DOI:
10.1039/C5RA03073K
(Paper)
RSC Adv., 2015,
5, 35497-35505
Binding of a quaternary ammonium polymer-grafted-chitosan onto a chemically modified wool fabric surface: assessment of mechanical, antibacterial and antifungal properties
Received
17th February 2015
, Accepted 13th April 2015
First published on 13th April 2015
Abstract
It is quite challenging to bind any chemical compound or polymer onto a wool fibre surface because of the lack of any functional groups available on its surface. In this work, anionic sulphonate groups were introduced onto a wool fibre surface by grafting with polystyrene sulphonate, which enabled binding of cationic quaternized chitosan by ionic bonding. A bioactive quaternary ammonium polymer, poly[2-(acryloyloxy)ethyl]trimethylammonium chloride, was grafted onto chitosan to enhance chitosan's limited antimicrobial activity. Two types of wool fabrics, untreated and polystyrene sulphonate grafted, were coated with this quaternized chitosan. The antibacterial properties of the wool fabrics coated with quaternized chitosan were tested against both Gram-positive and Gram-negative bacteria, Staphylococcus aureus and Klebsiella pneumoniae and also against Aspergillus fumigatus fungus. The coated wool fabric surface was further characterised by attenuated total reflectance – Fourier transform infrared and Raman spectroscopy, scanning electron microscopy (SEM), and also by measuring tensile strength and surface contact angle. The grafting of wool fabric with polystyrene sulphonate improved its tensile strength and elongation, but coating with quaternized chitosan increased the strength further and reduced elongation at break. Before washing both types of wool fabrics showed strong bacterial resistance against both types of bacteria but marginal antifungal activity. However, after five cycles of washing the untreated wool fabric coated with quaternized chitosan almost completely lost antibacterial activity. SEM analysis reveals that most of the quaternized chitosan was removed by washing. However, the polystyrene sulphonate-grafted wool fabric coated with quaternized chitosan retained its antibacterial activity, indicating that surface sulphonate groups on wool fibre improve the durability of the quaternized chitosan to washing.
1. Introduction
A wide class of micro-organisms coexists in a natural equilibrium with the human body and living environments. However, a rapid and uncontrolled multiplication of even a few non-pathogenic microbes can seriously compromise hygiene and health standards. It is known that clothing and other textile materials can act as carriers for microbial infection.1 Additionally, there is a growing volume of literature demonstrating the survival and growth of microorganisms in textiles that could be a vector for health risk. Wool fibres are composed of keratin protein and can absorb water up to 30% of its weight at high humid conditions which are ideal for microbial growth.
Various antibacterial agents, such as triclosan,2 polyhexamethylene biguanide3 and quaternary ammonium compounds,4 have been investigated to provide antibacterial properties to wool but none of them provide a permanent solution. They cannot be bound to untreated wool as the fibre surfaces do not have any appropriate functionality. Instead of synthetic antimicrobial agents, consumers are looking for green antibacterial agents derived from nature. The bio-resourced antibacterial agents have many advantages including they are presumed to be safe, low cost, biodegradable and therefore could be an eco-friendly alternative to synthetic harmful antimicrobial agents. The use of products derived from nature including chitosan,5,6 natural dyes,7 plant extracts such as, tea tree oil, neem oil, and Aloe Vera,8–10 and spice extracts such as, clove oil and garlic oil,11,12 have been investigated for this purpose. Some of the disadvantages of using these natural compounds are their antibacterial strength is not standardised and not available in enough quantity to meet the industry demand. Moreover, some of them are smelly and also can change the colour of the substrates.
Zhao and Sun bonded several cationic quaternary ammonium compounds to the carboxyl groups of wool through ionic bond formation at mild alkaline conditions.4,13 However, the antimicrobial treatment of wool fibre with quaternary ammonium based antibacterial agents has limited durability to laundering. These exudates can pollute watercourses as they are relatively toxic.
Chitosan and its derivatives have been investigated as antibacterial agents against a wide range of target microorganism including bacteria, fungi, and yeasts in experiments involving in vitro and in vivo studies in film, solution, and coatings.14–16 It is believed that chitosan's polycationic nature helps it to bind to negatively charged residues of macromolecules at the cell-surface causing the inhibition of growth of bacteria.17,18 The chemical structure of chitosan is similar to cellulose except one of the hydroxyl groups in each unit is substituted with an acetylamine group. Chitosan has been characterised as a bacteriostatic agent rather than a bactericidal agent as it mostly inhibits the growth of bacteria instead of killing them.19 Although chitosan shows some levels of antimicrobial properties, applications are somewhat limited due to its limited water solubility (especially at pH above 4). Water soluble chitosan derivatives can be obtained by the quaternization of the nitrogen atoms of the amino groups, which introduce permanent positive charges in the polymer chains, resulting in a cationic polyelectrolyte characteristic independent of the pH of the aqueous medium. It was found that quaternization not only improved its solubility at various pH but also enhanced its antimicrobial activity.20,21
The investigation of quaternization of chitosan has been mainly limited to the binding of quaternary ammonium compounds to chitosan or simple quaternization of amino groups of chitosan. However, the binding of quaternary ammonium polymer to chitosan, which may increase the number of quaternary ammonium groups to each unit of chitosan substantially, has rarely been considered. Poly[2-(acryloyloxy)ethyl]trimethylammonium chloride is a quaternary ammonium bioactive polymer, but the grafting or coating of this polymer onto the surface of wool fabric makes the surface feel greasy which is undesirable to consumers. Moreover, quaternary ammonium polymers are expensive and to fully coat wool fibres with quaternary ammonium polymers will thus be expensive. Therefore, modification of chitosan by grafting a quaternary ammonium polymer onto it and then coating wool fibre with this modified chitosan would be a viable option. In this work, we reduced the greasiness as well as the cost by grafting poly(acryloyloxy)ethyltrimethylammonium chloride on to the backbone of chitosan, which not only enhanced chitosan's antimicrobial performance but also made the antimicrobial agent partly natural. Polystyrene sulphonate (pSS) was grafted onto the wool fibre surface to introduce anionic groups so that cationic quaternized chitosan could be ionically bonded to them.
