborane porphyrins were designed and synthesized by the Pd-catalyzed Sonogashira cross-coupling reaction. The incorporation of triarylborane unit results in a red shifted absorbance and fluorescence. The sensing ability of these porphyrins was studied for different anions. The triarylborane porphyrin 4 and 5 selectively detects fluoride ions as shown by the UV/vis absorption and fluorescence titration experiments. The binding constants for triarylborane porphyrin 4 and 5 in dichloromethane at 25 °C were found to be 1.0 × 106 M−1 and 5.0 × 105 M−1 respectively.
Introduction
In recent years triarylborane containing molecular systems have attracted considerable attention due to their applications in optoelectronic devices and anion sensing.1–8 A variety of boron containing conjugated donor–acceptor systems have been documented in the literature for sensing applications.7–17
Selective detection of fluoride ions is of wide interest because of their importance towards human health and impact on the environment.18,19 The importance of this anion in the treatment of osteoporosis and dental care leads to the continuous pursuit of the design and synthesis of new selective fluoride ion sensors.
Literature reveals couple of reports, where meso substituted3–5 borylated porphyrins have been synthesized and studied as potential candidates for anion sensing.20,21 Kubo et al. reported the meso substituted triarylborane porphyrin using Sonogashira coupling reaction, which has been used for fluoride ion sensing (Chart 1).20 Moreover Thilagar et al. reported the synthesis and anion sensing properties of meso-substituted tetra triarylborane appended porphyrin (Chart 1).21
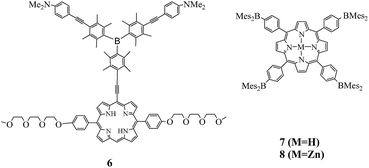 |
| Chart 1 Structures of previously reported meso substituted triarylborane porphyrin. | |
To the best of our knowledge, there are no reports on β-substituted borylated porphyrins for anion binding. Our group is interested in the design and synthesis of β-substituted porphyrins for various optoelectronic applications.22 In this manuscript, we have incorporated triarylborane unit at the β-position of porphyrin which is in direct conjugation with the 18π-molecular system and results in significant perturbation of the photophysical properties. The β-substituted triarylborane porphyrin 4 and its metalated derivative 5 were synthesized by the Pd-catalyzed Sonogashira cross-coupling reaction and metalation reaction and their photophysical and anion binding properties were studied.
Results and discussion
The synthetic routes for the β-substituted triarylborane porphyrin 4 and its metalated triarylborane porphyrin 5 are shown in Scheme 1. The triarylborane porphyrin 4 was synthesized by the Pd-catalysed Sonogashira cross-coupling reaction of the β-bromo tetraphenyl porphyrin with the (4-ethynylphenyl)dimesitylborane 3 (Scheme 1). The 5,10,15,20-tetraphenylporphyrin (TPP) was synthesized by the condensation reaction of pyrrole, and benzaldehyde following the Lindsey procedure.23
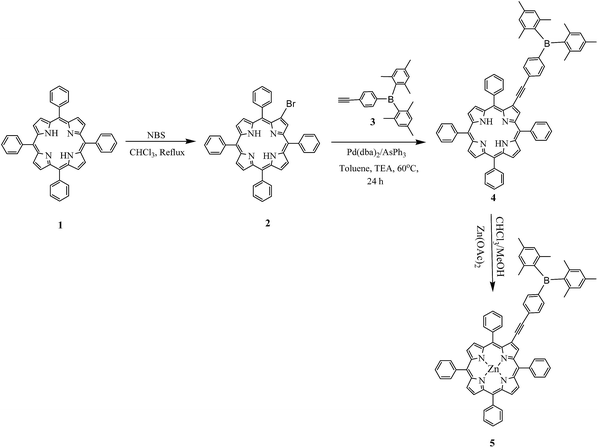 |
| Scheme 1 Synthesis of triarylborane porphyrin 4 and 5. | |
The bromination reaction of tetraphenylporphyrin was carried out using N-bromosuccinimide (NBS) in CHCl3.24 The β-bromo tetraphenyl porphyrin 2 was purified by the column chromatography using toluene/cyclohexane (30
:
70).24 The precursor (4-ethynylphenyl)dimesitylborane 3 was synthesized by the reaction of 1,4-dibromobenzene with dimesitylboron fluoride in presence of n-butyllithium.25
The Sonogashira cross-coupling reaction of β-bromo porphyrin 2 with (4-ethynylphenyl)dimesitylborane 3 using the catalyst Pd(dba)2/AsPh3, resulted triarylborane porphyrin 4 in 50% yield.26,27 The triarylborane porphyrin 4 was reacted with zinc acetate (Zn(OAc)2·2H2O) in refluxing chloroform/methanol (2
:
1) which resulted porphyrin 5 in 80% yield (Scheme 1). The triarylborane porphyrin 4 and 5 are readily soluble in common organic solvents. The triarylborane porphyrin 4 and 5 were well characterized by 1H NMR, 13C NMR and HRMS techniques.
