DOI:
10.1039/C5RA02825F
(Paper)
RSC Adv., 2015,
5, 26517-26520
Catalytic activity of reusable nickel oxide nanoparticles in the synthesis of spirooxindoles
Received
13th February 2015
, Accepted 10th March 2015
First published on 10th March 2015
Abstract
This study focuses on nickel oxide nanoparticles as efficient nanocatalysts in the synthesis of oxindoles as an important class of potentially bioactive compounds. The oxindole derivatives were prepared by the condensation of malononitrile, 1,3-dicarbonyl/4-hydroxycoumarine and isatin compounds in water, an excellent solvent in terms of environmental impact and reduction waste production.
Introduction
Recently, great attention has also been focused from both environmental and economical points on the use of heterogeneous solid acid catalysts for various organic transformations.1,2 Heterogeneous catalysts are always superior to their homogeneous counterparts in terms of many aspects such as operational simplicity, reusability, environmental compatibility and high selectivity. With the advancement of nanoscience and nanotechnology, nanoparticulate heterogeneous catalysts have received notable attention in organic transformations on the grounds of their ability to enhance faster rates of organic reactions, their high catalytic activity and higher yield of products which is due to their ability to afford a high particle size-to-volume ratio along with greater surface area.3 During recent years, nickel oxide nanoparticles have attracted much attention as inexpensive and nonhazardous catalysts or effective promoters that can enhance the reactivity and selectivity of various organic reactions.4,5
On a different note, indoline and oxindole which are important fragments of a large number of natural products and medicinal agents have shown good biological activity.6–11 Oxindoles are known to possess antibacterial, antiprotozoal and anti-inflammatory activities and are also patented as PR (progesterone receptors) agonists.12,13 Among the oxygen-containing heterocycles fused with spirooxindole ring system, chromenes are of particular utility as they belong to ‘privileged medicinal scaffolds’—certain molecular frameworks serving for the generation of ligands for functionally and structurally discreet biological receptors.14 Functionally substituted 4H-chromenes have received considerable attention due to their wide range of useful biological properties, which include spasmolitic, diuretic, anticoagulant, anticancer, and antianaphylactic activities.15
In many cases, organic solvents which are used in huge amounts for many different applications have a negative impact on the health and the environment. One of the key areas of green chemistry is the elimination of solvents in chemical processes or the replacement of hazardous solvents with environmentally benign solvents. Water emerged as a useful alternative solvent for several organic reactions owing to many of its potential advantages such as safety, economy and environmental concern. Sometimes it shows higher reactivity and selectivity compared to other conventional organic solvents due to its strong hydrogen bonding ability.16,17
Due to the pharmacological properties of oxindoles, development of synthetic methods enabling easy access to these compounds are desirable. In continuation of our work on the synthesis of biologically important compounds using simple, efficient and nontoxic catalysts,18–22 in this paper we report synthesis of spirocyclic (5,6,7,8-tetrahydro-4H-chromene)-4,3′-oxindole and dihydro[pyrano[3,2-c]quinoline]-4,3-indoline derivatives by the coupling of malononitrile, 1,3-dicarbonyl/4-hydroxycoumarine and isatin derivatives in the presence of nano-NiO as an inexpensive and efficient catalyst in aqueous medium (Scheme 1).
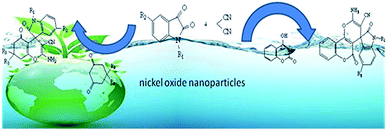 |
| Scheme 1 | |
Results and discussion
Characterization of nickel oxide nanoparticles
Initially, nickel oxide nanoparticles were synthesized by the reaction of nickel nitrate hexahydrate with urea in deionized water and was heated at 115 °C for 1.5 h in an oil bath. Resulting compound was dried and was calcined in at 400 °C for 1 h. Then Nano NiO were characterized by XRD, EDS and TEM.18
Application of NiO nanoparticles for the synthesis of spirooxindoles
In order to show the merit of synthesized heterogeneous catalyst in organic reactions, nano NiO was used as an efficient and inexpensive catalyst for synthesis of a series of analogues of oxindole using various isatins and dicarbonyls. 1,3-Cyclohexadione (1), isatin (2) and malononitrile (3) were selected as the model substrates and reacted under different experimental variants (Scheme 2).
