DOI:
10.1039/C5RA01723H
(Paper)
RSC Adv., 2015,
5, 25051-25058
Investigation of antiglycation activity of isoprenaline†
Received
28th January 2015
, Accepted 2nd March 2015
First published on 2nd March 2015
Abstract
Advanced glycation end products (AGEs) are implicated in pathogenesis of diabetes and its complications. In this study, we report the ability of isoprenaline to inhibit the AGE modification of protein by fluorescence spectroscopy and western blotting. Isoprenaline was more effective in inhibiting AGE modification than aminoguanidine, a well known glycation inhibitor. Further, we show that isoprenaline inhibits at Amadori product formation during glycation reaction by various techniques such as MALDI-TOF-MS, LC-MS/MS, and fructosamine assay. Mass spectrometric analysis of the glycation reaction mixture incubated with isoprenaline suggested that it forms adducts with glucose and thus inhibits glycation. The finding of the additional property of isoprenaline of inhibiting glycation suggests that it is a potential candidate for drug repositioning for the treatment of diabetes and its complications, as it is an FDA approved drug.
Introduction
Diabetes is a group of metabolic diseases characterized by elevated levels of blood sugar over a prolonged period. Poorly controlled diabetes is associated with serious complications such as cardiovascular disease, stroke, kidney failure, foot ulcers and damage to the eyes. Advanced glycation end products (AGEs) have been implicated as one of the major causal factors in pathogenesis of diabetes and its complications. AGEs are formed as a result of a series of non-enzymatic reactions between reducing sugars and proteins.1,2 In diabetes, the levels of AGEs are substantially increased due to hyperglycaemic condition. Especially plasma proteins are known to undergo AGE modification and interact with the receptor for AGEs (RAGE). The AGE-RAGE interaction leads to oxidative stress and activation of proinflammatory pathways. AGE-RAGE axis is involved in the development of many degenerative diseases such as diabetes, atherosclerosis, chronic renal failure and Alzheimer's disease.2–4 Therefore, reducing AGE levels has been considered as a therapeutic strategy in the treatment and management of diabetes. Although widely used FDA approved drugs like metformin, aspirin, diclofenac, hydralazine, rifampicin known to possess antiglycation activity, they are not directly used for the reduction of AGEs.4–8 Many efforts have been made to seek new AGE inhibitors; however aminoguanidine and pyridoxamine are the only drugs that were evaluated in clinical trials but their adverse effects made them less favourable in clinical applications.9 Previously we have reported antiglycation activity of rifampicin, hydralazine and protriptyline by using MALDI-TOF-MS based insulin glycation assay.7,8,10,11 The present study deals with demonstration of antiglycation activity of isoprenaline and its mode of action by various physicochemical approaches like fluorescence spectroscopy, MALDI-TOF-MS, LC-MS/MS etc.
Results and discussion
Fluorescence inhibition and IC50 studies
AGEs display a characteristic fluorescence (Ex 370/Em 440 nm) that can be used to quantify the extent of AGE-modification.12 Using AGE fluorescence assay isoprenaline was found to be a potent AGE inhibitor as reflected by decreased AGE fluorescence (A, Fig. 1). Isoprenaline was more effective in decreasing AGE fluorescence compared to aminoguanidine at the same concentrations. AGE fluorescence based assay was used to determine the IC50 of aminoguanidine.13 Therefore, using this assay, the IC50 of isoprenaline was determined, which was found to be lower (0.07 mM) than aminoguanidine (6.99 mM) (B and C Fig. 1). The lower IC50 of isoprenaline suggests that it is a more potent glycation inhibitor than aminoguanidine.
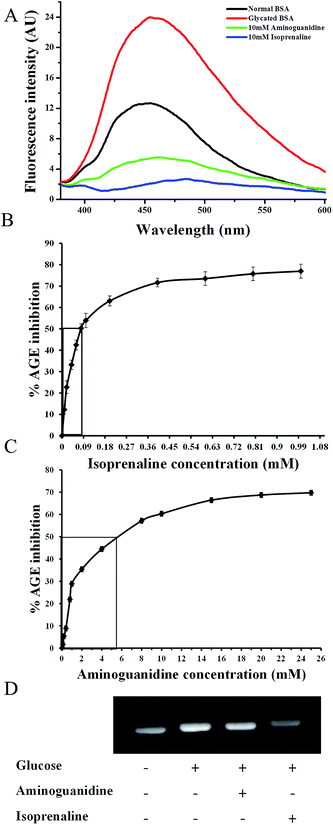 |
| Fig. 1 (A) AGE fluorescence (Ex/Em 370/440 nm) spectra of BSA, glycated BSA or glycated BSA treated with 10 mM aminoguanidine or 10 mM isoprenaline; IC50 values for AGE inhibition (B) isoprenaline and (C) aminoguanidine; (D) western blotting of BSA, glycated BSA or glycated BSA treated with 10 mM aminoguanidine or 10 mM of isoprenaline. | |
The isoprenaline and aminoguanidine induced decrease in AGE fluorescence of BSA was also reflected in western blotting by anti-AGE antibodies (D, Fig. 1). In vitro glycation of BSA showed increase in the extent of AGE modifications, which was not considerably inhibited by aminoguanidine. Densitometric analysis of western blot with anti-AGE antibodies showed significant reduction in AGE modifications in isoprenaline treated glycated BSA than with aminoguanidine.