2. Experimental
2.1 Materials used
The wool fabric used was 210 g m−2 2/2 twill woven having 34 ends per cm and 24 picks per cm. 80% solution of 2-(acryloyloxy)ethyltrimethylammonium chloride and styrene sulphonate (90% pure) monomers were purchased from Sigma-Aldrich Chemicals (USA). Teric GN9, a non-ionic detergent, and Albegal FFA, an antifoaming agent, were purchased from Huntsman Chemicals USA. Hostapal MRN, a non-ionic wetting agent, was purchased Clariant Chemicals (Switzerland). Acetic acid and potassium peroxydisulphate were also purchased from Sigma-Aldrich Chemicals (USA). ‘Softly’, a wool detergent was supplied by Pental Products Pty Ltd (Australia). All chemicals were used without any further purification except [2-(acryloyloxy)ethyl]trimethylammonium chloride which was purified by column separation to remove added polymerisation inhibitor monomethyl ether hydroquinone. The cultures of Staphylococcus aureus, Klebsiella pneumoniae and Aspergillus fumigatus were procured from Environmental Science Research (ESR) of New Zealand.
2.2 Grafting of pSS onto wool fibre
The grafting of pSS was carried out in an Ahiba laboratory dyeing machine. The fabric was initially treated with 1 g l−1 Teric GN9 at 50 °C for 15 minutes followed by cold rinsing, warm and cold washing to remove processing lubricants used at the spinning stage of wool yarn manufacturing. Typically, the bath was filled with water and dosed with 0.2 g l−1 Sandozin MRN (wetting agent), 1 g l−1 Albegal FFA and 1 ml l−1 acetic acid. The required quantity of pre-dissolved styrene sulphonate monomer was added. The fabric sample was then introduced into the dye pot, the pH adjusted to 4.5 with acetic acid, and sodium acetate solution. The temperature was then raised to 45 °C, and potassium peroxydisulphate (5% on the weight of monomer) was added to the bath. After good mixing, the temperature was raised to 65 °C, held for 60 minutes, after which the temperature of the bath was dropped to 45 °C, the liquor was drained. The treated fabric was washed in cold water, then in hot water and rinsed again in cold water. This modified wool fabric is denoted as pSS-g-wool fabric in the rest of the document.
2.3 Synthesis of poly(acryloyloxy)ethyltrimethylammonium chloride-grafted chitosan
4 g chitosan was dissolved in 100 ml 1% solution of acetic acid. The solution was filtered with a coarse filter to remove any insoluble admixtures and was then placed in a 500 ml size three neck conical flask. The solution was purged with nitrogen for 30 minutes and the flask was placed in a glycerine bath. 25.8 ml of 0.5 M [2-(acryloyloxy)ethyl]trimethylammonium chloride solution was added to it drop-wise with vigorous stirring and continuous purging with nitrogen. When the addition was completed, the reaction was kept under agitation at room temperature for six hours. After good mixing, the temperature was raised to 45 °C, and 3.7 ml of 0.1 M potassium peroxydisulphate was added with stirring under nitrogen atmosphere. The temperature was then raised to 70 °C, held for 2 hours, after which it was cooled down to room temperature. This cationic quaternized chitosan is denoted as Q-chitosan.
2.4 Coating of wool fabric with Q-chitosan
The control fabric and the pSS-g-wool fabric were coated with Q-chitosan by a laboratory padding mangle. The fabric samples were soaked in a 20 g l−1 Q-chitosan solution for 10 minutes and then passed once through the laboratory padding mangle at 0.4 psi pressure and at 2 m min−1 speed. The coated fabric was then dried in a curing oven at 80 °C for 5 minutes and cured at 130 °C for 2 minutes. Q-chitosan binds to the surface of pSS-g-wool fabric through ionic bond formation as shown in Scheme 1.