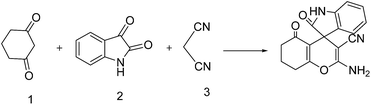 |
| Scheme 2 Synthesis of 2-amino-2′,5-dioxo-5,6,7,8-tetrahydro spiro-[chromene-4,3′-indoline]-3-carbonitrile. | |
Optimization of the reaction conditions
In preliminary experiment, this reaction was carried out in various solvents, with nano-NiO (0.0007 g) as a catalyst under room temperature.
The reaction proceeded perfectly in polar solvents (Table 1, entries 1–6), but the yields decreased when the reaction was carried out in nonpolar solvents (Table 1, entries 7–11). The reaction could be carried out under solvent-free conditions and gave good yield (Table 1, entry 12).
Table 1 Effect of solvents on the synthesis of oxindole catalyzed by nano-NiOa
Entry |
Solvent |
Yieldb (%) |
Reaction conditions: reagents (1 mmol), catalyst (0.0007 g), solvent (2 mL) for 5 min. Isolated yields. |
1 |
H2O |
98 |
2 |
EtOH |
85 |
3 |
MeOH |
88 |
4 |
EtOAc |
85 |
5 |
DMSO |
93 |
6 |
DMF |
90 |
7 |
CH3CN |
70 |
8 |
ClCH2CH2Cl |
68 |
9 |
THF |
50 |
10 |
CHCl3 |
62 |
11 |
CH2Cl2 |
55 |
12 |
Solvent-free |
75 |
Comparison of catalytic activities of nano-NiO and bulk metal oxides
To evaluate catalytic activity of nano-NiO, the model reaction was carried out in water (2 mL) at room temperature for 5 min in the presence of different catalytic systems (0.0007 g), separately. The results are shown in Table 2. As it is evident from the results, nano-NiO was the most effective catalyst in terms of yield of the oxindole (98%) while other catalysts formed the product with the yields of 5–38% (Table 2, entries 1–9).
Table 2 Synthesis of oxindole in the presence of various catalytic systemsa
Entry |
Catalyst |
Yieldb (%) |
Reaction conditions: 1,3-cyclohexadione (1 mmol), isatin (1 mmol), malononitrile (1 mmol), catalyst (0.0007 g) and H2O (2 mL) stirred at room temperature for 5 min. Yields are related to isolated pure products. |
1 |
SiO2 |
20 |
2 |
Al2O3(acidic) |
18 |
3 |
Al2O3(basic) |
20 |
4 |
MgO |
5 |
5 |
CaO |
20 |
6 |
CdO |
25 |
7 |
CuO |
15 |
8 |
As2O3 |
33 |
9 |
CrO3 |
38 |
10 |
Nano-NiO |
98 |
Extension of substrate scope
Further explore the scope and limitation of this protocol under the optimized conditions (0.0007 g catalyst in H2O at r.t.), particularly in regard to library construction, was evaluated using various isatin and 1,3-dicarbonyl compounds (Table 3). In all cases, the reaction proceeded readily to afford the corresponding oxindoles in high to excellent yields (70–98%) in very short reaction times (5 min). The reaction of dimedone took place faster than 1,3-cyclohexadione analogues that this behavior could result from the more reactivity of dimedone by electron-donating groups (Me).
Table 3 Preparation of oxindole derivativesa
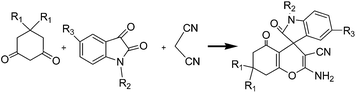
|
Entry |
Reactant |
Yield (%) |
Melting Point |
Dicarbonyl |
Isatin |
R1 |
R2 |
R3 |
Reaction conditions: dicarbonyl compounds (1 mmol), isatins (1 mmol), malononitrile (1 mmol), catalyst (0.0007 g), and H2O (2 mL); reactions conducted at r.t. for 5 min. |
1 |
H |
H |
H |
98 |
251–253 |
2 |
H |
PhCH2 |
H |
89 |
283–284 |
3 |
H |
H |
Cl |
88 |
275–276 |
4 |
H |
H |
Br |
90 |
289–292 |
5 |
H |
H |
NO2 |
92 |
279–280 |
6 |
H |
H |
Me |
70 |
263–265 |
7 |
H |
H |
OMe |
82 |
268–269 |
8 |
H |
PhCH2 |
Br |
71 |
295–296 |
9 |
H |
Et |
NO2 |
82 |
276–277 |
10 |
Me |
Et |
NO2 |
94 |
281–283 |
11 |
Me |
H |
Cl |
91 |
273–274 |
12 |
Me |
H |
H |
98 |
289–290 |
13 |
Me |
H |
OMe |
78 |
282–283 |
Due to the biological and pharmacological importance of the indoline ring system and to further expand the scope of this reaction, we also investigated reaction of 4-hydroxycoumarine instead of diketone were obtained products in excellent yields under the same reaction conditions.
