DOI:
10.1039/C4RA16396F
(Paper)
RSC Adv., 2015,
5, 19686-19695
Optimization of the microwave-assisted extraction and antioxidant activities of anthocyanins from blackberry using a response surface methodology
Received
17th December 2014
, Accepted 22nd January 2015
First published on 22nd January 2015
Abstract
Blackberry contains high amounts of anthocyanins, whose extraction method is closely related with anthocyanin content and antioxidant activity. The extraction yield and antioxidant capacity acted as the comprehensive evaluation indexes, and a Box–Behnken design (BBD) of response surface methodology (RSM) was employed to further optimize the microwave-assisted extraction (MAE) conditions for blackberry anthocyanins (BBAC). A significant correlation was found between the double indexes extraction yield and the antioxidant capacity (P < 0.01). The results showed that the optimized extraction conditions included a microwave power of 469 W, a solvent concentration of 52%, a liquid–solid ratio of 25 g mL−1, and a microwave time of 4 min. Under these conditions, the mean experimental value of the extraction yield (2.18 ± 0.06 mg g−1), ABTS assay (32.18 ± 1.54 μM TEAC per g) and DPPH assay (27.18 ± 1.33 μM TEAC per g) corresponded well with the predicted values. Moreover, these mean experimental values were 120% higher than those obtained during ethanol leaching extraction.
1. Introduction
Blackberry is a species of fruit belonging to the Rubus genus of the Rosaceae family, native chiefly to northern temperate regions, and is abundant found in Northeastern America as well as on the Pacific coast.1,2 Blackberry has not only been used in food industries to produce juice, ice cream, yoghurt, jams, wines and jellies,3,4 but also been used for the treatment of various diseases as an astringent, antiscorbutic, diuretic, antidiabetic, and as a therapeutic for chronic diarrhea and spleen enlargement.5–8
Recent research on the chemical components and pharmacologic effects of blackberry reveals that it contains many bioactive constituents such as carbohydrates, flavonoids, amino acids, vitamins, sugars, organic acids, crude proteins.9,10 It has been shown that blackberry contains higher amounts of anthocyanins and other antioxidants than other fruits.11–13
Anthocyanins are natural water-soluble pigments responsible for the orange, red, purple and blue colors of fruits, vegetables and flowers.14 They have been regarded as potential replacements for synthetic food colorants, and thus play an important role in human nutrition.15 Anthocyanins have been reported to exhibit not only the already known anti-oxidative, anti-inflammatory, immunizing activity, anti-tumor, anti-diabetic effects, and anti-aging properties,16–18 but also some other properties that help in fighting coronary heart disease,19 obesity, hypoglycemia,20 memory loss,21 and prevention of cancer.22–24 Therefore, it is important to pursue research on blackberry anthocyanins (BBAC) owing to the fact that the antioxidant activity of BBAC is much higher than that found in other common fruits and vegetables.25
Anthocyanins are highly unstable and very susceptible to degradation. It has been widely acknowledged that the bioactivity of anthocyanins can be affected by many factors, including pH, their own chemical structure, concentration, storage temperature, light, oxygen, and the presence of enzymes, flavonoids, proteins and metal ions.26 Several extraction technologies have been recently suggested for enabling the rapid extraction of anthocyanins from berries and to prevent their degradation during processing such as microwave-assisted extraction,27 supercritical carbon dioxide extraction28 and ultrasound-assisted ethanol extraction.29 Among these, the process of microwave-assisted extraction (MAE) has gained particular attention due to its improved efficiency, reduced extraction time, low solvent consumption, and high level of automation.30,31 MAE utilizes the energy of microwaves to cause the molecular movement and rotation of liquids with a permanent dipole, leading to a rapid heating of the solvent and the sample. MAE has been recently reported as a more efficient method for the extraction of anthocyanins from red raspberries, sour cherry marasca and blueberry, as compared to the conventional solvent extraction.27,32,33 To the best of our knowledge, there are no data on MAE for the isolation of BBAC. Therefore, in this study, a microwave-assisted ethanol extraction method was used to prepare anthocyanin-containing extracts from blackberries.
Response surface methodology (RSM), as an effective statistical method, is widely used for the optimization of complex processes and extraction technologies, because it can depict the complete effects of variables, evaluate the interactions between multiple parameters, reduce the number of experimental trials and shorten the process time. Moreover, it is more precise and effective than many other approaches.34,35 Therefore, in this study, a three-level, four-variable (microwave power, solvent concentration, liquid–solid ratio and microwave time) Box–Behnken design (BBD) of RSM was employed to further optimize the MAE conditions for BBAC. The antioxidant properties of the isolated microwave extracts were correlated with their anthocyanin content.
2. Materials and methods
2.1. Materials and reagents
Fresh blackberry was purchased from Polar Bear Ecological Agriculture Co., Ltd. (China), and then was kept at −18 °C. Cyanidin-3-O-glucoside chloride was obtained from Guizhou Di Da Technology Co., Ltd. (China). 1,1-Diphenyl-2-picrylhdrazyl (DPPH) was gained from Tokyo Kasei Kogyo Co., Ltd. (Japan). 2,2′-Azino-bis(3-ethylbenzthiazoline-6-sulfonic acid) (ABTS) was acquired from Beijing Lark Technology Co., Ltd. (China). All other chemicals and solvents used were of analytical grade.
2.2. Microwave assisted extraction of BBAC
The MAE procedure that was used in the experiment was developed by Li et al. with some modification.36 After the frozen blackberries were taken out and thawed for 10–12 h at room temperature (25–28 °C), it was homogenized using a household electrical blender (MJ-220BP01A, Guangdong Beauty Life Electrical Appliance Manufacturing Co., Ltd., China), which was further used as sample. A necessary amount of sample (20 g) was weighted and put into a conical flask. Next, 200 mL of 50% ethanol was added into the flask. Then, the mixtures were extracted in a microwave extraction reaction workstation (Model EM-202MS1, Hefei Royalstar Sanyo Electrical Co., Ltd., China; working at frequency of 2450 MHz with maximum power level of 1080 W) with a pre-determined extraction power (400 W), and with an extraction time of 5 min. After the flask was taken out and immediately cooled to room temperature using a water bath, the weight loss was offset by the addition of the same solvent. Finally, the solution in the flask was centrifuged at 4390g for 5 min in a low-speed centrifuge (Model TDZ5-WS, Changsha Ordinary Instrument Co., Ltd., China), and the supernatant was used for the determination of the total anthocyanin content (TAC).