Photophysical properties
The electronic absorption and emission spectra of the triarylborane porphyrin 4 and 5 were recorded in dichloromethane (Fig. 1).
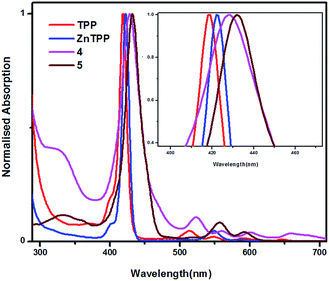 |
| Fig. 1 Electronic absorption spectra of the TPP, ZnTPP and triarylborane porphyrin 4 and 5 at 1.0 × 10−6 M, recorded in dichloro methane. The inset shows enlarged view. | |
The triarylborane porphyrins 4 show boryl absorption band at 328 nm, intense Soret band at 428 nm, and four Q-bands in the region of 500–670 nm. The metalated triarylborane porphyrin 5 exhibit boryl absorption band at 333 nm, intense Soret band at 431 nm, and two Q-bands in the region of 550–600 nm. The substitution of the dimesitylborane on the β-position of porphyrin resulted in red shift of the Soret band and the Q-bands. The Soret band of triarylborane porphyrin 4 and 5 are red shifted by 10 nm compared to H2TPP and ZnTPP respectively (Table 1).
Table 1 Absorption and emission data of triarylborane porphyrin 4 and 5
Compound |
λabsa (nm) |
λem (nm) |
Φfc |
boryl abs. band |
Soret band |
εb (M−1 cm−1) × 103 |
Q-bands |
Measured in dichloromethane at T = 25 °C, λabs (nm): absorption maximum of the Soret band. ε, extinction coefficient. Determined by using H2TPP as a standard (Φst = 0.11). |
TPP |
— |
418 |
— |
515, 551, 589, 647 |
650, 716 |
0.11 |
ZnTPP |
— |
422 |
— |
549, 587 |
595, 645 |
|
4 |
328 |
428 |
577 |
523, 559, 602, 658 |
663, 730 |
0.20 |
5 |
333 |
432 |
439 |
556, 592 |
604, 659 |
0.12 |
The emission properties of the β-substituted triarylborane porphyrin 4 and 5 were studied by steady state fluorescence technique. Their emission spectra are shown in Fig. 2. The triarylborane porphyrin 4 and 5 show considerable red shift in fluorescence maxima compared to TPP and ZnTPP respectively. The porphyrins 4 and 5 show enhanced fluorescence quantum yields of 0.20 and 0.12 respectively. These results indicate that there is considerable electronic communication between β-substituted boryl unit and the porphyrin core.
