DOI:
10.1039/C4RA16184J
(Review Article)
RSC Adv., 2015,
5, 18097-18110
Spectroscopic studies of keto–enol tautomeric equilibrium of azo dyes
Received
11th December 2014
, Accepted 5th February 2015
First published on 5th February 2015
Abstract
Azo dyes account for 60–70% of all dyes known to date. Understanding the factors that affect the direction of keto–enol tautomerism in azo dyes through spectral measurements is crucial to their potential applications. This review encompasses the most important spectroscopic studies of different azo dyes categorized by their structures within the last few years (2010–2014). It is concluded that the stability of the keto and enol forms largely arises from the ability to establish intra-molecular and inter-molecular hydrogen bonding, respectively. There are many factors that affect the keto or enol form, for example, polar solvent, high temperature, neutral pH and electron withdrawing substituents favor the keto form through intramolecular hydrogen bonding, whereas, nonpolar solvent, low temperature, high pH and electron donating groups favor the enol form through intermolecular hydrogen bonding. Encapsulation inside nanocaged microheterogenous systems creates a rigid environment that stabilizes the enol form mostly, while in the solid state, most of the tautomeric equilibrium lies in favor of the keto form. Understanding of keto–enol tautomerism in azo dyes helps to probe solvation dynamics, to tune the pKa values in chemical sensing, and to explain proton transfer in the excited state.
1 Introduction
When the keto and enol forms of an organic compound are at chemical equilibrium, they are said to be tautomers of each other. Since tautomerism is considered as a process of the exchange of a proton between two or more forms, it leads to rearrangement of the electronic density in the whole molecule and, therefore, to significant changes in the spectral and photophysical behaviors.1 Proton transfer in the case of tautomerization is dynamic in nature (Scheme 1), where, kf and kr are the forward and the reverse rate constants, respectively, and the ratio kf/kr is defined as the equilibrium constant (KT). The observed rate constant, kobs, which is the sum of the two rate constants determines the measured rate, while the equilibrium will shift in the direction which has a higher rate constant value. Tautomeric equilibrium (1 → 2, 3 → 4, 5 → 6, and 7 → 8) is driven by the acid–base nature of the species, wherein, the more stable form predominates. The inter-conversion of the two forms involves the movement of an alpha-hydrogen and the shifting of bonding electrons.
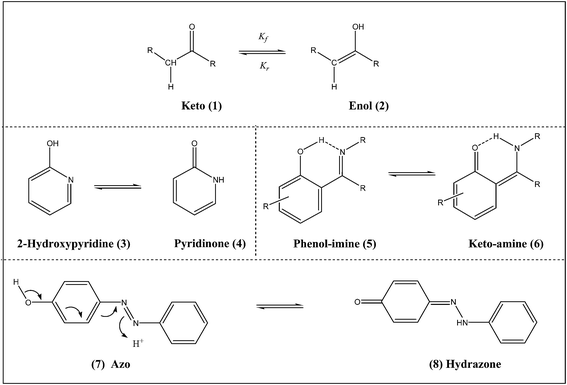 |
| Scheme 1 | |
Spectroscopic studies of tautomeric equilibrium can be carried out in all the three phases (gas, liquid/solution and solid state) of the sample, but is not commonly studied in water as it catalyzes the prototropic exchanges. The studies on tautomerism depend on whether the migrating group is a proton or another entity or whether the study concerns the thermodynamic or the kinetic part, and it also depends on the medium. Keto–enol tautomerism is important in several areas of chemical and biological reactions. Many biochemical reactions have relied deeply on studies of tautomers, e.g., enol tautomers of the bases guanine and thymine can lead to mutation because of their altered base-pairing properties. DNA is built from bases, all of which have a number of different tautomers. Photoinduced tautomerism in which the excited state intramolecular proton transfer (ESIPT) reactions takes place, have also drawn a great deal of attention.2–6 These reactions generally involves transfer of a hydroxyl (or amino) proton to the carbonyl oxygen (or aromatic nitrogen) through a preexisting hydrogen bonding (HB) configuration. As a result of this, the proton transfer tautomer possesses considerable differences in structure and electronic configuration from its corresponding normal species.7 Usually a large Stokes shift (S1 → S0 fluorescence) is observed in such cases. Many important applications of these phenomena are attributed to this rare photophysical property. Some typical examples are probes for solvation dynamics and biological environments,8 the development of laser dyes,9 ultraviolet stabilizers,10 metal ion sensors11 and scintillator counters.12
2 Tautomerism in azo dyes
Dyes are an important class of organic molecules which are an integral part of our lives and are used in numerous industries. For example, textile, paint and plastic industry, dye sensitized solar cells, optics, metal extraction, sensors, etc. are industries that depend heavily on dyes. Molecular structure, source, color and method of application in color index (CI) are some of the properties used for the classification of dyes. Dyes can be more systematically classified based on the chromophore present in these chemicals. These include the acridine dyes, azo dyes, arylmethane dyes, anthraquinone dyes, nitro dyes, xanthene dyes, and quinine–amine dyes. The azo compound class accounts for 60–70% of all dyes used in various applications. These dyes contain an azo group (–N
N–) which links two sp2 hybridized carbon atoms. Often, these carbons are part of aromatic systems, but this is not always the case. Tautomerism in these molecules would arise by the removal of hydrogen from one part of the molecule, and the addition of hydrogen to a different part of the molecule (Scheme 1, 7 → 8). This is common when there is a –OH group ortho or para to the azo group. The azo dyes can be further classified as monoazo (Acid Orange 7, Orange G, Methyl Red, etc.), diazo (Congo Red, Trypan Blue), and triazo (Direct Blue 71, Chlorantine Fast Green BLL).
Spectroscopic investigations on keto–enol tautomerism in the ground and excited states have attracted much interest during the last few years. The fact that the equilibrium involved is sufficiently slow to permit keto and enol tautomeric forms to be detected, has allowed many researchers to work on these processes. Synthetic and naturally tautomeric species of dyes have found wide applications in various fields of science and technology such as optical switches, laser technology, telecommunication, optical signal processing, molecular data processing, ion sensors and biological applications. In this paper, we present the literature findings within the last few years (2010–2014) on the spectroscopic studies of tautomeric behavior of various azo dyes. The dyes are categorized based on their main functional group as follows:
2.1 Hydroxynaphthalene-based azo dyes
The dyes which have one or more azo groups (–N
N–) substituted with benzene or naphthalene groups and contains many different substituents fall in this class of dyes. A typical example of such a dye is Acid Orange 7, which contains both the benzene and naphthalene groups and substituents on the ring structure. Literature findings on tautomeric behavior in this class of dyes are presented below.