The TAC was investigated according to the procedure described by Ivanovic et al. with some modification.37 Briefly, 1 mL of the supernatant and 5 mL of a 1% (v/v) solution of hydrochloric acid in methanol were added to the hydrolysis tube, and then kept in a 100 °C water bath for 10 min. The absorbance of the solution was measured at 530 nm using cyanidin-3-O-glucoside chloride (0.2113 mg mL−1) as a standard on a Microplate Reader (Spectra Max Plus 384, Molecular Devices Co., Ltd., America). The BBAC yield (mg g−1) was calculated using the following formula:
|
 | (1) |
where
C is the concentration of BBAC in the standard curve (μg mL
−1),
V represents the volume of extraction solution (mL),
N represents the dilution multiple and
M represents the sample weight (g).
2.3. Determination of the ABTS radical scavenging activity of BBAC
The BBAC were evaluated for their ability to scavenge ABTS˙+ radicals. The measurements were carried out using a Microplate Reader in the kinetic mode following procedures described by Re et al.38 ABTS˙+ was produced by the reaction of 7 mM ABTS solution with 2.5 mM potassium persulfate for 16 h in a dark at room temperature. The ABTS˙+ solution was diluted with water to an absorbance of 0.70 (±0.02) at 734 nm and equilibrated at 30 °C. Subsequently, 50 μL of the BBAC solution samples or Trolox standards in ethanol were added to 1000 μL of the diluted ABTS˙+ solution, which was then introduced into the ELISA plate. The absorbance values were recorded continuously for 20 min at 734 nm, and at 25 °C. The standard curve was generated based on the percentage of inhibition of the blank absorbance by Trolox at 20 min versus the Trolox concentration (25–800 μmol L−1). The total antioxidant capacity of the samples was calculated and termed as the Trolox equivalent (TE) based on the percentage of inhibition of the blank absorbance by the samples after 20 min. The scavenging activity (SA) and Trolox equivalent antioxidant capacity (TEAC) of the ABTS˙+ radicals of BBAC were determined using the following equations: |
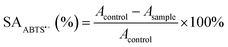 | (2) |
|
 | (3) |
where Acontrol is the absorbance of 1 mL ABTS˙+ mixed with 50 μL ethanol solution, Asample represents the absorbance of 1 mL ABTS˙+ mixed with 50 μL samples, TEACsample is the equivalent Trolox concentration of the samples in the standard curve (μM) and Csample represents the sample concentration (mg mL−1). The determination was carried out thrice and in triplicate.
2.4. Determination of DPPH scavenging activity of BBAC
DPPH has been used extensively as a free radical to evaluate the reducing substances. The BBAC were evaluated for its abilities to scavenge DPPH˙ radicals. The measurements were carried out using a modified protocol based on the report by Yang et al.39 Briefly, 0.5 mL of various concentrations of the BBAC solution or Trolox standards (3.125–100 μmol L−1) in ethanol were added to a 0.5 mL DPPH˙ solution (0.2 mM in anhydrous ethanol), which was then introduced into an ELISA plate. The absorbance readings were recorded continuously for 40 min at 517 nm at 25 °C. The standard curve was generated based on the percentage of inhibition of the blank absorbance by Trolox at 40 min versus the Trolox concentration. The total antioxidant capacity of the samples was calculated as the Trolox equivalent (TE) based on the percentage of inhibition of the blank absorbance by the samples after 40 min. The scavenging activity (SA) and TEAC of the BBAC with the DPPH˙ radicals were determined using the following equation: |
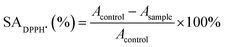 | (4) |
|
 | (5) |
where Acontrol is the absorbance of 0.5 mL DPPH˙ mixed with 0.5 mL ethanol solution, Asample represents the absorbance of 0.5 mL DPPH˙ mixed with 0.5 mL samples, TEACsample is the equivalent Trolox concentration of the samples in the standard curve (μM) and Csample represents the sample concentration (mg mL−1). The determination was carried out thrice and in triplicate.
2.5. Experimental design
After determining the yield and the bioactivity of BBAC, single-factor test was used for obtaining the preliminary range of extraction variables, and a three-level-four-factor BBD of RSM was used to determine the optimal combination of the variables for both the extraction and antioxidant activities. Based on the results of single factor experiments, the four independent variables (Table 1) included microwave power (X1, W), solvent concentration (X2, %), liquid–solid ratio (X3, g mL−1) and microwave time (X4, min), whereas the response variables included the extraction yield and antioxidant activities of BBAC. Each variable was designated at three levels and coded as +1, 0 and −1 for high, intermediate and low values, respectively. The response could be related to the selected variables by the following second-order polynomial model: |
 | (6) |
where Y is the response variable, A0, Ai, Aii and Aij are the regression coefficients for the intercept, linear, quadratic and interaction terms, respectively, and Xi and Xj are the encoded independent variables (i ≠ j) affecting the response of Y.
Table 1 The coded values and the corresponding actual values of the optimization parameters
Solvent |
Code |
Microwave power (W) |
Solvent concentration (%) |
Liquid–solid ratio (g mL−1) |
Microwave time (min) |
Ethanol |
−1 |
250 |
20 |
15 |
1 |
0 |
400 |
40 |
20 |
3 |
1 |
550 |
60 |
25 |
5 |
2.6. Statistical analysis
SPSS 18.0 software (SPSS Inc., Chicago, IL, USA) was used to analyze the bioactivity experimental and single-factor data. The experimental design and the regression analysis of the experimental data exploited Design-Expert 8.0.5 (Trial version, Stat-Ease Inc., Minneapolis, MN, USA).
The regression coefficients were determined from the analysis of variance (ANOVA). Student's t-test was employed for evaluating the statistical significance of the regression coefficient, and Fischer's F-test at a probability (P) of 0.001, 0.01 or 0.05 was used to determine the second-order model equation; its fitness was expressed by the regression coefficient R2. The adequacy and significance of the model were tested from the F-value, determination coefficient (R2) and a lack of fit, as determined from ANOVA.