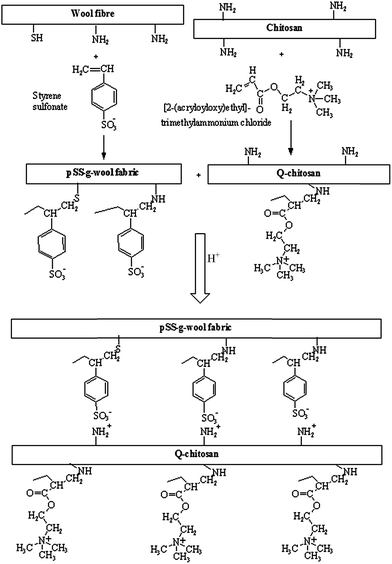 |
| Scheme 1 Mechanism of ionic bond formation between the sulphonate groups of pSS-g-wool fabric and the amino groups of the Q-chitosan in an acidic aqueous solution. | |
2.5 Assessment of antibacterial and antifungal properties
The antibacterial properties of wool fabrics treated with poly[2-(acryloyloxy)ethyl]trimethylammonium chloride grafted-chitosan were assessed by qualitatively according to the AATCC Test Method 147-2011: Antibacterial Activity Assessment of Textile Materials: Parallel Streak Method. The nutrient broth was prepared with 5 g l−1 peptone and 3 g l−1 beef extracts and the pH was adjusted to 6.8 ± 0.1 with 1 N sodium hydroxide solution. The bacterial culture, 100 times diluted, was transferred to the nutrient by using a 4 mm inoculating loop and was incubated at 37 ± 2 °C for 24 hours. The bacteria used were Staphylococcus aureus (ATCC 6538), and Klebsiella pneumoniae (ATCC 4352). 1 ml of 24 hour broth culture of bacteria was mixed with 9 ml of sterile distilled water in a Petri dish and one loopful of diluted inoculum was transferred to the surface of the sterile agar plate by making 5 streaks, approximately 60 mm in length and spaced 10 mm apart covering the central area of a standard Petri dish. Samples of fabric (25 × 50 mm) were gently pressed transversely across the inoculum streak to ensure intimate contact with the agar plate and incubated at 37 ± 2 °C for 24 hours, after which the interruption of bacterial growth along the streaks of inoculum under the specimen and zone of inhibition beyond its edge were examined. Photographs of growth of bacteria were taken by a Sony Cybershot DSCW40 digital camera and the zone inhibition was measured by a digital calipers. At least three samples were tested and the average size of zone inhibition is reported here.
Antifungal properties of untreated and Q-chitosan-coated pSS-g-wool fabric were assessed according to the AATCC Test Method 30-1999 (Antifungal Activity: Assessment on Textile Materials: Mildew and Rot Resistance of Textile Materials). For this method 3.8 ± 0.5 cm diameter disk-shaped samples were cut from the treated and control fabrics. The fungus used was Aspergillus fumigatus (ATCC 1022). The culture medium was comprised of 3 g l−1 ammonium nitrate, 2.5 g l−1 potassium dihydrogen phosphate, 2 g l−1 dipotassium hydrogen phosphate, 0.2 g l−1 magnesium sulphate, 0.1 g l−1 ferrous sulphate and 20 g l−1 agar. Aspergillus fumigatus was grown for 7–14 days in that culture medium. 50 ml sterile water was placed in a sterile Erlenmeyer flask and 3% glucose and scrapings of ripen culture of Aspergillus fumigatus was added to it. The flask was shaken to produce a suspension of spores and 1 ml of inoculum was then evenly spread over the surface of the agar. The fabric sample to be tested was wetted in a solution containing non-ionic wetting agent and placed on the agar surface. Then 0.2 ml inoculum was distributed over the each sample by means of a sterile pipette, incubated at 28 ± 1 °C for 14 days, and the growth of fungus was observed.
2.6 Durability to washing
The durability of the treatment is quite important; otherwise it will be useless as by washing the treatment will be lost. The durability of the treatment to washing was tested up to five cycles in a Wascator at 40 °C according to the International Wool Secretariat (IWS) 7A washing protocol. The samples were loaded in the Wascator with cotton fabric ‘makeweights’ weighing a total of 1 kg. The required quantity of ‘Softly’ detergent (40 g) was added per kilo load of fabric as suggested by the detergent manufacturer.
2.7 Chemical characterisation of Q-chitosan film
A predetermined quantity of chitosan (1.0 g) was dissolved in 1% acetic acid solution and poured on a Petri dish. The water was evaporated leaving behind film of chitosan. In the same way, Q-chitosan film was prepared. Both chitosan and Q-chitosan films were then characterised by Fourier transform infra-red spectrometry by directly scanning the film using a Perkin Elmer Spectrum 2000 Fourier transform infra-red (FTIR) spectrometer at 4 cm−1 resolution. For each sample 64 scans were carried out and the average is reported here. 1H NMR spectra of Q-chitosan was recorded at 299.1 K on a Varian VNMRS 400 nuclear magnetic resonance spectroscope (Agilent Technologies, Inc., Santa Clara, USA) operating at 400 MHz using a D2O solvent.
2.8 Characterisation of Q-chitosan-coated wool fabrics
The tensile strength and elongation properties of the treated wool fabrics in the direction of warp were assessed by using an Instron Tensile Strength Tester (Model 4204) at 20 °C and 65% relative humidity according to the ASTM Test Method D5035-06: Standard Test Method for Breaking Force and Elongation of Textile Fabrics (Strip Method) using a 100N load cell. The sample size was 25.4 × 152.4 mm, and the gauge length and the traversing speed were 100 mm and 50 mm min−1 respectively. At least 10 samples were tested and the average is reported here. The samples were conditioned at the above-mentioned temperature and humidity for 3 days. A Perkin Elmer Spectrum 2000 FTIR spectroscope with ATR attachment using zinc-selenium crystal was used for infrared studies to characterise the surfaces of the untreated and polystyrene-g-wool fabrics as well as polystyrene-g-wool fabric coated with Q-chitosan. For each sample 32 scans were carried out and the average is reported here. Fourier Transform (FT) Raman spectra were recorded using an Equinox 55 interferometer bench (Bruker Optics, Ettlingen, Germany) equipped with a FRA-106 Raman accessory and a D418-T liquid nitrogen cooled Ge detector. A 1064 nm Nd:YAG laser was used to generate Raman scattering. Samples were analysed using the vertical objective in a 180° backscattering arrangement. Laser power was 160 mW; 500 scans were performed for each spectrum; the laser spot size used was 1 mm in diameter; and the resolution of the spectra is 4 cm−1.