In a study of three monoazo hydroxynaphthalene dyes, the relative predominance of the azo–hydrazone tautomerism (Scheme 2, 9 → 10) has been reported in binary solvent mixtures.13 The azo tautomer was found to predominate when it became easier for the less polar component of the binary mixture with DMF to protonate the dye and thereby permitting full hyper conjugation in the dyes. The hydrazone tautomer, however, predominates when factors such as distortion of the conjugation, reduced basicity and dipolarity are present. The azo–hydrazone tautomerism was further used as probe to determine the relative formation of H-bonding solvated complexes in binary solvent mixtures.
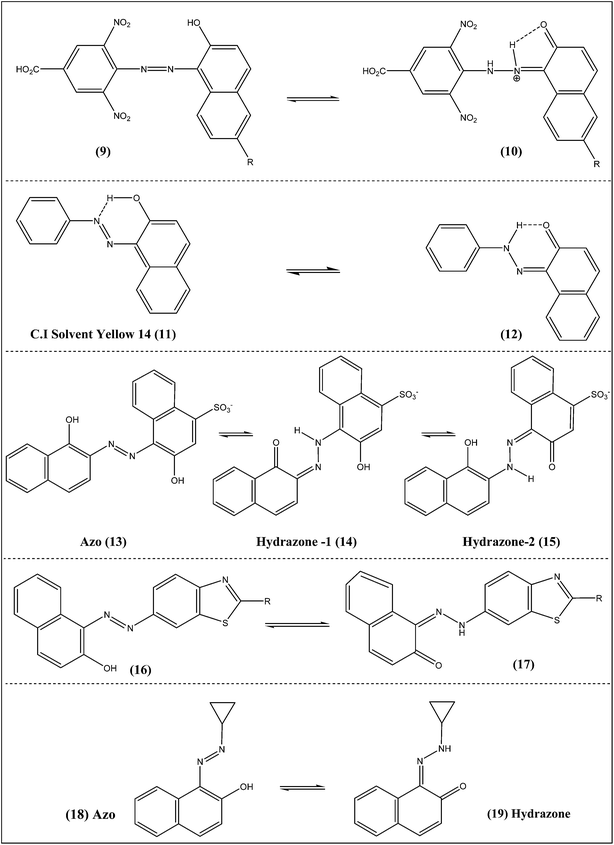 |
| Scheme 2 | |
Azo–hydrazone tautomerism in Acid Orange 7 and C.I Solvent Yellow 14 (Scheme 2, 11 → 12) was modeled by using density functional theory (DFT).14 In the gas phase, the most stable tautomer is the azo compound for model compounds with a phenyl group. However, the hydrazone form is more stable for azo dyes Acid Orange 7 and Solvent Yellow 14 when the naphthyl group replaces the phenyl. Sulfonate group substitution increases the solubility of the compound in water, whereas it does not affect the stability trends of the tautomers. Changes in structural factors during proton-transfer process provided insight in the azo–hydrazone tautomerism. Specific solute–solvent interactions such as hydrogen bonding of the tautomeric oxygen with solvent molecules affects the intramolecular hydrogen bonding most, hence enhancing or decreasing the stability of a certain tautomer but never altering the direction of stability.
The azo–hydrazone tautomeric equilibrium of Eriochrome Blue Black B (1-(1-hydroxy-2-naphthylazo)-2-naphthol-4-sulphonic acid), EBB (Scheme 2, 13 → 14 → 15), was investigated by using UV-visible absorption spectroscopy and DFT calculations.15 The calculated results of relative energies and mole fraction for three tautomers showed that the azo tautomer was the only stable form of EBB in gas phase. The pH-dependence of the UV-vis absorption spectra indicated that the EBB compound existed in acid–base, azo–hydrazone and aggregation equilibria in aqueous solution. Azo–hydrazone tautomer formation was observed in DMF and water solutions which have different dielectric constants. This behavior indicated that specific solute solvent interactions such as H-bonding and basicity effects played major role in the tautomerization and aggregation of EBB. The UV-vis absorption spectra of EBB were studied in DMF solutions at different temperatures. The changes in the absorption spectra showed that the absorbance of azo–hydrazone dye forms was decreased with increasing temperature. The most probable explanation is that increasing the temperature of EBB solution increased the charge delocalization, reduced the intermolecular hydrogen bonding, and consequently assisted dimer dissociation. At low concentrations, the less stable intermolecular hydrogen bonding converted to the intramolecular hydrogen bonding.
Single-crystal X-ray diffraction, NMR and DFT calculations were used to investigate the tautomeric behavior of 6-[(2-hydroxy-1-naphthyl)diazenyl]-2-methylbenzothiazole, and 6-[(2-hydroxy-1-naphthyl) diazenyl] benzothiazole (Scheme 2, 16 → 17).16 The X-ray data showed that crystals of the azo dye derived from 2-methylbenzothiazole were mainly the azo form. All three approaches showed that in solution the equilibrium is only slightly shifted towards the hydrazone form. It was also observed that the electron-donating substituents decreased the value of the equilibrium constant, moving the position of the equilibrium towards the azo form.
The azo derivative 1-(cyclopropyl)diazo-2-naphthol (Scheme 2) was isolated in low-temperature cryogenic matrices, and its molecular structure, tautomeric equilibrium, and photochemical transformations were characterized by infrared spectroscopy and theoretical calculations.17 Only azo–enol forms (18) having the OH group involved in a strong intramolecular hydrogen forming a six-membered ring with the azo group, were found experimentally. Irradiation with a narrowband source in the near-UV range generates different rotameric and tautomeric azo–enol and keto–hydrazone forms (18 → 19) that can be interconverted at different irradiation wavelengths.
The X-ray structure for Sudan I (Scheme 3) showed the presence of the NH tautomer in the solid state; weak intermolecular interactions were observed, such as π-stacking and hydrogen bonding of the unconventional type N–H⋯O.18 For all derivatives studied, the infrared and Raman spectra were suggested that the hydrazone form (21) was predominant. The analysis in solution by NMR spectroscopy supported the presence of NH isomer. All the spectroscopic and theoretical results showed that the Sudan family of compounds presented the hydrazo structure (21) as the prevalent, as compared to the azo form (20), mainly in highly polarizable solvents.