3. Results and discussion
3.1. Effect of microwave power on the yield and antioxidant capacity of BBAC
It is very important to maintain the microwave at an optimal working power during anthocyanin extraction.40 In the present study, the effect of various microwave power points (100–700 W) on the extraction and antioxidant capacity of BBAC was investigated, while keeping the solvent concentration, liquid–solid ratio and the microwave time at 50%, 10 g mL−1 and 5 min, respectively. As shown in Fig. 1a, BBAC content in the extract, ABTS assay and DPPH assay increased with the increase of microwave power from 100 to 400 W, and peaked at around 400 W. Further enhancement in the power, however, resulted into a reduced BBAC content from the extract. Thus, the optimal power to be chosen for BBAC extraction was set at 400 W. This was identical to the result reported by Zou et al.41
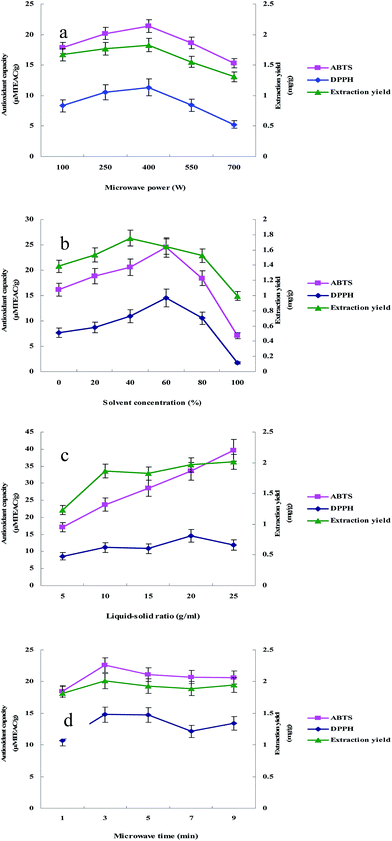 |
| Fig. 1 The effects of different microwave power settings (a), solvent concentrations (b), liquid–solid ratios (c) and microwave times (d) on the extraction yield and antioxidant capacity of BBAC. | |
3.2. Effect of solvent concentration on yield and antioxidant capacity of BBAC
Solvent concentration played a prominent role in obtaining a high extraction efficiency of the anthocyanins during MAE.42 As shown in Fig. 1b, the effect of solvent concentration on the extraction yield was investigated in this study. Increasing the tested ratio of ethanol to raw material (from 0% to 100%) could improve BBAC extraction, and the increase leveled off at a ratio of 40%. However, for the antioxidant capacity of BBAC, the increase leveled off at a ratio of 60%. The results of statistical analysis showed significant differences for the solvent concentration tested (P < 0.05). Therefore, considering the solvent cost problem, an optimal ratio of 40% was found to be favorable for anthocyanin production. Zheng et al. also reported similar results previously.33
3.3. Effect of liquid–solid ratio on yield and antioxidant capacity of BBAC
Liquid–solid ratio played an outstanding role in getting a high extraction efficiency for the anthocyanins during MAE.33 To investigate the effect of liquid–solid ratio on the extraction yield and antioxidant capacity of BBAC, a liquid–solid ratio range from 5 to 25 g mL−1 was tested, while the microwave power, solvent concentration and microwave time were kept at 400 W, 40% and 5 min, respectively. An increase of BBAC content and ABTS assay output was observed with the increase of liquid–solid ratio from 5 to 25 g mL−1, but a further increase in the liquid–solid ratio could not significantly increase the BBAC content, and the DPPH assay output reached a maximum at 20 g mL−1 (Fig. 1c). Therefore, a liquid–solid ratio 20 g mL−1 was chosen as the optimal ratio for the present experiment. Similar results were obtained by Yang and co-workers.43
3.4. Effect of microwave time on the yield and antioxidant capacity of BBAC
Microwave time played significant roles in getting a high extraction efficiency of anthocyanins during MAE.40 It is expected to show an effect on the final yield and antioxidant capacity of BBAC during the recovery, and on the energy cost as well as on the extraction efficiency. In this study, an increase in the BBAC extraction was observed with an increase in the microwave time from 1 to 9 min, reaching a maximum at 3 min. However, a further increase in the microwave time resulted into a decreased BBAC content, and ABTS as well as DPPH assay outputs (Fig. 1d). Thus, the microwave time 3 min was chosen as the optimal time, based on this study. This conclusion was in agreement with the results published by Zou et al.41
3.5. Optimization of the extraction conditions of BBAC
3.5.1. Correlation analysis for extraction yield and antioxidant capacity. The aim of the correlation analysis was to seek a possible statistical correlation between anthocyanins and their antioxidant activity and to develop an extraction method for blackberries for the production of anthocyanin extracts with high antioxidant activity. To study the relationships between anthocyanins and the antioxidant activities of blackberries, the data from the extraction yield, ABTS assay and DPPH assay outputs for BBAC from Table 2 were analyzed using a bivariate correlation analysis. It can be seen from Table 3 that the correlation between the double indexes extraction yield and the antioxidant capacity were very significant with P values (P < 0.01). Therefore, the selection of one index can be used to reasonably evaluate the results corresponding to the optimization of the MAE conditions for BBAC. Therefore, the extraction yield index was chosen to evaluate the result of RSM.
