DOI:
10.1039/C4RA16098C
(Paper)
RSC Adv., 2015,
5, 14631-14636
Simultaneous extraction of bisphenol A and tetrabromobisphenol A from milk by microwave-assisted ionic liquid microextraction†
Received
13th December 2014
, Accepted 23rd January 2015
First published on 23rd January 2015
Abstract
Considering bisphenol A (BPA) and tetrabromobisphenol-A (TBBPA) are ubiquitous environmental pollutants and widely manufactured into packaging materials, foodstuffs may be polluted by BPA and TBBPA. Additionally, there are very limited analytical methods for determining TBBPA in commercial milk samples. In this study, a rapid, simple and efficient method based on microwave-assisted ionic liquid microextraction (MAILME) combined with high-performance liquid chromatography (HPLC) was first applied to the simultaneous analysis of BPA and TBBPA in commercial milk samples. To achieve the optimum extraction performance, the experimental parameters of the MAILME, including type and amount of ionic liquid, microwave power, extraction time, pH and salt concentration in the sample, were evaluated and optimized. Under the optimum conditions, the detection limits were 0.01 and 0.02 μg L−1, and the quantification limits were 0.03 and 0.05 μg L−1 for BPA and TBBPA, respectively. The proposed method had good repeatability and reproducibility with RSDs lower than 5.6%. The recoveries of the analytes in the aged milk samples varied from 79.8 to 97.3%. The proposed method has been applied successfully to the determination of BPA and TBBPA in commercial milk samples with recoveries ranging from 78.2 to 99.8%. Compared with the other previous methods, the proposed method did not need to remove the fats and proteins in the milk, which made the sample pretreatment simple and rapid (5.0 min). And it also avoided the use of organic solvents. The proposed approach provides a new and promising alternative for simultaneously determining trace amounts of BPA and TBBPA in commercial milk samples or other similar samples.
1 Introduction
Bisphenol A (BPA) and tetrabromobisphenol-A (TBBPA) are two phenolic organic compounds which are extensively used in consumer products.1 BPA is a major industrial product widely used in the production of resins and polycarbonate plastics ranging from plastic bottles to inner coatings of food packages to even feeding-bottles. Tetrabromobisphenol-A (TBBPA) is one of the most important individual brominated flame retardants (BFRs), which has the highest product volume covering around 60% of the total BFRs market.2 The release of BPA and TBBPA into the environment from these products has attracted great attention all over the world because of their estrogenic activity or potential estrogenic activity. It has been proved that BPA can affect endocrine systems and cause adverse effects such as endocrine dyscrasia, precocity and even cancer,3 and TBBPA can lead to potentially toxic endocrine-disruptor effects, immunotoxicity and neurotoxicity.4
Because BPA and TBBPA are widely used, they have been abroad found in environment.5–9 Considering these compounds are ubiquitous environmental pollutants and widely manufactured into packaging materials, foodstuffs may also be polluted by BPA10,11 and TBBPA12 during the treatment processes or by bioaccumulation in foods of animal origin. The milk is an important nutritional food and can provide an essential developmental factor and immunological components for the aged and infants. Therefore monitoring these compounds in milk samples is important to the health and safety of human. The extraction plays a key role in determination of pollutants in environment prior to chromatographic analysis. But it is often difficult to extract pollutant in the presence of fats, lactose and proteins in the samples, such as milk. To date, there are many pretreatment methods for extracting BPA from milk, such as solid phase extraction (SPE),13 matrix solid phase dispersion (MSD)14 and molecularly imprinted solid-phase extraction (MISPE).15–17 Although there were some reports about TBBPA in breast milk,18–20 there is very limited method for extracting TBBPA from commercial milk samples. Shi et al.12 determined TBBPA in human milk samples which were collected in 2007. The human milk samples were freeze-dried, extracted by a Soxhlet apparatus with a mixture of n-hexane and acetone used as the solvent, and then cleaned by gel permeation chromatography (GPC) followed by a sulfuric acid treatment. Shi et al.20 also applied accelerated solvent extraction (ASE) using hexane/acetone as solvent to extract TBBPA from human milk, and then cleaned by GPC followed by a sulfuric acid treatment. However, no method for commercial milk samples has ever been published for TBBPA. Additionally, all of the described methodologies for human milk samples require cleanup steps to decrease the complexity of raw extracts from samples. Moreover, because both BPA and TBBPA are ubiquitous pollutants and widely manufactured into packaging materials, and TBBPA can be transformed to BPA in environmental conditions, thus they may co-exist in environmental matrixes.21–23 Therefore, it is very essential to develop a rapid, simple and accurate method to simultaneously detect BPA and TBBPA in milk samples.