Circular dichroism
Protein glycation is associated with change in protein conformation and function.14 The circular dichroism spectroscopy analysis showed both isoprenaline and aminoguanidine treatment resulted in decrease of beta sheet percentage in glycated BSA (Fig. 2). Isoprenaline was able to protect the conformation of BSA at concentration as low as 0.1 mM. At higher concentration (>0.2 mM), isoprenaline interferes in CD analysis.
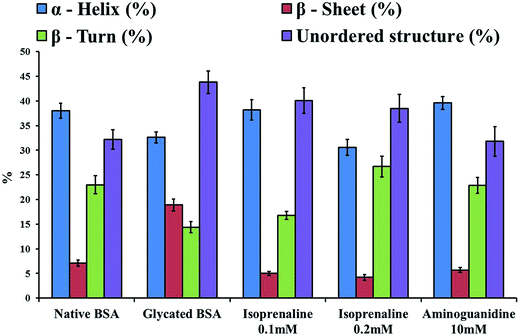 |
| Fig. 2 CDPro analysis of native BSA, glycated BSA, or glycated BSA treated with 0.1 mM isoprenaline, or 0.2 mM isoprenaline or 10 mM aminoguanidine. | |
Probable mechanism of action of isoprenaline
AGE fluorescence and western blotting have suggested that isoprenaline is capable of reducing AGE modification of proteins. Thus, to understand the mechanism by which isoprenaline reduces AGE modification of proteins, MALDI-TOF-MS based Insulin glycation assay, fructosamine assay, LC-MS analysis of glycated BSA and isoprenaline–glucose adduct were studied.
Isoprenaline inhibits Schiff's base or Amadori modification
We have previously reported MALDI-TOF-MS based insulin glycation inhibition assay, where in insulin (m/z 5808) was allowed to react with glucose to form Amadori modified insulin (m/z 5970) and the intensity of Amadori modified insulin was monitored in presence or absence of inhibitors.7,8 This assay was used to evaluate whether isoprenaline inhibits at Amadori modification during glycation reaction. Fig. 3C shows that isoprenaline was able to inhibit Amadori modification, as the intensity of Amadori modified insulin decreased in presence of isoprenaline. The extent of inhibition was more in presence of isoprenaline than aminoguanidine (Fig. 3). Further, isoprenaline's ability to inhibit Amadori modification was studied by high resolution accurate mass spectrometer (Q-Exactive, Orbitrap mass spectrometer). BSA was glycated in absence or presence of isoprenaline or aminoguanidine and formation of Amadori modified peptides after tryptic digestion was monitored by LC-MS/MS. A representative MS/MS spectrum of Amadori modified peptide is depicted in ESI Fig. 1.† As expected glycated BSA had maximum number (23) of Amadori modified peptides. While glycated BSA treated with aminoguanidine and isoprenaline had 16 and 10 Amadori modified peptides, respectively (A, Fig. 4, Table 1). Next, the extent of glycation was determined by calculating the ratio of area under curve (AUC) of few modified to unmodified peptides (Table 2, ESI Fig. 2†), as well as by comparing the area under curve for some of the glucose sensitive peptides (GSPs)15 in glycated BSA, glycated BSA treated with isoprenaline or aminoguanidine (B, Fig. 4, ESI Fig. 3 and 4†). Based on these results, it was observed that the extent of glycation was higher in glycated BSA, followed by glycated BSA treated with aminoguanidine and isoprenaline.
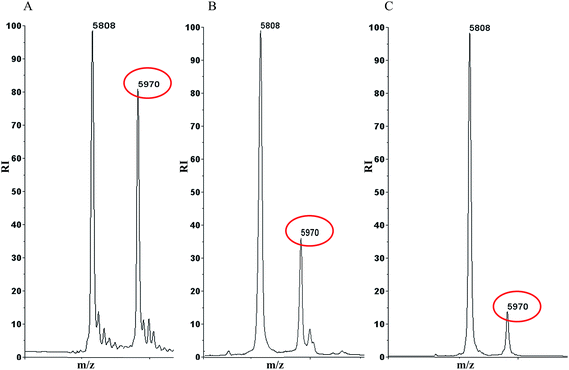 |
| Fig. 3 MALDI-TOF-MS based insulin glycation inhibition assay, A. Glycated insulin B. Glycated insulin treated with 10 mM aminoguanidine C. Glycated insulin treated with 10 mM isoprenaline. | |
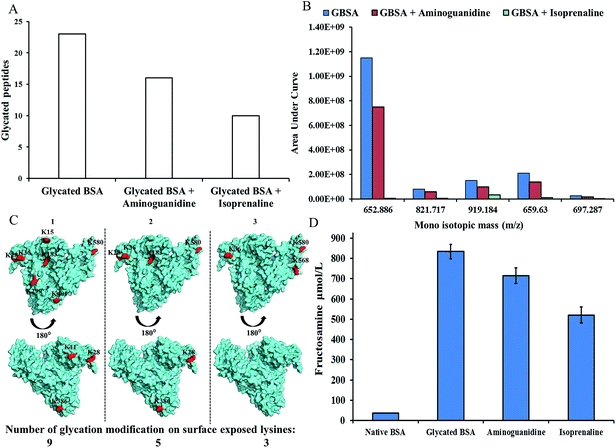 |
