DOI:
10.1039/C5RA01720C
(Paper)
RSC Adv., 2015,
5, 27387-27392
Recognition of fluoride anions at low ppm level inside living cells and from fluorosis affected tooth and saliva samples†
Received
28th January 2015
, Accepted 23rd February 2015
First published on 10th March 2015
Abstract
A simple Schiff base chemosensor 2-((2-(2,4-dinitro phenyl)hydrazono)methyl)-4-nitrophenol (L) has been developed as a colorimetric and fluorimetric ‘turn on’ sensor for fluoride (F−). F− recognition at ppm levels from mouth rinses and a toothpaste water solution has been successful. Significantly, L can detect F− from fluorosis affected tooth and saliva samples by similar colorimetric changes. A test kit for F− detection from a DMSO–water (1
:
1) mixture is also engineered. Intracellular F− from pollen grains of Techoma stans and Candida albicans (a diploid fungus), grown in 10−6 (M) F− contaminated water has been successfully detected under a fluorescence microscope.
Introduction
Fluoride is one of the most biologically important anions which has a significant role in dental care and osteoporosis.1 High F− intake can cause serious effects on skeletal tissues; skeletal fluorosis may be noticed when F− content in drinking water reaches 3–6 mg L−1. In 2006 an investigation by the United States National Research Council supported the fact that in India and China a risk to bone structure and skeletal fluorosis occurs at a total intake of 14 mg F− per day.2 Therefore it is necessary to find a cheap and easy detection method for quick and accurate diagnosis of fluorosis concerning its immense importance for the well-being of mankind. 20 states in India and 43 blocks of seven districts of West Bengal (WB) are identified as endemic for fluorosis and almost 66 million common people are at high risk in these regions from F− contamination.3
In this situation it is very much essential for researchers to tailor made design and to synthesize organic chemosensors which can exemplify the specific anion detection capability and at the same time also have the potential to alter the detection result into a readable signal. For detection of bio relevant anions, colorimetric and fluorimetric technique has attracted considerable interest among other available methodologies because of several advantages like sensitivity, selecting capability, quick response and cost effectiveness.4
Electronegative F− preferably forms strong hydrogen bonding interaction with neighboring hydrogen of immediate surroundings. In this regard, it is highly demanding to make a cost effective and easy to make organo-functional materials having selective sensing of F−. Till date very less is explored regarding the utility of functionalized Schiff base organic chemo sensors with specific anion selectivity. Therefore attempts are made for fabrication of a Schiff base type compound having strong hydrogen bond donor sites e.g.; –OH and –NH groups with presence of active chromophore and electron withdrawing group like –NO2 (ref. 5) to increase the acidity of –OH and –NH protons for better sensitivity towards incoming guest like F− at low ppm level. As a part of our ongoing research in supramolecular chemistry,1d,6 in this communication we are discussing the sensor molecule L, which have shown rapid change in color with tetrabutyl ammonium fluoride (TBA+F−) and sodium fluoride (Na+F−) in dimethyl sulfoxide (DMSO) whereas no interactions (within the same time limit, as for F− anion; i.e., few seconds) were observed with other TBA salts of anions Cl−, Br−, I−, HPO42−, SO42−, C2O42−, SCN−, HSO4−, ClO4−, PF6−, BF4−, N3−, NO2−, NO3−. It is also noteworthy to mention, the chemosensor L can detect fluoride by visual color change from fluorosis affected tooth and saliva samples. In addition, L could detect F− from commercially available mouth rinser and toothpaste water solution (F− level: ∼1 ppm); depicting its capability in detection of F− from water solution. We have also prepared an economic cheap test kit for F− detection from DMSO–water (1
:
1) mixture towards exploring its practical utility too. Nevertheless, it also has the ability to detect F− in vitro from pollen grains of Techoma stans and Candida albicans (a diploid fungus) incubated in 10−6 (M) F− infected water solution.