The contact angle was measured in dynamic mode by using a KSV CAM 100 Contact Angle Measurement Apparatus. For each sample, contact angle was measured at 10 places and the average contact angle was reported. For each sample, the first measurement was taken immediately after placing the water droplet and then at 15 s intervals until 45 s had passed.
The control wool fabric, control wool fabric coated with Q-chitosan and pSS-g-wool fabric coated with Q-chitosan before and after washing were coated with a thin layer of gold in a vacuum sputtering equipment to make the surface electrically conductive and then were scanned by a JEOL Model 6100 scanning electron microscope.
3. Results
3.1 Characterisation of chitosan and Q-chitosan film
The chitosan film produced by evaporating its solution in 1% acetic acid was transparent and also insoluble in water. The Q-chitosan film produced in the same way also produced transparent and water insoluble film.
Fig. 1(a) shows the FTIR spectra of untreated chitosan and Q-chitosan. The FTIR spectra of unmodified chitosan film showed the emergence of a broad –OH peak between 2600 and 3600 cm−1. The peak at 1653 and 1550 cm−1 could be attributed to the carbonyl stretching vibration of secondary amide (amide I band) groups in chitosan film.22 On the other hand, Q-chitosan shows bands similar to the bands shown by the unmodified chitosan but shows a sharp band at 1735 cm−1, which could be attributed to the stretching vibrations of saturated aliphatic ester (–C
O) coming from the grafted poly(acryloyloxy)ethyltrimethylammonium chloride. In the case of Q-chitosan, there is a small band visible at 2872 cm−1, which could be attributed to the NH3+Cl− groups, coming from the quaternary ammonium groups of the grafted polymer.23
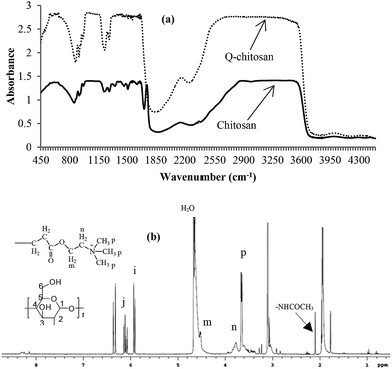 |
| Fig. 1 FTIR (a) and 1H NMR (b) spectra of chitosan and Q-chitosan films (NMR spectrum is shown only for Q-chitosan). | |
Fig. 1(b) shows the 1H NMR spectrum of Q-chitosan. The assignment of chitosan and poly(acryloyloxy)ethyltrimethylammonium chloride peaks have already been reported in the published literature.24–26 The signal of the protons of –OCH2– in the quaternary ammonium polymer unit is visible in the region of 3.83–4.2 ppm (peak i). The peak observed at 3.4–3.6 ppm could be assigned to the protons of the –N+(CH3)3 groups of the quaternary ammonium polymer. The peaks observed at 3.43–3.81, 3.1 and 1.96 ppm could be for H2, H3, H4, H5, H6, and –NHCOCH3– of chitosan respectively.27 The sharp peak observed at 4.46 ppm is for water. The NMR spectrum indicates that poly(acryloyloxy)ethyltrimethylammonium chloride was successfully bonded to chitosan.
3.2 Antibacterial properties
Antibacterial properties of control wool fabric, control wool fabric coated with Q-chitosan and pSS-g-wool fabric coated with Q-chitosan are shown in Table 1. Control wool fabric did not show any bacterial resistance as bacterial growth was seen directly under and up to the edge of the control fabric samples for both types of bacteria as shown in Fig. 2. On the other hand, the control wool fabric coated with Q-chitosan showed an excellent antibacterial performance and the size of inhibition zone was 5 and 3 mm for Staphylococcus aureus and Klebsiella pneumoniae respectively. Similar results were also achieved for the pSS-g-wool fabric coated with Q-chitosan but in the case of Klebsiella pneumoniae the size of the zone inhibition slightly reduced. No bacterial growth was observed under the sample for both the pSS-g-wool fabric and the control wool fabric coated with Q-chitosan. The results show that the Q-chitosan is quite effective against both Gram-positive and Gram-negative bacteria.
Table 1 Antibacterial performance and mechanical properties of control and various treated wool fabrics before and after washing
Sample ID. |
Antibacterial properties |
Staphylococcus aureus |
Klebsiella pneumonia |
Control wool fabric |
No zone of inhibition. There was strong growth directly under the fabric strip |
No zone of inhibition was seen. There was continuous strong growth directly under the fabric strip |
Control wool fabric coated with Q-chitosan (before washing) |
Zone of inhibition = 5 mm. There was no growth directly under the fabric strip |
Zone of inhibition = 3 mm. There was no growth directly under the fabric strip |
Control wool fabric coated with Q-chitosan (after washing) |
Zone of inhibition = 1 mm. There was small growth directly under the fabric strip |
Zone of inhibition = 1 mm. There was small growth directly under the fabric strip |
pSS-g-wool fabric coated with Q-chitosan (before washing) |
Zone of inhibition = 5 mm. There was no growth directly under the fabric strip |
Zone of inhibition = 2 mm. There was no growth directly under the fabric strip |
pSS-g-wool fabric coated with Q-chitosan (after washing) |
Zone of inhibition = 5 mm. There was no growth directly under the fabric strip |
Zone of inhibition = 10 mm. There was no growth directly under the fabric strip |
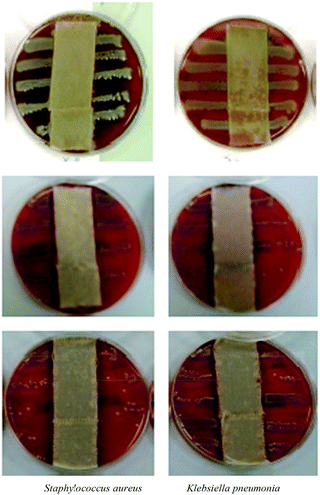 |
| Fig. 2 Images of bacterial growth in the presence of control fabric, control fabric coated with Q-chitosan and the pSS-g-wool fabric coated with Q-chitosan. Top: control fabric; middle: control fabric treated with Q-chitosan; bottom: pSS-g-wool fabric treated with Q-chitosan. | |
3.3 Antifungal properties
The representative images of antifungal activity of control wool fabric and pSS-g-wool fabric coated with Q-chitosan are shown in Fig. 3. It is evident that control wool fabric doesn't show any antifungal activity against Aspergillus fumigatus as an extensive growth of fungus is visible near to the edge of the fabric and also on the fabric. The pSS-g-wool fabric coated with Q-chitosan also shows very little antifungal activity against the same fungus. However, in the case of Q-chitosan-coated pSS-grafted wool fabric, slightly less fungus growth is observed compared to the growth observed in the case of the control untreated fabric. It is evident that Q-chitosan is quite ineffective against this fungus.
