DOI:
10.1039/C5RA00873E
(Paper)
RSC Adv., 2015,
5, 26596-26603
Synthesis, characterization and nonlinear optical studies of novel blue-light emitting room temperature truxene discotic liquid crystals
Received
15th January 2015
, Accepted 6th March 2015
First published on 6th March 2015
Abstract
A new series of discotic liquid crystals based on a truxene core has been synthesized to study the structure–property relationship in view of the self-assembling property and their linear and nonlinear optical properties. All these branched alkyl chain truxene derivatives show a mesogenic property in a columnar hexagonal fashion at room temperature which is studied by a combination of different techniques. The newly synthesized truxene discotic 7a possesses a clearing temperature of 117 °C, one of the lowest clearing temperatures known in truxene discotic liquid crystals. We have discovered that the introduction of branching near to the core even in a small alkyl chain drastically reduces the isotropic temperature. Due to their C3 symmetry and their large first hyperpolarizability, these truxene derivatives show long-lived emissions in the solution state at room temperature. We also report large effective three-photon absorption in these materials under nanosecond laser pulse excitation at 532 nm, which makes them suitable candidates for optical limiting applications.
1. Introduction
Supramolecular structures formed due to non-covalent interactions in functionalized disc-shaped molecules, commonly referred to as discotic liquid crystals (DLCs), are of enormous scientific interest because of their extraordinary unidirectional charge and energy migration properties along the columnar axis as well as their optical properties. As these systems are of great fundamental and technological importance, significant research work is going on around the globe which has been reviewed in several articles, for example see ref. 1–19.
The majority of discotic liquid crystals are derived from polycyclic aromatic cores such as, triphenylene, anthraquinone, phthalocyanine, and hexabenzocoronenes etc., which possess strong π–π interactions favouring columnar stacking of the molecules. In the columnar mesophase of DLCs, aromatic cores are oriented in columns separated by molten aliphatic hydrocarbon chains. The intra-columnar (core–core) separation in a columnar mesophase is usually of the order of 0.35 nm while the intercolumnar (neighbouring columns) separation is generally in the range of 2–4 nm, depending on the length of the flexible chains. Thus, these materials function as quasi-one-dimensional semiconductors.1–19
In 1981, Destrade and co-workers first studied the truxene in discotic liquid crystals by preparing the hexaesters of truxene showing inverted nematic-columnar phase sequence.20 So far limited truxene derivetives are reported as DLCs because of synthetic difficulties. Truxene based compounds are attractive for many applications such as optoelectronics, photovoltaic, polymers, dyes, semiconductors, polymeric light emitting devices etc.21–30 because of many reasons: it is electron rich system, possess C3 symmetrical structure, its good florescence capacity, its ability to act as potential semiconductors, its derivatives are coloured, its extraordinary thermal stability and its electronic properties.
Liquid crystals possess large and versatile optical nonlinearities suitable for photonic applications spanning the femtoseconds to milliseconds time scales, and across a wide spectral window.31 The nonlinear optical properties of liquid crystals (LCs) have been studied for more than 20 years starting from the paper by Zeldovich et al.32 who reported the first observation of a Giant Optical Nonlinearity (GON). The presence of extended π electron conjugation makes discotic liquid crystals highly polarizable, which makes them potential candidates for nonlinear optical applications. This is due to the particular spatial arrangement of the molecules in space and their characteristics.33 Large two photon absorption (2 PA) and three photon absorption (3 PA) coefficients can be obtained by optimal design and synthesis of chromophores and dendrimers.33 The molecules with large differences in polarization upon excitation and extended π-conjugation have received much attention in DLCs ultrafast optical responses, which depends upon excitation wavelength. The electronic structure of these materials usually supports strong nonlinear absorption (NLA) in the visible as well as IR regions. During the last two decades a number of studies like conducting, optical, non-linear optical and electroluminescent properties34–40 have been reported on different truxene derivatives.
Though organic materials are well known candidates for non-linear optical applications, only a few studies have been reported on the preparation and NLO characterization of truxene derivatives.41,42 Liquid crystals exhibit high nonlinear optical responses due to the fact that they are strongly anisotropic and their molecular reorientation is highly correlated. Their refractive index changes drastically due to collective director reorientation under an external field. As such, their extraordinarily large nonlinear optical responses can be observed even under a relatively weak optical field from a low power laser. The nonlinear optical response of thermotropic liquid crystals has been extensively studied.33,43,44 Because of the extreme sensitivity of LCs to the action of external fields, a powerful light wave changes the orientation of the molecules. Light-induced structural changes are reflected in all of the macroscopic properties of LC and are easily observed both in the mesophase and in the isotropic phase.45–48
To explore the potential applications of these intriguing materials, DLCs having mesophase at room temperature and mesophase stability over a wide temperature range are desired. Further, LCs with not very high clearing temperature are required for various physical studies. Here we wish to report synthesis and characterisation of a series of new discotic materials based on hexaalkoxytruxene derivatives with branched alkyl chains at periphery which exhibit mesophase at ambient temperature and also possess low isotropic temperature compared with reported truxene DLCs. The newly synthesized materials were characterized from their spectroscopic, optical, thermal, self-assembling behaviour and X-ray diffraction studies. Our intention is to tune the linear and non-linear optical properties using Z-scan technique of truxene-based liquid crystals by the introducing alkyl chain at periphery. These modified truxene derivatives may be used as non-linear optical materials and applicable in semiconductor industry.