Results and discussion
Synthesis and characterization
L is synthesized following a simple condensation of less expensive precursors, i.e.; 2-hydroxy-5-nitrobenzaldehyde (167 mg, 1 mmol) and 2,4-dinitro phenyl hydrazine (198 mg, 1 mmol) (Fig. 1 and see ESI,† Scheme S1). Its formation is confirmed by ESI-MS, single crystal X-ray, 1H-NMR and FT-IR like sophisticated analytical tools (see ESI,† Fig. S1 and S5–S8; crystallographic details are tabulated in Table S1–S3†). The ORTEP with atom numbering schemes are shown in Fig. 1b.
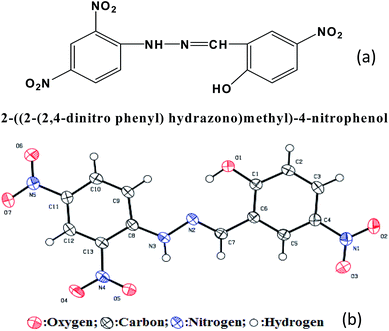 |
| Fig. 1 (a) Schematic representation of L, (b) ORTEP with atom numbers for L. | |
Visual Colorimetric fluoride detection from TBAF and NaF
In case of visual color change study, an intense and sharp color change from yellow to reddish brown is noticed in DMSO after addition of TBA+F− or Na+F to the solution of L (1.0 × 10−4 M). Interestingly, addition of other anions did not show any detectable color change (Fig. 2) except OAc−, where a feeble color change is noticed (vide infra, detailed proposition regarding plausible sensing mechanistic path is discussed). In this context it is noteworthy, to verify any interference with variation of pH on sensor L; pH metric sensitivity studies of L in pH 6.00 to 8.00 are performed. The pH responsive experimentation is repeated thrice and is concluded no such interference is observed due to variation of pH in the desired pH range over sensor L (see ESI,† Fig. S2).
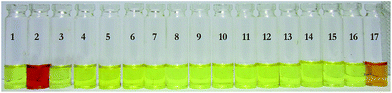 |
| Fig. 2 Visual Colorimetric response of L with different anions ((1) L(blank), (2) F−, (3) Cl−, (4) Br−, (5) I−, (6) HPO42−, (7) SO42−, (8) C2O42−, (9) SCN−, (10) HSO4−, (11) ClO4−, (12) PF6−, (13) BF4−, (14) N3−, (15) NO2−, (16) NO3−, (17) OAc−). | |
Visual Colorimetric fluoride detection from toothpaste and mouth rinser
Visualising the selectivity of L towards F− over other anions, we are motivated to check its capability in detecting F− from commercially available mouth rinser (225 ppm in 250 mL) and toothpaste (1000 ppm in 100 g), where F− content is high and recent reports describe that use of high F− content toothpaste can cause fluorosis.7a L (1.0 × 10−4 M) can successfully detect F− from mouth rinser and toothpaste by changing color from yellow to reddish brown (Fig. 3). We have directly used mouth rinser and in case of toothpaste a 10 mL 10−6 (M) water solution was prepared to conduct F− detection.
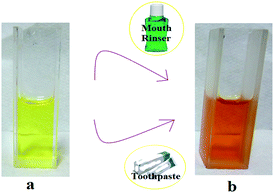 |
| Fig. 3 Detection of F−, (a) before and (b) after exposure to commercially available mouth rinser and toothpaste water solution. | |
By this experimentation we are getting confirmation regarding the capability of L in detection of F− from water solution at ppm level; in fact ppm level F− sensor is scanty in literature8 and in most of the cases the reported sensor molecule was prepared following a multiple steps synthetic route,8 but in this report the sensor molecule L can be synthesized using easily available materials and can be produced by simple Schiff base condensation technique with high yield (ca. 90%).