In other hand, efforts have been directed towards miniaturizing the extraction procedure by reducing the solvent to aqueous phase volume ratio in the last few years.24 Dispersive liquid–liquid microextraction (DLLME) is a popular method to extract the pollutants from matrix because of its easy handling, miniaturized sample preparation and cost-effectiveness. Nevertheless, this technology still needs volatile and hazardous organic compounds as solvents or co-solvents, which are harmful to human health and the environment. Ionic liquids (ILs), which are defined as organic molten salts and liquid at or below 100 °C,25 has been proposed as the prominent alternative to organic solvent because of their excellent properties, including negligible vapor pressure, high thermal stability, good solubility and extractability for organic compounds. ILs have been already widely used as solvent in DLLME.26,27 To improve extraction efficiency and reduce extraction time, other extraction technologies, such as ultrasonic extraction (UE) and microwave-assisted extraction (MAE), have been coupled with DLLME. The rapidity, simplicity, low cost of MAE make this technique to be developed into a good alternative to traditional extraction methods and become a popular routine technique in environmental analysis, especially in organic analysis.25 Especially, ILs are a combination of organic cations and anions27 and can efficiently absorb microwave energy.28 Based on the result, microwave-assisted ionic liquid microextraction (MAILME) has been reported to successfully extract the organic compound from water,29,30 milk,24,30 soil31 and plants.32–34 But to the best of our knowledge, there is no report on microwave-assisted ionic liquid microextraction of TBA and TBBPA from milk samples.
In this study, a rapid, simple, effective and sensitive method, MAILME followed by high performance liquid chromatography (HPLC), is proposed to detect BPA and TBBPA in commercial milk samples. Various influential parameters, such as the type and volume of ILs, microwave power, extraction time, pH and ionic strength, were evaluated and optimized. The proposed method was then successfully applied to the determination of BPA and TBBPA in some milk samples.
2 Experimental
2.1 Reagents and materials
Individual standards of BPA and TBBPA (99%) were purchased from Aladdin (Shanghai, China). Standard stock solutions (1.0 mg L−1) were prepared in acetonitrile and stored at 4 °C. 1-Butyl-3-methylimidazolium hexafluorophosphate ([C4MIM][PF6]), 1-hexyl-3-methylimidazolium hexafluorophosphate ([C6MIM][PF6]) and 1-octyl-3-methylimidazolium hexafluorophosphate ([C8MIM][PF6]) with purity of 99% were purchased from Lanzhou Greenchem ILS, LICP, CAS (Lanzhou, China). HPLC-grade acetonitrile was purchased from J.T. Baker (Phillipsburg, NJ, USA). Ultrapure water was prepared by a Milli-Q SP reagent water system (Millipore, Milford, MA, USA). All milk samples were purchased from local large-scale supermarket and stored at 4 °C until analysis was performed.
2.2 Instrumentations
A microwave oven (Midea, Shunde, China) with a 2450 MHz magnetron, which was modified with the addition of a water condenser, was used for MAILME. The whole system was run at atmospheric pressure and could be employed at the maximum power of 700 W. A centrifuge (Shanghai, China) was used for the phase separation. An Agilent 1200 LC system consisting of a quaternary pump, an auto sampler, a vacuum degasser and a diode-array detector and a reversed phase C18 analytical column of 150 × 4.6 mm (Agilent TC-C18), was used for chromatographic evaluation and separation.
2.3 Sample preparation
The fresh spiked milk samples containing BPA and TBBPA (1.0 μg L−1) were prepared by spiking the stock standard solutions into the negative liquid milk samples (milk sample that did not contain any of the target compounds in detectable amount) and shaking for 10 min. The aged spiked samples were prepared the same as the above method. Then the samples were kept in sealed bottle and stored for 1, 7, 14, 21 and 30 days at 4 °C, respectively.