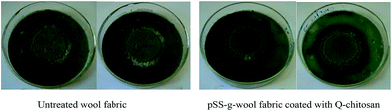 |
| Fig. 3 Antifungal performance of untreated wool fabric and pSS-g-wool fabric coated with Q-chitosan against Aspergillus fumigatus after 7 days incubation. | |
3.4 Durability to washing
The antibacterial performance as well as the surface of both the untreated wool fabric coated with Q-chitosan and the pSS-g-wool fabrics coated with Q-chitosan was examined after five washings and the results obtained are shown Fig. 4. It is evident that in the case of the control wool fabric coated with Q-chitosan, no bacterial growth was observed for both the Staphylococcus aureus and Klebsiella pneumoniae bacteria. However, both types of bacterial growth were visible directly under the tested sample that was washed for five times. It indicates that most of the Q-chitosan applied to the untreated wool fabric has been lost during laundering and therefore the tested sample lost effectiveness against both types of bacteria to some levels.
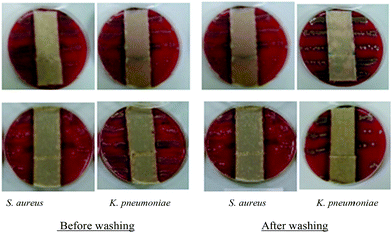 |
| Fig. 4 Images of bacterial growth in the presence of untreated control fabric coated with Q-chitosan and the pSS-g-wool fabric coated with Q-chitosan before and after 5 cycles of washing. Top: control fabric treated with Q-chitosan; bottom: pSS-g-wool fabric treated with Q-chitosan. | |
The size of zone of inhibition also went down to 1 mm for both the Staphylococcus aureus and the Klebsiella pneumoniae bacteria for the washed samples from 5 and 3 mm respectively shown by the same treated unwashed sample (Table 1). On the other hand, no bacterial growth was seen directly under the both unwashed and washed samples of pSS-g-wool fabric coated with Q-chitosan. In the case of Staphylococcus aureus, the size of zone inhibition was same before and after 5 cycles of washing but for Klebsiella pneumoniae the zone of inhibition was extended to double (10 mm) after washing. The reason of extended zone inhibition observed for the washed samples could be that some of the low molecular weight component of the Q-chitosan loosened but adhered to the surface of fabric which leached to bacterial solution during the 48 hours of antibacterial test period. The results indicate that in the case of case of pSS-g-wool fabric coated with Q-chitosan, the coatings were mostly intact even after washing. The achieved results show that the grafting with pSS highly improved the durability of Q-chitosan treatment to multiple washings.
3.5 Mechanical properties
Specimen stress–strain curves of control wool fabric, pSS-g-wool fabric, and Q-chitosan-coated pSS-g-wool fabric are shown in Fig. 5. Stress–strain curves of the untreated and treated fabrics showed similar pattern and the deformation of fabric samples started when the highest stress was applied. The tensile strength and elongation at break of the control wool fabric, the wool fabric coated with Q-chitosan and the pSS-g-wool fabric coated with Q-chitosan are presented in Table 2. It can be seen that the grafting with pSS improved the tensile strength of wool fabric samples as well as elongation but the subsequent coating with Q-chitosan improved the tensile strength but decreased the elongation. The tensile strength of the untreated control fabric was 13.9 ± 0.08 MPa, which increased to 15.9 ± 0.15 MPa after grafting with pSS. The tensile strength of the pSS-g-wool fabric further increased to 17.7 ± 0.12 MPa after the coating with Q-chitosan. On the other hand, the control fabric showed elongation of ∼49.4%, which increased to ∼52.9% for the pSS-g-wool fabric. However, in the case of the pSS-g-wool fabric coated with the Q-chitosan, the elongation slightly decreased to ∼50.0%. The results obtained suggests that Q-chitosan coating acts as a reinforcing scaffold and contributed in increasing tensile strength but decreased elongation as the elongation of the wool fibre was affected probably by the poor elongation of the Q-chitosan coating.