Visual Colorimetric fluoride detection from fluorosis affected tooth and saliva specimens
From health and development concern of rural Indians it is highly recommendable to detect F− from fluorosis affected tooth as well as saliva samples for easy diagnosis of dental fluorosis in rural and remote part of India as 20 states in India and seven districts of WB state are suffering from fluorosis. This disease can happen in different ways e.g.; dental, skeletal and non-skeletal. Dental fluorosis may affect the children's (after 8 years of age) teeth.3 Skeletal fluorosis used to happens in the major joints and bones of human body.3 Where as non-skeletal fluorosis affects invariably any organs, soft tissues of human being.3
L has successfully detected F− from fluorosis affected tooth sample with changing color within 5 minutes from yellow to reddish brown in DMSO. We strongly believe that this interesting outcome must be helpful for diagnosis of dental fluorosis (Fig. 4). It is noteworthy to mention that L remains silent with non fluorosis tooth sample even after hours of exposure (Fig. 4).
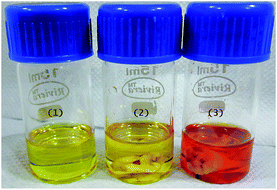 |
| Fig. 4 Colorimetric detection of F− from fluorosis affected tooth sample: (1)1 × 10−4 M DMSO solution of L; (2) L remains silent with non fluorosis tooth sample; (3) after dipping the fluorosis affected tooth sample into the DMSO solution of L for 5 minutes. | |
As dental fluorosis can cause enhancement of F− concentration in saliva, therefore the obvious target must be the detection of F− from human saliva. A prima facie survey report is hereby depicted; 30 dental patients from different parts of rural places of district Burdwan of West Bengal, India were examined (see ESI† Section 4 for details about sampling process). Five of them were suffering from dental fluorosis (clinically diagnosed). Those patients spit on a clean, sterilized and dry petridish and L (0.5 mL of 10−4 M concentration) was poured on the collected saliva into the Petridish. It was observed that for every case of fluorosis affected patients, the color of their saliva and the detector L solution mixture was instantaneously changed from yellow to intense reddish brown. Among the others, two individual samples where fluorosis was not clinically diagnosed, still shows a very weak color change after standing for a prolong time period, is perhaps due to the presence of F− in saliva, possibly coming from use of high F− content (∼1000 ppm) toothpastes.7a For rest of the samples where patients are already clinically diagnosed as non-fluorosis patient, reflects no visible colour changes on adding test detector solution L. In this perspective, it may be noted that the human salivary analysis has established acetate anion as one of the components of saliva. L can detect the acetate anion in DMSO medium, but it fails to detect acetate either in water or in DMSO–water (1
:
1) mixture. Interestingly, F− can easily be detected both in water or DMSO–water (1
:
1) mixture by L. In saliva, it is quite obvious that its components are residing in water medium. So whatever colour changes are coming using sensor L are actually coming only due to fluoride; noway it is related to acetate anion detection. This is the only reason in support of distinct color change in saliva of fluorosis affected patients and high F− content toothpaste user's. Concerning other patients (saliva specimens) shows no such color change with L. In addition, from UV-Vis study it is also observed that binding equilibrium constant of acetate with L is ∼102 M−1 which is appreciably less than the binding equilibrium constant of F− with L (∼104 M−1, vide infra). Moreover the mode of collection of saliva specimens for proper sampling process (see ESI† for details about sampling process) also instigates to minimize the presence of acetate anion in clinical specimens and also keeps systemic F− undisturbed via ductile salivary secretions and cariostatic mechanism.7b
UV-Vis titration experiment of L with fluoride
