DOI:
10.1039/C5RA03403E
(Paper)
RSC Adv., 2015,
5, 32290-32297
Effect of freezing methods on sulforaphane formation in broccoli sprouts
Received
24th February 2015
, Accepted 23rd March 2015
First published on 23rd March 2015
Abstract
Broccoli sprouts were directly frozen at −20 °C (DF-20), −40 °C (DF-40) and −80 °C (DF-80) or stored at −20 °C (LN-20), −40 °C (LN-40) and −80 °C (LN-80) after being frozen in liquid nitrogen for 5 min or always frozen in liquid nitrogen (LN). The effect of these treatments on glucoraphanin and ascorbic acid content, myrosinase activity, sulforaphane and sulforaphane nitrile formation in broccoli sprouts was investigated. The results showed that glucoraphanin content was not significantly affected by freezing. Treatments of DF-20, DF-40, LN-20 and LN-40 for 24 and 48 h enhanced myrosinase activity and decreased the ascorbic acid content. The enhancement of myrosinase activity after freezing was in parallel with more sulforaphane and less sulforaphane nitrile formation. Besides, freezing at −20 °C was more favorable for sulforaphane formation than −40 °C. Directly freezing at −20 °C increased the sulforaphane yield by 1.54–2.11 fold in the broccoli sprouts of the three cultivars investigated in this study.
1 Introduction
Broccoli sprouts have considerably high levels of glucosinolates compared with broccoli florets, especially glucoraphanin, which is an important class of sulfur-containing secondary metabolites and accounts for more than 50% of total glucosinolate content.1,2 In intact broccoli sprouts cells, glucoraphanin is found in the vacuoles and is spatially separated from myrosinase, which is in myrosin cells.3 When tissue is damaged, for example by cutting or chewing, glucoraphanin comes into contact with myrosinase and is converted into sulforaphane and sulforaphane nitrile.4 Sulforaphane is a naturally potent inducer of phase II detoxification enzymes like glutathione S-transferase and quinone reductase.5,6 Many studies have demonstrated the efficacy of sulforaphane as a cancer preventative agent in a number of animal models of carcinogenesis.7–9 In addition, sulforaphane also exhibits other biological activities such as anti-inflammatory, anti-bacterial and cardioprotection.10 However, the conversion rate of glucoraphanin to sulforaphane is very low and sulforaphane nitrile predominates over sulforaphane in many cultivars.4,11 In the presence of the epithiospecifier protein (ESP), glucoraphanin degrades to sulforaphane nitrile, which decreases the sulforaphane yield and does not provide the same health benefits.4,12 Hence, the increase of sulforaphane formation from glucoraphanin is crucial for anticancer activity.
Recently, numerous studies have investigated the changes of glucoraphanin and sulforaphane formation in broccoli during storage, processing and cooking.13–16 Thermal processing such as steaming and microwave treatment increased sulforaphane formation and decreased sulforaphane nitrile formation. However, heating time should be strictly controlled because myrosinase may be denatured by overcooking.14 Freezing is one of the most frequently used methods for long-term storage, which can effectively preserve the nutritive constituents of food.17 The quality of frozen food is closely related to the freezing time and temperature. It has been shown that long-term freezer storage of blanched cauliflower did not affect total glucosinolates, glucoraphanin and anthocyanin, but decreased ascorbic acid content.17 Cieślik et al.16 also found that glucosinolate content in different cruciferous vegetables did not change after blanching and freezing for 48 h at −22 °C. However, freezing decreased ascorbic acid, polyphenols and antioxidant activity in broccoli, but resulted in more bioactive compounds and a higher antioxidant activity when broccoli was stored at −30 °C compared to that stored at −20 °C.18 Moreover, when broccoli is processed commercially for freezing, it usually undergoes blanching. Blanching is a thermal treatment using steam or hot water to inactivate myrosinase, yielding a broccoli product with no ability to form sulforaphane. Nevertheless, broccoli sprouts can be eaten raw without thermal treatment. Broccoli sprouts can also be used as functional food materials. However, little information is available in previous studies on the effect of freezing storage on the formation of sulforaphane in broccoli sprouts.
Hence, the objective of this study was to investigate the effect of freezing for 48 h on glucoraphanin and ascorbic acid content, myrosinase activity and the formation of sulforaphane and sulforaphane nitrile in broccoli sprouts.