Table 2 The coded experimental and predicted values for the RSM design using ethanol as the solvent
Run |
X1 |
X2 |
X3 |
X4 |
Extraction yield (mg g−1) |
ABTS (μM TEAC per g) |
DPPH (μM TEAC per g) |
Experimental |
Predicted |
Experimental |
Predicted |
Experimental |
Predicted |
1 |
1 |
0 |
1 |
0 |
2.16 |
1.96 |
30.39 |
26.18 |
26.99 |
21.26 |
2 |
0 |
0 |
0 |
0 |
2.14 |
2.10 |
28.48 |
23.04 |
24.48 |
22.82 |
3 |
1 |
−1 |
0 |
0 |
2.09 |
2.08 |
23.06 |
26.82 |
23.55 |
24.69 |
4 |
0 |
1 |
1 |
0 |
2.15 |
2.13 |
30.07 |
28.74 |
26.09 |
24.74 |
5 |
0 |
0 |
0 |
0 |
2.11 |
2.02 |
27.44 |
22.25 |
25.15 |
20.30 |
6 |
0 |
0 |
0 |
0 |
2.10 |
1.98 |
29.85 |
26.54 |
28.00 |
24.83 |
7 |
0 |
0 |
−1 |
−1 |
2.01 |
1.85 |
22.03 |
20.18 |
20.23 |
20.87 |
8 |
0 |
−1 |
−1 |
0 |
2.01 |
2.12 |
19.67 |
31.44 |
19.21 |
27.43 |
9 |
0 |
−1 |
0 |
−1 |
2.04 |
1.95 |
23.57 |
27.34 |
21.35 |
21.39 |
10 |
1 |
0 |
−1 |
0 |
1.96 |
2.06 |
18.58 |
27.60 |
19.41 |
24.95 |
11 |
0 |
1 |
0 |
1 |
2.08 |
1.95 |
28.22 |
29.63 |
25.22 |
25.73 |
12 |
0 |
0 |
1 |
−1 |
1.98 |
2.03 |
26.30 |
28.14 |
26.02 |
23.78 |
13 |
0 |
0 |
0 |
0 |
2.18 |
1.99 |
29.22 |
18.90 |
23.79 |
18.63 |
14 |
0 |
−1 |
0 |
1 |
1.98 |
2.03 |
24.12 |
19.56 |
21.93 |
21.36 |
15 |
−1 |
1 |
0 |
0 |
2.10 |
2.07 |
26.38 |
24.17 |
24.57 |
24.23 |
16 |
0 |
1 |
0 |
−1 |
2.11 |
2.17 |
25.29 |
29.85 |
23.34 |
26.86 |
17 |
0 |
−1 |
1 |
0 |
2.07 |
1.95 |
24.34 |
23.82 |
23.26 |
21.26 |
18 |
1 |
0 |
0 |
−1 |
2.05 |
1.96 |
28.58 |
19.82 |
24.64 |
20.32 |
19 |
0 |
0 |
1 |
1 |
2.13 |
1.98 |
31.23 |
28.22 |
28.13 |
25.06 |
20 |
1 |
1 |
0 |
0 |
2.12 |
2.15 |
28.96 |
30.99 |
25.31 |
27.62 |
21 |
−1 |
0 |
0 |
−1 |
1.93 |
2.03 |
27.71 |
23.88 |
20.66 |
21.37 |
22 |
0 |
0 |
−1 |
1 |
1.85 |
2.09 |
20.01 |
25.87 |
20.30 |
23.39 |
23 |
1 |
0 |
0 |
1 |
2.04 |
2.00 |
28.77 |
24.12 |
24.32 |
22.30 |
24 |
−1 |
0 |
0 |
1 |
1.95 |
2.09 |
29.65 |
28.47 |
25.84 |
25.63 |
25 |
0 |
1 |
−1 |
0 |
2.02 |
2.14 |
20.39 |
28.45 |
22.13 |
25.47 |
26 |
0 |
0 |
0 |
0 |
2.18 |
2.14 |
27.25 |
28.45 |
25.91 |
25.47 |
27 |
−1 |
−1 |
0 |
0 |
1.97 |
2.14 |
25.53 |
28.45 |
21.31 |
25.47 |
28 |
−1 |
0 |
1 |
0 |
1.97 |
2.14 |
28.89 |
28.45 |
25.55 |
25.47 |
29 |
−1 |
0 |
−1 |
0 |
1.94 |
2.14 |
23.86 |
28.45 |
21.46 |
25.47 |
Table 3 The correlation analysis of the extraction yield and the antioxidant capacity double indexes
Index |
Extraction yield |
DPPH |
ra |
P |
ra |
P |
**Significant at P < 0.01. |
DPPH |
0.611** |
0.001 |
|
|
ABTS |
0.534** |
0.003 |
0.847** |
0.001 |
3.5.2. Statistical analysis and the model fitting. The BBD of RSM in the experimental design involves four independent variables, three levels and five replicates at the center point (Table 1), which was carried out to measure the inherent variability and process stability. The experimental conditions and the fit statistics of the extraction yield of 29 runs with BBD design are shown in Table 2, and all the tests were performed in triplicate. As shown in Table 2, the extraction yield of the BBAC values (mg g−1) varied from 1.85 to 2.18 mg g−1, the ABTS assay and DPPH assay outputs of BBAC (μM TEAC per g) varied from 20.01 to 30.39 and from 19.21 to 28.13 μM TEAC per g, respectively.The results of the extraction yield were affected by the microwave power, solvent concentration, liquid–solid ratio and microwave time and were fitted to a second-order polynomial equation. The values of the regression coefficients were subsequently calculated.
The effects of the four variables on the extraction yield of BBAC (Table 4) were highly significant. The predicted model of the extraction yield value was obtained from the following second-order polynomial equation:
|
Y1 = 2.14 + 0.048X1 + 0.034X2 + 0.057X3 − 0.021X1X2 + 0.042X1X3 + 0.016X2X3 + 0.078X3X4 − 0.066X12 − 0.011X22 − 0.068X32 − 0.082X42
| (7) |
Table 4 ANOVA for the effects of microwave power (X1), solvent concentration (X2), liquid–solid ratio (X3) and microwave time (X4) on the extraction yield of BBAC with ethanol as the solvent using predicted polynomial models
Source |
Sum of squares |
Df |
Mean square |
F-value |
P-Value |
Significant |
Model |
0.195 |
14 |
0.014 |
22.014 |
<0.0001 |
*** |
X1 |
0.027 |
1 |
0.027 |
42.739 |
<0.0001 |
*** |
X2 |
0.015 |
1 |
0.015 |
23.049 |
0.0003 |
*** |
X3 |
0.039 |
1 |
0.039 |
61.085 |
<0.0001 |
*** |
X4 |
0.001 |
1 |
0.001 |
1.165 |
0.2987 |
|
X1 X2 |
0.002 |
1 |
0.002 |
3.743 |
0.0735 |
|
X1 X3 |
0.007 |
1 |
0.007 |
11.243 |
0.0047 |
** |
X1 X4 |
0.000 |
1 |
0.000 |
0.537 |
0.4759 |
|
X2 X3 |
0.001 |
1 |
0.001 |
1.717 |
0.2111 |
|
X2 X4 |
0.000 |
1 |
0.000 |
0.376 |
0.5494 |
|
X3 X4 |
0.025 |
1 |
0.025 |
38.996 |
<0.0001 |
*** |
X12 |
0.028 |
1 |
0.028 |
43.917 |
<0.0001 |
*** |
X22 |
0.001 |
1 |
0.001 |
0.976 |
0.3400 |
|
X32 |
0.031 |
1 |
0.031 |
48.870 |
<0.0001 |
*** |
X42 |
0.044 |
1 |
0.044 |
70.143 |
<0.0001 |
*** |
Residual |
0.009 |
14 |
0.001 |
|
|
|
Lack of fit |
0.003 |
10 |
0.000 |
0.247 |
0.9670 |
Not significant |
Pure error |
0.005 |
4 |
0.001 |
|
|
|
Cor total |
0.203 |
28 |
|
|
|
|
R2 |
0.958 |
|
|
|
|
|
Adj. R2 |
0.916 |
|
|
|
|
|
Pred. R2 |
0.872 |
|
|
|
|
|
Adequate precision |
18.143 |
|
|
|
|
|
The predicted values of the extraction yield based on the above quadratic predictive model are shown in Table 2.