| Fig. 4 (A) LC-MS/MS analysis depicting the number of Amadori modified peptides in glycated BSA, glycated BSA treated with 10 mM of aminoguanidine or 10 mM of isoprenaline; (B) AUC for glucose sensitive peptides of glycated BSA, glycated BSA treated with aminogaunidine or isoprenaline; (C) glycation modifications depicting surface exposed lysine residues, (1) glycated BSA (2) glycated BSA treated with aminoguanidine (3) glycated BSA treated with isoprinaline; (D) fructosamine levels of native BSA, glycated BSA, glycated BSA treated with aminoguanidine or isoprinaline. | |
Table 1 List of Amadori modified peptides of in vitro glycated BSA, glycated BSA treated with isoprenaline (10 mM) or aminoguanidine (10 mM)a
Sr. no. |
Mod. site |
Peptide sequence |
MH+ |
m/z |
CS |
RT |
XCorr |
MC |
Modification |
AUC |
Static modification: carbamidomethyl (57.02146 Da), CS-charge state, RT-retention time, MC-missed cleavage. |
Glycated BSA |
1 |
183 |
RHPYFYAPELLYYANK*YNGVFQECCQAEDK |
3935.746 |
787.955 |
+5 |
25.07 |
6.42 |
2 |
Amadori |
5.04 × 107 ± 20071 |
2 |
580 |
TVMENFVAFVDK*CCAADDKEACFAVEGPK |
3470.531 |
868.388 |
+4 |
26.94 |
6.05 |
2 |
Amadori |
5.98 × 107 ± 22342 |
3 |
346 |
NYQEAK*DAFLGSFLYEYSR |
2463.143 |
821.719 |
+3 |
26.21 |
4.95 |
1 |
Amadori |
8.42 × 107 ± 30953 |
4 |
437 |
K*VPQVSTPTLVEVSR |
1801.988 |
601.334 |
+3 |
17.40 |
3.51 |
1 |
Amadori |
2.99 × 107 ± 14022 |
5 |
318 |
SHCIAEVEK*DAIPENLPPLTADFAEDKDVCK |
3673.719 |
919.185 |
+4 |
21.74 |
5.41 |
2 |
Amadori |
1.50 × 108 ± 42489 |
6 |
587 |
CCAADDK*EACFAVEGPK |
2089.844 |
697.286 |
+3 |
14.09 |
3.49 |
1 |
Amadori |
2.79 × 107 ± 14544 |
7 |
28 |
DTHK*SEIAHR |
1355.653 |
452.555 |
+3 |
2.77 |
2.15 |
1 |
Amadori |
9.37 × 106 ± 13408 |
8 |
266 |
VHK*ECCHGDLLECADDRADLAK |
2774.213 |
925.409 |
+3 |
14.30 |
4.68 |
2 |
Amadori |
5.37 × 107 ± 39711 |
9 |
386 |
EYEATLEECCAK*DDPHACYSTVFDK |
3200.297 |
800.829 |
+4 |
17.86 |
4.23 |
1 |
Amadori |
3.16 × 107 ± 12925 |
10 |
140 |
LK*PDPNTLCDEFK |
1738.818 |
580.277 |
+3 |
17.51 |
3.07 |
0 |
Amadori |
3.04 × 107 ± 79816 |
11 |
36 |
FK*DLGEEHFK |
1411.670 |
471.228 |
+3 |
12.74 |
2.13 |
1 |
Amadori |
7.46 × 107 ± 31968 |
12 |
548 |
K*QTALVELLK |
1304.764 |
652.885 |
+2 |
19.29 |
2.86 |
1 |
Amadori |
1.15 × 109 ± 28202 |
13 |
299 |
LK*ECCDKPLLEK |
1694.828 |
847.917 |
+2 |
28.95 |
2.53 |
1 |
Amadori |
2.16 × 108 ± 47561 |
14 |
401 |
LK*HLVDEPQNLIK |
1708.944 |
854.975 |
+2 |
16.00 |
3.17 |
1 |
Amadori |
3.78 × 107 ± 27453 |
15 |
463 |
CCTK*PESER |
1328.543 |
443.519 |
+3 |
3.59 |
1.89 |
0 |
Amadori |
1.98 × 107 ± 54866 |
16 |
197 |
YNGVFQECCQAEDK*GACLLPK |
2649.158 |
883.724 |
+3 |
19.00 |
2.05 |
1 |
Amadori |
3.96 × 107 ± 13231 |
17 |
117 |
ETYGDMADCCEK*QEPER |
2279.865 |
760.626 |
+3 |
11.30 |
1.34 |
1 |
Amadori |
2.29 × 106 ± 11890 |
18 |
304 |
ECCDK*PLLEK |
1453.653 |
485.222 |
+3 |
10.58 |
1.67 |
0 |
Amadori |
1.07 × 107 ± 29797 |
19 |
151 |
LKPDPNTLCDEFK*ADEKK |
2310.118 |
578.285 |
+4 |
17.15 |
1.99 |
2 |
Amadori |
5.71 × 107 ± 30900 |
20 |
130 |
QEPERNECFLSHK*DDSPDLPK |
2703.217 |
541.449 |
+5 |
14.18 |
2.15 |
2 |
Amadori |
1.36 × 107 ± 23347 |
21 |
256 |
AEFVEVTK*LVTDLTK |
1854.992 |
928.000 |
+2 |
26.98 |
3.50 |
1 |
Amadori |
5.04 × 107 ± 20071 |
22 |
374 |
LAK*EYEATLEECCAK |
1976.880 |
988.943 |
+2 |
14.13 |
4.04 |
1 |
Amadori |
5.98 × 107 ± 22342 |
23 |