2 Materials and methods
2.1 Plant material, cultivation conditions and treatment
Three cultivars of broccoli seeds (B. oleracea var. italica cv. Lvxiong, cv. Mantuolv and cv. Huilvzaosheng) were obtained from Nanjing Lixiang Seed Co. (Jiangsu, China). The seeds were rinsed in distilled water, immersed in 5 g L−1 sodium hypochlorite for 15 min and drained, then were washed with distilled water until they reached a neutral pH and were soaked in distilled water for 4 h at 30 °C. After pouring off the soaking water, the seeds were spread evenly on trays filled with vermiculite and were irrigated with distilled water. The trays were transferred to a controlled environment chamber with a 16 h light/8 h dark cycle and an air temperature of 25 °C. The sprouts were watered with 20 mL distilled water every 12 h. Finally, the 7 day-old sprouts were rapidly and gently collected, weighed, and kept in polyethylene bags, then treated differently. For each treatment, three replicates were taken for analysis. Experiment designs were as below.
Selection of freezing methods. (a) The 7 day-old Lvxiong sprouts were directly frozen at −20 °C (DF-20), −40 °C (DF-40) and −80 °C (DF-80). (b) Samples were frozen in liquid nitrogen for 5 min and then stored at −20 °C (LN-20), −40 °C (LN-40) and −80 °C (LN-80). (c) Samples were always frozen in liquid nitrogen (LN). The treatments were conducted for 6, 24 and 48 h.
Verification of the selected freezing methods. The 7 day-old sprouts of Mantuolv and Huilvzaosheng were directly frozen at −20 °C (DF-20) and −40 °C (DF-40) for 6, 24 and 48 h.
2.2 Extraction and determination of glucoraphanin
The extraction and analysis of glucoraphanin was performed according to the procedure previously reported by Guo et al.19 Samples (0.50 g) were ground and homogenized in a mortar before thawing with 2 mL of 75% boiling methanol, and then the homogenized samples were transferred to a new tube and extracted for 15 min at 80 °C. After recovery of the supernatant, the residues were extracted again. Sinigrin (2-propenyl glucosinolate) (Sigma-Aldrich, USA) was added to each sample and used as an internal standard for calculation of molar concentrations of glucoraphanin. An Agilent 1200 HPLC system (Agilent Technologies Co. Ltd., USA) with an Eclipse XDB-C18 column (5 μm particle size, 4.6 × 150 mm; Agilent Technologies Co. Ltd., USA) was used for analysis of glucoraphanin. The glucoraphanin content was expressed as μmol per g dry weight (DW) of broccoli sprouts.
2.3 Myrosinase activity determination
Myrosinase activity was determined according to the method of Guo et al.19 A total of 500 mg of each sample was homogenized with 3 mL of 0.1 mol L−1 sodium-phosphate buffer (pH 6.5) in an ice bath, and then centrifuged at 10
000g for 15 min at 4 °C. The supernatant was used as the crude enzyme. The protein content in the crude enzyme was determined according to Bradford20 using bovine serum albumin as a standard. The myrosinase assays followed the procedure of Guo et al.19 The amount of glucose was measured using a Glucose GOD/PAP Kit (Nanjing Jiancheng Biotech Inc. (Jiangsu, China)). One myrosinase unit corresponded to 1 nmol of glucose formed per minute. The specific activity was expressed as units per milligram of protein.
2.4 Ascorbic acid content determination
Ascorbic acid content was determined according to the method described by Guo et al.21 Briefly, 200 mg of sprouts were homogenized with 4 mL of 1.0% (w/v) oxalic acid, centrifuged at 10
000 g for 10 min. Separation and detection were performed using an Agilent 1200 HPLC system (Agilent Technologies Co. Ltd., USA) with a ZORBAX SB-C18 column (5 μm particle size, 4.6 × 250 mm; Agilent Technologies Co. Ltd., USA) at 254 nm. Ascorbic acid was quantified by external calibration and results were recorded as mg per g DW.
2.5 Sulforaphane and sulforaphane nitrile formation determination
The extraction of sulforaphane and sulforaphane nitrile was carried out using a procedure previously described by Gu et al.22 with minor modifications. A total of 500 mg of broccoli sprouts were homogenized with 4 mL of distilled water, then hydrolyzed at 37 °C for 3 h. After hydrolyzing, it was extracted three times with 9 mL of dichloromethane. The dichloromethane layer was dried over anhydrous sodium sulfate, and concentrated to about 100 μL in a nitrogen stream. Samples were analyzed using gas chromatography according to the method of Wang et al.14 with minor modifications. An Agilent 6890N GC system (Agilent Technologies Co. Ltd., USA) with a flame ionization detector (FID) and a fused silica capillary Agilent Technology HP-5 ms (5% phenyl methyl siloxane) column (30 m × 0.25 mm i.d., film thickness 0.1 μm) was used for analysis. The injector and detector temperatures were 210 and 280 °C, respectively. The column oven temperature was initially set at 40 °C for 2 min, then gradually increased to 260 at a rate of 10 °C min−1, and held for 5 min. The carrier gas was helium, at 25 psi. Sulforaphane and benzonitrile (Sigma-Aldrich, USA) were used as standards. The content of sulforaphane and sulforaphane nitrile was expressed as mg per g DW of broccoli sprouts.