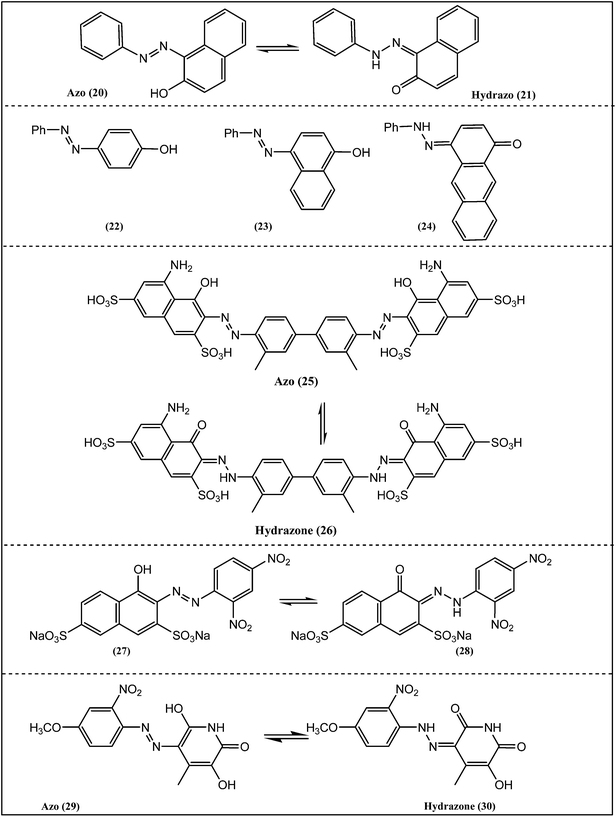 |
| Scheme 3 | |
Electron ionization-mass spectrometry (EI-MS) technique was used to estimate the tautomeric forms of a series of azo dyes (Scheme 3: 22, 23 and 24).19 The gas-phase tautomerism in 4-phenylazo-1-phenol, 4-phenylazo-naphthalen-1-ol and 4-phenylazo-anthracen-1-ol has been studied. The peaks in the mass spectra were assigned to the individual tautomeric forms by analogy with the fragmentation of –OCH3 and –NCH3 substituted tautomers of 4-phenylazonaphthalen-1-ol. The tautomeric equilibrium in 4-phenylazo-1-phenol fully shifted to the enol tautomer. Benzene ring annelation is known to increase the amount of the keto form of the ortho-hydroxy analogues of the compounds studied, and the same behavior was observed for the compounds in the current review. However, the results for the tautomeric ratio in 4-phenylazo-anthracen-1-ol have shown that the amount of the enol tautomer is dominant. This result was also indirectly confirmed by flash photolysis measurements in benzene.
2.2 Trypan Blue/Nitrazine Yellow azo dyes
The tautomeric equilibrium of Trypan Blue (Scheme 3, 25 → 26) was investigated in several solvents and it was found that the keto form dominated in all cases.20 DFT calculations supported this and it was suggested that the preference arises largely from internal hydrogen bonding between the hydrazone and sulfonate groups as shown in Fig. 1.20 The calculations also indicated that the two visible absorptions observed experimentally arose from a single species in solution, rather than the presence of keto and enol tautomers. Use of the full structure of Trypan Blue in calculations, as compared to models, correctly predicted the keto form as dominant which was also confirmed by 13C NMR in DMSO-d6 as shown in Fig. 2.20
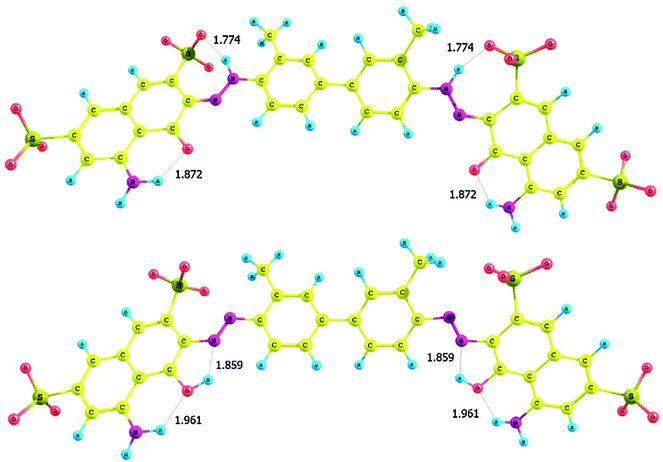 |
| Fig. 1 Optimized structures of Trypan Blue (keto form is shown above enol form).20 | |
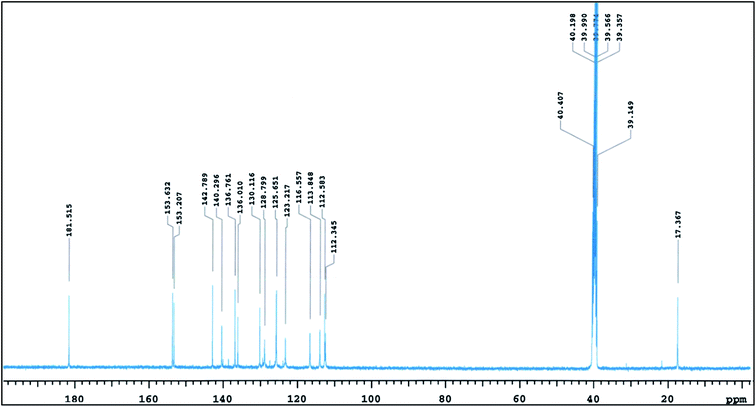 |
| Fig. 2 13C NMR spectrum of Trypan Blue in DMSO-d6.20 The spectrum depicts the keto form of the dye (Scheme 3, 26). | |
Halochromism of Nitrazine Yellow in solution was found to originate from azo–hydrazone tautomerism (Scheme 3, 27 → 28).21 In neutral pH, the hydrazone tautomer was more stable while in basic solution, bathochromic shift was observed which was attributed to the azo tautomer. The tautomerism was also studied in polyamide matrices and the equilibrium was affected by the polymeric environment resulting in a shift. The halochromism of Nitrazine Yellow was present in all cases and was based on an azo–hydrazine tautomerism which was altered by polyamide matrices.
2.3 Pyridone-based azo dyes
The azo–hydrazone tautomerism of two pyridine-2,6-dione based Disperse Yellow dyes (Scheme 3, 29 → 30) are sensitive to pH changes and metal-ion complexation. This was observed by UV-visible spectra using pH-titration, NMR and X-ray single-crystal diffraction techniques for two dyes and one neutral dinuclear dye–metal complex.22 The hydrazone form for the two dyes in solution was found to be dominant under both acidic and basic conditions. Proton NMR further confirmed the presence of hydrazone form, which was verified by the hydrogen–deuterium exchange. X-ray single-crystal structures also revealed the presence of cooperative six-membered intramolecular hydrogen rings involving the hydrazone proton. Moreover, the azo–hydrazone tautomerism was evidenced by the formation of a novel neutral dinuclear dye–metal complex Cu2(L2−A)4.