130 |
NECFLSHK*DDSPDLPK |
2063.920 |
516.735 |
+4 |
14.94 |
1.89 |
1 |
Amadori |
8.42 × 107 ± 30953 |
|
Glycated BSA treated with isoprenaline |
1 |
346 |
NYQEAK*DAFLGSFLYEYSR |
2463.136 |
821.716 |
+3 |
26.50 |
3.26 |
1 |
Amadori |
5.04 × 106 ± 8633 |
2 |
256 |
AEFVEVTK*LVTDLTK |
1854.992 |
927.999 |
+2 |
27.34 |
2.81 |
1 |
Amadori |
1.35 × 107 ± 22150 |
3 |
587 |
CCAADDK*EACFAVEGPK |
2089.846 |
697.287 |
+3 |
14.47 |
2.42 |
1 |
Amadori |
2.72 × 106 ± 10972 |
4 |
548 |
K*QTALVELLK |
1304.767 |
652.887 |
+2 |
19.90 |
2.16 |
1 |
Amadori |
6.94 × 106 ± 12061 |
5 |
580 |
TVMENFVAFVDK*CCAADDKEACFAVEGPK |
3486.516 |
872.384 |
+4 |
23.56 |
2.46 |
2 |
Amadori |
4.34 × 106 ± 78917 |
6 |
318 |
SHCIAEVEK*DAIPENLPPLTADFAEDKDVCK |
3673.716 |
919.184 |
+4 |
22.65 |
5.06 |
2 |
Amadori |
3.36 × 107 ± 35344 |
7 |
568 |
ATEEQLK*TVMENFVAFVDK |
2377.155 |
793.056 |
+3 |
26.35 |
3.98 |
1 |
Amadori |
2.59 × 107 ± 45698 |
8 |
36 |
FK*DLGEEHFK |
1411.671 |
471.228 |
+3 |
13.67 |
1.41 |
1 |
Amadori |
2.46 × 106 ± 34145 |
9 |
266 |
VHK*ECCHGDLLECADDRADLAK |
2774.213 |
555.648 |
+5 |
14.65 |
2.96 |
2 |
Amadori |
1.32 × 107 ± 17338 |
10 |
374 |
LAK*EYEATLEECCAK |
1976.878 |
659.630 |
+3 |
14.57 |
2.68 |
1 |
Amadori |
1.25 × 107 ± 23067 |
|
Glycated BSA treated with aminoguanidine |
1 |
580 |
TVMENFVAFVDK*CCAADDKEACFAVEGPK |
3470.520 |
868.385 |
+4 |
26.77 |
6.31 |
2 |
Amadori |
4.04 × 107 ± 60140 |
2 |
183 |
RHPYFYAPELLYYANK*YNGVFQECCQAEDK |
3935.768 |
984.697 |
+4 |
25.28 |
6.75 |
2 |
Amadori |
1.62 × 107 ± 10204 |
3 |
346 |
NYQEAK*DAFLGSFLYEYSR |
2463.139 |
821.717 |
+3 |
26.31 |
5.10 |
1 |
Amadori |
6.24 × 107 ± 14931 |
4 |
266 |
VHK*ECCHGDLLECADDRADLAK |
2774.214 |
925.409 |
+3 |
14.71 |
4.72 |
2 |
Amadori |
3.36 × 107 ± 80061 |
5 |
587 |
CCAADDK*EACFAVEGPK |
2089.847 |
697.287 |
+3 |
14.51 |
3.20 |
1 |
Amadori |
1.79 × 107 ± 61888 |
6 |
386 |
EYEATLEECCAK*DDPHACYSTVFDK |
3200.299 |
800.830 |
+4 |
17.94 |
4.11 |
1 |
Amadori |
2.22 × 107 ± 67252 |
7 |
437 |
K*VPQVSTPTLVEVSR |
1801.987 |
601.334 |
+3 |
17.49 |
3.68 |
1 |
Amadori |
1.98 × 107 ± 10180 |
8 |
318 |
SHCIAEVEK*DAIPENLPPLTADFAEDKDVCK |
3673.721 |
919.185 |
+4 |
22.28 |
5.13 |
2 |
Amadori |
1.36 × 108 ± 325001 |
9 |
140 |
LK*PDPNTLCDEFK |
1738.817 |
580.277 |
+3 |
17.53 |
3.02 |
0 |
Amadori |
2.15 × 107 ± 19161 |
10 |
548 |
K*QTALVELLK |
1304.765 |
652.886 |
+2 |
19.62 |
2.84 |
1 |
Amadori |
7.65 × 108 ± 13907 |
11 |
36 |
FK*DLGEEHFK |
1411.672 |
471.229 |
+3 |
13.97 |
2.12 |
1 |
Amadori |
4.82 × 107 ± 17859 |
12 |
130 |
NECFLSHK*DDSPDLPK |
2063.920 |
516.735 |
+4 |
15.07 |
1.77 |
1 |
Amadori |
4.55 × 106 ± 30820 |
13 |
401 |
LK*HLVDEPQNLIK |
1708.945 |
854.976 |
+2 |
15.96 |
2.70 |
1 |
Amadori |
2.37 × 107 ± 98943 |
14 |
28 |
DTHK*SEIAHR |
1355.651 |
452.555 |
+3 |
2.5 |
2.16 |
1 |
Amadori |
7.04 × 106 ± 23819 |
15 |
463 |
CCTK*PESER |
1328.544 |
443.519 |
+3 |
3.42 |
2.00 |
0 |
Amadori |
1.36 × 107 ± 23415 |
16 |
304 |
ECCDK*PLLEK |
1453.654 |
485.222 |
+3 |
11.50 |
1.59 |
0 |
Amadori |
7.07 × 106 ± 82741 |
Table 2 Area under curve ratio of few modified to unmodified peptides in glycated BSA, glycated BSA treated with isoprenaline (10 mM) or aminoguanidine (10 mM)
Sr. no. |
Mod. site |
Sequence |
MH+ |
m/z |
CS |
RT |
AUC ratio (modified/control) |
Glycated BSA |
1 |