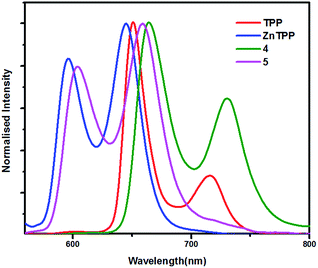 |
| Fig. 2 Emission spectra of TPP, ZnTPP and triarylborane substituted porphyrin 4 and 5 at 1.0 × 10−6 M concentration, the excitation wavelength was 418 nm for TPP, 422 nm for ZnTPP, 428 nm for 4, and 432 nm for 5 in DCM. | |
Anion binding studies
The sensing ability of the triarylborane–porphyrins 4 and 5 were investigated by absorption and emission studies, using various anions (F−, Cl−, Br−, I− and CN−) as their tetrabutylammonium salts. The absorption and emission studies show no significant change in the presence of Cl−, Br−, I− and CN− anions. However porphyrins 4 and 5 exhibit significant change in the absorption and emission spectra in the presence of fluoride anion. The binding constant for fluoride anion was determined from the emission data by using the Benesi–Hildebrand plot analysis.28–30
The titration result for triarylborane porphyrin 4 with fluoride anion is shown in Fig. 3. The absorption titration experiment of triarylborane porphyrin 4 shows blue shifted (ca. 420 nm) Soret band upon increasing the concentration of TBAF. The emission titration spectra exhibits gradual quenching of the emission band at 663 and 730 nm upon increasing the fluoride ion concentration indicating the F− ion binding to the boron centre of porphyrin 4 (Fig. 3). The binding constant was found to be 1.0 × 106 M−1, (log
K = 6.0) by the Benesi–Hildebrand plot (Fig. S1†).
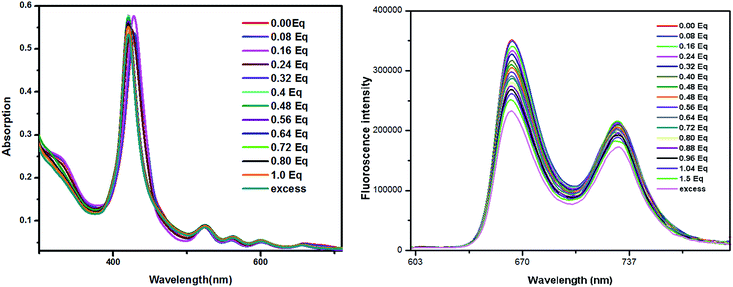 |
| Fig. 3 Absorption and fluorescence titration spectra of triarylborane porphyrin 4 with TBAF in 1.0 × 10−6 M DCM solution at λex = 428 nm. | |
The electronic absorption spectra of fluoride ion titration of metalated triarylborane porphyrin 5 shows initial decrease in intensity of Soret band, and gradual bathochromic shift of the Soret band from 432 nm to 443 nm (Fig. 4). The Q-bands at 556 and 592 nm were also shifted to 578 and 618 nm respectively. In the emission spectra, the addition of fluoride ions to a solution of metalated triarylborane porphyrin 5 results in a substantial decrease in the intensity of the emission band at 604 and 659 nm. The binding constant towards the fluoride ion was found 5.0 × 105 M−1, (log
K = 5.69) using the Benesi–Hildebrand plot (Fig. S1†).
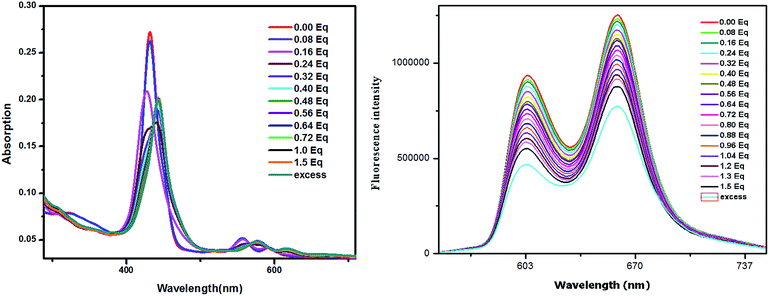 |
| Fig. 4 Absorption and Fluorescence titration spectra of metalated triarylborane porphyrin 5 with TBAF in 1.0 × 10−6 M DCM solution at λex = 432 nm. | |
The meso substituted triarylborane porphyrin 6 with one triarylborane unit as reported by Kubo et al. exhibits the binding constant 99
700 M−1 (log
K = 4.99) in THF, whereas the β-substituted porphyrin 4 and 5 with one triarylborane substitutent show the binding constant log
K = 6.0 and log
K = 5.69 respectively. This observation suggests that β-substituted triarylborane porphyrins show better binding compared to meso substituted triarylborane porphyrin 6 which has donor group present on meso triarylborane substituents. Moreover, Thilagar et al. have reported the binding of each triarylborane unit in porphyrin 7 and 8, the binding constant of first triarylborane unit is log
K1 = 5.2, which is comparable to that of β-substituted porphyrin 4 and 5 (Table 2).