A study of pyridone dyes with alkyl and aryl groups in ortho position to azo group showed2-pyridone/2-hydroxypyridine tautomerism (Scheme 4, 31 → 32), while those containing OH and NHR groups conjugated with the azo group showed azo–hydrazone tautomerism.23 The equilibrium between two tautomers is influenced by the structure of the compounds and by the solvents used. The tautomeric behavior pattern of the arylazo pyridone dyes has been studied using various instrumental techniques, such as FT-IR, UV-vis, and NMR. The quantum chemical calculations related to the azo–hydrazone tautomerism have also supported the findings. The study also showed that a large number of pyridone dyes exists in hydrazone form in solid state, using X-ray single-crystal diffraction, whereas in solvents there is a mixture of tautomers. Substituted arylazo pyridone dyes were synthesized and characterized using UV-vis spectrophotometry and DFT calculations.24 Tautomeric and acid–base equilibrium of the investigated dyes (Scheme 4: 33, 34, 35 and 36) were found to be dependent on the phenyl ring substituents. The substituents exerted stronger effect on the conjugation between azo bond and the pyridone ring than on the conjugation between azo bond and the benzene ring. The absorption spectra showed that all dyes existed in the hydrazone form in acidic media, while in the basic solution, the anion form was dominant. The hydrazone form of the anion (36) was more favorable with electron-withdrawing groups, while anionic form of the enol (35) was predominant with electron-donating groups. DFT calculations correctly predicted the effects of the substituents on the position of the absorption maxima. The results also showed that pKa values were strongly related to the electronic structure of dyes, showing good correlations with Hammett constants.
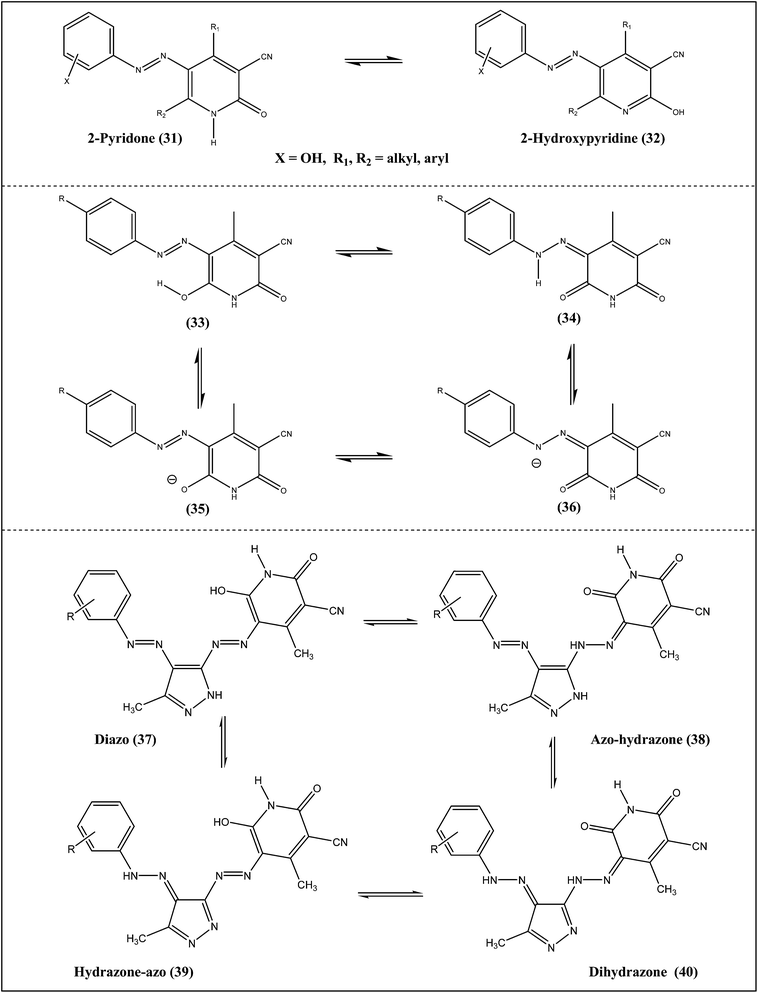 |
| Scheme 4 | |
Pyridone-based disperse disazo dyes can exist in four possible tautomeric forms (Scheme 4), namely the disazo–enol form (37), the azo–hydrazoketo form (38), the hydrazo–azo–enol form (39) and the dishydrazoketo form (40).25 The deprotonation of tautomeric forms leads to a common anion and their FTIR spectra suggested that these are predominantly in azohydrazo–keto form (38) or dihydrazo–keto form (40). Numerous investigations were carried out to establish the tautomeric structure of azo pyridone in the solid state using a variety of spectroscopic techniques. The spectral data generally lead to the conclusion that the tautomeric equilibrium of the azo pyridone dyes is in favor of the hydrazone form in the solid state. Based on NMR spectra it was found that the dye were present in a single tautomeric form in DMSO.
The solvatochromic behavior and tautomeric structures of some bis-heterocyclic monoazo dyes (Scheme 5) in various solvents were evaluated.26 These dyes can exist in three possible tautomeric forms, namely the azo–keto forms (41), the azo–enol forms (42) and the hydrazo–keto forms (43). Acid–base influences on the wavelength of maximum absorption of these dyes were also studied. Most of the dyes showed bathochromic shifts in most polar solvents, such as DMSO and DMF. It was also observed that the absorption spectra of dyes in methanol and chloroform were quite sensitive to the addition of base. These results suggest that the dyes are present in a different tautomeric form in basic mediums and DMSO, DMF than that in acetonitrile, methanol, acetic acid and chloroform.
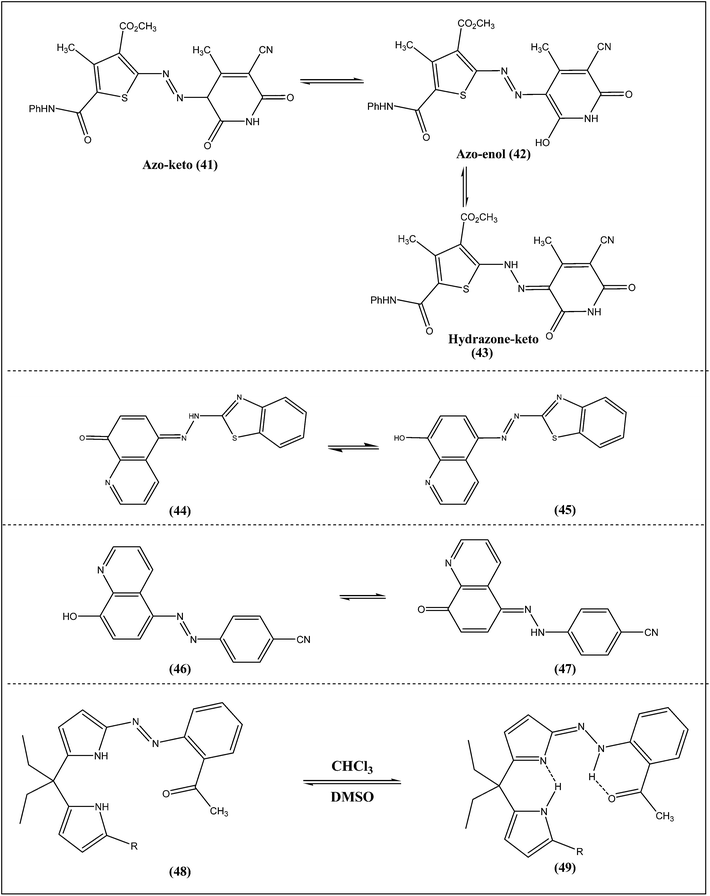 |
| Scheme 5 | |
2.4 Hydroxyquinoline-based azo dyes
The absorption and emission spectra of three 5-(2-benzothiazolylazo)-8-hydroxyquinoline dyes with different substituents were examined in a series of liquid and liquid crystalline solvents.27 The dyes showed azo/hydrazone tautomerism (Scheme 5, 44 → 45) which was influenced by the solvent properties. The results showed the influence of substituents on the aromatic skeleton in these compounds. An electron donor substituent (–OCH3) in the benzothiazol ring of the tautomeric system shifted the tautomeric equilibrium towards the azo form, while introduction of an electron acceptor substituent (–NO2) moved the tautomeric equilibrium towards the hydrazone form.