2.4 MAILME procedure
The procedure of microwave-assisted ionic liquid microextraction of BPA and TBBPA from commercial milk samples was shown in Fig. 1. Briefly, 10 mL of fresh spiked milk samples containing 1.0 μg L−1 of target compounds were extracted by MAILME. The vessels were placed into the modified domestic microwave oven system for extraction. The optimum IL and its volume, microwave power, extraction time, pH and ionic strength were systematically studied. After MAILME, the extracts cooled with ice water to room temperature. After centrifugation at 15
000 rpm for 5 min, the upper aqueous phase was removed with a syringe. 50 μL of the residue was diluted to 0.5 mL by acetonitrile to decrease viscosity. Finally, the obtained extraction phase was homogenized and filtered by 0.22 μm PTFE filter membrane for HPLC analysis. All extraction experiments were performed in triplicate.
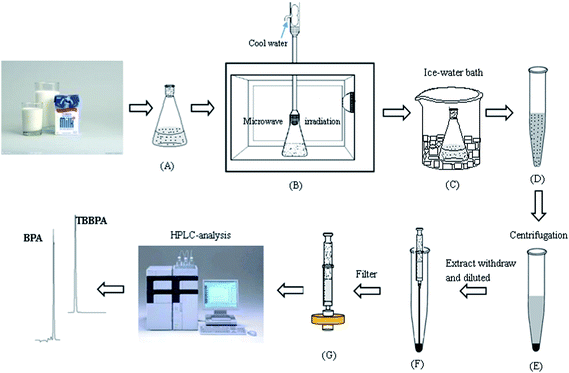 |
| Fig. 1 Schematic illustration of MAILME procedure and analysis. (A) Injection of IL and dispersant into milk sample; (B) microwave-assisted ionic liquid microextraction (C) cooling with ice water; (D) transferred to a centrifuge tube; (E) phase separation by centrifugation; (F) extract withdraw and diluted; (G) filter. | |
2.5 HPLC analysis
The gradient elution was performed as described by Li et al.5 just with a little modification. Briefly, 42% acetonitrile (0–8.0 min) ramped up to 85% acetonitrile (8.0–16.5 min) at a flow rate of 1.0 mL min−1. Injection volume was 20 μL. BPA and TBBPA were recorded at a wavelength of 280 nm. The chromatographic peaks of the analytes were confirmed by comparing their retention time with the reference standard. The extraction efficiency was expressed as recoveries.
3 Results and discussion
3.1 Optimization of MAILME conditions
In order to obtain the best extraction performance of the analytes, the type and volume of IL, microwave power, extraction time, pH and ionic strength were optimized.
3.1.1 Influence of type and volume of IL. To obtain the better extraction efficiency of MAILME for the target compounds, a suitable extraction solvent should first be found due to the different extraction capacity of ionic liquids. In this study, three 1-alkyl-3-methylimidazolium hexafluorophosphate ([CnMIM][PF6], n = 4, 6, 8) were selected and investigated, because this kind of IL is water immiscible, good soluble for organic molecules and fairly inexpensive compared with other ILs.35 As shown in Fig. 2, the recoveries of [C8MIM][PF6] for BPA and TBBPA were best among the ILs studied. The reason that [C8MIM][PF6] could lead to the best recoveries may due to the fact that the increasing alkyl chain length in [CnMIM][PF6] decreases the polarity and water miscibility of the ILs,26 and further increases the interaction and affinity between the analytes and ILs. Thus, [C8MIM][PF6] was selected as the optimal extraction solvent.
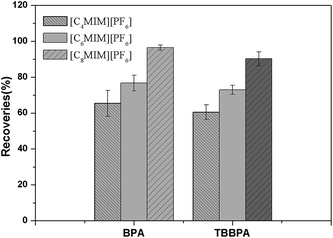 |
| Fig. 2 Influence of the type of ionic liquid on the recoveries of the analytes. Experimental conditions: IL volume, 150 μL; microwave power, 700 W; extraction time, 5.0 min; pH, 3.0; ionic strength, 5% of NaCl. | |
The volume of extraction solvent was another crucial parameter that could affect the extraction efficiency. Fig. 3 depicts the recoveries increased with the increasing of the volume of [C8MIM][PF6] from 50 to 150 μL, and then decreased. In addition, large volume of extraction solvent could easily result in larger deviation errors. Therefore, an extraction volume of 150 μL was selected in further extraction.