Reusability of the catalyst
At the end of the reaction, the catalyst could be recovered by centrifuge. The recycled catalyst was washed with dichloromethane and subjected to a second reaction process. The results show that the yield of product after five runs was only slightly reduced (Table 5).
Table 4 Preparation of indoline derivativesa
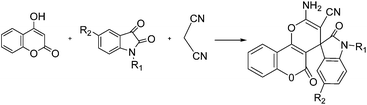
|
Entry |
R1 |
R2 |
Yield (%) |
Reaction conditions: 4-hydroxycoumarine (1.0 mmol), isatins (1.0 mmol), malononitrile (1.0 mmol), catalyst (0.0007 g), and H2O (2 mL); reactions conducted at r.t. |
1 |
H |
H |
96 |
2 |
H |
Cl |
91 |
3 |
H |
Br |
98 |
4 |
H |
NO2 |
95 |
5 |
H |
OMe |
83 |
6 |
H |
Me |
82 |
7 |
Me |
NO2 |
98 |
8 |
Et |
NO2 |
91 |
9 |
PhCH2 |
Br |
75 |
10 |
PhCH2 |
H |
83 |
Table 5 Recyclability of nano-NiO in the synthesis of oxindolesa
Entry |
Cycle |
Yield (%) |
Reaction conditions: reagents (1 mmol), catalyst (0.0007 g), H2O (2 mL) for 5 min. |
1 |
0 |
98 |
2 |
1 |
98 |
3 |
2 |
96 |
4 |
3 |
95 |
5 |
4 |
91 |
6 |
5 |
89 |
Experimental
General methods
Malononitrile, 1,3-dicarbonyl, 4-hydroxycoumarin and isatin derivatives were purchased from Merck Chemical Company. Purity determination of the products were accomplished by TLC on silica-gel polygram SILG/UV 254 plates. Melting points were measured on an Electro thermal 9100 apparatus. IR spectra were taken on a Perkin Elmer 781 spectrometer in KBr pellets and reported in cm−1. 1H NMR and 13C NMR spectra were measured on a Bruker DPX-250 Avance instrument at 250 MHz and 62.9 MHz in CDCl3 or DMSO-d6 with chemical shift given in ppm relative to TMS as internal standard. The morphology of the products was determined by using CMPhilips10 model Transmission Electron Microscopy (TEM) at accelerating voltage of 100 KV. Power X-ray diffraction (XRD) was performed on a Bruker D8-advance X-ray diffractometer with Cu Kα (λ = 0.154 nm) radiation.
Preparation of nano NiO
Nickel oxide nanoparticles were prepared through the following process. The molar ratio of nickel nitrate hexahydrate to urea at 1
:
4, a stoichiometric amount of Ni(NO3)2·6H2O (0.08 mol) and CO(NH2)2 (0.32 mol) was accurately weighed and dissolved into 60 mL of deionized water, respectively. The two solutions were mixed in a beaker and stirred with a magnetic stirrer at room temperature until a homogeneous solution obtained. Thereafter, the mixture was transferred into a round bottom flask, sealed and maintained heating at 115 °C for 1.5 h in an oil bath. In this process, a kind of light green sediment (i.e., the precursor) was formed. After the reaction was completed, the precipitated powders were filtered and washed with deionized water to neutral and colorless. This was to remove the possibly adsorbed ions and chemicals to reduce the potential of agglomeration. After being dried in an oven at 90 °C for 6 h, the precursors were calcined in a muffle furnace at 400 °C for 1 h to obtain the products in dark color (i.e., NiO nanoparticles). The calcined products were then collected for further analyses.5
General procedure for the preparation of oxindol derivatives
A mixture of malononitrile (1 mmol), isatin (1 mmol), dicarbonyl (1 mmol), nano-NiO (0.0007 g) and water (2 mL) was stirred for the appropriate time at room temperatures, as shown in Tables 3 and 4 Completion of the reaction was indicated by TLC monitoring. After completion of the reaction, the reaction mixture was dissolved in acetone and catalyst was isolated by centrifuged. The product was afforded by evaporation of solvent and was recrystallized from EtOH to afforded the pure products in high purity and yield. Structural assignments of the products are based on their 1H NMR, 13C NMR and IR spectra.