548 |
K*QTALVELLK |
1304.764 |
652.885 |
2 |
19.29 |
9.01 ± 2.75 |
KQTALVELLK |
1142.716 |
571.861 |
2 |
19.48 |
2 |
580 |
TVMENFVAFVDK*CCAADDKEACFAVEGPK |
3470.531 |
868.388 |
4 |
26.94 |
16.95 ± 3.4 |
TVMENFVAFVDKCCAADDKEACFAVEGPK |
3308.468 |
827.872 |
4 |
27.01 |
3 |
318 |
SHCIAEVEK*DAIPENLPPLTADFAEDKDVCK |
3673.719 |
919.185 |
4 |
21.74 |
1.70 ± 0.08 |
SHCIAEVEKDAIPENLPPLTADFAEDKDVCK |
3511.667 |
1171.22 |
3 |
21.82 |
4 |
266 |
VHK*ECCHGDLLECADDRADLAK |
2774.213 |
925.409 |
3 |
14.3 |
1.40 ± 0.06 |
VHKECCHGDLLECADDRADLAK |
2612.159 |
871.391 |
3 |
14.47 |
|
Glycated BSA treated with isoprenaline |
1 |
548 |
K*QTALVELLK |
1304.767 |
652.887 |
2 |
19.9 |
0.01 ± 0.0025 |
KQTALVELLK |
1142.716 |
571.861 |
2 |
20.1 |
2 |
587 |
CCAADDK*EACFAVEGPK |
2089.846 |
697.287 |
3 |
14.47 |
0.03 ± 0.0002 |
CCAADDKEACFAVEGPK |
1927.793 |
964.4 |
2 |
14.63 |
3 |
580 |
TVMENFVAFVDK*CCAADDKEACFAVEGPK |
3486.516 |
872.384 |
4 |
23.56 |
0.47 ± 0.025 |
TVMENFVAFVDKCCAADDKEACFAVEGPK |
3324.466 |
831.872 |
4 |
23.78 |
4 |
318 |
SHCIAEVEK*DAIPENLPPLTADFAEDKDVCK |
3673.716 |
919.184 |
4 |
22.65 |
0.61 ± 0.05 |
SHCIAEVEKDAIPENLPPLTADFAEDKDVCK |
3511.667 |
1171.22 |
3 |
22.6 |
|
Glycated BSA treated with aminoguanidine |
1 |
548 |
K*QTALVELLK |
1304.765 |
652.886 |
2 |
19.62 |
6.56 ± 1.0 |
KQTALVELLK |
1142.716 |
571.861 |
2 |
19.59 |
2 |
580 |
TVMENFVAFVDK*CCAADDKEACFAVEGPK |
3470.531 |
868.388 |
4 |
26.94 |
11.23 ± 1.5 |
TVMENFVAFVDKCCAADDKEACFAVEGPK |
3308.468 |
827.872 |
4 |
27 |
3 |
318 |
SHCIAEVEK*DAIPENLPPLTADFAEDKDVCK |
3673.719 |
919.185 |
4 |
22.28 |
1.78 ± 0.05 |
SHCIAEVEKDAIPENLPPLTADFAEDKDVCK |
3511.667 |
1171.22 |
3 |
22.44 |
4 |
587 |
CCAADDK*EACFAVEGPK |
2089.847 |
697.287 |
3 |
14.51 |
0.15 ± 0.002 |
CCAADDKEACFAVEGPK |
1927.793 |
964.4 |
2 |
14.49 |
The lysine residues of the Amadori modified peptides were analyzed for their solvent accessibility using PDB structure of BSA. The solvent accessible lysine residues are highlighted in red color (C, Fig. 4). The glycated BSA showed nine surface exposed glycated lysine residues (K28, 36, 117, 151, 183, 299, 386, 401 and 580), whereas the glycated BSA treated with aminoguanidine showed five surface exposed glycated lysine residues (K28, 36, 183, 386 and 580). Interestingly only three surface lysine residues were identified to be glycated in the glycated BSA treated with the isoprenaline (K36, 568 and 580). However, in case of glycated BSA, several additional lysine residues were found to Amadori modified in mass spectrometric analysis but were not observed as solvent accessible. As glycation promotes beta sheet formation, during this process, exposure of buried lysine residues could be possible.16
Fructosamine assay
Mass spectrometric analysis suggested that isoprenaline inhibits at the Amadori product formation. This was also corroborated with fructosamine assay. Fructosamine is one of the early stage products of glycation reaction.17 It has been used commonly to measure short-term control of blood sugar in diabetic patients. In glycated BSA fructosamine levels were found to be 834.32 μmol L−1, whereas glycated BSA treated with 10 mM aminoguanidine or 10 mM isoprenaline showed decreased fructosamine level (714.62 μmol L−1 and 520.84 μmol L−1, respectively) (D, Fig. 4).