The statistical significance of the regression equation for the response surface quadratic polynomial model, as evaluated from the F-test, T-test and ANOVA are presented in Table 4. The results of the high model F-value (22.014) and low P-value (P < 0.0001) show that the models were highly significant. The determination coefficient (R2) for the model (0.958) was close to 1.0, thus implying a satisfactory correlation between the actual and predicted values. The value of the adjusted determination coefficient R2 (adj. R2) value was 0.916, suggesting that most of the variation (>91.6%) in the extraction yield could be predicted by the model, and that less than 8.4% variations could not be explained by the model.
A lack-of-fit was used to measure the failure of the model to represent the data in the experimental domain at points that were not included in the regression. The F-value of 0.247 and P-value of 0.967 for the extraction yield implied that the lack of fit was not significant relative to the pure error owing to the noise. Adequate precision compared the range of the predicted values at the design points to the average prediction error. A ratio greater than 4 indicates adequate model discrimination. During this study, the values were well above 4.
The P-values were used as a tool to check the significance of each coefficient, which in turn may indicate the pattern of the interactions between the variables. The smaller the value of P, the more significant was the corresponding coefficient. It can be seen from Table 4 that the linear coefficients (X1, X2, X3), the quadratic term coefficient (X12, X32, X42) and the cross product coefficients (X1X3, X3X4) were very significant with P values (P < 0.01). The other term coefficients were considered significant (P > 0.05).
3.5.3. Analysis of response surfaces. The 3D response surface and the 2D contour plots are the graphical representations of regression equation. They provided a method to visualize the relationship between the responses and the experimental levels of each variable, as well as the type of interactions between two test variables. The shapes of the contour plots, circular or elliptical, indicate whether the mutual interactions between the variables are significant or not. A circular contour plot indicates that the interactions between the corresponding variables are negligible, whereas an elliptical contour plot indicates that the interactions between the corresponding variables are significant. In this study, the results of the extraction yield of BBAC was affected by microwave power, solvent concentration, liquid–solid ratio and microwave time, as shown in Fig. 2 and 3.
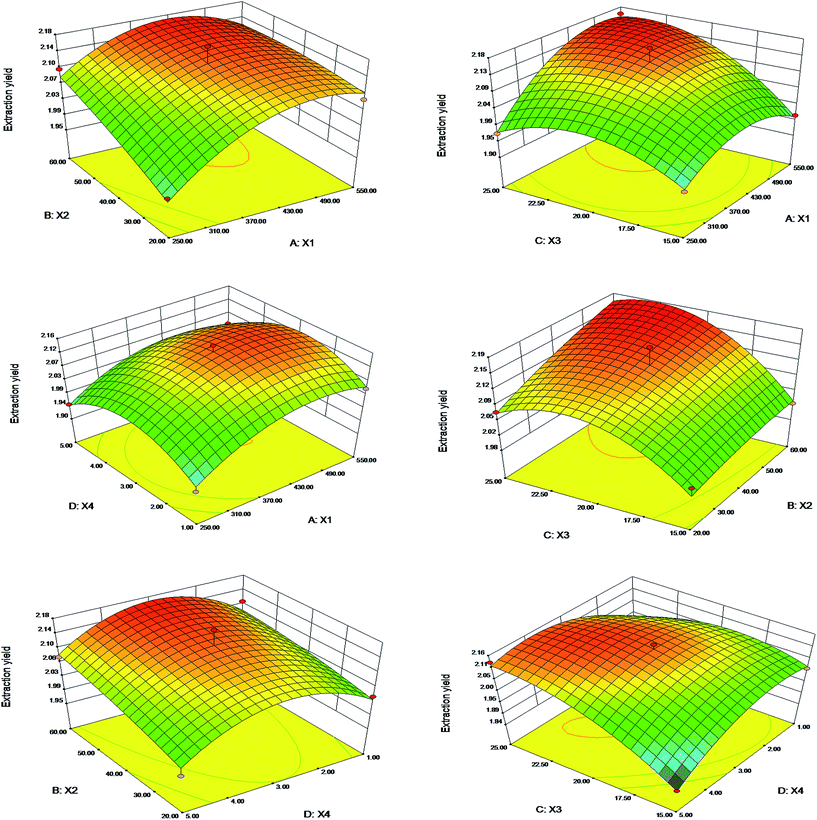 |
| Fig. 2 Response surface (3D) showing the effect of microwave power (X1), solvent concentration (X2), liquid–solid ratio (X3) and microwave time (X4) on the extraction yield of BBAC. | |
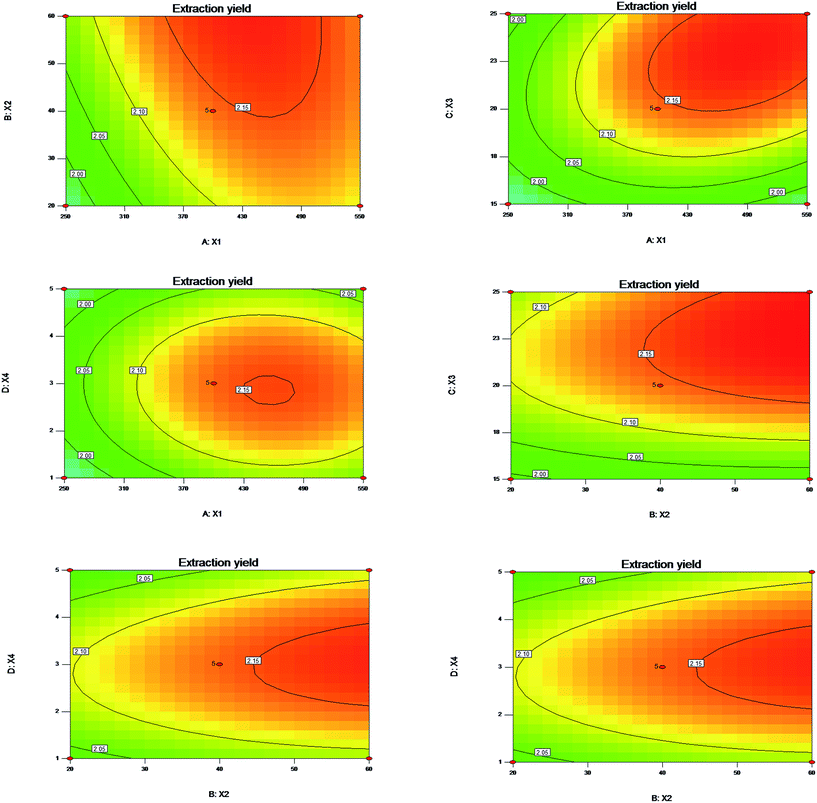 |
| Fig. 3 Contour plots showing the effect of microwave power (X1), solvent concentration (X2), liquid–solid ratio (X3) and microwave power (X4) on the extraction yield of BBAC. | |
Fig. 2a and 3a, which give the extraction yield of BBAC as a function of the microwave power and solvent concentration at a fixed liquid–solid ratio (20 g mL−1) and microwave time (3 min), indicated that the extraction yield increased rapidly with an increase in the microwave power from 250 to 470 W and decreased slowly with an increase in the microwave power from 500 to 550 W. The extraction yield of BBAC increased with the increase in the solvent concentration from 20% to 60%. It can be seen that the maximum extraction yield of BBAC was attained when the microwave power and the solvent concentration were around 470 W and 60%, respectively. A similar result was also reported previously by Zou et al.41
The extraction yields of BBAC, corresponding to the different microwave power settings and liquid–solid ratios are shown in Fig. 2b and 3b. The solvent concentration and the microwave time were fixed at 40% and 3 min, respectively. The extraction yield increased rapidly with an increase in the microwave power from 250 to 410 W, and also increased with an increase in the liquid–solid ratio from 15 to 21. However, the extraction yield decreased rapidly with an increase in the microwave power from 410 to 550 W and in the liquid–solid ratio from 21 to 25 g mL−1. These results were in agreement with a study done by Zou et al.41