2-Assmino-2′,5-dioxo-5,6,7,8-tetrahydrospiro-[chromene-4,3′-indoline]-3-carbonitrile (compound 1, Table 3). White solid, mp > 250 °C. 1H NMR (250 MHz, DMSO-d6): 1.91 (2H, m, CH2), 2.10 (2H, m, CH2), 2.62 (2H, m, CH2), 6.68 (d, 1H, J = 7.4 Hz, Ph), 6.88 (t, 1H, J = 7.4 Hz, Ph), 7.10 (d, 1H, J = 7.4 Hz, Ph), 7.22 (t, 1H, J = 7.4 Hz, Ph), 7.35 (s, 2H, NH2), 10.65 (s, 1H, NH) ppm. 13C NMR (62.9 MHz, DMSO-d6) 195.1(C
O), 178.2(C
O), 166.1(C), 158.7(C), 142.0(C), 134.6(C), 128.5(CH), 123.3(CH), 121.8(CH), 117.4(CH), 112.9(CN), 109.2(C), 57.6(C), 46.9(C), 36.4(CH2), 26.8(CH2), 19.8(CH2). IR (KBr, cm−1) 3352, 3295, 3175, 2952, 2204, 1713, 1655, 1352, 1216, 1076. Anal. calcd for C17H13N3O3: C, 66.44; H, 4.26; N, 13.67%. Found: C, 66.42; H, 4.31; N, 13.72%.
2-Amino-7,7-dimethyl-2′,5-dioxo-5,6,7,8-tetrahydrospiro[chromene-4,3′-indoline]-3-carbonitrile (compound 12, Table 3). White solid, mp > 250 °C. 1H NMR (250 MHz, DMSO-d6): 1.00 (3H, s, CH3), 1.1 (3H, s, CH3), 2.08–2.12 (2H, m, CH2), 2.56 (2H, m, CH2), 6.70 (d, 1H, J = 7.4 Hz, Ph), 6.88 (t, 1H, J = 7.4 Hz, Ph), 7.10 (d, 1H, J = 7.4 Hz, Ph), 7.22 (t, 1H, J = 7.4 Hz, Ph), 7.35 (s, 2H, NH2), 10.65 (s, 1H, NH). 13C NMR (62.9 MHz, DMSO-d6) 195.3(C
O), 178.5(C
O), 166.58(C), 159.20(C), 152.4(C), 142.48(C), 134.83(CH), 128.59(CH), 123.4(CH), 122.16(CH), 117.76(C), 112.22(CN), 109.7(C), 57.96(C), 50.45(CH2), 47.23(C), 32.35(CH2), 28.01(CH3), 27.1(CH3). IR (KBr, cm−1): 3376, 3312, 3144, 2928, 2196, 1724, 1656, 1348, 1224, 1056 cm−1. Anal. calcd for C19H17N3O3: C, 68.05; H, 5.11; N, 12.53%. Found: C, 67.86; H, 5.14; N, 12.65%.
2-Amino-2′,5-dioxo-5,6-dihydro-spiro[pyrano[3,2-c]quinoline-4,3′-indoline]-3-carbonitrile (compound 1, Table 4). White solid, mp > 250 °C. 1H NMR (250 MHz, DMSO-d6): 6.83 (1H, d, J = 7.6 Hz, Ph), 6.92 (1H, t, J = 7.6 Hz, Ph), 7.17 (1H, d, J = 7.2 Hz, Ph), 7.32 (1H, d, J = 7.6 Hz, Ph), 7.44 (1H, t, J = 7.6 Hz, Ph), 7.48 (1H, t, J = 8.2 Hz, Ph), 7.52 (1H, d, J = 8.4 Hz, Ph), 7.53 (2H, s, NH2), 7.95 (1H, d, J = 8.2 Hz, Ph), 10.65 (1H, s, NH). 13C NMR (62.9 MHz, DMSO-d6) 177.4(C
O), 158.9(C
O), 155.7(C), 155.5(C), 152.4(CH), 142.6(CH), 134.1(CH), 133.5(CH), 129.38(C), 125.4(C), 124.5(CH), 123.12(CH), 122.52(CH), 117.42(CH), 117.09(C), 112.9(CN), 109.9(C), 101.9(C), 70.2(C), 57.5(C). IR (KBr, cm−1): 3415, 3241, 3260, 2218, 1719, 1679, 1623, 1581; Anal. calcd for C20H11N3O4: C, 67.23; H, 3.10; N, 11.76%. Found: C, 67.39; H, 3.12; N, 11.65%.