Tautomeric behaviors of some azoquinoline dyes (Scheme 5, 46 → 47) in liquid and liquid crystalline media were investigated.28 The spectral behavior of the compounds and the ratio of azo/hydrazone mixture were largely influenced by the position of the electron-attracting group. The experimental result indicated that the strong azo/hydrazone tautomerism occurred in some polar solvents such as DMF. This phenomenon was more evidenced for m-azoquinoline. However, in solvents of low polarity, the azo form dominated. Interestingly, the compounds appeared to exist almost entirely in its azo form in the anisotropic media (both the polar and low polar). It was concluded that the anisotropic solvents provided relatively rigid, packed and polarizable media for the dye molecules, and therefore, prevent azo/hydrazone tautomerism.
2.5 Pyrrole-based azo dyes
Three azopyrrole compounds were synthesized and their structures were determined by single crystal X-ray diffraction.29 The compound 2-(2-acylphenyl)diazopyrrole adopts the azo tautomeric form, whereas, 5,5′-bis(2-acylphenyl) diazo-dipyrromethane and 5-(2-acylphenyl) diazo-dipyrromethane crystallized in the hydrazone form. The crystal structures revealed that intramolecular hydrogen bonds play an important role in the azo–hydrazone tautomeric equilibrium of azopyrroles. The azopyrrole tautomerism is affected by the presence of hydrogen bond acceptor on the benzene ring to the ortho-position of azo linkage. Azo–hydrazone tautomeric equilibria (Scheme 5, 48 → 49) of these compounds were further confirmed by NMR and UV-vis spectral studies in solution. Compounds mainly adopt hydrazone form (49) tautomer in apolar solvent (CHCl3), whereas, azo form tautomer (48) in polar solvents (DMSO) is more, in which intramolecular hydrogen bonds are destroyed by interaction with the solvent.
2.6 Morpholine–thiazole-based azo dyes
Novel yellow azo dyes were synthesized by diazotization of aromatic amines followed by coupling with 2-morpholin-4-yl-1,3-thiazol-4(5H)-one and fully characterized.30 The geometries of the synthesized dyes for azo and hydrazone tautomeric forms (Scheme 6, 50 → 51) were optimized and their electronic excitation properties were evaluated using density functional theory (DFT). The optimized geometries reveal that the hydrazone form is more stable than the azo form. Photophysical properties of the synthesized dyes were evaluated by UV-visible spectroscopy and compared with computed vertical excitation. The results clearly illustrate that the position of electron withdrawing groups affects the tautomeric equilibrium: in the para position the hydrazone tautomeric form is dominant, whereas, in the ortho position, both azo and hydrazone forms exist.
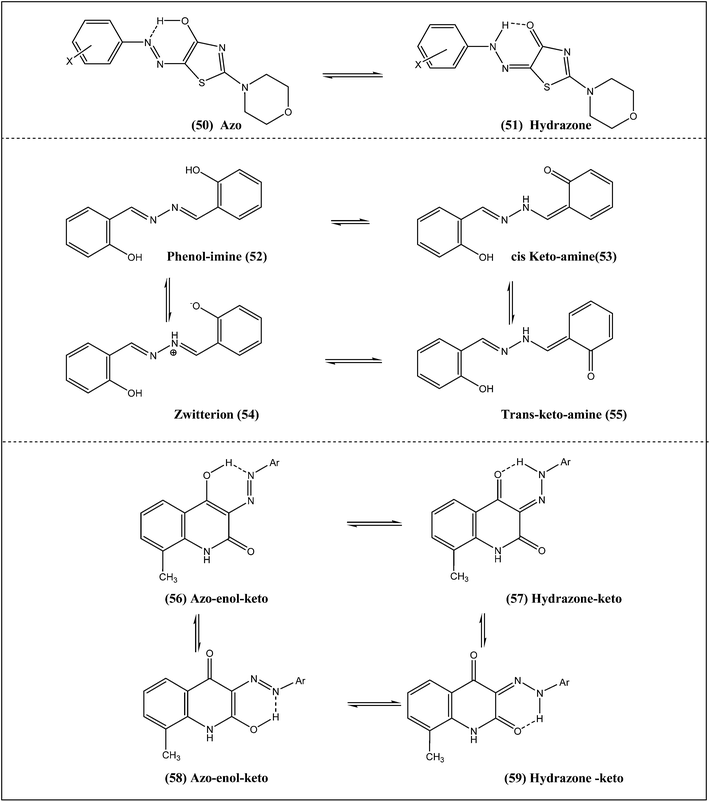 |
| Scheme 6 | |
2.7 Salicylaldehyde-based azo dyes
Absorption and emission spectra of salicylaldehyde azo and related Schiff bases with similar molecular structures (Scheme 6) but different functional groups were studied in solvents of varying polarities.31
The enol and keto anion structures of salicylaldehyde azine (Scheme 6: 52, 53, 54 and 55) in solvents of different viscosities and polarities were studied using femto to nanosecond time-resolved emission techniques.32 In the enol form, an ESIPT reaction occurs (time constant < 80 fs) to produce an excited keto tautomer in which intramolecular-vibrational energy redistribution and vibrational cooling occurred. The viscosity-dependent emission at higher wavelength of the spectrum (5–11 ps) reflects a twisting motion leading to rotamers of these keto structures which were assigned to n → π* transition.
In another work, the steady-state and femtosecond to nanosecond time-resolved spectroscopic measurements of salicylaldehyde azine (Scheme 6) in dichloromethane within the cages of the faujasite zeolite (NaX) was measured.33 In NaX zeolite cage, a zwitterionic (54) form is present both at S0 and S1 states, and a large hypsochromic shift of the stationary emission spectrum was observed. The increase in fluorescence lifetime upon encapsulation (from 54 ps to 0.2–2.8 ns) is mainly due to hindrance in twisting motion of the confined (54) imposed by the nanocage. A significant dependence of the lifetimes on the guest concentration inside the zeolite indicated an interaction between neighboring guest molecules leading to a quenching of the fluorescence.