Table 2 Comparative study of binding constants
Compound |
Binding constanta |
Solvent |
All values are derived experimentally. Literature reported values. |
Porphyrin 6b |
99 700(±0.02) M−1; log K = 4.99 |
THF |
Porphyrin 7b |
log K1 = 5.2, log K2 = 11.0, log K3F = 16.5, log K4F = 21.6 |
DCM |
Porphyrin 8b |
log K1 = 5.3, log K2 = 10.6, log K3 = 16.1, log K4 = 21.4 |
DCM |
Porphyrin 4 |
1.0(±0.05) × 106 M−1; log K = 6.0 |
DCM |
Porphyrin 5 |
5.0(±0.05) × 105 M−1; log K = 5.69 |
DCM |
In order to gain further insight in the fluoride anion binding 1H NMR titration studies were performed for the triarylborane–porphyrins 4 and 5. The comparison of partial 1H NMR spectra of porphyrins 4 and 5 before and after addition of F− ion is presented in Fig. S10 and S11 (see ESI† for details).
The addition of fluoride ion in the triarylborane–porphyrin 4 results in lower frequency shift of peaks at 9.10 ppm and 6.90 ppm which are observed at 8.99 ppm and 6.62 ppm respectively. The inner NH proton peak of the porphyrin 4 is intact after binding with F− ion which shows the absence of deprotonation mechanism. The partial 1H NMR spectra of porphyrin 5 shows that the peaks at 9.26 and 6.88 ppm are shifted in the lower frequency region at 8.98 ppm and 6.61 ppm respectively upon addition of F− ion, which confirms that the fluoride ion is binding at the boron centre of the porphyrins.
Conclusion
In summary, β-substituted triarylborane porphyrin 4 and its Zn-metalated derivative 5 were designed and synthesized for selective anion detection. The absorption and emission studies exhibit strong electronic communication. The β-substituted triarylborane porphyrin reported here are potential candidate for optoelectronic application and their detailed study is currently in progress in our laboratory.
Experimental section
Chemicals were used as received unless otherwise indicated. All oxygen or moisture sensitive reactions were performed under nitrogen/argon atmosphere using standard Schlenk method. Triethylamine (TEA) was received from commercial source, and distilled on KOH prior to use. 1H NMR (400 MHz) was recorded on the Bruker Avance (III) 400 MHz, using CDCl3 as solvent. Tetramethylsilane (TMS) was used as reference for recording 1H (of residual proton; δ = 7.26 ppm), spectra in CDCl3. UV-visible absorption spectra of all compounds in dichloromethane were recorded on a Carry-100 Bio UV-visible Spectrophotometer. HRMS was recorded on Brucker-Daltonics, micrO TOF-Q II mass spectrometer. The quantum yields (Φ) were calculated using H2TPP (Φ = 0.11) as references. The association constant of the metal complex formed in solution has been estimated by using the standard Benesi–Hildebrand equation.
where I0 is the intensity before addition of anion, I is the intensity in the presence of F−, I1 is intensity upon saturation with anion, and Ka is the association constant of the complex formed. The solutions of anions were prepared (1 × 10−3 M) in CH2Cl2.
Fluorescence quantum yield
The fluorescence quantum yields (ΦF) of compounds 4–5 were calculated (eqn (1)) by the steady-state comparative method using H2TPP as a standard (Φst = 0.11). |
ΦF = Φst × Su/Sst × Ast/Au × n2Du/n2Dst
| (1) |
where ΦF is the emission quantum yield of the sample, Φst is the emission quantum yield of the standard, Ast and Au represent the absorbance of the standard and the sample at the excitation wavelength, respectively, while Sst and Su are the integrated emission band areas of the standard and the sample, respectively, and nDst and nDu are the solvent refractive index of the standard and the sample, and u and st refer to the unknown and the standard, respectively.