These interesting results of L as chemosensor enthralled us to investigate the reason lying behind the chemo sensing of F− by L. We have carried out several spectroscopic studies for insightful discussions of L and F− binding. In case of UV-Vis study in DMSO, absorption band of L (1 × 10−4 M) is appearing at 285, 390 (sh) and 425 nm. First absorption at 285 nm can be assigned as π → π* transition of the aromatic rings, whereas second response at 390 nm is due to π → π* transition involving the π electrons of the azomethine group.9 425 nm peak can be assigned as n → π* transition of azomethine group and ICT (intramolecular charge transfer)9 transition inside the whole scaffold (Fig. 5). After addition of F− the peak at 285 nm is blue shifted to 265 nm with an appreciable change in absorption intensity, whereas 390 nm (sh) peak is reduced to some extent. 425 nm peak is red shifted to 435 nm. Another absorption band at 495 nm is appeared which divulge the construction of a sensor–anion (host–guest) complexation. The interaction of F− with L have influences on the electronic distribution of chromophore, which results in an appreciable color change with a new intraligand or internal charge transfer band.9 Distinct isosbestic point at 407 nm (Fig. 5) also supports the complexation of L with F−. Sensing of acetate anion (upto 2 equivalents) in DMSO medium is also carried out and corresponding weak changes in UV-Vis spectrum is shown in Fig. S3a.† Higher equivalents addition (even upto 5 equivalents) of acetate anion shows no further distinguish changes in UV-Vis spectrum. To establish L as a universal sensor, we have simply carried out the interference study for F− with other tested anions. In DMSO medium L can detect F− in presence of other anions (Cl−, Br−, I−, HPO42−, SO42−, C2O42−, SCN−, HSO4−, ClO4−, PF6−, BF4−, N3−, NO2−, NO3− and OAc−) (see ESI,† Fig. S3c). A feeble interference from OAc− is observed in DMSO. It is noteworthy that, the application of this sensor is mainly related to detection of fluoride from human saliva (water based medium). Interestingly, when we have chosen the solvent medium as DMSO–water (1
:
1) no interference was observed eventually from OAc−. As a consequence L now become a selective sensor towards F− (see ESI,† Fig. S3d). Association equilibrium constant of L with F− is in the order of 104 M−1 (at 298 K) determined by Benesi Hildebrand10a and by method originally taken from Connors10b (see ESI,† Fig. S4). In both cases the correlation coefficient (R > 0.99) established the formation of 1
:
1 complexation between L and F−.10c
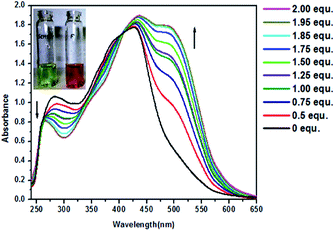 |
| Fig. 5 UV-Vis titration of L (1 × 10−4 M) with TBA+F− (1 × 10−4 M) in DMSO (0–2 equivalent). | |
Fluorescence titration experiment of L with fluoride
The fluorescence of L in DMSO–water (9
:
1, v/v, HEPES buffer, pH 7.4) (1 × 10−5 M) is appearing around 530 nm upon excitation at 425 nm. Addition of TBA+F− (up to 2 equivalent, vide Fig. 6) results in concomitant increase of the band around 530 nm and the color of the solution also changes from yellow to reddish brown (Fig. 6 inset). For other anions no distinct changes are found. Inhibition of photoinduced electron transfer (PET) and rigidness due to binding of F− with L might be the two important causes for fluorescence enhancement. Before binding with F− the imine nitrogen of L could form an intramolecular hydrogen bond with adjacent phenolic hydrogen (distance between imine nitrogen and phenolic hydrogen is ∼1.8 Å), which results in PET and de-excitation of the tautomer via nonradiative pathway. Thus weak fluorescence of L is observed. Binding of F− in turn facilitates the deprotonation of OH group and inhibits the PET which results in an enhancement in fluorescence. The free sensor L has a flexible configuration and could rotate easily. However, after binding with F− the L becomes rigid and thus inhibits the vibrational and rotational relaxation modes of nonradiative decay.9,11 Therefore ‘turn on’ fluorescence is observed.