Fig. 2c and 3c show the 3D response surface plot and the contour plot at varying microwave power and microwave time settings, at a fixed solvent concentration (40%) and at a liquid–solid ratio of 20 g mL−1. It indicates that the maximum extraction yield of BBAC can be achieved when the microwave power and the microwave time are at the threshold level of around 430 W and 2.8 min, respectively. A similar result was also reported previously by Liazid et al.41
Fig. 2d and 3d illustrate the 3D response surface plot and the contour plot at varying solvent concentrations and liquid–solid ratios and at a fixed microwave power (400 W) and microwave time (3 min), and indicate that the extraction yield of BBAC increases with an increase in the solvent concentration from 20% to 48%. The extraction yield of BBAC reached the plateau region where the yield was maximized and did not further increase the yield. The extraction yield increased rapidly with an increase in the liquid–solid ratio from 15 to 21 g mL−1 and decreased slowly with an increase in the microwave power from 22 to 25 g mL−1. It can be seen that the maximum extraction yield of BBAC can be achieved when the solvent concentration and the liquid–solid ratio are around 48% and 21 g mL−1, respectively. This was accordant with the result reported by Zheng et al.33
In Fig. 2e and 3e, the 3D response surface plot and the contour plot were developed for the extraction yield of BBAC with a varying solvent concentration and microwave time at fixed microwave power (400 W) and liquid–solid ratio (20 g mL−1). The maximum extraction yield of BBAC was achieved when the solvent concentration and the microwave time were at the threshold level of around 60% and 2.7 min, respectively. Zou et al. reported similar results.41
The 3D response surface plot and the contour plot based on the independent variable liquid–solid ratio and the microwave time are shown in Fig. 2f and 3f, whereas the other two independent variables, viz. the microwave power and the solvent concentration were maintained at 400 W and 40%, respectively. An increase in the extraction yield of BBAC could be significantly achieved with the increasing liquid–solid ratio. The extraction yield of BBAC increased with the microwave time from 1 to 3.8 min, and reached the maximum value at an extraction time of around 4.2 min. However, beyond this time, the extraction yield of BBAC decreased. This conclusion conformed to the opinion of Elez Garofulić et al.32
3.6. Verification of predictive model
Response surface optimization is more advantageous than the traditional single parameter optimization, in which it saves time, space and raw material. In order to validate the adequacy of the model equations, a verification experiment was carried out under the optimal conditions: microwave power: 469 W, solvent concentration: 52%, liquid–solid ratio: 25 g mL−1 and microwave time: 4 min. A good agreement exists between the values predicted using the model equations and the experimental values at the points of interest. To ensure that the predicted result was not biased toward the practical value, an experimental rechecking was performed using these deduced optimal conditions. This set of conditions was determined to be optimal from the RSM optimization approach and was also used to validate experimentally, as well as to predict, the values of the response using the model equation. The mean value of the extraction yield (2.18 ± 0.06 mg g−1), ABTS assay (32.18 ± 1.54 μM TEAC per g) and DPPH assay (27.18 ± 1.33 μM TEAC per g) (n = 5), obtained from the real experiments, demonstrated the validation of the RSM model. The validation result revealed that there was no significant difference between the experimental and predicted values, suggesting that the response model was adequate for reflecting the expected optimization (Table 5). The result of this analysis indicated that the experimental values were in good agreement with the predicted ones, and also suggested that the model of eqn (7) was satisfactory and accurate.