2.8 Azoquinololin-based azo dyes
Arylazoquinololin-2-one dyes can exist as a mixture of four tautomeric forms (Scheme 6), namely the azo–enol–keto (56), hydrazone–keto (57), azo–enol–keto (58) and hydrazone–keto (59).34 The infrared spectra of all the compounds (in KBr) suggested that these compounds do not exist in the hydrazone–keto form in solid state. However, in DMSO, proton NMR revealed the presence of hydrazone–keto forms (57) and (59). Proton NMR studies eliminated the possibility of tautomers involving ring NH rearrangement. The presence of two broad singlets provided firm evidence for presence of the amide bonds and is related to amide protons of two types of tautomeric forms (57) and (59). Hence it was concluded that in DMSO, the tautomeric equilibrium of the arylazoquinolone dyes is a mixture of two hydrazone–keto forms (57) and (59).
2.9 Benzoylacetone-based azo dyes
NMR studies of azo derivatives of benzoylacetone indicated that these compounds existed as a mixture (Scheme 7) of hydrazo (60) and Z-enol–azo (61) forms.35 Chemical shift of the hydrazo NH proton indicated its involvement in hydrogen bonding with a carbonyl group, which was further confirmed by the separation of the two carbonyl resonances in the 13C NMR spectrum. On the other hand, the 1H NMR spectra of revealed the OH resonance of the Z-enol–azo (61) form in DMSO-d6 solution, while in the 13C NMR spectra the Z-enol–azo (61) C
O resonance appeared in the higher field range. The data indicated that the tautomeric equilibria depends on the solvent and on the substituents in the phenyl ring. Thus, the tautomeric balance shifts to the hydrazo (60) form with decrease in the solvent polarity (from DMSO to methanol) and also depends on the electron-withdrawing properties of the phenyl ring substituent. Moreover, the third tautomeric form, namely the keto–azo, was not detected in solution under any experimental conditions, presumably due to its lower stability in comparison with the others.
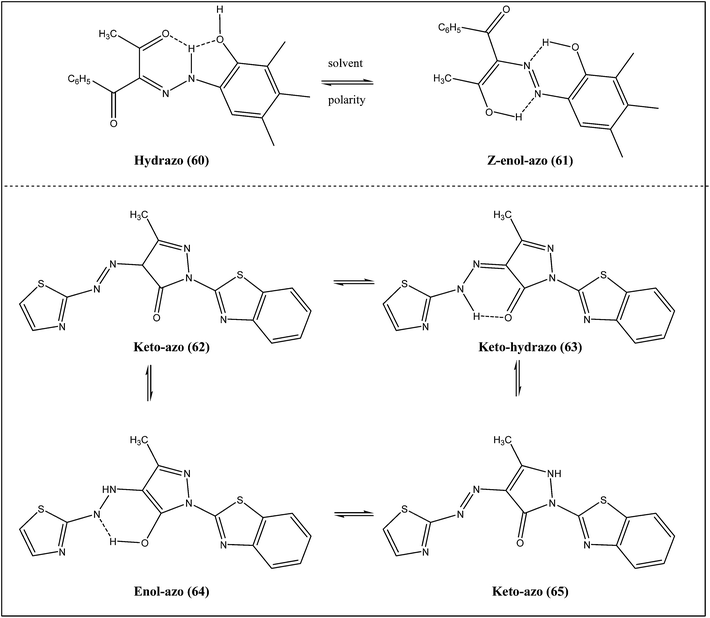 |
| Scheme 7 | |
2.10 Hetarylazopyrazolone dyes
Hetarylazopyrazolone dyes (Scheme 7) can exist as four different tautomeric forms (Scheme 7: 62, 63, 64 and 65). Spectrophotometric studies of a series of hetarylazopyrazolone dyes indicated that most dyes existed in only one tautomeric form except one which showed two absorption bands in both DMF and DMSO.36 The isosbestic points in absorption spectra of the dyes indicated the existence of an equilibrium. This equilibrium may exist either between the tautomeric forms or between the anionic form and one tautomeric form. Addition of base led to no change in the wavelengths and this concluded that the dyes totally ionized in methanol, DMF and DMSO. Such effects of these solvents demonstrated that ionization occurred rather than azo–anion tautomerism.
3 Factors affecting tautomerism in azo dyes
Reading the preceding sections one could conclude that the following factors play a major role in tuning the tautomeric equilibrium between the enol and keto forms in azo dyes in particular: hydrogen bonding, nanocaged effects, and crystallization. However, the reader is advised to read more general reviews and textbooks frequently found in literature on other factors that affect tautomerism such as conjugation and changes in the aromaticity, etc. Tautomerism in azo dyes is not controlled by one factor but in fact is dependent on many factors at the same time. Some typical examples are highlighted below:
3.1 Hydrogen bonding
Intramolecular hydrogen bonding involving the hydrazone protons stabilize the keto form. However, the enol form is stabilized through forming an intermolecular hydrogen bonding between two units. Factors that enforce or destroy such intramolecular hydrogen bonding are pH of the media, solvent polarity, temperature, substituents, and concentration of the azo dyes. For example, changing the solvent polarity causes the change in hydrogen bonding leading to change in tautomerism as shown in Scheme 2, 9 → 10 and Scheme 5, 48 → 49.
3.2 Effect of solvents
Solvent can play an important role in the relative stability of the tautomeric forms. For example polar solvents promote intermolecular type of bonding between solvent and dye and thus favors the keto form, whereas the enol form is more stable in nonpolar solvents. An example of this is shown in Scheme 2, 9 → 10, Scheme 3, 20 → 21 and Scheme 5, 48 → 49.
3.3 Effect of temperature
Keto–enol tautomerization are known to be temperature dependent. An example is the change in the tautomeric form of EBB as shown in Scheme 2, 13 → 14 → 15. High temperatures impart high kinetic energy to the solvent molecules which disrupt the intermolecular hydrogen bonding between the solvent and the dye molecules thereby increasing the intramolecular forces in the probe molecule. Thus at high temperatures, the keto form is favored.
3.4 Effect of pH
Acidic–basic nature of the dye solution will have a profound effect on the tautomeric form of the dye. At high pH values, the labile protons usually associated with hydrogen bonding are removed thus favoring the enolate form, whereas in neutral solution the keto form was observed. This is depicted for azodyes in Scheme 3, 27 → 28 and Scheme 4, 33 → 34 → 35 → 36.