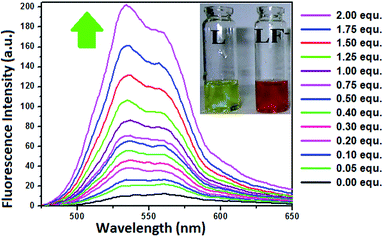 |
| Fig. 6 Fluorescence titration spectra of L (1 × 10−5 M) with F− (1 × 10−4 M) (0–2 equivalent) in DMSO–water (9 : 1, v/v, HEPES buffer, pH 7.4). | |
1H-NMR titration experiment of L with fluoride
In order to investigate the binding properties of L with F− we have performed 1H-NMR titration in DMSO-d6. The –OH and –NH proton responses are coming at ∼11.84 ppm and the other proton responses are coming at expected region (see ESI,† Fig. S5–S6). The chemical shift in 1H-NMR titration experiment with stepwise addition of TBA+F− is shown in Fig. 7. With gradual addition of guest F−, acidic protons of –OH and –NH groups respond first. The disappearance of –OH signal suggest deprotonation1c,12 with featured peak of HF2− at ∼16.1 ppm (Fig. 7 inset). With increasing level of F− addition, electron density is slowly rising in the imine side of L due to through bond electronic propagation12a and an upfield shift10c (from ∼9 ppm to ∼8.85 ppm) for the proton of –CH
N– is observed. In addition to through bond propagation, the deprotonation of L creates a considerable charge density throughout the skeleton, results in an upfield shift for all skeletal protons.10c
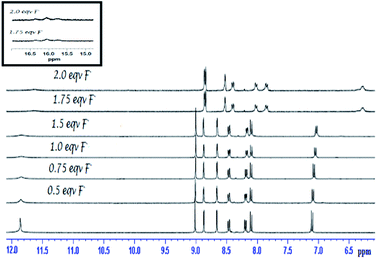 |
| Fig. 7 1H-NMR (400 MHz) titration of L with TBA+F− (0–2 equivalent) [inset shows the zoomed view of triplet HF2− at ∼16.1 ppm]. | |
Although we have executed the experiment in presence of TBA+F−, however in real life aspect, Na+F− should be considered as main sources of F−. Therefore, concerning the utility of sensor L, detection of F− coming from Na+F− is very much essential and sounds practical. In doing so, we have titrated L with Na+F− solution in DMSO-d6. The similar trend in 1H-NMR chemical shifts for protons are also observed in this case (Fig. 8). Chemical Shifts of L remains unchanged for several other tested anions. This prediction about the binding of F− with L is finally bolstered by ESI-MS and FT-IR study (see ESI,† Fig. S7–S8). A peak at m/z ∼366 confirms the complexation of L with F− (see ESI,† Fig. S7). Non hydrogen bonded –OH stretching at ∼3300 cm−1 is broadened due to weak interaction with incoming guest F− and swing to ∼2960 cm−1. Similar is also true for corresponding –NH stretching, coming at ∼3090 cm−1 and shifted to ∼2870 cm−1 after F−⋯sensor adduct formation.13a In case of imine –CH unit, after host⋯guest complexation the electron density is rearranged13b and eventually influences the –C
N– linkage. This is reflected from shifting in characteristic stretching at ∼1610 cm−1 to ∼1617 cm−1 (see ESI,† Fig. S8). The swing in stretching frequencies due to electronic redistribution in FT-IR study is in well accordance with 1H-NMR study.