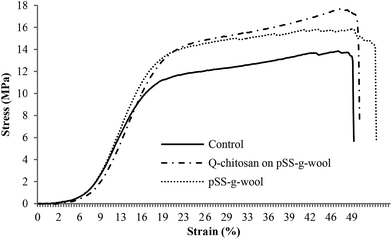 |
| Fig. 5 Specimen stress–strain curves of control, pSS-g-wool and pSS-g-wool fabric coated with Q-chitosan. | |
Table 2 Mechanical properties of control and various treated wool fabrics before washing
Sample ID. |
Mechanical properties |
Tensile strength (MPa) |
Elongation (%) |
Control wool fabric |
13.9 ± 0.08 |
49.4 ± 0.2 |
pSS-g-wool fabric (before washing) |
15.9 ± 0.15 |
52.9 ± 0.5 |
pSS-g-wool fabric coated with Q-chitosan (before washing) |
17.7 ± 0.12 |
50.0 ± 0.6 |
3.6 ATR-FTIR analysis
The FTIR spectra of untreated, pSS-g-wool fabric and pSS-g-wool fabric coated with Q-chitosan are shown in Fig. 6(a). The spectra of untreated wool shows the absorbency peak at 1620–1630 cm−1 which can be assigned to the elastic vibration peak of C
O bond, and the peak at 1510–1520 cm−1 is labelled as the bending deformation peak of C–N–H bond.28 The spectrum of pSS-g-wool fabric shows extra peaks at 1005 and 1040 cm−1, which could be attributed to the sulphonate groups of the grafted polystyrene sulphonate. In the case of the spectrum of pSS-g-wool fabric coated with Q-chitosan, new peaks are shown corresponding to the poly[2-(acryloyloxy)ethyl]trimethylammonium chloride moieties, which were at 1481 cm−1 (bending vibration of methyl groups of ammonium), 948 cm−1 (stretching vibration of quaternary ammonium), and 1736 cm−1 (stretching vibration of saturated ester linkage, –C
O). Confirmation of the binding of Q-chitosan onto pSS-g-wool fabric surface was determined by monitoring the peaks in the spectra at 2923 cm−1 indicating the presence of aliphatic hydrocarbons and at 2854 cm−1 indicating the presence of NH3+Cl−.23 The FTIR results suggest that Q-chitosan was successfully bonded to pSS-g-wool fabric surface.
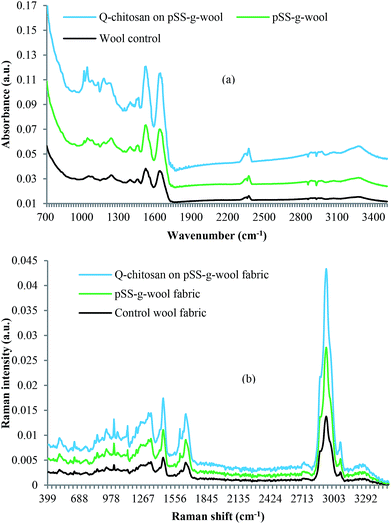 |
| Fig. 6 ATR-FTIR (a) and FT-Raman (b) spectra of control wool fabric, pSS-g-wool fabric and pSS-g-wool fabric coated with Q-chitosan. | |
3.7 Raman analysis
The surface of the various coated wool fabrics were further characterised by the Raman analysis and the band positions observed for the different wool fabric samples are shown in Fig. 6(b). The spectrum of the untreated wool shows a spectrum which is typical of the Raman spectrum corresponding to wool. The positions of the amide I band at 1655 cm−1, and C–C stretching vibrations of the skeletal backbone at 934 cm−1 are indicating the high content of α-helix in native wool.29,30 Notable features include cysteine (Cys) S–S stretch at 508–514 cm−1, the sharp phenylalanine stretch at 1002 cm−1, the amide I and amide II bands at 1655 and 3060 cm−1. The spectrum of the pSS-g-wool fabric shows quite distinct differences to the control sample. Distinct bands are observed at 1630 and 1600 cm−1 along with sharp features at 1205 and 1125 cm−1. Some of these features can be attributed to the wool sample, however the narrowness of the bands suggests they may be crystallised samples from the treatment, in this case an ammonium salt. The bands at 620 cm−1 could be attributed to the mono-substituted benzene ring of the grafted polystyrene sulphonate.
The control and the pSS-g-wool fabrics both show bands at 1003 and 1126 cm−1 (in the case of control wool the band is at 1125 cm−1), which are related to sulphonate groups. It is evident that the intensity of the peak in the case of the pSS-g-wool fabric was much higher compared to the intensity observed for the control wool. Some of the sulphur linkages of wool could be broken during processing producing, sulphonate groups, but the reason for high intensity showed by the pSS-g-wool is likely to be because of the sulphonate groups of the polystyrene sulphonate.
The spectrum of the treated samples shows quite distinct differences. Distinct bands are observed at 1630 and 1600 cm−1 along with sharp features at 1205 and 1125 cm−1. The spectrum of the Q-chitosan coated pSS-g-wool fabric shows two distinct bands at 827 and 1034 cm−1 those could be attributed to the (CH3)3N+ symmetrical stretching vibration and C–O–H stretching vibration of the Q-chitosan. The spectrum of the Q-chitosan coated pSS-g-wool fabric also shows a distinctive band at 1630 cm−1, which is absent in the spectra of control and pSS-g-wool fabric, and is related to the C
C stretching of the vinyl group of the poly[2-(acryloyloxy)ethyl]trimethylammonium chloride.31 The FTIR and FT-Raman analysis results obtained indicate that poly[2-(acryloyloxy)ethyl]trimethylammonium chloride successfully grafted onto chitosan.