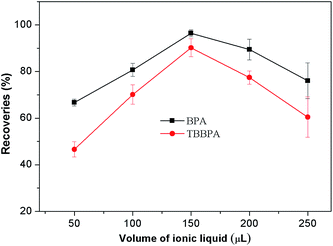 |
| Fig. 3 Influence of IL volume on the recoveries of the analytes. Other experimental conditions are as Fig. 2. | |
3.1.2 Influence of microwave power. As shown in Fig. S1 in ESI,† the recovery of BPA and TBBPA gradually increased as the microwave power increased from 120 to 700 W. It may due to that the increasing of microwave irradiation energy can increasingly enhance the penetration of the solvent into aqueous solutions and deliver analytes efficiently through molecular interaction with the electromagnetic field and offer a rapid transfer of energy to the solvent and aqueous solution, which allows the analytes to be extracted.36 Based on the result, the maximum microwave power (700 W) was selected for the MAILME method.
3.1.3 Influence of extraction time. The effect of extraction time was studied under the microwave power of 700 W. As shown in Fig. 4, the recoveries of the analytes increased significantly with extraction time increasing from 1.0 to 5.0 min. However, when the extraction time was longer than 5.0 min, no obvious improvement of the recoveries was observed, which may suggest that the extraction reached equilibrium in 5.0 min. Hence, 5.0 min was adopted as the optimal extraction time.
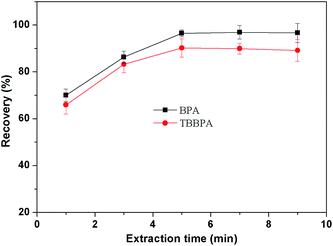 |
| Fig. 4 Influence of extraction time on the recoveries of the analytes. Other experimental conditions are as Fig. 2. | |
3.1.4 Influence of solution pH. The pH of the extracted solution is another essential factor that influences extraction efficiency particularly for acidic or basic analytes. BPA and TBBPA are weakly acidic compounds (pKa 9.73 and 6.33, respectively37), and the pH of solution has great influence on their degree of ionization. The high pH of the extracted solution can cause the ionization of the analytes. The ionic form of analytes does not interact with the extraction solvent as strongly as does at its neutral form. As a result, a small amount of analytes is extracted and the extraction efficiency is low. The extraction of BPA and TBBPA was studied with the pH of solution increasing from 1.5 to 6.0. As illustrated in Fig. S2,† the recoveries of the analytes increased with pH increasing from 1.5 to 3.0 and decreased when pH values rise from 3.0 to 6.0. The result was consistent with the studies of Li et al.5 and Zhong et al.3 Hence, the optimum pH value of 3.0 was selected for the analysis.
3.1.5 Influence of ionic strength. Generally, the addition of NaCl decreases the solubility of analytes in aqueous solution and enhances the partitioning in the extraction phase at the same time. As shown in Fig. S3,† the recoveries of BPA and TBBPA increased with the increasing of NaCl concentration, and reached maximum at NaCl concentration of 5%. But when NaCl concentration was higher than 5%, the recoveries of target compounds decreased. The salt out effect, which was enhanced with the increase of the salt concentration, may result in the increasing recoveries of the analytes. However, when the salt concentration was too high, the ion exchange between [PF6]− in [C8MIM][PF6] and Cl− in solution occurred and thus enhances the solubility of IL in water.38 This process may lead to the decrease of the amount of the settled IL phase and the poor extraction performance. At the same time, too high salt concentration would influence the phase separation which decreased the volume of IL and the recoveries correspondingly. Therefore, 5% of NaCl was added in further experiments.
3.2 Method evaluation
A series of experiments with regard to the linearity, precision and detection limits were performed to validate the proposed method at the optimum conditions. The results are illustrated in Table 1. The calibration graph for each analyte was constructed by extracting seven standard solutions in triplicate. The calibration curve was linear in the range 0.06–15 μg L−1 and 0.08–15 μg L−1 for the BPA and TBBPA, with correlation coefficients (R2) 0.9996 and 0.9999 for BPA and TBBPA, respectively. The limits of detection (LODs) and the limits of quantification (LOQs) were determined at a concentration at which signal-to-noise ratios (S/N) were equal to 3 and 10, respectively. As shown in Table 1, the LODs were 0.01 μg L−1 for BPA and 0.02 μg L−1 for TBBPA, and the LOQ were 0.03 μg L−1 for BPA and 0.05 μg L−1 for TBBPA respectively. The intra-day and inter-day variations were 3.4% and 5.6%, 5.3% and 4.7% for BPA and TBBPA, respectively, which indicated that the method has good repeatability and reproducibility. In addition, the proposed method was applied to the analysis of the aged samples to further evaluate the performances of the method. As illustrated in Table 1, the recoveries varying from 79.8 to 97.3% were obtained, showing satisfying results.