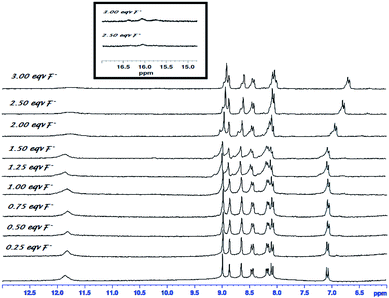 |
| Fig. 8 1H-NMR (300 MHz) titration of L with Na+F− (0–3 equivalent) [inset shows the zoomed view of triplet HF2− at ∼16.1 ppm]. | |
Insight on fluoride sensing mechanism
After careful and thorough investigation, we firmly suggest that initially an equilibrium is established through the formation of a hydrogen bonded complex (no chemical shifts in 1H-NMR proton position other than –NH and –OH signals observed upto ∼1.5 equivalent of F− addition in sensor L) (Fig. 9a).10c,12a As L is a receptor having acidic protons, therefore addition (>1.5 equivalent) of basic F− anion will cause deprotonation leading to an acid base reaction (Fig. 9b). The similar colorimetric change and UV-Vis titration spectra are also observed for L with TBAOH (see ESI,† Fig. S3b), which further strengthen our inference about the base induced deprotonation of sensor L. In fact, it is also observed from 1H-NMR titration that addition of ∼1.75 equivalents of F− results in deprotonation of L with formation of (L)− along with HF2− (Fig. 7). Herein an acid base reaction is going on in between host (L) and guest (F−).12a On the other way, for OAc− the weak colorimetric responses in DMSO medium is perhaps due to weak hydrogen bonding interaction between sensor L and guest OAc− (see ESI,† Scheme S2).14
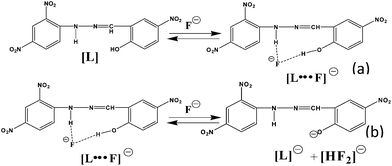 |
| Fig. 9 Proposed mechanistic pathway for L and F− complexation (weak bonds are shown in dotted black line). | |
Application in practical life
Test kit preparation
We are interested to explore an another direct application for mankind of chemosensor L, an easy to handle and economic medicinal kit for detection of F− for rural part of India is prepared (Fig. 10). This could detect F− from experimental DMSO–water (1
:
1) solution with visible color change (see ESI,† for details about the preparation of test kit).
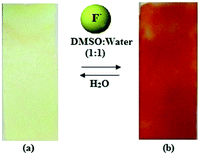 |
| Fig. 10 Test kit (a) L coated test paper (b) F− solution sprayed on L coated test paper. | |
Bio-medical relevance
As non skeletal fluorosis affects all the soft tissues and organs of the body, therefore in vitro F− detection is necessary for quick and easy identification of non skeletal fluorosis. Interestingly, L can easily detect intracellular F− at ppm level; which is confirmed with two different types of cells viz. Candida albicans (IMTECH no. 3018) (Fig. 11a and b) and pollen grains of Techoma stans (Fig. 11c and d). L can easily permeate through the cell membrane without causing any harm to the cells (see ESI† for the details about preparation of cells and procedure of cell imaging). Noticeably, blue-green emission15 was observed in cells grown in F− contaminated (ppm level) water upon addition of L at desired concentration.
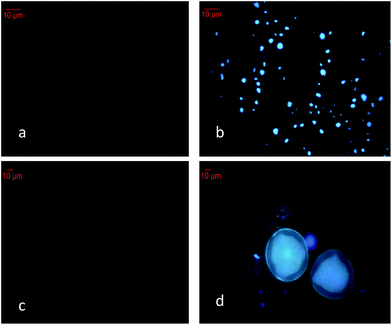 |
| Fig. 11 In vitro detection of F− in ppm level concentration. Candida albicans cells (IMTECH no. 3018): (a) fluorescence image in absence of L and (b) L stained cells incubated in F− (10 μM) for 1 h under 100× objective lens. Pollen grains: (c) fluorescence image in absence of L and (d) L stained cells incubated in F− (10 μM) for 1 h under 100× objective lens. Incubation was performed at 37 °C. | |
The photographs indicate that L can be used to detect the presence of intracellular F− in samples (living cells such as bacteria, fungi, protozoa etc.).