Table 5 Results of the model validation experiments
No. |
Optimum conditions |
Extraction yield (mg g−1) |
ABTS (μM TEAC per g) |
DPPH (μM TEAC per g) |
Microwave power (W) |
Solvent concentration (%) |
Liquid–solid ratio (g mL−1) |
Microwave time (min) |
Experimental |
Predicted |
Experimental |
Predicted |
Experimental |
Predicted |
1 |
469 |
52 |
25 |
4 |
2.22 |
2.19 |
34.13 |
32.94 |
26.23 |
27.78 |
2 |
469 |
52 |
25 |
4 |
2.1 |
2.19 |
30.08 |
32.94 |
29.08 |
27.78 |
3 |
469 |
52 |
25 |
4 |
2.14 |
2.19 |
33.13 |
32.94 |
25.93 |
27.78 |
4 |
469 |
52 |
25 |
4 |
2.17 |
2.19 |
31.54 |
32.94 |
26.65 |
27.78 |
5 |
469 |
52 |
25 |
4 |
2.25 |
2.19 |
32.04 |
32.94 |
28.01 |
27.78 |
Average |
|
|
|
|
2.18 |
|
32.18 |
|
27.18 |
|
![[thin space (1/6-em)]](https://www.rsc.org/images/entities/char_2009.gif) |
Ethanol leaching extraction |
6 |
0 |
52 |
25 |
60 |
1.82 |
|
21.34 |
|
17.14 |
|
7 |
0 |
52 |
25 |
60 |
1.77 |
|
22.02 |
|
16.79 |
|
8 |
0 |
52 |
25 |
60 |
1.84 |
|
19.16 |
|
17.09 |
|
Average |
|
|
|
|
1.81 |
|
20.84 |
|
17.01 |
|
Furthermore, ethanol leaching extraction were compared with the MAE method, and as seen in Table 5, a mean extraction yield of 1.81 ± 0.04 mg g−1, an ABTS assay of 20.84 ± 1.49 μM TEAC per g and a DPPH assay of 17.01 ± 0.19 μM TEAC per g (n = 5) were obtained from the ethanol leaching extraction. These mean values corresponding to microwave treatment increased 120% as compared to those obtained from the ethanol leaching treatments. Moreover, the mean value of the extraction yield was higher than that reported by Oancea et al.44 The mean value of the antioxidant capacities was higher than that reported by Reátegui et al.45 Therefore, this finding corroborates previous reports that with respect to the anthocyanin content, the microwave technique shows superiority in improving the efficiency, shortening the extraction time, and reducing the solvent consumption. The anthocyanin content and the antioxidant capacity reported, herein, are higher than those previously reported. This may be partly due to the increased extraction efficiency.
4. Conclusions
In the present study, a microwave-assisted extraction method and an ethanol leaching extraction method were screened for the extraction treatment of blackberry, and the extracts exhibited different yields, and different levels of scavenging effects on the DPPH˙ free radicals as well as on the ABTS˙+ free radicals. The microwave-assisted extraction method was found to be the most effective method for improving the yield and antioxidant capacity of BBAC among the other methods tested. When ethanol was used as the solvent, the optimal extraction conditions for the microwave-assisted extract of BBAC are obtained as follows: microwave power: 469 W, solvent concentration: 52%, liquid–solid radio: 25 g mL−1, and microwave time: 4 min. Under these conditions, the mean experimental value of the extraction yield (2.18 ± 0.06 mg g−1), ABTS assay (32.18 ± 1.54 μM TEAC per g) and DPPH assay (27.18 ± 1.33 μM TEAC per g) corresponded well with the predicted values and increased more than 120%, as compared to those obtained during the ethanol leaching extraction experiments.
Acknowledgements
The authors gratefully acknowledge the financial support of the present study by the national sci-tech support plan of China (2011BAC09B01), Guizhou province science and technology plan projects ([2013]3016, KY-2012-005, and 2013-2069) and Guiyang science and technology plan project ([2012401]-4).
References
- J. Dai, A. Gupte, L. Gates and R. J. Mumper, Food Chem. Toxicol., 2009, 47, 837–847 CrossRef CAS PubMed.
- F. Aqil, A. Gupta, R. Munagala, J. Jeyabalan, H. Kausar, R. J. Sharma, I. P. Singh and R. C. Gupta, Nutr. Cancer, 2012, 64, 428–438 CrossRef CAS PubMed.
- A. Rommel, R. E. Wrolstad and D. A. Heatherbell, J. Food Sci., 1992, 57, 385–391 CrossRef CAS PubMed.
- J. L. P. Reátegui, A. P. da Fonseca Machado, G. F. Barbero, C. A. Rezende and J. Martínez, J. Supercrit. Fluids, 2014, 94, 223–233 CrossRef PubMed.
- M. S. Baliga, S. Fernandes, K. R. Thilakchand, P. D'souza and S. Rao, J. Altern. Complement. Med., 2013, 19, 191–197 CrossRef PubMed.
- A. Chaturvedi, G. Bhawani, P. K. Agarwal, S. Goel, A. Singh and R. K. Goel, J. Physiol. Pharmacol., 2009, 53, 137–146 Search PubMed.
- H. Sagrawat, A. S. Mann and M. D. Kharya, Pharmacogn. Mag., 2006, 2, 96 CAS.
- J. M. Veigas, M. S. Narayan, P. M. Laxman and B. Neelwarne, Food Chem., 2007, 105, 619–627 CrossRef CAS PubMed.
- T. J. Hager, L. R. Howard, R. Liyanage, J. O. Lay and R. L. Prior, J. Agric. Food Chem., 2008, 56, 661–669 CrossRef CAS PubMed.
- E. Sariburun, S. Sahin, C. Demir, C. Turkben and V. Uylaser, J. Food Sci., 2010, 75, C328–C335 CrossRef CAS PubMed.