2.6 Statistical analyses
Experimental data were expressed as the mean ± standard deviation (SD) with three replications (n = 3). SPSS 18.0 (SPSS Inc., Chicago, IL, USA) was applied for significant difference tests. Data were analyzed by one-way ANOVA, followed by Duncan’s multiple-range tests. A difference at p < 0.05 was considered to be statistically significant.
3 Results
3.1 The effect of freezing methods on glucoraphanin content, sulforaphane and sulforaphane nitrile formation in Lvxiong sprouts
The levels of glucoraphanin in fresh and frozen Lvxiong sprouts are shown in Fig. 1A. No significant changes were found in the glucoraphanin content in Lvxiong sprouts under different freezing treatments (p > 0.05). Treatments of DF-20, LN-20, DF-40 and LN-40 significantly enhanced sulforaphane formation (Fig. 1B) and decreased sulforaphane nitrile formation (Fig. 1C) in Lvxiong sprouts compared with the control (p < 0.05). However, treatments of LN, DF-80, and LN-80 resulted in an insignificant difference in sulforaphane and sulforaphane nitrile formation compared with the control (p > 0.05). Sulforaphane content in Lvxiong sprouts under treatments of DF-20, LN-20, DF-40 and LN-40 for 24 and 48 h was significantly higher than treatments for 6 h. No significant difference was observed in sulforaphane formation between freezing for 24 and 48 h at the same freezing temperature. On the other hand, sulforaphane nitrile formation in Lvxiong sprouts under DF-20 and DF-40 treatments was lower than that of the control, and its formation decreased constantly with freezing time up to 24 h and was then stable from 24 to 48 h. The sulforaphane content in Lvxiong sprouts under treatments of DF-20, LN-20, DF-40 and LN-40 for 24 h was 3.11-, 3.09-, 2.62- and 2.41-fold of that of the control, respectively. Simultaneously, the sulforaphane nitrile content decreased by 85.5%, 87.8%, 52.2% and 47.9% compared with the control, respectively. After DF-20 and DF-40 treatments for 24 h, the sulforaphane content was 10.4- and 2.70-fold that of sulforaphane nitrile, respectively.
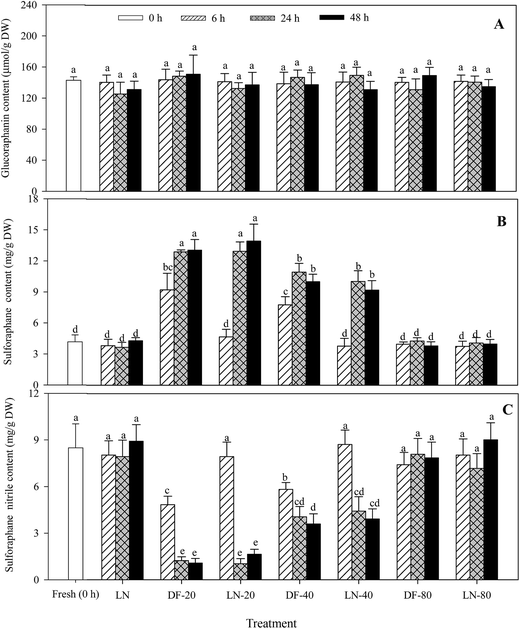 |
| Fig. 1 The effect of freezing methods on glucoraphanin content (A), sulforaphane (B) and sulforaphane nitrile (C) formation in Lvxiong sprouts. LN: sprouts were always frozen in liquid nitrogen; DF-20, DF-40 and DF-80: sprouts were directly and always frozen at −20 °C, −40 °C and −80 °C, respectively; LN-20, LN-40 and LN-80: sprouts were stored at −20 °C, −40 °C and −80 °C after being frozen in liquid nitrogen for 5 min, respectively. Each point was expressed as mean ± SD (n = 3). The different letters indicate statistical differences at p < 0.05. | |
3.2 The effect of freezing methods on myrosinase activity in Lvxiong sprouts
Treatments of DF-20, LN-20, DF-40 and LN-40 for 24 and 48 h significantly increased myrosinase activity in Lvxiong sprouts (p < 0.05), but no significantly different changes were found in myrosinase activity under treatments of LN, DF-80 and LN-80 compared with the control (p > 0.05) (Fig. 2). Myrosinase activity in Lvxiong sprouts treated with DF-20, LN-20, DF-40 and LN-40 for 24 and 48 h was significantly higher than that at 6 h. No significant difference was observed among the treatments of DF-20 and LN-20 for 24 and 48 h. After treatments of DF-20 and DF-40 for 24 h, myrosinase activity increased by 117% and 83.4% when compared with the control, respectively.