2. Experimental
2.1 Materials
All the chemicals and reagents were purchased locally and used as such without any further purification. Acme make silica gel of mesh size 200–400 were used to perform the column chromatographic purification.
2.2 Characterization
The chemical structures were confirmed by Bruker AMX 500 MHz 1H NMR spectrometer and Shimadzu FTIR-8400 FTIR spectrometer, respectively. Absorption and fluorescence spectra were recorded on a Perkin Elmer UV-Vis-lambda 35 double beam-spectrophotometer and Perkin Elmer Fluormax spectrophotometer, respectively. Elemental analysis was performed on a Carlo-Erba Flash 1112 analyzer. Optical textures of mesophases were observed using Olympus BX51 Polarized Optical Microscope (POM) in conjunction with a Mettler FP82HT hot stage and FP90 central processor. Differential scanning calorimetry (DSC) of liquid crystals and Composites were taken at the temperature range of 5 °C to 150 °C using Parkin-Elmer Pyris-1 DSC. X-Ray powder diffraction (XRD) patterns were obtained on DY 1042-Empyrean XRD with Pixel 3D detector at Cu-Kα radiation.
2.3 Synthesis
The synthesis is illustrated in Scheme 1. The synthetic work was commenced from acid treated aldol cylotrimerization of 5,6-dialkoxy-1-indanone using p-toluenesulfonic acid as dehydrating agent in mixed solvent toluene and ethylene glycol.49 All required branched chains alkyl bromides were prepared according to previously reported literature.50,51
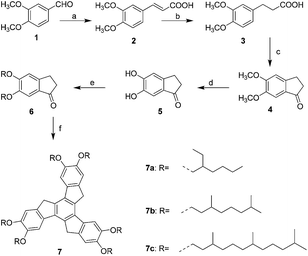 |
| Scheme 1 Synthesis of hexaalkoxytruxenes 7a, 7b, 7c; (a) CH2(COOH)2, pyridine, 80 °C, 16 h; (b) 10% Pd/C, H2 gas, CH3COOEt, 60 °C, overnight; (c) P2O5, 85% H3PO4, 110 °C, 6 h; (d) BBr3, CH2Cl2, −78 °C 4 h; (e) RBr, K2CO3, KI, EtOH, reflux, 12 h; (f) Ts-OH·H2O, (CH2OH)2, toluene, reflux, overnight. | |
3-(3,4-Dimethoxyphenyl) acrylic acid, (2). A mixture of 3,4-dimethoxybenzaldehyde (10 g, 60.0 mol), malonoic acid (10.01 g, 96.28 mol) and pyridine (11.9 g, 150 mol) was taken in a 250 mL round bottom flask, the mixture was heated to 80 °C with stirring for 16 hours. The crude white solid was then recrystallized using ethanol to give compound 2 as white crystals (yield 90%).1H-NMR (CDCl3, 500 MHz, ppm): δ 7.74 (d, J = 16.0 Hz, 1H), 7.14 (d, J = 8 Hz, 2H), 7.88 (d, J = 8 Hz, 1H), 6.32 (d, J = 16 Hz, 1H), 3.92 (s, 6H).
3-(3,4-Dimethoxyphenyl)propanoic acid, (3). A mixture of compound 2 (10 g) and 10% Pd/C (500 mg) was taken in 60 mL of ethylacetate in a round bottom flask were hydrogenated at 150 atmosphere of H2 gas for 14 hours at 60 °C. The reaction mixture was filtered to remove the catalyst then solvent was removed to give white solid. Solid product was then recrystallized in a mixture of petroleum ether and ethylacetate to yield compound 3 as white powder (yield 95%). 1H NMR (CDCl3, 500 MHz, ppm): δ 6.81–6.74 (m, 3H), 3.86 (s, 6H), 2.91 (t, J = 7.5 Hz, 2H), 2.67 (t, J = 7.5 Hz, 2H).
2,3-Dihydro-5,6-dimethoxyinden-1-one, (4). P2O5 (9 g) and 85% H3PO4 (3.5 g) were heated to 120 °C with stirring for about 1 and 1/2 hours. To this clear polyphosphoric acid, compound 3 (1 g) was added and continued the same reaction condition for 6 hours. Cooled reaction mixture was added slowly to the crushed ice and extracted with 3 × 100 mL chloroform; organic layer was concentrated under vacuum. The crude dark green solid was purified by column chromatography using dichloromethane as mobile phase to give pure indanone as yellow solid (yield 55%).1H NMR (CDCL3, 500 MHz, ppm): δ 7.27 (s, 1H), 6.90 (s, 1H), 3.97 (s, 3H), 3.91 (s, 3H), 3.06 (d, J = 5 Hz, 2H), 2.68 (d, J = 5 Hz, 2H).52
2,3-Dihydro-5,6-dihydroxyinden-1-one, (5). BBr3 (3.9 g, 15.6 mol) was added slowly to a stirred solution of compound 4 (1 g, 5.2 mol) in dichloromethane (30 mL) at −78 °C. After 4 hours mixture was then stirred at room temperature for 2 hours. Water was added to the reaction mixture to give red solid which was filtered and dried to afford titled compound 5 as dark red solid (yield, 80%). 1H NMR (CDCl3, 500 MHz, ppm): δ 10.0 (s, 1H), 9.42 (s, 1H), 6.92 (s, 1H), 6.83 (s, 1H), 2.89 (brs, 2H), 2.5 (brs, 2H).