3.8 Contact angle
Contact angle is a quantitative measure of the wetting of a solid surface by a liquid. It is defined as the angle formed by a liquid at the three-phase boundary where a liquid, gas and solid intersect. This is the measure of wettability as well as hydrophobicity of a surface. Wool fabrics are naturally hydrophobic because of the formation of polyethylene-like layer on the surface of wool by 18-methyl eicosanoic acid through a thioester bonding. The optical images of water droplets on the surfaces of the 5 × 7A washed samples of untreated wool, untreated wool coated with Q-chitosan and the pSS-g-wool fabric coated with Q-chitosan at 15 s intervals are shown in Table 3. In the case of untreated wool fabric, the average contact angle at 0 s was ∼124°. The water droplet was not absorbed by the fabric even after 45 s and the average contact angle after 45 s was still approximately 119° (Table 2).
Table 3 Dynamic contact angle of surface of control wool fabric, control wool fabric coated with Q-chitosan and pSS-g-wool fabric coated with Q-chitosan after washing
Treatments |
Contact angle (°) after certain time |
0 s |
15 s |
30 s |
45 s |
Left |
Right |
Left |
Right |
Left |
Right |
Left |
Right |
Control wool fabric |
 |
 |
 |
 |
Control wool fabric coated with Q-chitosan |
 |
 |
 |
 |
pSS-g-wool fabric coated with Q-chitosan |
 |
 |
 |
 |
In the case of the washed sample of the control fabric coated with Q-chitosan, the average contact angle at 0 s was 122° but even after 45 s the water droplet was not fully absorbed by the fabric. The contact angle diminished to ∼53.0° at 45 s from ∼122°. On the other hand, in the case of the pSS-g-wool fabric coated with Q-chitosan, the contact angle at 0 s was much lower (∼110°) compared to the contact angle shown by the control fabric and the control fabric coated with Q-chitosan. For the pSS-g-wool fabric coated with Q-chitosan, the water droplet was also fully absorbed into the fabric within 45 s and the contact angle diminished to 0° from ∼110° at 45 s. The results indicate that even after 5 washing cycles, a small quantity of Q-chitosan remained in the untreated fabric coated with Q-chitosan and therefore the contact angle reduced to ∼53.0° but the pSS-g-wool fabric showed excellent durability to washing. Grafting of wool fabrics with pSS shows a positive effect in improving the durability of the Q-chitosan treatment to wool.
3.9 Surface morphologies
The SEM images of control wool fabric, control wool fabric coated with Q-chitosan and pSS-g-wool fabric coated with Q-chitosan before and after washing are shown in Fig. 7. The control wool fabric shows the usual scaly structure and also debris of some damaged scales. The SEM images reveal that Q-chitosan was more evenly spread on the pSS-g-wool surface compared to the surface of untreated wool fibre, which suggests that the pSS-g-wool fabric surface was more compatible to Q-chitosan compared to the hydrophobic untreated wool fibre surface. The surface of wool fibre was mostly covered with Q-chitosan in a manner that scales were hardly visible. It can be seen that Q-chitosan coating on untreated wool fabric showed poor durability to laundering and most of the Q-chitosan coating was removed after 5 cycles of washing. SEM image reveals that the surface of wool fabric after washing looks quite similar to the surface of control wool fabric as cuticles are clearly visible. As a result it showed poor antibacterial performance. On the other hand, Q-chitosan coating was quite intact in the case of pSS-g-wool and the scaly surface of wool fibre was still covered. As a result, the pSS-g-wool fabric coated with Q-chitosan after washing still showed strong antibacterial performance after 5 cycles of washing. The results were consistent with the contact angle data as the contact angle only slightly reduced in the case of control fabric coated with Q-chitosan, but in the case pSS-g-wool fabric coated with Q-chitosan, the contact angle become zero after 30 s. The contact angle in the case of control wool fabric remained almost same even after 45 s and showed strong hydrophobicity. We found that the dried film of Q-chitosan was insoluble in water. In the case of untreated fabric, there was no bonding between the wool fibre and Q-chitosan. However, in the case of pSS-g-wool fabric there was strong ionic bonding between the fibre and Q-chitosan, which increased the durability of the treatment to laundering.
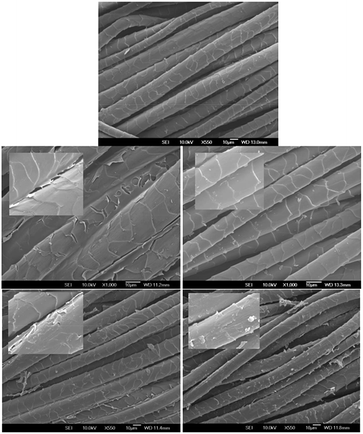 |
| Fig. 7 SEM micrographs of control fabric (top), control fabric coated with Q-chitosan (middle) and pSS-g-wool fabric coated with Q-chitosan (bottom) before (left) and after (right) 5 times washing according to the ISO 7A washing protocol. | |
4. Conclusions
We demonstrated that durability of the antibacterial treatment based-on Q-chitosan to wool could be considerably increased by grafting wool fabric with pSS. Q-chitosan treated wool fabrics showed excellent antibacterial activity against Staphylococcus aureus and Klebsiella pneumoniae but marginal activity against Aspergillus fumigatus fungus. The grafting of wool fabric with pSS not only improved tensile strength of the fabric but also improved the durability of the treatment to multiple washings. Contact angle and SEM analysis results show that even without grafting with pSS, a small amount of chitosan remained on the fabric surface after 5 cycles of washing, which conferred some levels of antibacterial activity. In the case of the pSS-g-wool fabric, Q-chitosan uniformly coated the treated fibre surface and after 5 cycles of washing the coating was still intact. Therefore, the combined treatment presented here is a prospect commercially viable antibacterial treatment for wool fabrics.