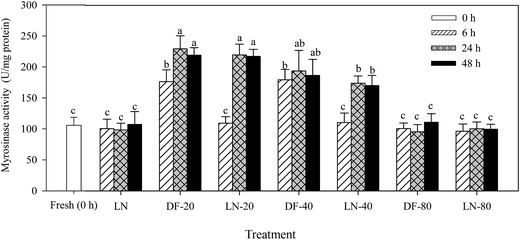 |
| Fig. 2 The effect of freezing methods on myrosinase activity in Lvxiong sprouts. LN: sprouts were always frozen in liquid nitrogen; DF-20, DF-40 and DF-80: sprouts were directly and always frozen at −20 °C, −40 °C and −80 °C respectively; LN-20, LN-40 and LN-80: sprouts were stored at −20 °C, −40 °C and −80 °C after being frozen in liquid nitrogen for 5 min, respectively. Each point was expressed as mean ± SD (n = 3). The different letters indicate statistical differences at p < 0.05. | |
3.3 The effect of freezing methods on ascorbic acid content in Lvxiong sprouts
Treatments of DF-20, LN-20, DF-40 and LN-40 for 24 and 48 h significantly decreased the ascorbic acid content in Lvxiong sprouts (p < 0.05), but no significant difference was found in the ascorbic acid content under the treatments of LN, DF-80 and LN-80 in comparison with the control (p > 0.05) (Fig. 3). A decrease was found in ascorbic acid after treatment with DF-20 for 24 and 48 h compared with 6 h for the Lvxiong sprouts. However, no significant difference was observed after treatment with DF-40 for 6, 24 and 48 h (p > 0.05). Treatments of DF-20 and DF-40 for 24 h reduced the ascorbic acid content in Lvxiong sprouts by 37.7% and 24.2% respectively compared with the control.
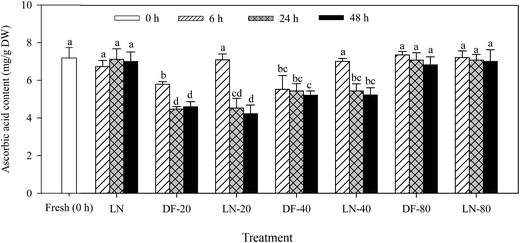 |
| Fig. 3 The effect of freezing methods on ascorbic acid content in Lvxiong sprouts. LN: sprouts were always frozen in liquid nitrogen; DF-20, DF-40 and DF-80: sprouts were directly and always frozen at −20 °C, −40 °C and −80 °C, respectively; LN-20, LN-40 and LN-80: sprouts were frozen in liquid nitrogen for 5 min, then stored at −20 °C, −40 °C and −80 °C, respectively. Each point was expressed as mean ± SD (n = 3). The different letters indicate statistical differences at p < 0.05. | |
3.4 The effect of DF-20 and DF-40 treatments on the glucoraphanin and ascorbic acid content, myrosinase activity and sulforaphane and sulforaphane nitrile formation in Mantuolv and Huilvzaosheng sprouts
Direct freezing is a simple and effective strategy to enhance the formation of sulforaphane in Lvxiong sprouts. Hence, we investigated the effect of DF-20 and DF-40 treatments on sulforaphane formation from glucoraphanin in the sprouts of the other two cultivars (Mantuolv and Huilvzaosheng). The results are presented in Table 1 and Fig. 4.