2,3-Dihydro-5,6-dialkoxyinden-1-one, (6a, 6b and 6c). A mixture of compound 5 (1 g, 6.09 mol), K2CO3 (3.4 g, 24.39 mol), KI as a catalyst and RBr (18.3 mol) in ethanol was refluxed for overnight. After careful observation in TLC, reaction mass was filtered and mother liquor were concentrated. The crude product was purified in column chromatogram to yield pure thick yellow liquid (yield, 70%).1H NMR for 6a: (CDCl3, 500 MHz, ppm): δ 7.08 (s, 1H), 6.78 (s, 1H), 3.8 (d, 4H), 2.98 (brs, 2H), 2.59 (brs, 2H), 1.8–1.4 (m, 30H); 6b: δ 7.27 (s, 1H), 6.87 (s, 1H), 4.0 (m, 4H), 3.0 (brs, 2H), 2.66 (brs, 2H), 1.8–1.4 (m, 38H); 6c: δ 7.27 (s, 1H), 6.89 (s, 1H), 4.1 (m, 4H), 3.0 (brs, 2H), 2.68 (brs, 2H), 1.8–1.4 (m, 58H).
Hexaalkoxytruxene liquid crystals (7a, 7b and 7c). A well mixture of para-toluenesulfonic acid, ethylene glycol and compound in toluene was heated to reflux for overnight. Toluene was removed under high vacuum after careful confirmation of completion of reaction by thin-layer chromatography; resulted red solution was purified by column chromatography. The crude solid was purified by repeated recrystallization in diethylether and methanol to give target compound as gummy solid (yield, 55%). 1H NMR for 7a (CDCL3, 500 MHz, ppm): δ 7.49 (s, 3H), 7.27 (s, 3H), 4.23 (s, 6H), 4.0 (d, 12H), 1.89 (m, 6H), 1.4–0.98 (m, 84H), (elemental anal. C75H114O6, calculated C 81.03, H 10.34; found C 81.0, H 10.50%); 7b: δ 7.49 (s, 3H), 7.27 (s, 3H), 4.24 (m, 18H), 2.0 (m, 12H), 1.6–1.2 (m, 102H) (elemental anal. C87H138O6, calculated C 81.63, H 10.87; found C 81.22, H 10.91%); 7c: δ 7.47 (s, 3H), 7.27 (s, 3H), 4.17 (m, 18H), 1.97 (m, 12H), 1.6–1.1 (m, 162H) (elemental anal. C117H198O6, calculated C 82.62, H 11.73; found C 82.32, H 11.76%).
3. Results and discussion
3.1 Liquid crystalline and structural properties
The thermal behaviour of these newly synthesised compounds was studied by polarising optical microscopy (POM), differential scanning calorimetry (DSC) and X-ray diffraction studies. In discotic LCs, the use of branched alkyl chains to modify thermal properties has been well documented.53–55 We have previously used branched alkyl chains to reduce the melting and isotropic temperatures of discotic nematic and columnar phases.54,55
The polarised optical microscope textures reveals the typical columnar mesophase textures of discotic liquid crystalline materials. Textures obtained under POM for the compounds 7a, 7b and 7c are depicted in Fig. 1.
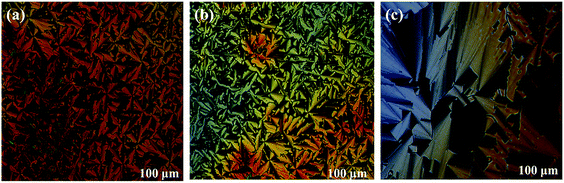 |
| Fig. 1 Polarizing optical microscopy (POM) images of truxene discogens (Colh) observed between two glass slides upon slow cooling (2 °C min−1) from isotropic phase at room temperature: pictures of a, b and c corresponds to 7a, 7b and 7c at 90 °C (crossed polarizers, magnification X100). | |
3.2 Differential scanning calorimetry (DSC)
All three different truxene derivatives showed LC properties at room temperature. Upon heating, the columnar phase of 7a compound transferred to isotropic liquid at about 117 °C. On slow cooling texture appears at 103 °C it was stable to room temperature. The compound 7b having decyloxy periphery with methyl group branching at 3 and 7 positions cleared to isotropic liquid phase at 213 °C. Upon slow cooling, typical fan-type of texture appeared at about 205 °C, reflects the existence of columnar liquid crystalline phase. With the three methyl branching in dodecyloxy chain at 3, 7 and 11 positions in the periphery, compound 7c enters to isotropic phase on heating to 126 °C. The well-defined fan-type textures appeared at 120 °C in POM which confirms the columnar LC phase and it was stable to room temperature. Truxene DLCs consisting of rigid big core unit usually possess high clearing temperature.27 Interestingly, the clearing temperature of 7a having one ethyl branching at the β-position of the hexyloxy chain was 117 °C with shorter chain than the 7b containing two methyl branches in longer decyloxy and 7c having three methyl branching in internal longer periphery dodecyloxy alkyl chain. Previously it was observed that the branching near the core destroys mesomorphism due to steric hindrance.54 However, if the π–π interactions between the cores are large and peripheral space is available, the material can preserve mesomorphism despite the branching near the core. The DSC traces of compound 7a, 7b and 7c are shown in Fig. 2 and thermal behaviour of truxene DLCs is presented in Table 1.