Synthesis and characterization
Synthesis of Compound 4. A solution of 2-bromo-5,10,15,20-tetraphenylporphyrin (100 mg, 0.14 mmol) and 4-ethynylphenyl dimesitylborane 3 (65 mg, 0.187 mmol) in toluene/triethylamine 5
:
1 (60 mL), was deaerated for 30 min with argon bubbling and then, Pd(dba)2 (40 mg, 0.07 mmol) and AsPh3 (170 mg, 0.55 mmol) were added. The solution was deaerated for further 5 min; after that, reaction was left under argon at 60 °C. After completion, the mixture was cooled at room temperature and the solvent was evaporated. The product was purified by column chromatography on silica gel eluting with CH2Cl2/Hexane. Rf value = 0.5 (DCM–Hexane 1
:
1). The product was further recrystallized from dichloromethane/methanol to give porphyrin 4 in 50% yield. Mp > 280 °C, 1H NMR (400 MHz, CDCl3): δ (ppm) 9.09 (s, 1H), 8.89 (s, 2H), 8.83 (d, 1H, J = 4.9 Hz), 8.80–8.74 (m, 3H), 8.25–8.18 (m, 8H), 7.80–7.73 (m, 9H), 7.67 (t, 2H), 7.58 (t, 1H), 7.49 (d, 2H, J = 4.9 Hz), 7.34 (d, 2H, J = 8.1 Hz), 6.88 (s, 4H), 2.36 (s, 6H), 2.07 (s, 12H), −2.66 (s, 2H). 13C NMR (100 MHz, CDCl3): δ = 142.08, 142.04, 141.82, 141.68, 141.19, 140.92, 138.81, 135.77, 134.61, 134.56, 134.45, 131.51, 128.85, 128.26, 127.93, 127.81, 127.31, 126.82, 126.78, 126.74, 120.49, 120.16, 120.14, 120.12, 99.21, 88.38, 23.51, 21.31. HRMS (ESI) m/z, calcd for MH+ (C70H55BN4): 962.4525; found: 962.4520.
Synthesis of Compound 5. A solution of Zn(OAc)2.2H2O (15 mg, 0.06 mmol) in MeOH (2 mL) was added to a solution of compound 4 (20 mg, 0.02 mmol) in 15 mL of chloroform and the reaction mixture was stirred for overnight at room temperature. The reaction mixture was concentrated in vacuo and further purified by column chromatography using dichloromethane/hexane. The porphyrin 5 was obtained as a purple colour solid in 80% yield. Mp > 280 °C, 1H NMR (400 MHz, CDCl3): δ (ppm) 9.26 (s, 1H), 8.97–8.86 (m, 5H), 8.78 (d, 1H), 8.28–8.15 (m, 8H), 7.81–7.70 (m, 9H), 7.66 (t, 2H), 7.59 (d, 1H), 7.51 (d, 2H, J = 9.8 Hz), 7.38 (d, 2H, J = 7.9 Hz), 6.89 (s, 4H), 2.36 (s, 6H), 2.08 (s, 12H). 13C NMR (100 MHz, CDCl3): δ = 150.04, 149.79, 149.68, 149.05, 147.10, 145.85, 141.54, 140.92, 140.62, 139.85, 138.26, 138.04, 137.74, 134.70, 133.38, 133.35, 133.12, 131.81, 131.33, 131.21, 130.75, 130.47, 127.60, 127.20, 126.63, 126.52, 126.36, 125.58, 124.56, 120.44, 120.08, 119.95, 113.03, 98.30, 87.51, 32.79, 30.90, 30.57, 28.66, 28.33, 28.12, 27.97, 22.45, 21.66, 20.24, 13.10. HRMS (ESI) m/z, calculated for MH+ (C70H53BN4Zn): 1025.3739; found: 1025.3922.
Acknowledgements
The work was supported by DST and CSIR, Govt. of India, New Delhi. We gratefully acknowledge Sophisticated Instrumentation Centre (SIC), IIT Indore.
Notes and references
-
(a) S. Yamaguchi, S. Akiyama and K. Tamao, J. Am. Chem. Soc., 2000, 122, 6335–6336 CrossRef CAS;
(b) S. Yamaguchi, S. Akiyama and K. Tamao, J. Am. Chem. Soc., 2001, 123, 11372–11375 CrossRef CAS PubMed.