Table 1 The effect of DF-20 and DF-40 treatments on glucoraphanin (μmol per g dry weight) and ascorbic acid content (mg per g DW), and myrosinase activity (U mg per protein) in Mantuolv and Huilvzaosheng sproutsa
Index |
Cultivar |
Fresh (0 h) |
DF-20 |
DF-40 |
6 h |
24 h |
48 h |
6 h |
24 h |
48 h |
DF-20: sprouts were directly and always frozen at −20 °C, DF-40: sprouts were directly and always frozen at −40 °C. Each point was expressed as mean ± SD (n = 3). Values not sharing the same letter on the same line are significantly different at p < 0.05. |
Glucoraphanin |
Mantuolv |
34.12 ± 4.16 a |
30.93 ± 4.99 a |
32.04 ± 1.39 a |
33.15 ± 4.58 a |
30.10 ± 2.77 a |
30.24 ± 3.35 a |
34.95 ± 5.02 a |
Huilvzaosheng |
5.76 ± 0.90 a |
5.25 ± 0.64 a |
5.12 ± 0.65 a |
6.02 ± 0.91 a |
6.27 ± 1.15 a |
5.09 ± 0.89 a |
5.39 ± 0.77 a |
Ascorbic acid |
Mantuolv |
8.29 ± 0.16 a |
7.04 ± 0.09 b |
6.96 ± 0.41 b |
4.92 ± 0.12 c |
6.99 ± 0.36 b |
7.25 ± 0.78 b |
6.80 ± 0.82 b |
Huilvzaosheng |
9.10 ± 0.68 a |
5.91 ± 0.34 c |
5.22 ± 0.11 d |
4.96 ± 0.56 d |
7.60 ± 0.81 b |
7.08 ± 1.02 bc |
6.89 ± 0.19 b |
Myrosinase activity |
Mantuolv |
113.77 ± 11.81 b |
168.60 ± 6.82 a |
174.66 ± 32.96 a |
157.70 ± 10.86 a |
171.12 ± 9.46 a |
153.21 ± 25.64 a |
165.21 ± 15.94 a |
Huilvzaosheng |
110.51 ± 15.28 c |
146.17 ± 12.68 b |
190.77 ± 28.86 a |
202.26 ± 35.92 a |
159.32 ± 16.33 ab |
180.18 ± 27.98 ab |
173.80 ± 21.49 ab |
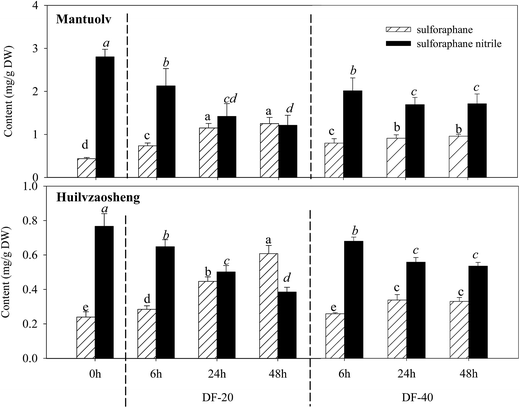 |
| Fig. 4 The effect of DF-20 and DF-40 treatments on the formation of sulforaphane and sulforaphane nitrile in Mantuolv and Huilvzaosheng sprouts. DF-20: sprouts were directly and always frozen at −20 °C, DF-40: sprouts were directly and always frozen at −40 °C. Each point was expressed as mean ± SD (n = 3). The different normal letters and italic letters indicate the statistical differences for each compound (p < 0.05). | |
Similar to Lvxiong sprouts, DF-20 and DF-40 treatments resulted in an insignificant difference in the glucoraphanin content in Mantuolv and Huilvzaosheng sprouts compared with the control (p > 0.05) (Table 1).
Treatments of DF-20 and DF-40 significantly decreased the ascorbic acid content in the two cultivar sprouts in comparison with the control (p < 0.05) (Table 1). For the two cultivars, no significant difference was observed in the ascorbic acid content of the sprouts treated with DF-40 for 6, 24 and 48 h (p > 0.05). DF-20 treatment resulted in more of a decrease in the ascorbic acid content in Huilvzaosheng sprouts than DF-40 treatment.
On the contrary, treatments of DF-20 and DF-40 significantly increased myrosinase activity in the two cultivar sprouts compared with the control (p < 0.05), but the freezing time and temperature had no significant difference for the same cultivar (Table 1). After treatment of DF-20 for 24 h, myrosinase activity increased by 53.5% and 72.6% in Mantuolv and Huilvzaosheng sprouts compared with the control, respectively.