3.5 Effect of substituents
The electron donating and electron withdrawing properties of various substituents can stabilize a particular tautomeric form of a dye molecule either through inductive or resonance effect. Substituting an electron donating group in an azo dye will cause an increase in the electron density in the dye skeleton thereby favoring the enol form. On the other hand, inserting an electron withdrawing group will decrease the electron density in the dye skeleton and the equilibrium is shifted towards the keto form (Scheme 2, 16 → 17, Scheme 3, 22 → 23 → 24, Scheme 5, 44 → 45, 46 → 47, Scheme 6, 50 → 51).
3.6 Nanocaged effects
This effect is mainly observed when dye molecules reside in a cavity. Encapsulation of dye molecule inside some nanocaged microheterogenous systems created a rigid environment for the probe molecule that stabilizes mostly the enol form as shown in Scheme 6, 52 → 53 → 54 → 55.
3.7 Crystallization
Azo dyes in the solid state have restricted motion and the major forces affecting the tautomeric form is mostly van der Waals' forces due to large π–π stacking interactions. Some studies of azo dyes in solid state revealed the isolation of enol form as shown in Scheme 2, 16 → 17 based on the above observation. On the other hand many cases favor the keto form of the dye in the solid state as shown in Scheme 3, 20 → 21, Scheme 4, 31 → 32, Scheme 5, 48 → 49.
4 Analyzing tautomeric forms of azo dyes using selective techniques
There are several experimental techniques which have been employed to study keto–eonl tautomerism in azo dyes. The underlying principle is to monitor the exchange of a proton between two or more forms when the solute molecule undergoes such change. Different spectroscopic methods such as UV-vis, FTIR, NMR spectroscopy, Mass spectrometry and single. X-ray analyses are commonly used methods for studying tautomerism.
4.1 UV-vis spectroscopy
This is the one of the most popular techniques used to probe keto–enol tautomerism in various molecules. The technique has also been employed for this purpose in azodyes. This is because of the reason that both keto and enol form will absorb at different wavelengths, as a result of which peaks belonging to each form can be distinguished and can also be quantified if proper standards are available. Scheme 5, 44 → 45 illustrates the use of the technique for monitoring the keto and enol form of azoquinoline dyes in various solvents. In general, the equilibrium is shifted to the keto form as the solvent polarity is increased as shown in Fig. 3.27
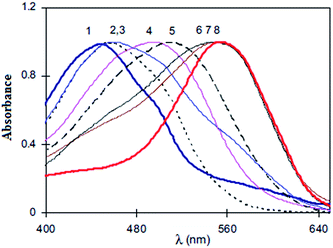 |
| Fig. 3 Absorption spectra of azoquinoline dye in cyclohexane (1), benzene (2), acetone (3), DMSO (4), benzyl alcohol (5), acetonitrile (6), methanol (7) and DMF (8). Reprinted from ref. 27 with permission from Elsevier. | |
4.2 FTIR spectroscopy
This technique is versatile in detecting functional groups in molecules in various matrices. Since keto and enol have distinct stretching frequencies, it can be utilized for monitoring the tautomeric forms. An example of using this technique was used by researchers to distinguish the various forms of Pyridone-based disperse disazo dyes (Scheme 4, 37 → 40). The FT-IR spectra of dye showed three imino bands (NH) at 3262–3117 cm−1 and two carbonyl (C
O) bands at 1697–1660 cm−1. However, the FT-IR spectra of dye did not show any broad band for hydroxyl group. This suggested that the dye is present predominantly in keto form in the solid state.
4.3 NMR spectroscopy
This technique is capable of monitoring the chemical shifts in real time. This property can be used to distinguish between the two tautomeric forms in azo dyes, The method was used to probe the two forms of azo derivatives of benzoylacetone. Scheme 7, 60 → 61 shows the two forms of the compound studied using 1H NMR. The NMR spectrum is shown in Fig. 4.35 The hydrazo NH proton appeared downfield as compared to the OH resonance of the Z-enol–azo form. The relative abundance of tautomeric forms was determined by the integration of the methyl group of each tautomer. The data indicated that the tautomeric equilibria depended on the solvent and on the substituents in the phenyl ring.
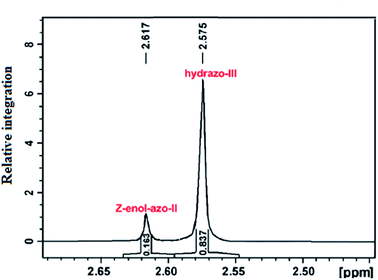 |
| Fig. 4 NMR determination of the tautomeric ratio for azoderivatives of benzoylacetone in CD3OD. Reprinted from ref. 35 with permission from Elsevier. | |
4.4 Mass spectrometry
Mass spectrometry (MS) can be used to estimate tautomeric equilibrium in the gas phase. The method is advantageous as it is not influenced by external factors like solvents and intermolecular interactions. The distinctive fragmentation pattern can be used to probe the tautomeric forms. The MS technique was used by researchers to distinguish the tautomeric forms of three phenylazo dyes as shown in Scheme 3: 22, 23, and 24. In all the three compounds under investigation,19 the parent ion could not be used to distinguish between the tautomeric forms. However, the consecutive fragments/daughter ions were of informative value for assigning the tautomeric forms.
4.5 X-ray technique
X-ray crystallography is a useful method to study tautomerism. The method is suitable for solids and provide valuable information about the conformation and spatial distribution of atoms, from which exact bond distances can be obtained. The method was used to study the azo–hydrazone tautomerism of azopyrrole in the solid state. Scheme 5, 48 → 49 shows the two forms of the compound under investigation. The azo and the hydrazone forms in the crystal structure as shown in Fig. 529 were distinguished by analyzing the –N–N– bond length which is shorter in the case of azo form (48) as compared to the hydrazone form (49). The crystal structure of azopyrole shows both azo and hydrazone form as a pair. In this compound, the N(5)–N(6) bond distance was 1.274 Å, whereas N(2)–N(3) bond is 1.330 Å. Moreover, the C(3)–C(4) = 1.342 Å and C(2)–N(1) = 1.318 Å bonds showed double bond characterization and C(2)–C(3) = 1.465 Å and C(1)–C(4) = 1.446 Å bonds showed single bond characterization. The longer N–N bond distance and pyrrole ring distortion in the compound indicated that one azopyrrole arm of the compound is in hydrazone form and the other is in azo form.
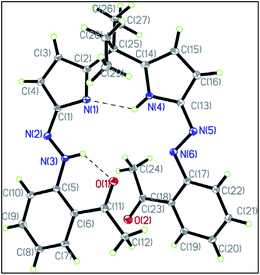 |
| Fig. 5 X-ray crystallography of azopyrrole showing tautomerism in the solid state. Reprinted from ref. 29 with permission from Elsevier. | |
5 Summary
Synthetic and natural tautomeric species of azo dyes have found wide applications in various fields of science and technology. For instance, they are in used optical switches, laser technology, telecommunication, optical signal processing, molecular data processing, ion sensors and biological applications. With the advancement of knowledge, new azo dyes showing tautomerism are being designed for specific uses. This review provides a non-exhaustive compilation of studies of various azo dyes which can exist in tautomeric forms. A good understanding of these can lead to the design of azo dye molecules with targeted properties for applications in specific areas.