-
(a) S. Yamaguchi, T. Shirasaka, S. Akiyama and K. Tamao, J. Am. Chem. Soc., 2002, 124, 8816–8817 CrossRef CAS PubMed;
(b) Y. H. Lee, N. V. Nghia, M. J. Go, J. Lee, S. U. Lee and M. H. Lee, Organometallics, 2014, 33, 753–762 CrossRef CAS.
- V. N. Nemykin, C. D. Barrett, R. G. Hadt, R. I. Subbotin, A. Y. Maximov, E. V. Polshin and A. Y. Koposov, Dalton Trans., 2007, 3378–3389 RSC.
- P. Tagliatesta, A. Lembo and A. Leoni, New J. Chem., 2013, 37, 3416–3419 RSC.
- B. Xu, H. Fang, F. Chen, H. Lu, J. He, Y. Li, Q. Chen, H. Sun and W. Tian, New J. Chem., 2009, 33, 2457–2464 RSC.
-
(a) S. Solé and F. P. Gabbaï, Chem. Commun., 2004, 1284–1285 RSC;
(b) X. Y. Liu, D. R. Bai and S. Wang, Angew. Chem., Int. Ed., 2006, 45, 5475–5478 CrossRef CAS PubMed.
-
(a) M. Melaimi and F. P. Gabbaı, J. Am. Chem. Soc., 2005, 127, 9680–9681 CrossRef CAS PubMed;
(b) Z. Q. Liu, M. Shi, F. Y Li, Q. Fang, Z. H. Chen, T. Yi and C. H. Huang, Org. Lett., 2005, 7, 5481–5484 CrossRef CAS PubMed;
(c) T. Jadhav, R. Maragani, R. Misra, V. Sreeramulu, D. Narayana Rao and S. M. Mobin, Dalton Trans., 2013, 42, 4340–4342 RSC.
- S. Yamaguchi, T. Shirasaka and K. Tamao, Org. Lett., 2000, 2, 4129–4132 CrossRef CAS PubMed.
- Y. W. Liu, M. X. Kao and A. Wu, Sens. Actuators, B, 2015, 429–435 CrossRef CAS PubMed.
- C. Saravanan, S. Easwaramoorthi, C. Hsiow, K. Wang, M. Hayashi and L. Wang, Org. Lett., 2014, 16, 354–357 CrossRef CAS PubMed.
- M. S. Yuan, Z. Q. Liu and Q. Fang, J. Org. Chem., 2007, 72, 7915–7922 CrossRef CAS PubMed.
- A. K. Mahapatra, S. K. Manna, B. Pramanik, K. Maiti, S. Mondal, S. S. Alia and D. Mandal, RSC Adv., 2015, 5, 10716–10721 RSC.
- Z. Lin, Y. Ma, X. Zheng, L. Huang, E. Yang, C. Wu, T. J. Chow and Q. Ling, Dyes Pigm., 2015, 113, 129–137 CrossRef CAS PubMed.
- J. Liu, X. He, J. Zhang, T. He, L. Huang, J. Shen, D. Li, H. Qiu and S. Yin, Sens. Actuators, B, 2015, 538–545 CrossRef CAS PubMed.
- C. R. Wade and F. P. Gabbaï, Organometallics, 2011, 30, 4479–4481 CrossRef CAS.
- D. Cao, H. Zhao and F. P. Gabbaï, New J. Chem., 2011, 35, 2299–2305 RSC.
-
(a) C. R. Wade, A. E. J. Broomsgrove, S. Aldridge and F. P. Gabbaï, Chem. Rev., 2010, 110, 3958–3984 CrossRef CAS PubMed;
(b) R. Misra, B. Dhokale, T. Jadhav and S. M. Mobin, New J. Chem., 2014, 38, 3579–3585 RSC.
- I. S. Turan, F. P. Cakmak and F. Sozmen, Tetrahedron Lett., 2014, 55, 456–459 CrossRef PubMed.
-
(a) J. R. Farley, J. E. Wergedal and D. J. Baylink, Science, 1983, 222, 330–332 CAS;
(b) M. Kleerekoper, Endocrinol. Metab. Clin. North Am., 1998, 27, 441–452 CrossRef CAS;
(c) Z. Yang, K. Zhang, F. Gong, S. Li, J. Chen, J. S. Ma, L. N. Sobenina, A. I. Mikhaleva, G. Yang, B. Trofimov and A. Beilstein, J. Org. Chem., 2011, 7, 46–52 CAS;
(d) H. S. Horowitz, J. Publ. Health Dent., 2003, 63, 3–8 CrossRef PubMed;
(e) M. A. Lennon, Bull. W. H. O., 2006, 84, 759–760 CrossRef.