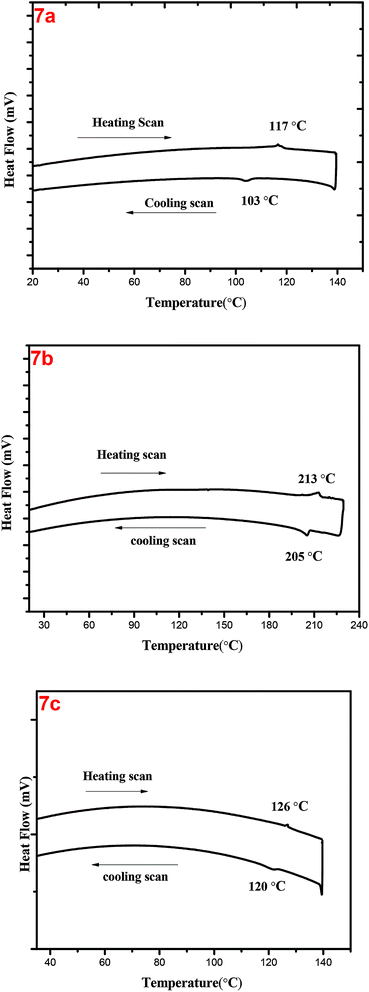 |
| Fig. 2 Differential scanning calorimetry (DSC) curves of 7a, 7b and 7c recorded at scan rate of 10 °C min−1 (1st heating/cooling cycle). | |
Table 1 Phase transition temperatures (peak, °C) observed in DSC of compounds 7a, 7b and 7c. Cr = crystal; Colh = hexagonal columnar mesophase, Iso = isotropic phase
Compound |
Transition temperature (°C) and enthalpy changes (kJ mol−1 in parentheses) |
Heating scan |
Cooling scan |
7a |
Colh 117 (2.2) Iso |
Iso 103 (1.9) Colh |
7b |
Colh 213 (7.0) Iso |
Iso 205 (7.0) Colh |
7c |
Colh126 (2.7) Iso |
Iso120 (4.6)Colh |
3.3 Small angle X-ray scattering (SAXS)
The liquid crystalline phases of the compounds were further studied by SAXS study. The SAXs pattern of all compounds are recorded at 50 °C on heating runs and the intensity vs. 2θ diffraction pattern is shown in Fig. 3. All the three compounds 7a, 7b and 7c shows the diffraction peaks of hexagonal columnar phase in small angle region with the reciprocal ratio 1
:
1/√3
:
1/2
:
1/√7. As mentioned before, compounds 7a, 7b and 7c were in LC state at room temperature, so we focused more on results at room temperature compound (7a) with lowest isotropic temperature as it is more useful for applications. XRD results of 7a at room temperature shows the diffraction peaks of d spacing d1 = 20.26 Å, d2 = 11.68 Å, d3 = 10.11 Å and d4 = 7.64 Å. In the wide angle region, they showed the relatively broad peak at higher 2θ value corresponds to stacking of one molecular core on the other in each columns which is typical characteristic of hexagonal columnar phase and as the chain length increases the core–core separation is decreased which reveals that the packing is more compact in compound 7c, theses values are 3.70 Å, 3.59 Å and 3.42 Å, respectively for 7a, 7b and 7c. The above results confirmed that all these three hexaalkoxytruxene derivatives are self-assembled in the columnar hexagonal fashion in their liquid crystal phase. Crystal data and miller indexes for all the compounds is presented in Table 2.