Compared with the control, sulforaphane formation in the two cultivar sprouts was significantly increased and sulforaphane nitrile formation was decreased after treatment with DF-20 and DF-40 (p < 0.05), but no significant difference was observed between 24 and 48 h under the same treatment (Fig. 4). The sulforaphane content in the sprouts of the two cultivars under DF-20 treatment was higher than that under DF-40 treatment. After DF-20 treatment for 48 h, the sulforaphane content in Mantuolv and Huilvzaosheng sprouts was 2.86- and 2.54-fold that of the control, respectively. Meanwhile, the sulforaphane nitrile content decreased by 56.7% and 49.8% compared with the control, respectively.
4 Discussion
Glucoraphanin is the precursor of the chemoprotective isothiocyanate, sulforaphane. Therefore, its level is crucial for the level of sulforaphane formation. Variations in the relative content of glucoraphanin may occur between different plant cultivars.2,23 In the present study, glucoraphanin content presented a great difference among experimental cultivars. Lvxiong sprouts were found to have a greater glucoraphanin content than the other two cultivars, and this directly resulted in a higher sulforaphane yield. Freezing was found to be an excellent way to preserve the content of glucosinolates in the inflorescences of cauliflower and broccoli.24 Similar results were also observed in broccoli sprouts; freezing resulted in an insignificant change in the glucoraphanin content in the sprouts of the three cultivars. It suggested that glucoraphanin was not hydrolyzed by myrosinase. One reason might be that the damage caused by ice crystals was not sufficient to bring glucoraphanin into contact with myrosinase. Hence, the hydrolysis did not occur. Another reason might be that the sprouts were ground before thawing, and myrosinase did not exhibit a catalytic effect at −20 °C and −40 °C.25 The phenomenon in our study was also consistent with the previous report of Brown et al.,26 who reported that if plant tissues remain frozen, glucosinolates are stable in disrupted tissues.
In the present study, the treatments of DF-20 and DF-40 had a negative impact on the ascorbic acid content in the broccoli sprouts of the three cultivars. This result was in agreement with the previous study of Gębczyński and Lisiewska,18 who found that the ascorbic acid content significantly reduced in broccoli florets during freeze-storage. Moreover, the loss of ascorbic acid was related to the vegetable species and the freezing temperature.27 Freezing at −30 °C resulted in a lower loss of ascorbic acid in the broccoli florets compared with −20 °C.27 Similarly, DF-20 treatment for 48 h resulted in a higher loss of ascorbic acid than DF-40 treatment in this study. This phenomenon could probably be due to the higher oxidation level of ascorbic acid at −20 °C.27 On the other hand, the treatments of LN, DF-80 and LN-80 could well preserve ascorbic acid in the Lvxiong sprouts against oxidation. A possibility could be that the higher temperature could result in more destructive ice crystals which would damage the plant tissue, resulting in the oxidation of ascorbic acid. Additionally, when Lvxiong sprouts were treated with LN-20 and LN-40 for 6 h, the ascorbic acid content remained at the same level as the control. However, the ascorbic acid content decreased significantly after treatments with LN-20 and LN-40 for 24 and 48 h. It may be assumed that ice crystals could become larger after treatment with LN-20 and LN-40 for 24 and 48 h due to the increase in the temperature, thus resulting in tissue damage.
Myrosinase activity in the three cultivars was enhanced by treatments of DF-20 and DF-40 in this study. Treatments of LN-20 and LN-40 for 24 and 48 h also enhanced myrosinase activity in Lvxiong sprouts compared with the control, whereas myrosinase activity was not affected by treatments of LN-80, DF-80 and LN. Previous studies showed that the level of ascorbic acid had some impact on myrosinase activity.28,29 Low and moderate concentrations of ascorbic acid significantly improved myrosinase activity in broccoli seeds and sprouts, while excessive ascorbic acid actually inhibited the activity and thus resulted in less isothiocyanate and sulforaphane formation.29–31 In the present study, an opposite changing trend was observed in the ascorbic acid content and myrosinase activity in the process of freezing. The increase in myrosinase activity under treatments of DF-20, LN-20, DF-40 and LN-40 could probably be due to the decrease in the original high ascorbic acid content in the broccoli sprouts. This suggested that myrosinase activity in broccoli sprouts could be regulated by regulating ascorbic acid content during the freezing process. After freezing for 48 h, DF-20 resulted in a lower ascorbic acid content than DF-40; however, no significant difference was observed in myrosinase activity between the DF-20 and DF-40 treatments. It is necessary to conduct further research to clearly define the accurate relationship between the ascorbic acid content and myrosinase activity during the freezing process.