References
- Tautomerism: Methods and Theories, ed. L. Antonov, Wiley-VCH, 2013 Search PubMed.
- H. Roohi, F. Hejazi, N. Mohtamedifar and M. Jahantab, Spectrochim. Acta, Part A, 2014, 118, 228–238 CrossRef CAS PubMed.
- Q. Huang, X. F. Yang and H. Li, Dyes Pigm., 2013, 99, 871–877 CrossRef CAS PubMed.
- J. Mech, M. A. Grela and K. Szaciłowski, Dyes Pigm., 2014, 103, 202–213 CrossRef CAS PubMed.
- X. Liu, L. Chen, Q. Zhou, X. Zhou and S. Liu, J. Photochem. Photobiol., A, 2013, 269, 42–48 CrossRef CAS PubMed.
- N. A. Carmona, B. Cohen, J. A. Organero and A. Douhal, J. Photochem. Photobiol., A, 2012, 234, 3–11 CrossRef CAS PubMed.
- R. I. Bakalska and V. B. Delahey, Acta Chim. Slov., 2012, 59, 75–82 CAS.
- V. Sauri, J. P. Gobbo, J. J. S. Perez, M. Lundberg, P. B. Coto, L. S. Andres, A. C. Borin, R. Lindh, M. Merchan and D. R. Sanjuan, J. Chem. Theory Comput., 2013, 9, 481–496 CrossRef CAS.
- Laser Chemistry: Spectroscopy, Dynamics, and Applications, ed. H. H. Telle, A. G. Urena and R. J. Donovan, Wiley, 2007 Search PubMed.
- B. Kutlu, A. Leuteritz, L. Häußler, U. Oertel and G. Heinrich, Polym. Degrad. Stab., 2014, 102, 9–14 CrossRef CAS PubMed.
- A. Ceylan, S. Z. Bas, M. Bayrakcı, S. Ertul and A. Uysal, Acta Chim. Slov., 2012, 59, 656–663 CAS.
- A. R. Todorov, M. Nieger and J. Helaja, Chem.–Eur. J., 2012, 18, 7269–7277 CrossRef CAS PubMed.
- O. A. Adegoke and O. S. Idowu, Spectrochim. Acta, Part A, 2010, 75, 719–727 CrossRef PubMed.
- A. S. Ozen, P. Doruker and V. Aviyente, J. Phys. Chem. A, 2007, 111, 13506–13514 CrossRef PubMed.
- A. Unal, B. Eren and E. Eren, J. Mol. Struct., 2013, 1049, 303–309 CrossRef CAS PubMed.
- L. Racane, Z. Mihalic, H. Ceric, J. Popovic and V. T. Kulenovic, Dyes Pigm., 2013, 96, 672–678 CrossRef CAS PubMed.
- L. Duarte, B. M. Giuliano, I. Reva and R. Fausto, J. Phys. Chem. A, 2013, 117, 10671–10680 CrossRef CAS PubMed.
- G. R. Ferreira, H. C. Garcia, M. R. C. Couri, H. F. Dos Santos and L. F. C. de Oliveira, J. Phys. Chem. A, 2013, 117, 642–649 CrossRef CAS PubMed.
- D. Nedeltcheva, V. Kurteva and I. Topalova, Rapid Commun. Mass Spectrom., 2010, 24, 714–720 CrossRef CAS PubMed.
- J. P. Graham, M. A. Rauf, S. Hisaindee and M. Nawaz, J. Mol. Struct., 2013, 1040, 1–8 CrossRef CAS PubMed.
- L. Van der Schueren, K. Hemelsoet, V. Van Speybroeck and K. De Clerck, Dyes Pigm., 2012, 94, 443–451 CrossRef CAS PubMed.
- X. C. Chen, T. Tao, Y. G. Wang, Y. X. Peng, W. Huang and H. F. Qian, Dalton Trans., 2012, 41, 11107–11115 RSC.
- J. M. Mirkovic, G. S. Uscumlic, A. D. Marinkovic and D. Z. Mijin, Hem. Ind., 2013, 67, 1–15 CrossRef CAS.
- J. Dostanic, D. Mijin, G. Uscumlic, D. M. Jovanovic, M. Zlatar and D. Loncarevic, Spectrochim. Acta, Part A, 2014, 123, 37–45 CrossRef CAS PubMed.
- F. Karci, F. Karci, A. Demirçali and M. Yamaç, J. Mol. Liq., 2013, 187, 302–308 CrossRef CAS PubMed.
- F. Karci and F. Karci, J. Mol. Struct., 2012, 1024, 117–122 CrossRef CAS PubMed.
- A. G. Gilani, M. Moghadam, M. S. Zakerhamidi and E. Moradi, Dyes Pigm., 2012, 92, 1320–1330 CrossRef PubMed.
- A. G. Gilani, E. Moradi, S. Binay and M. Moghadam, Spectrochim. Acta, Part A, 2012, 87, 112–118 CrossRef PubMed.
- J. Chen and Z. Yin, Dyes Pigm., 2014, 102, 94–99 CrossRef CAS PubMed.
- P. G. Umape, V. S. Patil, V. S. Padalkar, K. R. Phatangare, V. D. Gupta, A. B. Thate and N. Sekar, Dyes Pigm., 2013, 99, 291–298 CrossRef CAS PubMed.
- M. S. Zakerhamidi, K. Nejati, S. Golghasemi Sorkhabi and M. Saati, J. Mol. Liq., 2013, 180, 225–234 CrossRef CAS PubMed.
- M. Ziolek, M. Gil, J. A. Organero and A. Douhal, Phys. Chem. Chem. Phys., 2010, 12, 2107–2115 RSC.
- M. Gil, M. Ziolek, J. A. Organero and A. Douhal, J. Phys. Chem. C, 2010, 114, 9554–9562 CAS.
- E. O. M. Rufchahi, H. Pouramir, M. R. Yazdanbakhsh, H. Yousefi, M. Bagheri and M. Rassa, Chin. Chem. Lett., 2013, 24, 425–428 CrossRef PubMed.
- K. T. Mahmudov, R. A. Rahimov, M. B. Babanly, P. Q. Hasanov, F. G. Pashaev, A. G. Gasanov, M. N. Kopylovich and A. J. L. Pombeiro, J. Mol. Liq., 2011, 162, 84–88 CrossRef CAS PubMed.
- E. Aktan, N. Ertan and T. Uyar, J. Mol. Struct., 2014, 1060, 215–222 CrossRef CAS PubMed.
|
This journal is © The Royal Society of Chemistry 2015 |
Click here to see how this site uses Cookies. View our privacy policy here.