- B. L. Halvorsen, M. H. Carlsen, K. M. Phillips, S. K. Bohn, K. Holte, D. R. Jacobs Jr and R. Blomhoff, Am. J. Clin. Nutr., 2006, 84, 95–135 CAS.
- R. A. Moyer, K. E. Hummer, C. E. Finn, B. Frei and R. E. Wrolstad, J. Agric. Food Chem., 2002, 50, 519–525 CrossRef CAS PubMed.
- G. E. Pantelidis, M. Vasilakakis, G. A. Manganaris and G. Diamantidis, Food Chem., 2007, 102, 777–783 CrossRef CAS PubMed.
- H. Li, Z. Deng, H. Zhu, C. Hu, R. Liu, J. C. Young and R. Tsao, Food Res. Int., 2012, 46, 250–259 CrossRef CAS PubMed.
- C. W. Haminiuk, G. M. Maciel, M. S. Plata Oviedo and R. M. Peralta, Int. J. Food Sci. Technol., 2012, 47, 2023–2044 CrossRef CAS PubMed.
- C. A. Rice-Evans, N. J. Miller, P. G. Bolwell, P. M. Bramley and J. B. Pridham, Free Radical Res., 1995, 22, 375–383 CrossRef CAS.
- N. P. Seeram and M. G. Nair, J. Agric. Food Chem., 2002, 50, 5308–5312 CrossRef CAS PubMed.
- J. Valls, S. Millán, M. P. Martí, E. Borràs and L. Arola, J. Chromatogr. A, 2009, 1216, 7143–7172 CrossRef CAS PubMed.
- G. J. Mazza, Ann. Ist. Super. Sanita, 2007, 43, 369–374 CAS.
- B. Jayaprakasam, L. K. Olson, R. E. Schutzki, M. H. Tai and M. G. Nair, J. Agric. Food Chem., 2006, 54, 243–248 CrossRef CAS PubMed.
- C. Andres-Lacueva, B. Shukitt-Hale, R. L. Galli, O. Jauregui, R. M. Lamuela-Raventos and J. A. Joseph, Nutr. Neurosci., 2005, 8, 111–120 CrossRef CAS PubMed.
- H. S. Aiyer, C. Srinivasan and R. C. Gupta, Nutr. Cancer, 2008, 60, 227–234 CrossRef PubMed.
- G. D. Stoner, L. S. Wang, N. Zikri, T. Chen, S. S. Hecht, C. Huang, C. Sardo and J. F. Lechner, Semin. Cancer Biol., 2007, 17, 403–410 CrossRef CAS PubMed.
- L. S. Wang and G. D. Stoner, Cancer Lett., 2008, 269, 281–290 CrossRef CAS PubMed.
- F. Aqil, R. Munagala, J. Jeyabalan, T. Joshi, R. C. Gupta and I. P. Singh, Cancer, 2014, 101–113 CAS.
- M. Rein, PhD thesis, University of Helsinki, 2005, 87.
- H. Teng, W. Y. Lee and Y. H. Choi, J. Sep. Sci., 2013, 36, 3107–3114 CAS.
- T. Vatai, M. Škerget and Ž. Knez, J. Food Eng., 2009, 90, 246–254 CrossRef CAS PubMed.
- Ö. Aybastıer, E. Işık, S. Şahin and C. Demir, Ind. Crops Prod., 2013, 44, 558–565 CrossRef PubMed.
- P. L. Buldini, L. Ricci and J. L. Sharma, J. Chromatogr. A, 2002, 975, 47–70 CrossRef CAS.
- C. Sparr Eskilsson and E. Björklund, J. Chromatogr. A, 2000, 902, 227–250 CrossRef CAS.
- I. Elez Garofulić, V. Dragović-Uzelac, A. Režek Jambrak and M. Jukić, J. Food Eng., 2013, 117, 437–442 CrossRef PubMed.
- X. Zheng, X. Xu, C. Liu, Y. Sun, Z. Lin and H. Liu, Sep. Purif. Technol., 2013, 104, 17–25 CrossRef CAS PubMed.
- M. Hosseinpour, M. Vossoughi and I. Alemzadeh, J. Environ. Health Sci. Eng., 2014, 12, 33 CrossRef PubMed.
- S. P. Kumar, Indian J. Exp. Biol., 2013, 51, 979–983 CAS.
- Y. Li, L. Han, R. Ma, X. Xu, C. Zhao, Z. Wang, F. Chen and X. Hu, J. Food Eng., 2012, 109, 274–280 CrossRef CAS PubMed.
- J. Ivanovic, V. Tadic, S. Dimitrijevic, M. Stamenic, S. Petrovic and I. Zizovic, 2014, 53, 274–281.
- R. Re, N. Pellegrini, A. Proteggente, A. Pannala, M. Yang and C. Rice-Evans, J. Free Radicals Biol. Med., 1999, 26, 1231–1237 CrossRef CAS.
- B. Yang, M. Zhao, J. Shi, N. Yang and Y. Jiang, Food Chem., 2008, 106, 685–690 CrossRef CAS PubMed.
- Y. Sun, X. Liao, Z. Wang, X. Hu and F. Chen, Eur. Food Res. Technol., 2007, 225, 511–523 CrossRef CAS.
- T. Zou, D. Wang, H. Guo, Y. Zhu, X. Luo, F. Liu and W. Ling, J. Food Sci., 2012, 77, C46–C50 CrossRef CAS PubMed.
- A. Liazid, R. F. Guerrero, E. Cantos, M. Palma and C. G. Barroso, Food Chem., 2011, 124, 1238–1243 CrossRef CAS PubMed.
- Z. Yang and W. Zhai, Innovative Food Sci. Emerging Technol., 2010, 11, 470–476 CrossRef CAS PubMed.
- S. Oancea, C. Grosu, O. Ketney and M. Stoia, Acta Chim. Slov., 2013, 60, 383–389 CAS.
- J. L. P. Reátegui, A. P. da Fonseca Machado, G. F. Barbero, C. A. Rezende and J. Martínez, J. Supercrit. Fluids, 2014, 94, 223–233 CrossRef PubMed.
Footnote |
† These authors contributed equally to the work. |
|
This journal is © The Royal Society of Chemistry 2015 |