In the present study, the sulforaphane nitrile yield from glucoraphanin in the fresh broccoli sprouts of three cultivars was higher than the sulforaphane yield, similar to the results obtained for broccoli seeds and florets.29,32 The endogenous sulforaphane yield was significantly higher for fresh Lvxiong sprouts than for those of Mantuolv and Huilvzaosheng as was the sulforaphane nitrile yield, which was similar to the glucoraphanin content in the sprouts of the three cultivars. The formation of sulforaphane in broccoli sprouts might result not only from a difference in the glucoraphanin content but also from differences in myrosinase and ESP activity. ESP, a non-catalytic cofactor of myrosinase, is capable of directing the formation of sulforaphane nitrile at the expense of bioactive sulforaphane.12 The research of Wang et al.14 indicated that steaming, superior to boiling and microwave heating, provided a lower nitrile and a higher sulforaphane yield from broccoli. They speculated that steaming would result in a greater retention of myrosinase and destruction of ESP. Our data showed that treatments of DF-20, LN-20, DF-40 and LN-40 for 24 and 48 h significantly improved sulforaphane formation and reduced sulforaphane nitrile formation in broccoli sprouts which might result from the increase in myrosinase activity after freezing. On the other hand, sulforaphane formation in Lvxiong sprouts was not improved by treatments of LN-80, DF-80 and LN. The likely explanation for this was the fact that myrosinase activity did not increase after treatment with LN-80, DF-80 and LN. Moreover, the conversion capability of glucoraphanin into sulforaphane was limited in broccoli seeds and fresh broccoli by the action of endogenous myrosinase.11,29 In our study, treatments of DF-20 and DF-40 increased the activity of endogenous myrosinase in broccoli sprouts of the three cultivars, enhancing sulforaphane production from glucoraphanin.
Approximately 86% of sulforaphane nitrile was lost in the Lvxiong sprouts after DF-20 treatment for 48 h compared with the control, which was higher than the other two cultivars in this study. This result might probably be due to the greater enhancement of myrosinase activity in the Lvxiong sprouts treated with DF-20 treatment compared with the Mantuolv and Huilvzaosheng sprouts. It was also possible that ESP activity in the three cultivar sprouts decreased differently after treatment of DF-20. Treatments of DF-20 and LN-20 were more conducive to the formation of sulforaphane than DF-40 and LN-40; however, no significant difference was observed in myrosinase activity after freezing at −20 °C and −40 °C. This phenomenon could be due to the difference of ESP activity variation in sprouts at −20 °C and −40 °C. Further studies are needed to explain the changes in the ESP activities and the mechanism of sulforaphane formation in broccoli sprouts during freezing at −20 °C and −40 °C.
5 Conclusion
Freezing at −20 °C and −40 °C (DF-20, LN-20, DF-40 and LN-40) resulted in the formation of more sulforaphane and a decrease in the formation of the alternative product, sulforaphane nitrile, which was probably due to the enhancement of myrosinase activity via a decrease of the ascorbic acid content. The sprouts of three cultivars frozen at −20 °C (DF-20 and LN-20) contained higher levels of sulforaphane than those frozen at −40 °C (DF-40 and LN-40). It indicated that freezing at −20 °C was an effective method to enhance the yield of sulforaphane and improve the health benefit of broccoli sprouts. These results can provide valuable information for the production of broccoli sprouts in functional foods.
Conflict of interest
The authors declare no conflict of interest.
Acknowledgements
Financial support was provided by the National Natural Science Foundation of China (Grant no. 31271912). The present study was also a project funded by the Priority Academic Program Development of Jiangsu Higher Education Institutions (PAPD).
References
- A. F. Brown, G. G. Yousef, E. H. Jeffery, B. P. Klein, M. A. Wallig, M. M. Kushad and J. A. Juvik, J. Am. Soc. Hortic. Sci., 2002, 127, 807–813 CAS.
- S. Pérez-Balibrea, D. A. Moreno and C. García-Viguera, Food Chem., 2011, 125, 348–354 CrossRef PubMed.
- R. Kissen, J. T. Rossiter and A. M. Bones, Phytochem. Rev., 2009, 8, 69–86 CrossRef CAS.
- N. V. Matusheski and E. H. Jeffery, J. Agric. Food Chem., 2001, 49, 5743–5749 CrossRef CAS PubMed.
- M.-K. Kwak, N. Wakabayashi and T. W. Kensler, Mutat. Res., Fundam. Mol. Mech. Mutagen., 2004, 555, 133–148 CrossRef CAS PubMed.
- Y. Zhang, P. Talalay, C.-G. Cho and G. H. Posner, Proc. Natl. Acad. Sci. U. S. A., 1992, 89, 2399–2403 CrossRef CAS.