Table 1 Analytical parameters of BPA and TBBPA measured with MAILME method
|
BPA |
TBBPA |
Linear range (μg L−1) |
0.06–15 |
0.08–15 |
R2 |
0.9996 |
0.9999 |
LOD (μg L−1) |
0.01 |
0.02 |
LOQ (μg L−1) |
0.03 |
0.05 |
RSD (n = 5, %) |
Intra-day |
3.4 |
5.6 |
Inter-day |
5.3 |
4.7 |
Recovery (%, ±SD) |
1 day |
89.2 ± 4.2 |
90.2 ± 5.6 |
7 days |
90.2 ± 5.3 |
86.9 ± 3.8 |
14 days |
88.5 ± 4.7 |
97.3 ± 2.4 |
21 days |
91.3 ± 2.1 |
82.1 ± 5.6 |
30 days |
85.0 ± 3.7 |
79.8 ± 5.1 |
3.3 Applications
The proposed method was applied to the determination of BPA and TBBPA in nine commercial milk samples under the optimum conditions. The liquid milk samples were determined without any pretreatment. For the solid milk samples, 1 g of milk powder was first dissolved in 10 mL of water, mixed fully and then determined. As presented in Table 2, BPA was found in three liquid milk samples with concentration ranging from 0.50 to 2.01 μg L−1. Nevertheless, BPA was not detected in solid milk products. Migration of BPA from cans into solid products is unlikely because those usually are not coated, and even if there is a coating inside, migration will be extremely slow compared to that for the liquid products.39 TBBPA was detected one liquid milk sample (A2) with 0.12 μg L−1. TBBPA was also not found in solid milk products. The chromatograms of both blank and the spiked A2 milk sample were shown in Fig. 5. The performance of MAILME in the spiked milk samples was also investigated. As can be seen from Table 2, the relative recoveries ranged from 89.9 to 99.2%.
Table 2 Determination of BPA and TBBPA in the milk samples by the proposed methoda
Sample |
Type |
State |
Concentrations (μg L−1, ±SD) |
Recoveriesc (%, ±SD) |
BPA |
TBBPA |
BPA |
TBBPA |
A, pure milk; B, infant formula; C, powdered milk. Not detected. Spiked with 1.0 μg L−1 of BPA and TBBPA. |
1 |
A1 |
Liquid |
1.51 ± 0.06 |
NDb |
90.2 ± 3.9 |
91.4 ± 7.2 |
2 |
A2 |
Liquid |
0.50 ± 0.02 |
0.12 ± 0.01 |
87.8 ± 2.1 |
95.6 ± 3.2 |
3 |
A3 |
Liquid |
ND |
ND |
89.2 ± 4.3 |
99.2 ± 7.1 |
4 |
B1 |
Liquid |
ND |
ND |
90.8 ± 1.9 |
80.9 ± 2.2 |
5 |
B2 |
Liquid |
2.01 ± 0.08 |
ND |
79.8 ± 4.1 |
99.8 ± 4.7 |
6 |
B3 |
Solid |
ND |
ND |
82.6 ± 3.3 |
78.6 ± 2.4 |
7 |
C1 |
Solid |
ND |
ND |
90.2 ± 4.5 |
89.8 ± 2.8 |
8 |
C2 |
Solid |
ND |
ND |
83.8 ± 5.6 |
97.9 ± 4.6 |
9 |
C3 |
Solid |
ND |
ND |
78.2 ± 1.7 |
82.9 ± 3.9 |
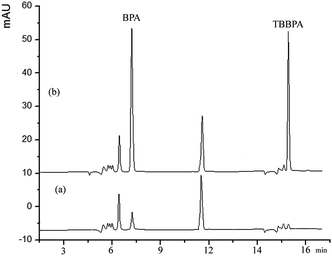 |
| Fig. 5 The chromatograms of both blank (a) and the spiked (b) A2 milk sample. | |
4 Conclusions
The presented method demonstrated a rapid, simple and feasible MAILME followed by HPLC for simultaneous determination of BPA and TBBPA in commercial milk samples. Compared with other previous method, the proposed method did not need to eliminate the fats and proteins in milk, which made the sample pretreatment simple and rapid (5.0 min). The proposed method also avoided use organic solvent. Under the optimal conditions, the LODs were 0.01 μg L−1 for BPA and 0.02 μg L−1 for TBBPA, and the LOQ were 0.03 μg L−1 for BPA and 0.05 μg L−1 for TBBPA, respectively. The proposed method had good repeatability and reproducibility with RSD lower than 5.6%. In addition, the proposed method was successfully applied to the analysis of BPA and TBBPA in the aged milk samples with the recoveries varying from 79.8 to 97.3%. The proposed method was applied to the determination of BPA and TBBPA in commercial milk samples. BPA was found in three liquid milk samples with concentration ranging from 0.50 to 2.01 μg L−1, and TBBPA was detected one liquid milk sample with 0.12 μg L−1. The recoveries of the spiked commercial milk samples were also satisfying. It is possible to extend this method to the extraction of BPA and TBBPA in other similar samples by varying the extraction conditions.