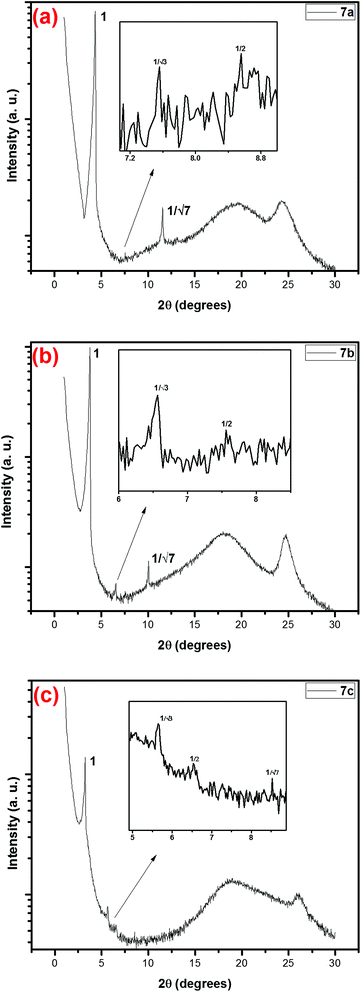 |
| Fig. 3 Small angle X-ray diffraction pattern of compounds (a) 7a (b) 7b and (c) 7c. (At 50 °C from heating runs.) | |
Table 2 Crystal data for compounds 7a, 7b and 7c
Compounds |
2θ (degrees) |
d spacing (Å) |
Colh parameter |
Miller indexes |
Alkyl-chain length (Å) |
Core–core separation (Å) |
7a |
4.37 |
20.26 |
1 |
100 |
4.61 |
3.70 |
7.56 |
11.68 |
1/√3 |
110 |
8.74 |
10.11 |
1/2 |
200 |
11.57 |
7.64 |
1/√7 |
210 |
7b |
3.78 |
23.40 |
1 |
100 |
4.78 |
3.59 |
6.55 |
13.50 |
1/√3 |
110 |
7.56 |
11.68 |
1/2 |
200 |
10.04 |
8.8 |
1/√7 |
210 |
7c |
3.25 |
27.20 |
1 |
100 |
4.87 |
3.42 |
5.64 |
15.68 |
1/√3 |
110 |
6.55 |
13.50 |
1/2 |
200 |
8.57 |
10.32 |
1/√7 |
210 |
3.4 Linear optical properties
The application of discotic liquid crystals in devices is strongly depend on their optoelectronic properties. The absorption spectrum and emission spectrum for 7a, 7b and 7c derivatives were measured. The absorption and photoluminescent (PL) spectrum of these compounds were recorded in chloroform solution (0.1 mg mL−1) as shown in Fig. 4. All these three compounds show the absorption maximum at 238 nm, 290 nm and 317 nm. The absorption spectra in chloroform of all compounds is similar to that previously reported similar compounds.56 Bright strong blue fluorescence peak was observed at 408 nm, 432 nm and 457 nm when the all three sample were excited at 317 nm (Fig. 4). Both absorption and Photoluminescence spectrum of 7a, 7b and 7c are almost same and mirror image to each other, so these results reveals that the difference in branching at peripheral chains in truxene discotics has no effect on their optical properties of the liquid crystals.
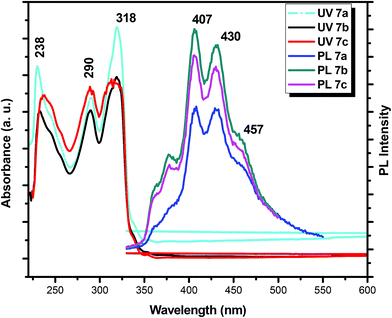 |
| Fig. 4 Normalized absorption 7a, 7b and 7c and emission spectrum of 7a, 7b and 7c (obtained upon exited at λmax = 317 nm) (in CH2Cl2 at room temperature). | |
3.5 Non-linear optical characterization
Nonlinear optical absorption studies of 7a, 7b and 7c in the ns excitation time scale was carried out using the open aperture Z-scan technique (Fig. 5), employing linearly polarized 5 ns Gaussian laser pulses obtained from a Q-switched frequency-doubled Nd:YAG laser, operating in the single-shot mode. The laser beam was focused using a converging lens of focal length 10.75 cm. Beam propagation direction is taken as the z axis, and the focal point is taken as z = 0. The sample were taken in a 1 mm path length cuvette and had a linear transmission of 69% at the excitation wavelength of 532 nm. The cuvette was mounted on a stepper motor controlled linear translational stage and was translated from −z to +z through the focal point in successive steps, and the transmitted energy was measured at each step using a pyro-electric energy detector (Laser Probe, RjP-735). The graph plotted between the sample position and light transmission is known as the Z-scan curve, from which the coefficient of nonlinear optical absorption can be extracted. All the NLO studies were taken in molecular magnitude for the sample 7a, 7b and 7c by making samples in chloroform solution of the concentration of 0.1 mg mL−1.
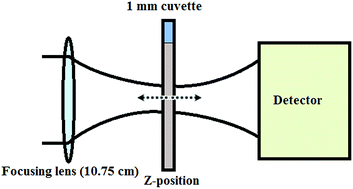 |
| Fig. 5 Representative schematic of the open aperture Z-scan setup used for nonlinear optical measurements. A laser beam is focused using a converging lens. The light energy transmitted by the sample (taken in a 1 mm cuvette) at different positions is monitored as the sample is translated along the z-axis, through the focal point. | |
Nonlinear absorption in a typical Z-scan measurement is indicated by a smooth valley shaped curve symmetric about z = 0 (focal point) position. The Z-scan curve, and nonlinear transmission as a function of input intensity calculated from the Z-scan curve, obtained for compounds 7a, 7b and 7c are shown in Fig. 6 and 7 respectively. These figures indicate a typical absorptive nonlinearity. To identify the exact nature of the absorptive nonlinearity, the Z-scan data was fitted to different nonlinear transmission equations. The best fit was obtained for a three photon absorption (3 PA) type process. The net nonlinear absorption coefficient for a 3 PA process can be expressed as
where
I is the input intensity,
α0 is the unsaturated linear absorption coefficient and
γ is the 3 PA coefficient. The transmitted intensity for a given input intensity is calculated by numerically solving the following propagation equation,
where
z′ indicates the propagation distance within the sample.