Conclusion
In conclusion we have described herein a rational strategy for fabricating a simple Schiff base organic chemo sensor. Host–guest type complexation with fluoride is occurred and confirmed via several sophisticated analytical techniques. Significantly, this newly developed chemosensor L detects fluoride from fluorosis affected tooth and saliva samples. Additionally L can detect fluoride from commercially available mouth rinser and toothpaste water solution where fluoride content is ∼1 ppm mL−1. An easy to operate test kit for tracing F− even in lower concentration is also developed in sake of helping and also for diagnosis of fluorosis affected people in remote and rural part of India. Constant search on further development of several other small organic chemo sensor molecules towards bio essential anions is under active progress.
Acknowledgements
Supra institutional research grant (ESC-0203/09) under CSIR-XIIth five year plan is hereby acknowledged for financial support. P.B. is extremely thankful to Dr Prosenjit Bhakat, ex-house surgeon, Dept. of Dentistry, BMCH, Govt. of WB and Ms Tina Dey for their enormous support and value added discussions. We are grateful to the Sophisticated Instrument Centre (SIC) Single Crystal X-Ray Diffraction facility, IIT Indore. PG is thankful to DST (GAP-183112) for his fellowship.
Notes and references
-
(a) P. D. Beer and P. A. Gale, Angew. Chem., Int. Ed., 2001, 40, 486 CrossRef CAS
;
(b) S. Y. Kim and J.-I. Hong, Org. Lett., 2007, 9, 3094 Search PubMed
;
(c) D. Esteban-Gómez, L. Fabbrizzi and M. Licchelli, J. Org. Chem., 2005, 70, 5717 CrossRef PubMed
;
(d) A. Roychowdhury, P. Ghosh, S. K. Saha, P. Mitra and P. Banerjee, Spectrochim. Acta, Part A, 2014, 124, 492 CrossRef CAS PubMed
;
(e) C. Suksai and T. Tuntulani, Chem. Soc. Rev., 2003, 32, 192 RSC
. - WHO, Guidelines for Drinking-Water Quality, World HealthOrganization, Geneva, Switzerland, 4th edn, 2011 Search PubMed
. - K. K. Majumdar, Indian J. Public Health, 2011, 55, 303 CrossRef PubMed
. - B. Dey, R. Saha and P. Mukherjee, Chem. Commun., 2013, 49, 7064 RSC
. - X.-F. Shang, Spectrochim. Acta, Part A, 2009, 72, 1117 CrossRef PubMed
. -
(a) P. Ghosh, A. Roychowdhury, M. Corbella, A. Bhaumik, P. Mitra, S. M. Mobin, A. Mukherjee, S. Basu and P. Banerjee, Dalton Trans., 2014, 13500 RSC
;
(b) P. Banerjee, A. D. Jana, G. Mostafa and S. Goswami, Eur. J. Inorg. Chem., 2008, 44 CrossRef CAS
;
(c) P. Banerjee, S. Kar, A. Bhaumik, G.-H. Lee, S.-M. Peng and S. Goswami, Eur. J. Inorg. Chem., 2007, 835 CrossRef CAS
;
(d) S. Das, P. Banerjee, S.-M. Peng, G.-H. Lee, J. Kim and S. Goswami, Inorg. Chem., 2006, 45, 562 CrossRef CAS PubMed
;
(e) P. Ghosh, A. R. Chowdhury, S. K. Saha, M. Ghosh, M. Pal, N. C. Murmu and P. Banerjee, Inorg. Chim. Acta, 2015, 429, 99 CrossRef CAS