Isoprenaline forms adduct with glucose
LC-MS analysis of glycation reaction mixture incubated with isoprenaline (m/z 212.12) suggested that it forms glucose adduct (m/z 374.18) (A, Fig. 5). The probable mechanism of isoprenaline–glucose adduct formation is depicted in Fig. 5B. Although the drug–glucose adduct formed was relatively less, formation of adduct could be one of the probable ways by which isoprenaline inhibits glycation, along with its ability to induce conformation changes in albumin.
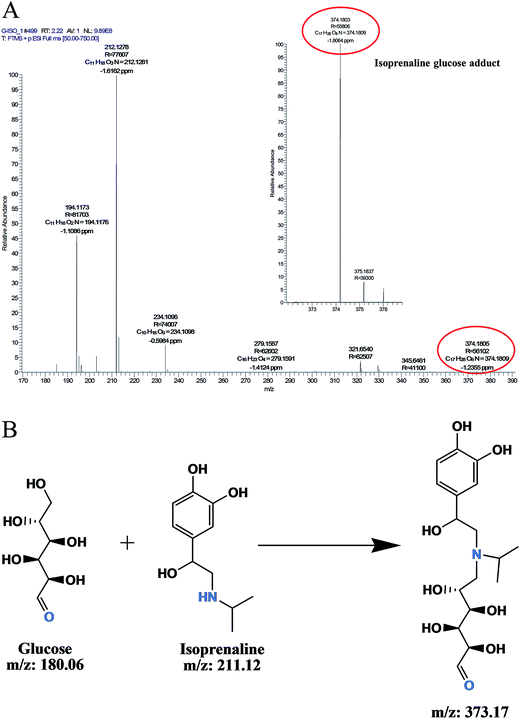 |
| Fig. 5 (A) LC-MS analysis of isoprenaline glucose adduct formation. (B) The probable mechanism of isoprenaline glucose adduct formation. | |
As AGEs are associated with various diseases including aging, diabetes, cancer, neurodegenerative diseases, it is important to keep their levels minimal. Thus, there has been great effort in discovering molecules that inhibit glycation and AGE formation. Drug repositioning is emerging as a powerful approach in the area of drug discovery, as it has several advantages including reduced time and cost necessary for clinical trials. In this study we report the discovery of isoprenaline as a potent effective AGE inhibitor amongst several screened drugs. Mass spectrometric analysis and fructosamine assays suggested that it inhibits at Amadori product formation by forming adduct with glucose. Isoprenaline is an FDA approved drug used for the treatment of bradycardia and asthma. It has also been patented as an eye drop for AGE inhibition for the treatment of cataract.18 Thus isoprenaline is a potential candidate for repositioning for the treatment of glycation associated diseases.
Experimental
Chemicals
All the chemicals were procured from Sigma-Aldrich unless otherwise mentioned. AGE antibody, protein A-HRP conjugate were purchased from Merck Millipore (India).
In vitro glycation of bovine serum albumin (BSA)
In vitro glycation of BSA was done as described,19 with slight modifications. Briefly, the reaction was carried out by incubating 225 μL of 50 mg mL−1 BSA in 0.1 M phosphate buffer (pH 7.4) and 75 μL of 2 M D-glucose containing 5 mM sodium azide as a bacteriostat with or without 10 mM (final concentration) of isoprenaline and aminoguanidine drugs at 37 °C for 15 days. BSA glycation was monitored by fluorescence spectroscopy, excitation at 370 and emission at 440 nm by using a spectrofluorometer (Thermo, Varioskan Flash Multimode Reader).
IC50 of isoprenaline and aminoguanidine
The percent inhibition of AGE formation was calculated by formula: inhibition = (1 − Fi/Fc) × 100, Where Fi = fluorescence intensity of glycated BSA treated with inhibitor and Fc = fluorescence intensity of glycated BSA without inhibitor. The apparent IC50 was determined by plotting the percent glycation inhibition versus inhibitor concentration.
Western blot analysis using anti AGE antibody
BSA, glycated BSA, glycated BSA with 10 mM of aminoguanidine or 10 mM of isoprenaline was incubated at 37 °C for 15 days. 2 μg of each protein sample was separated on 12% SDS-PAGE and transferred onto the PVDF membrane. The membranes were blocked with 5% skimmed milk powder dissolved in TBS (20 mM Tris–HCl (pH 7.5), 0.15 M NaCl). The proteins were probed by AGE antibodies, and the antibody dilution was adopted according to the manufacturer instructions. Protein ‘A’ HRP conjugate was used in 1
:
5000 dilutions. Immunoreactive bands were visualized using WesternBright Quantum western blotting detection kit (Advansta) and documented on Dyversity system (Syngene, UK).