57 The calculated nonlinear optical parameters are presented in
Table 3.
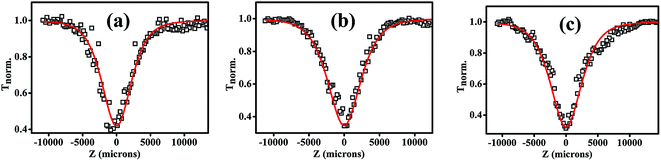 |
| Fig. 6 Open aperture Z-scan curves measured in (a) 7a (b) 7b and (c) 7c for 532 nm, 5 ns laser pulse excitation, at laser pulse energy of 50 μJ. Solid curves are numerical fits to the data points using eqn (2). | |
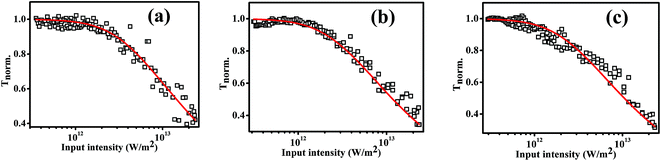 |
| Fig. 7 Input intensity dependent normalized transmission curves calculated from the open aperture Z-scan curves measured in (a) 7a (b) 7b and (c) 7c for 532 nm, 5 ns laser pulse excitation, at laser pulse energy of 50 μJ. Solid curves are numerical fits to the data points using equation. | |
All three truxene discotic liquid crystal compounds (7a, 7b and 7c) exhibits large three photon absorptive nonlinearity and strong optical limiting property in the ns excitation domain at 532 nm. The optical limiting threshold value (the input fluence at which the transmission drops to 50% of the linear transmission) values calculated are presented in Table 3.
Table 3 Nonlinear optical absorption coefficient and optical limiting threshold calculated for samples 7a, 7b and 7c
532 nm, 5 ns excitation |
Sample name |
Input pulse energy (μJ) |
γ (×10−22 m3 W−2) |
Optical limiting threshold (J cm−2) |
7a |
50 |
1.3 |
6.75 |
7b |
50 |
2.2 |
5.4 |
7c |
50 |
2.8 |
4.8 |
It may be noted that the present intensity range of investigation (obtained by using ns laser pulses) is not sufficient for the occurrence of a genuine 3 PA process (which is a fifth order nonlinearity) strong enough to result in the high optical limiting observed. In fact open aperture Z-scans performed with nanosecond laser pulses often lead to dominant excited state absorption in organic molecules. Thus, even though the obtained nonlinearity fits numerically to a fifth order process, in reality this needs not be the actual physical process taking place. For instance, 2 PA followed by excited state absorption (ESA), which is a sequential χ(3): χ(1) process, will give nonlinear transmission curves that fit well numerically to a 3 PA (χ(5)) process.58 We believe that the 3 PA observed in the present sample originates from a similar process involving excited state absorption. Among the three compounds 7c has the maximum nonlinear absorption, which can be due to the presence of long chain group associated with the truxene core compared to 7a and 7b. The nonlinear absorption is increases with increase in the chain length.59
4 Conclusion
Three novel hexaalkoxytruxene derivatives with branched peripheral alkyl chains at different positions have been successfully synthesized, and characterized using different instruments, to ensure that the compounds are liquid crystalline materials at room temperature. These complexes are found to be blue-light emissive at room temperature with rich π-electron conjugation structures. These complexes are also found to exhibit optical limiting property, based on nonlinear absorption. Laser induced director axis reorientation, changes in order parameter and the presence of π-electrons in truxene liquid crystals give rise to the observed large nonlinear optical response.
Acknowledgements
We thank Mrs K. N. Vasudha for her technical support in the characterization of the samples.
References
- Handbook of Liquid Crystals, ed. J. Goodby, P. J. Collings, T. Kato, C. Tschierske, H. F. Gleeson and P. Raynes, Wiley-VCH, Weinheim, 2014, vol. 4, part III, p. 467 Search PubMed.
- Nanoscience with Liquid Crystals: From Self-organized Nanostructures to Applications, ed. Q. Li, Springer, 2014 Search PubMed.
- S. Kumar, NPG Asia Mater., 2014, 6, e82 CrossRef CAS.
- S. Kumar, Isr. J. Chem., 2012, 52, 820 CrossRef CAS.
- S. Kumar, Chemistry of Discotic Liquid Crystals: From Monomers to Polymers, CRC Press, Boca Raton, FL, 2011 Search PubMed.
- H. K. Bisoyi and S. Kumar, Liq. Cryst., 2011, 38, 1427 CrossRef CAS.
- R. J. Bushby and K. Kawata, Liq. Cryst., 2011, 38, 1415 CrossRef CAS.
- W. Pisula, X. Feng and K. Mullen, Chem. Mater., 2011, 23, 554 CrossRef CAS.