- Y. Li, T. Zhang, H. Korkaya, S. Liu, H. F. Lee, B. Newman, Y. Yu, S. G. Clouthier, S. J. Schwartz, M. S. Wicha and D. Sun, Clin. Cancer Res., 2010, 16, 2580–2590 CrossRef CAS PubMed.
- J. W. Fahey, X. Haristoy, P. M. Dolan, T. W. Kensler, I. Scholtus, K. K. Stephenson, P. Talalay and A. Lozniewski, Proc. Natl. Acad. Sci. U. S. A., 2002, 99, 7610–7615 CrossRef CAS PubMed.
- V. Villa-Cruz, J. Davila, M. T. Viana and R. Vazquez-Duhalt, Chemosphere, 2009, 74, 1145–1151 CrossRef CAS PubMed.
- M. Traka and R. Mithen, Phytochem. Rev., 2009, 8, 269–282 CrossRef CAS.
- R. Mithen, K. Faulkner, R. Magrath, P. Rose, G. Williamson and J. Marquez, Theor. Appl. Genet., 2003, 106, 727–734 CAS.
- Z. Gu, Q. Guo and Y. Gu, J. Integr. Agric., 2012, 11, 1804–1816 CrossRef CAS.
- G. Yuan, B. Sun, J. Yuan and Q. Wang, J. Zhejiang Univ., Sci., B, 2009, 10, 580–588 CrossRef PubMed.
- G. C. Wang, M. Farnham and E. H. Jeffery, J. Agric. Food Chem., 2012, 60, 6743–6748 CrossRef CAS PubMed.
- N. V. Matusheski, J. A. Juvik and E. H. Jeffery, Phytochemistry, 2004, 65, 1273–1281 CrossRef CAS PubMed.
- E. Cieślik, T. Leszczynska, A. Filipiakflorkiewicz, E. Sikora and P. Pisulewski, Food Chem., 2007, 105, 976–981 CrossRef PubMed.
- J. Volden, G. B. Bengtsson and T. Wicklund, Food Chem., 2009, 112, 967–976 CrossRef CAS PubMed.
- P. Gębczyński and Z. Lisiewska, Innovative Food Sci. Emerging Technol., 2006, 7, 239–245 CrossRef PubMed.
- L. Guo, R. Yang, Z. Wang, Q. Guo and Z. Gu, J. Funct. Foods, 2014, 9, 70–77 CrossRef CAS PubMed.
- M. M. Bradford, Anal. Biochem., 1976, 72, 248–254 CrossRef CAS.
- L. Guo, R. Yang, Z. Wang, Q. Guo and Z. Gu, Int. J. Food Sci. Nutr., 2014, 65, 476–481 CrossRef CAS PubMed.
- Y. Gu, Q. Guo, L. Zhang, Z. Chen, Y. Han and Z. Gu, J. Agric. Food Chem., 2012, 60, 209–213 CrossRef CAS PubMed.
- L. G. West, K. A. Meyer, B. A. Balch, F. J. Rossi, M. R. Schultz and G. W. Haas, J. Agric. Food Chem., 2004, 52, 916–926 CrossRef CAS PubMed.
- A. S. Rodrigues and E. A. S. Rosa, J. Sci. Food Agric., 1999, 79, 1028–1032 CrossRef CAS.
- M. Springett and J. Adams, Food Chem., 1989, 33, 173–186 CrossRef CAS.
- P. Brown and M. Morra, Plant Soil, 1996, 181, 307–316 CrossRef CAS.
- Z. Lisiewska and W. Kmiecik, Food Chem., 1996, 57, 267–270 CrossRef CAS.
- G. C. Yen and Q. K. Wei, J. Sci. Food Agric., 1993, 61, 471–475 CrossRef CAS.
- L. Shen, G. Su, X. Wang, Q. Du and K. Wang, Food Chem., 2010, 119, 987–994 CrossRef CAS PubMed.
- Q. Guo, L. Guo, Z. Wang, Y. Zhuang and Z. Gu, Food Chem., 2013, 141, 1580–1586 CrossRef CAS PubMed.
- L. Ludikhuyze, L. Rodrigo and M. Hendrickx, J. Food Prot., 2000, 63, 400–403 CAS.
- R. B. Jones, C. L. Frisina, S. Winkler, M. Imsic and R. B. Tomkins, Food Chem., 2010, 123, 237–242 CrossRef CAS PubMed.
|
This journal is © The Royal Society of Chemistry 2015 |
Click here to see how this site uses Cookies. View our privacy policy here.