Acknowledgements
The author acknowledges the financial support received from the Ministry of Business Innovation and Employment (MBIE) of the New Zealand Government thorough Grant no. C10X0824. I also acknowledge help received from Professor Keith Gordon of the Department of Chemistry of Otago University (Dunedin, New Zealand) for the FT-Raman analysis of untreated and treated wool fabric samples. I would like to thank Stewart Collie for his help in improving the quality of the article. The author would like to thank Barbara Müller (RJ Hill Laboratories Ltd.) and Malik Altaf Hossain (Lincoln University) for the assessment of antibacterial and antifungal performance respectively.
References
- M. Tischer, G. Pradel, K. Ohlsen and U. Holzgrabe, ChemMedChem, 2012, 7, 22 CrossRef CAS PubMed.
- Y. Gao and R. J. Cranston, Text. Res. J., 2008, 78, 60 CrossRef CAS PubMed.
- Y. Gao and R. J. Cranston, Appl. Polym. Sci., 2010, 117, 3075 CAS.
- T. Zhao and G. Sun, J. Appl. Microbiol., 2008, 104, 824 CrossRef CAS PubMed.
- O. Wiarachai, N. Thongchul, S. Kiatkamjornwong and V. P. Hoven, Colloids Surf., B, 2012, 92, 121 CrossRef CAS PubMed.
- F. S. Ghaheh, S. M. Mortazavi, F. Alihosseini, A. Fassihi, A. S. Nateri and D. Abedi, J. Cleaner Prod., 2014, 72, 139 CrossRef PubMed.
- N. A. Ibrahim, W. A. Abdalla, E. M. El-Zairy and H. M. Khalil, Carbohydr. Polym., 2013, 92, 1520 CrossRef CAS PubMed.
- M. Parthiban, M. R. Srikrishnan and S. Viju, Int. J. Pharm. Life Sci., 2011, 2, 1137 CAS.
- M. Joshi, S. W. Ali and R. Purwar, Indian J. Fibre Text. Res., 2009, 34, 295 CAS.
- I. Holme, Color. Technol., 2007, 123, 59 CAS.
- A. Javid, Z. A. Raza, T. Hussain and A. Rehman, J. Microencapsulation, 2014, 31, 461 CrossRef CAS PubMed.
- S. Durairaj, S. Srinivasan and P. Lakshmanaperumalsamy, Electronic Journal of Biology, 2009, 5, 5 Search PubMed.
- T. Zhao and G. Sun, J. Appl. Polym. Sci., 2006, 103, 482 CrossRef PubMed.
- X. Fu, Y. Shen, X. Jiang, D. Huang and Y. Yan, Carbohydr. Polym., 2011, 85, 221 CrossRef CAS PubMed.
- A. A. Tayel, S. Moussa, W. L. El-Tras, D. Knittel, K. Opwis and E. Schollmeyer, Int. J. Biol. Macromol., 2010, 47, 454 CrossRef CAS PubMed.
- Y. Park, M. H. Kim, S. C. Park, H. Cheong, M. K. Jang, J. W. Nah and K. S. Hahm, J. Microbiol. Biotechnol., 2008, 18, 1729 CAS.
- Y. J. Jeon, P. J. Park and S. K. Kim, Carbohydr. Polym., 2001, 44, 71–76 CrossRef CAS.
- Y. J. Jeon and S. K. Kim, Carbohydr. Polym., 2000, 41, 133–141 CrossRef CAS.
- V. Coma, A. Martial-Gros, S. Garreau, A. Copinet, F. Salin and A. Deschamps, J. Food Sci., 2002, 67, 1162 CrossRef CAS PubMed.
- T. Xu, M. Xin, H. Huang, S. Zhou and J. Liu, Carbohydr. Res., 2006, 346, 2445–2450 CrossRef PubMed.
- Z. Jia, D. Shen and W. Xu, Carbohydr. Res., 2001, 333, 1 CrossRef CAS.
- J. Xu, S. P. McCarthy, R. A. Gross and D. L. Kaplan, Macromolecules, 1996, 29, 3436 CrossRef CAS.
- M. M. Hassan, Ind. Eng. Chem. Res., 2014, 53, 10954 CrossRef CAS.
- G.-X. Sun, M.-Z. Zhang, J.-L. He and P.-H. Ni, J. Polym. Sci., Part A: Polym. Chem., 2009, 47, 4670 CrossRef CAS PubMed.
- M. Lavertu, Z. Xia, A. N. Serreqi, M. Berrada, A. Rodrigues, D. Wang, M. D. Buschmann and A. Gupta, J. Pharm. Biomed. Anal., 2003, 32, 1149 CrossRef CAS.
- J. Holappa, T. Nevalainen, P. Soininen, M. Máson and T. Järvinen, Biomacromolecules, 2006, 7, 407 CrossRef CAS PubMed.
- Y. Ding, G. Gu, X.-H. Xia and Q. Huo, J. Mater. Chem., 2009, 19, 795 CAS.
- R. H. Bradley, I. Mathieson and K. M. Byrne, J. Mater. Chem., 1997, 7, 2477 RSC.
- T. Shalev, A. Gopin, M. Bauer, R. W. Stark and S. Rahimipour, J. Mater. Chem., 2012, 22, 2026 RSC.
- J. S. Church, G. L. Corino and A. L. Woodhead, J. Mol. Struct., 1998, 440, 15 CrossRef CAS.
- K. Tashiro, K. Matsushima and M. Kobayashi, J. Phys. Chem., 1990, 94, 3197 CrossRef CAS.
|
This journal is © The Royal Society of Chemistry 2015 |