. -
(a) S. Jagtap, M. K. Yenkie, N. Labhsetwar and S. Rayalu, Chem.Rev., 2012, 112, 2454 CrossRef CAS PubMed
;
(b) P. Gron, H. G. McCann and F. Brudevold, Arch. Oral Biol., 1968, 13, 619 CrossRef
. -
(a) M. A. Palacios, R. Nishiyabu, M. Marquez and P. Anzenbacher Jr, J. Am. Chem. Soc., 2007, 129, 7538 CrossRef CAS PubMed
;
(b) Y. Kim and F. P. Gabbaï, J. Am. Chem. Soc., 2009, 131, 3363 CrossRef CAS PubMed
;
(c) I.-S. Ke, M. Myahkostupov, F. N. Castellano and F. P. Gabbaï, J. Am. Chem. Soc., 2012, 134, 15309 CrossRef CAS PubMed
;
(d) H. Khanmohammadi and K. Rezaeian, RSC Adv., 2014, 4, 1032 RSC
;
(e) T. Nishimura, S.-Y. Xu, Y.-B. Jiang, J. S. Fossey, K. Sakurai, S. D. Bull and T. D. James, Chem. Commun., 2013, 49, 478 RSC
. - R. Arabahmadi, M. Orojloo and S. Amani, Anal. Methods, 2014, 6, 7384 RSC
and references therein. -
(a) H. A. Benesi and J. H. Hildebrand, J. Am. Chem. Soc., 1949, 71, 2703 CrossRef CAS
;
(b) J. Xiong, L. Sun, Y. Liao, G.-N. Li, J.-L. Zuo and X.-Z. You, Tetrahedron Lett., 2011, 52, 6157 CrossRef CAS
and references therein;
(c) X. Bao, J. Yu and Y. Zhou, Sens. Actuators, B, 2009, 140, 467 CrossRef CAS
. - Z.-h. Lin, Y.-g. Zhao, C.-y. Duan, B.-g. Zhang and Z.-p. Bai, Dalton Trans., 2006, 3678 RSC
. -
(a) M. Boiocchi, L. Del Boca, D. Esteban-Gómez, L. Fabbrizzi, M. Licchelli and E. Monzani, Chem.–Eur. J., 2005, 11, 3097 CrossRef CAS PubMed
;
(b) V. Amendola, L. Fabbrizzi, L. Mosca and F.-P. Schmidtchen, Chem.–Eur. J., 2011, 17, 5972 CrossRef CAS PubMed
;
(c) J. V. Ros-Lis, R. Martínez-Máñez, F. Sancenón, J. Soto, K. Rurack and H. Weißhoff, Eur. J. Org. Chem., 2007, 2449 CrossRef CAS
;
(d) V. Amendola, M. Boiocchi, L. Fabbrizzi and A. Palchetti, Chem.–Eur. J., 2005, 11, 5648 CrossRef CAS PubMed
;
(e) D. Esteban-Gómez, L. Fabbrizzi, M. Licchelli and D. Sacchi, J. Mater. Chem., 2005, 15, 2670 RSC
;
(f) A. M. Costero, M. J. Bañuls, M. J. Aurell, M. D. Ward and S. Argent, Tetrahedron, 2004, 60, 9471 CrossRef CAS
. -
(a) D. Saravanakumar, S. Devaraj, S. Iyyampillai, K. Mohandoss and M. Kandaswamy, Tetrahedron Lett., 2008, 49, 127 CrossRef CAS
;
(b) J. Joseph and E. D. Jemmis, J. Am. Chem. Soc., 2007, 129, 4620 CrossRef CAS PubMed
. - D. Sharma, S. K. Sahoo, S. Chaudhary, R. K. Bera and J. F. Callan, Analyst, 2013, 138, 3646–3650 RSC
. - V. V. Roshchina, Fluorescing World of Plant Secreting Cells, Science, Enfield, UK, 2008 Search PubMed
.
Footnote |
† Electronic supplementary information (ESI) available: Crystallographic details, UV-Vis, ESI-MS, 1H-NMR and FT-IR of L are provided. CCDC 1012073. For ESI and crystallographic data in CIF or other electronic format see DOI: 10.1039/c5ra01720c |
|
This journal is © The Royal Society of Chemistry 2015 |
Click here to see how this site uses Cookies. View our privacy policy here.