- H. K. Bisoyi and S. Kumar, Chem. Soc. Rev., 2010, 39, 264 RSC.
- S. Kumar, Liq. Cryst., 2009, 36, 607 CrossRef CAS.
- J. W. Goodby, I. M. Saez, S. J. Cowling, V. Gortz, M. Draper, A. W. Hall, S. Sia, G. Cosquer, S. E. Lee and E. P. Raynes, Angew. Chem., Int. Ed., 2008, 47, 2754 CrossRef CAS PubMed.
- C. Tschierske, Chem. Soc. Rev., 2007, 36, 1930 RSC.
- S. Sergeyev, W. Pisula and Y. H. Geerts, Chem. Soc. Rev., 2007, 36, 1902 RSC.
- J. Wu, W. Pisula and K. Mullen, Chem. Rev., 2007, 107, 718 CrossRef CAS PubMed.
- S. Laschat, A. Baro, N. Steinke, F. Giesselmann, C. Hagele, G. Scalia, R. Judele, E. Kapatsina, S. Sauer, A. Schreivogel and M. Tosoni, Angew. Chem., Int. Ed., 2007, 46, 4832 CrossRef CAS PubMed.
- T. Kato, N. Mizoshita and K. Kishimoto, Angew. Chem., Int. Ed., 2006, 45, 38 CrossRef CAS PubMed.
- S. Kumar, Chem. Soc. Rev., 2006, 35, 83 RSC.
- B. Donnio, D. Guillon, R. Deschenaux and D. W. Bruce, Comprehensive Coordination Chemistry II, ed. J. A. McCleverty, T. J. Meyer, Elsevier, Oxford, 2003, Vol. 7, p. 357 Search PubMed.
- K. Ohta, K. Hatsusaka, M. Sugibayashi, M. Ariyoshi, K. Ban, F. Maeda, R. Naito, K. Nishizawa, A. M. Van de Craats and J. M. Warman, Mol. Cryst. Liq. Cryst., 2003, 397, 25 CrossRef.
- C. Destrade, H. Gasparoux, A. Babeau, N. H. Tinh and J. Malthete, Mol. Cryst. Liq. Cryst., 1981, 67, 37–48 CrossRef CAS.
-
(a) X. Y. Cao, W. B. Zhang, J. L. Wang, X. H. Zhou, H. Lu and J. Pei, J. Am. Chem. Soc., 2003, 125, 12430–12431 CrossRef CAS PubMed;
(b) M. S. Yuan, Z. Q. Liu and Q. Fang, J. Org. Chem., 2007, 72, 7915–7922 CrossRef CAS PubMed;
(c) K. M. Omer, A. L. Kanibolotsky, P. J. Skabara, I. F. Perepichka and A. J. Bard, J. Phys. Chem. B, 2007, 111, 6612–6619 CrossRef CAS PubMed.
-
(a) L. Sánchez, N. Martın, E. González-Cantalapiedra, A. M. Echavarren, G. M. A. Rahman and D. M. Guldi, Org. Lett., 2006, 8(12), 2451–2454 CrossRef PubMed;
(b) Y. Sun, K. Xiao, Y. Liu, J. Wang, J. Pei, G. Yu and D. Zhu, Adv. Funct. Mater., 2005, 15(5), 818–822 CrossRef CAS.
- X. Y. Cao, X. H. Zhou, H. Zi and J. Pei, Macromolecules, 2004, 37, 8874–8882 CrossRef CAS.
- J. L. Wang, X. F. Duan, B. Jiang, L. B. Gan and J. Pei, J. Org. Chem., 2006, 71, 4400–4410 CrossRef CAS PubMed.
- K. Q. Zhao, C. Chen, H. Monobe, P. Hu, B. Q. Wanga and Y. Shimizu, Chem. Commun., 2011, 47, 6290–6292 RSC.
- K. Isoda, T. Yasuda and T. Kato, Chem.–Asian J., 2009, 4, 1619–1625 CrossRef CAS PubMed.
- L. L. Lia, P. Hu, B. Q. Wang, W. H. Yu, Y. Shimizu and K. Q. Zhao, Liq. Cryst., 2010, 37(5), 499–506 CrossRef.
- H. Monobe, C. Chen, K. Q. Zhao, P. Hu, Y. Miyake, A. Fujii, M. Ozaki and Y. Shimizu, Mol. Cryst. Liq. Cryst., 2011, 545, 149–155 CAS.
- H. L. Ni, H. Monobe, P. Hu, B. Q. Wang, Y. Shimizuand and K. Q. Zhao, Liq. Cryst., 2013, 40(3), 411–420 CrossRef CAS.
- P. H. Huang, J. Y. Shen, S. C. Pu, Y. S. Wen, J. T. Lin, P. T. Chou and M. C. P. Yeh, J. Mater. Chem., 2006, 16, 850 RSC.
- I. C. Khoo, Prog. Quantum Electron., 2014, 38, 77–117 CrossRef PubMed.
- B. Y. Zeldovich, N. F. Pilipetski, A. V. Sukhov and N. V. Tabiryan, JEPT Lett., 1980, 31, 264 Search PubMed.