Circular dichroism spectroscopy measurements
0.05 mg mL−1 of BSA, glycated BSA, glycated BSA treated with 10 mM of aminoguanidine or 10 mM of isoprenaline was used to acquire the CD spectra. All the spectra were measured at room temperature on a JASCO J-815 Chiro-Optical Spectrometer (Jasco Inc., Easton, MD, USA). The spectra results from averaging three scans and were corrected for respective blanks. Results are expressed as molar ellipticity, [Θ] (deg cm2 dmol−1), based on a mean amino acid residue weight (MRW). The molar ellipticity was determined as [Θ]λ = (Θ100MRW)/(cl), where l, is the light path length in centimeters, ‘c’ is the protein concentration in mg mL−1, and Θ is the measured ellipticity in degrees at the relevant wavelength (250–190 nm). The CD spectra of the protein samples were analysed to calculate the content of secondary structure using CDPro software that has three algorithms: CONTINLL, CDSSTR and SELCON3.20
Insulin-MALDI based assay
For insulin glycation assay, insulin 50 μL (1.8 mg mL−1) was incubated in 200 μL of 0.1 M phosphate buffer (pH 7.4) containing 50 μL of glucose (250 mM) and 10mM of isoprenaline or 10mM of aminoguanidine. The reaction was monitored for 7 days at 37 °C till the relative intensity decreased to 50% on MALDI-TOF-TOF (AB SCIEX TOF/TOF™ 5800). The reaction mixture was mixed with sinapinic acid and analysed on MALDI-TOF-TOF system in linear mode using Anchor Chip 384 targets as described in.8
Fructosamine assay
Fructosamine level was measured by the NBT Labkit (Chemelex, S.A.). 300 μL of 0.75 mM NBT was added to a 96-well microplate containing 30 μL of 150 μg BSA, glycated BSA, glycated BSA with 10 mM of aminoguanidine and glycated BSA with 10 mM of isoprenaline. The reduction of NBT by fructosamine group was measured at 520 nm immediately after additions considered as (Abs1), the incubation at 37 °C for 15 min considered as (Abs2) the absorbance was monitored by using spectrophotometer (Thermo, Varioskan Flash Multimode Reader). The fructosamine level was calculated by using the Labkit (Chemelex, S.A.). Fructosamine level = ΔAsample/ΔA calibrant X concentration of calibrant (μmol L−1).
LC-HR/AM Q-Exactive Orbitrap analysis (full MS/dd-MS2)
Tryptic digestion. Prior to LC MS/MS analysis, 100 μg of protein was reduced with 100 mM dithiothreitol (DTT) at 60 °C for 15 min, then alkylated with 200 mM iodoacetamide in dark at room temperature for 30 min. Proteins were digested by adding proteomic grade trypsin in the ratio of 1
:
50 (final enzyme: proteins) at 37 °C overnight. The digestion reaction was stopped by adding concentrated HCL and incubated for 10 min at 37 °C before vertex and centrifugation.
Chromatographic separation
Peptide digest (1.5 μg) were separated on Hypersil Gold C18-reverse phase HPLC column (150 × 2.1 mm, 1.9 μm) with 98% of mobile phase A (100% water, 0.1% FA) and 2% of mobile phase B (100% ACN, 0.1% FA) at 350 μL min−1 flow rate with a 45 min linear gradient of 2% to 40% mobile phase B.
Mass spectrometry acquisition (full MS/dd-MS2)
All samples were analysed on hybrid quadrupole Orbitrap mass spectrometer (Q-Exactive). The instrument was tuned and calibrated before analysis. The tune parameters include: spray voltage 4200 V, capillary temperature 320 °C, heater temperature 200 °C, S-lens RF value 55, sheath and auxiliary gases pressure were 30 and 8 psi respectively. The samples were acquired in positive ionization (HESI) mode in data-dependent manner using a top-5 method with scan range from 350–1800 m/z. MS spectra were acquired at a resolution of 70
000 with maximum injection time (IT) of 120 ms and automatic gain control (AGC) value of 1 × e6 ions and MS/MS spectra were acquired at 17
500 resolution with maximum IT of 120 ms and AGC value of 1 × e5 ions. Precursor's selectivity was performed at an isolation width of 3 m/z, under fill ratio of 0.3% and dynamic exclusion time of 15 s. The peptide fragmentation was performed in HCD cell using normalized high energy collision induced dissociation at 30 eV.
Database search and PTM analysis
The mass spectrometric data was processed using Proteome discoverer 1.4 (Version 1.4.0.288), (Thermo Fisher Scientific, Bremen, Germany). SEQUEST HT search engine was used for peptide identification. The data was searched against UniProt Bovine Serum Albumin (P02769) sequence database. Carbamidomethylation of cysteine (C) and oxidation at methionine (M) was considered as fixed and variable modification respectively. Additionally glycation modifications at lysine position were searched as variable modifications. The search was performed using the following parameters: peptide and fragment mass tolerance were 10 ppm, 0.5 Da respectively with minimum of 2 missed cleavages and 1% false discovery rate (FDR). The identified glycation modified peptides were selected based on the criteria described earlier.21 The Glycation modification list includes carboxymethyllysine (CML) (+58.005 Da); carboxyethyllysine (CEL) (+72.021 Da), Amadori (+162.02 Da); FL-1H2O (+144.042 Da); FL-2H2O (+126.032 Da). The extent of glycation was monitored by extraction ion chromatogram (XIC) of modified peptides using Xcalibur (Thermo xcalibur 2.2 SP1.48).