Conclusions
In summary, an efficient protocol for the preparation of spirocyclic (5,6,7,8-tetrahydro-4H-chromene)-4,3′-oxindole and dihydro[pyrano[3,2-c]quinoline]-4,3-indoline derivatives was described. The procedure offers several advantages including the cheapness and the availability of the catalyst, mild reaction conditions and high yields of the products as well as simple experimental and isolation procedures. All these, make this protocol a useful and an attractive procedure for the synthesis of oxindole and indoline derivatives.
Acknowledgements
We gratefully acknowledge the support of this work by the Birjand University research council.
References
- B. Das, K. Venkateswarlu, H. Holla and M. Krishnaiah, J. Mol. Catal. A: Chem., 2006, 253, 107 CrossRef CAS PubMed.
- A. Shaabani, A. Rahmati and Z. Badri, Catal. Commun., 2008, 9, 13 CrossRef CAS PubMed.
- C. L. Carnes and K. J. Klabunde, J. Mol. Catal. A: Chem., 2003, 194, 227 CrossRef CAS.
- V. Biju and M. Abdul Khadar, Mater. Sci. Eng., A, 2001, 304, 814 CrossRef.
- Y. Wang, J. Zhu, X. Yang, L. Lu and X. Wang, Thermochim. Acta, 2005, 437, 106 CrossRef CAS PubMed.
- R. J. Sundberg, The Chemistry of Indoles, Academic Press, New York, 1996 Search PubMed.
- K. C. Joshi and P. Chand, Biologically active indole derivatives, Pharmazie, 1982, 37, 1 CAS.
- A. H. Abdel-Rahman, E. M. Keshk, M. A. Hanna and S. M. El-Bady, Bioorg. Med. Chem., 2004, 12, 2483 CrossRef CAS PubMed.
- T. H. Kang, K. Matsumoto, M. Tohda, Y. Murakami, H. Takayama, M. Kitajima, N. Aimi and H. Watanabe, Eur. J. Pharmacol., 2002, 444, 39 CrossRef CAS.
- J. Leclercq, M. C. De Pauw Gillet, R. Bassleer and L. Angenot, J. Ethnopharmacol., 1986, 15, 305 CrossRef CAS.
-
(a) J. Ma and S. M. Javaniside, Chem. Commun., 2004, 1190 RSC;
(b) R. Y. Guo, Z. M. An, L. P. Mo, R. Z. Wang, H. X. Liu, S. X. Wang and Z. H. Zhang, ACS Comb. Sci., 2013, 15, 557 CrossRef CAS PubMed.
- S. Pepeljnjak, I. Jalsenjak and D. Maysinger, Pharmazie, 1982, 37, 864 CAS.
- B. E. Evans, K. E. Rittle, M. G. Bock, R. M. DiPardo, R. M. Freidinger, W. L. Whitter, G. F. Lundell, D. F. Veber, P. S. Andersons and R. S. Chang, J. Med. Chem., 1988, 31, 2235 CrossRef CAS.
- L. L. Andreani and E. Lapi, Boll. Chim. Farm., 1960, 99, 583 Search PubMed.
- L. Bonsignore, G. Loy and D. Secci, Eur. J. Med. Chem., 1993, 28, 517 CrossRef CAS.
- C. J. Li, Chem. Rev., 2005, 105, 3095 CrossRef CAS PubMed.
- F. Bazi, H. Badaoui, S. Tamani, S. Sokori, A. Solhy, D. J. Macquarrie and S. Sebti, Appl. Catal., A, 2006, 211, 301 Search PubMed.
- M. A. Nasseri, F. Ahrari and B. Zakerinasab, RSC Adv., 2015, 5, 13901 RSC.
- M. A. Nasseri, B. Zakerinasab and M. M. Samieadel, RSC Adv., 2014, 4, 41753 RSC.
- M. A. Nasseri, S. A. Alavi and B. Zakerinasab, J. Iran. Chem. Soc., 2013, 10, 21 CrossRef PubMed.
- M. A. Nasseri and M. Salimi, Lett. Org. Chem., 2013, 10, 164 CrossRef CAS.
- M. A. Nasseri and S. M. Sadeghzadeh, J. Iran. Chem. Soc., 2014, 11, 27 CrossRef CAS PubMed.
|
This journal is © The Royal Society of Chemistry 2015 |