- P. Shao, B. Huang, L. Chen, Z. Liu, J. Qin, H. Gong, S. Ding and Q. Wang, J. Mater. Chem., 2005, 15, 4502 RSC.
- P. Shao, Z. Li, J. Qin, H. Gong, S. Ding and Q. Wang, Aust. J. Chem., 2006, 59, 49 CrossRef CAS.
- Q. Zheng, G. S. He and P. N. Prasad, Chem. Mater., 2005, 17, 6004 CrossRef CAS.
- J. Pei, J. L. Wang, X. Y. Cao, X. H. Zhou and W. B. Zhang, J. Am. Chem. Soc., 2003, 125, 9544 CrossRef PubMed.
- A. L. Kanibolotsky, R. Berrifge, P. J. Skabara, I. F. Perepichka, D. D. C. Bradley and M. Koeberg, J. Am. Chem. Soc., 2004, 126, 13695 CrossRef CAS PubMed.
- X. Y. Cao, X. H. Liu, X. H. Zhou, Y. Zhang, Y. Jiang, Y. Cao, Y. X. Cui and J. Pei, J. Org. Chem., 2004, 69, 6050 CrossRef CAS PubMed.
- M. Albota, D. Beljonne, J. L. Brédas, J. E. Ehrlich, J. Y. Fu, A. A. Heikal, S. E. Hess, T. Kogej, M. D. Levin, S. R. Marder, D. McCord- Maughon, J. W. Perry, H. Rockel, M. Rumi, G. Subramaniam, W. W. Webb, X. L. Wu and C. Xu, Science, 1998, 281, 1653 CrossRef CAS.
- I. C. Khoo, Liquid Crystals: Physical Properties and Nonlinear
Optical Phenomena, Wiley, New York, 1995 Search PubMed.
- C. Lambert, G. Noell, E. Schmaelzlin, K. Meerholz and C. Braeuchle, Chem.–Eur. J., 1998, 4(11), 2129 CrossRef CAS.
- L. Sanguinet, J. C. Williams, R. J. Twieg, G. Mao, K. D. Singer, G. Wiggers and R. G. Petschek, Chem. Mater., 2006, 18, 4259–4569 CrossRef CAS.
- C. W. Greef, J. Lu and M. Lee, Liq. Cryst., 1993, 15, 75 CrossRef.
- C. W. Greef, Mol. Cryst. Liq. Cryst., 1994, 238, 179 CrossRef.
- L. G. Koreneva, V. F. Zolin and B. L. Davydov, Molecular Crystals In Nonlinear Optics, Nauka, Moscow, 1975 Search PubMed.
- V. A. Pillpovlch, A. A. Kovalev, G. L. Nekrasov, Y. V. Razvln and S. V. Serak, DAN BSSR., 1978, 22(36), 234 Search PubMed.
- F. S. Fetter and J. H. Marburger, Appl. Phys. Lett., 1976, 28, 731 CrossRef PubMed.
-
(a) G. K. L. Wong and Y. R. Shen, Phys. Rev. Lett., 1976, 30, 895 CrossRef;
(b) G. K. L. Wong and Y. R. Shen, Physica, 1973, 59, 2068 Search PubMed;
(c) G. K. L. Wong and Y. R. Shen, Phys. Rev. A: At., Mol., Opt. Phys., 1974, 10, 1277 CrossRef CAS.
- A. W. Amick and L. T. J. Scott, Org. Chem., 2007, 72, 3412–3418 CrossRef CAS PubMed.
- S. Kumar, E. J. Wachtel and E. J. Keinan, Org. Chem., 1993, 58, 3821 CrossRef CAS.
- P. G. Schouten, J. F. van der Pol, J. W. Zwikker, W. Drenth and S. Picken, Mol. Cryst. Liq. Cryst., 1991, 195, 291 CrossRef CAS.
- C. Destrade, H. Yinh, L. Mamlok and J. Malthete, Mol. Cryst. Liq. Cryst., 1984, 114, 139–150 CrossRef CAS.
- K. Ohta, Y. Morizumi, H. Ema, T. Fujimoto and I. Yamamoto, Mol. Cryst. Liq. Cryst., 1991, 208, 55 CrossRef CAS.
- S. Kumar, D. S. S. Rao and S. K. Prasad, J. Mater. Chem., 1999, 9, 2751–2754 RSC.
- S. Kumar and S. K. Varshney, Angew. Chem., Int. Ed., 2000, 39/17, 3140–3142 CrossRef.
- P. Ruan, B. Xiao, H.-L. Ni, P. Hu, B.-Q. Wang, K.-Q. Zhao, Q.-D. Zeng and C. Wang, Liq. Cryst., 2014, 41(8), 1152–1161 CrossRef CAS.
- R. L. Sutherland, Handbook of Nonlinear Optics, Dekker, New York, 2nd edn, 1996 Search PubMed.
- G. P. Banfi, V. Degiorgio and H. M. Tan, J. Opt. Soc. Am. B, 1995, 12, 621 CrossRef CAS.
- Z. Biaszczak and P. Gauden, J. Chem. Soc., Faraday Trans. 2, 1988, 8(3), 239–245 RSC.
|
This journal is © The Royal Society of Chemistry 2015 |