Molecular modelling of BSA
Three dimensional structure of bovine serum albumin (BSA) was downloaded from the RCSB protein data bank (PDB ID:3V03). Solvent accessible residues in BSA were identified using Swiss PDB viewer (http://spdbv.vital-it.ch/) with solvent accessibility index >30%. Lysine residues that are exposed and identified as glycated in mass spectrometric analysis were marked on the surface model of BSA using PyMol software (PyMol Molecular Graphics System, Version 1.2r3 pre, Schrodinger LLC). Surface model of glycated BSA, glycated BSA treated with aminoguanidine or isoprenaline were compared to analyze the effect of these compounds on the glycation of surface exposed residues.
Isoprenaline–glucose conjugate adduct formation
To elucidate the AGE inhibition mechanism of isoprenaline, the isoprenaline (10 mM) were incubated with glucose (0.5 mM) in phosphate buffer pH 7.4 at 37 °C for 3 days. The reaction was analysed on Q-Exactive Orbitrap to find the drug glucose conjugate adduct formation.
Acknowledgements
This work was financially supported by CSIR-Network project CSC0111. YMK thanks CSIR, India for post doctoral Research Associateship.
References
- R. Singh, A. Barden, T. Mori and L. Beilin, Diabetologia, 2001, 44, 126–141 CrossRef PubMed
. - M. J. Kulkarni, A. M. Korwar, S. Mary, H. S. Bhonsle and A. P. Giri, Proteomics: Clin. Appl., 2013, 7, 155–170 CrossRef CAS PubMed
. - K. B. Batkulwar, S. B. Bansode, G. V. Patil, R. K. Godbole, R. S. Kazi, S. Chinnathambi, D. Shanmugam and M. J. Kulkarni, Proteomics, 2015, 15(2–3), 245–259 CrossRef CAS PubMed
. - S. Rahbar, R. Natarajan, K. Kumar, K. K. Yerneni, S. Scott, N. Gonzales and N. J. Nadler, Clin. Chim. Acta, 2000, 301, 65–77 CrossRef CAS
. - M. Sensi, M. PricciGrazla De Rossi, S. Morano and U. Mario, Clin. Chem., 1989, 35, 384–387 CAS
. - M. A. M. Van Boekel, P. J. P. C. Van den Bergh and H. J. Hoenders, Biochim. Biophys. Acta, 1992, 1120, 201–204 CrossRef CAS
. - S. B. Golegaonkar, H. S. Bhonsle, R. Boppana and M. J. Kulkarni, Eur. J. Mass Spectrom., 2010, 16, 221–226 CrossRef CAS PubMed
. - S. K. Kesavan, S. Bhat, S. B. Golegaonkar, M. G. Jagadeeshaprasad, A. B. Deshmukh, H. S. Patil, S. D. Bhosale, M. L. Shaikh, H. V. Thulasiram, R. Boppana and M. J. Kulkarni, Sci. Rep., 2013, 3, 2941 Search PubMed
. - M. E. William, Curr. Diabetes Rep., 2004, 4, 441–446 CrossRef PubMed
. - S. B. Bansode, A. K. Jana, K. B. Batkulwar, S. D. Warkad, R. S. Joshi, N. Sengupta and M. J. Kulkarni, PLoS One, 2014, 9(8), e105196 Search PubMed
. - M. M. Joglekar, S. N. Panaskar, A. D. Chougale, M. J. Kulkarni and A. U. Arvindekar, Mol. Biosyst., 2013, 9(10), 2463–2472 RSC
. - K. Nomoto, M. Yagi, U. Hamada, J. Naito and Y. Yonei, J. Anti-Aging Med., 2013, 10(5), 92–100 Search PubMed
. - L. Séro, L. Sanguinet, P. Blanchard, B. T. Dang, S. Morel, P. Richomme, D. Séraphin and S. Derbré, Molecules, 2013, 18, 14320–14339 CrossRef PubMed
. - E. Herczenik and M. F. B. G. Gebbink, FASEB J., 2008, 22(7), 2115–2133 CrossRef CAS PubMed
. - M. Zhang, W. Xu and Y. Deng, Diabetes, 2013, 62(11), 3936–3942 CrossRef CAS PubMed
. - A. Lapolla, D. Fedele, R. Reitano, N. C. Aricò, R. Seraglia, P. Traldi, E. Marotta and R. Tonani, J. Am. Soc. Mass Spectrom., 2004, 15(4), 496–509 CrossRef CAS PubMed
. - A. Ardestani and R. Yazdanparast, Int. J. Biol. Macromol., 2007, 41, 572–578 CrossRef CAS PubMed
. - Y. Konishi and A. Mullick, US Pat., 12, 293 339, 2007
. - U. Kanska and J. Boratyński, Arch. Immunol. Ther. Exp., 2002, 50, 61–66 CAS
. - N. Sreerama and R. W. Woody, Anal. Biochem., 2000, 287, 252–260 CrossRef CAS PubMed
. - H. S. Bhonsle, A. M. Korwar, S. K. Kesavan, S. D. Bhosale, S. B. Bansode and M. J. Kulkarni, Eur. J. Mass Spectrom., 2012, 18(6), 475–481 CrossRef CAS
.
Footnote |
† Electronic supplementary information (ESI) available. See DOI: 10.1039/c5ra01723h |
|
This journal is © The Royal Society of Chemistry 2015 |