- Y. Kubo, M. Yamamoto, M. Ikeda, M. Takeuchi, S. Shinkai, S. amaguchi and K. Tamao, Angew. Chem., Int. Ed., 2003, 42, 2036–2040 CrossRef CAS PubMed.
- C. A. Swamy, S. Mukherjee and P. Thilagar, Anal. Chem., 2014, 86, 3616–3624 CrossRef PubMed.
-
(a) R. Sharma, P. Gautam, S. M. Mobin and R. Misra, Dalton Trans., 2013, 42, 5539–5545 RSC;
(b) P. Gautam, B. Dhokale, V. Shukla, P. C. Singh, K. S. Bindra and R. Misra, J. Photochem. Photobiol., A, 2012, 239, 24–27 CrossRef CAS PubMed.
- A. D. Adler, F. R. Longo, J. D. Finarelli, J. Goldmacher, J. Assour and L. Korsakoff, J. Org. Chem., 1967, 32, 476 CrossRef CAS.
-
(a) G. Y. Gao, J. V. Ruppel, B. D. Allen, Y. Chen and P. X. Zhang, J. Org. Chem., 2007, 72, 9060–9066 CrossRef CAS PubMed;
(b) M. Yeung, A. C. H. Ng, M. G. B. Drew, E. Vorpagel, E. M. Breitung, R. McMahon and D. K. P. Ng, J. Org. Chem., 1998, 63, 7143–7150 CrossRef CAS PubMed.
- H. Sun, X. Dong, S. Liu, Q. Zhao, X. Mou, H. Y. Yang and W. Huang, J. Phys. Chem. C, 2011, 115, 19947–19954 CAS.
-
(a) J. S. Lindsey, S. Prataphan, T. E. Johnson and R. W. Wagner, Tetrahedron, 1994, 50, 8941–8968 CrossRef CAS;
(b) R. W. Wagner, T. E. Johnson and J. S. Lindsey, J. Am. Chem. Soc., 1996, 118, 1166–11180 Search PubMed;
(c) R. W. Wagner, T. E. Johnson, F. Li and J. S. Lindsey, J. Org. Chem., 1995, 60, 5266–5273 CrossRef CAS.
-
(a) P. D. Rao, S. Dhanalekshmi, B. J. Littler and J. S. Lindsey, J. Org. Chem., 2000, 65, 7323–7344 CrossRef CAS PubMed;
(b) S. Tamaru, L. Yu, W. J. Youngblood, K. Muthukumaran, M. Taniguchi and J. S. Lindsey, J. Org. Chem., 2004, 69, 765–777 CrossRef CAS PubMed.
- S. Das, S. S. Misra, P. K. Sahu, A. Nijamudheen, V. Mohan and M. Sarkar, Chem. Phys. Lett., 2012, 546, 90–95 CrossRef CAS PubMed.
-
(a) S. Hamai, Bull. Chem. Soc. Jpn., 1982, 55, 2721 CrossRef CAS;
(b) M. L. Benesi and J. H. Hildebrand, J. Am. Chem. Soc., 1949, 71, 2703–2707 CrossRef;
(c) P. Das, A. Chakrabarty, B. Halder, A. Mallick and N. Chattopadhyay, J. Phys. Chem. B, 2007, 111, 7401–7408 CrossRef CAS PubMed.
- Y. Fu, X. J. Jiang, Y. Y. Zhu, B. Jiang, Z. Shuang, Q. Zang, M. S. Tang, H. Yan Zhang and C. W. Maka, Dalton Trans., 2014, 43, 12624–12632 RSC.
Footnote |
† Electronic supplementary information (ESI) available: General experimental methods, and copies of 1H NMR, and HRMS spectra of all new compounds. See DOI: 10.1039/c5ra03931b |
|
This journal is © The Royal Society of Chemistry 2015 |
Click here to see how this site uses Cookies. View our privacy policy here.