Acknowledgements
The authors acknowledge the financial support from National Natural Science Foundation of China (51108447).
References
- Q. Guo, Z. Du, Y. Zhang, X. Lu, J. Wang and W. Yu, J. Sep. Sci., 2013, 36, 677–683 CrossRef CAS PubMed.
- R. J. Law, C. R. Allchin, J. de Boer, A. Covaci, D. Herzke, P. Lepom, S. Morris, J. Tronczynski and C. A. de Wit, Chemosphere, 2006, 64, 187–208 CrossRef CAS PubMed.
- S. Zhong, S. N. Tan, L. Ge, W. Wang and J. Chen, Talanta, 2011, 85, 488–492 CrossRef CAS PubMed.
- G. Qu, J. Shi, T. Wang, J. Fu, Z. Li, P. Wang, T. Ruan and G. Jiang, Environ. Sci. Technol., 2011, 45, 5009–5016 CrossRef CAS PubMed.
- Y. Li, C. Yang, J. Ning and Y. Yang, Anal. Methods, 2014, 6, 3285 RSC.
- M. A. Abdallah, S. Harrad and A. Covaci, Environ. Sci. Technol., 2008, 42, 6855–6861 CrossRef CAS PubMed.
- X. Wei, Y. Huang, M. H. Wong, J. P. Giesy and C. K. C. Wong, Chemosphere, 2011, 85, 122–128 CrossRef CAS PubMed.
- S. Yang, S. Wang, H. Liu and Z. Yan, Environ. Sci. Pollut. Res., 2012, 19, 4090–4096 CrossRef CAS PubMed.
- H. Passos, A. C. A. Sousa, M. R. Pastorinho, A. J. A. Nogueira, L. P. N. Rebelo, J. A. P. Coutinho and M. G. Freire, Anal. Methods, 2012, 4, 2664 RSC.
- Y. Deceuninck, E. Bichon, S. Durand, N. Bemrah, Z. Zendong, M. L. Morvan, P. Marchand, G. Dervilly-Pinel, J. P. Antignac, J. C. Leblanc and B. Le Bizec, J. Chromatogr. A, 2014, 1362, 241–249 CrossRef CAS PubMed.
- A. K. Sakhi, I. T. L. Lillegaard, S. Voorspoels, M. H. Carlsen, E. B. Løken, A. L. Brantsæter, M. Haugen, H. M. Meltzer and C. Thomsen, Environ. Int., 2014, 73, 259–269 CrossRef CAS PubMed.
- Z. Shi, Y. Wu, J. Li, Y. Zhao and J. Feng, Environ. Sci. Technol., 2009, 43, 4314–4319 CrossRef CAS PubMed.
- N. C. Maragou, E. N. Lampi, N. S. Thomaidis and M. A. Koupparis, J. Chromatogr. A, 2006, 1129, 165–173 CrossRef CAS PubMed.
- B. Shao, H. Han, X. Tu and L. Huang, J. Chromatogr. B: Anal. Technol. Biomed. Life Sci., 2007, 850, 412–416 CrossRef CAS PubMed.
- J. O Mahony, M. Moloney, M. McCormack, I. A. Nicholls, B. Mizaikoff and M. Danaher, J. Chromatogr. B: Anal. Technol. Biomed. Life Sci., 2013, 931, 164–169 CrossRef CAS PubMed.
- F. Wei, X. Liu, M. Zhai, Z. Cai, G. Xu, J. Yang, S. Du and Q. Hu, Food Anal. Methods, 2013, 6, 415–420 CrossRef.
- W. Zhan, F. Wei, G. Xu, Z. Cai, S. Du, X. Zhou, F. Li and Q. Hu, J. Sep. Sci., 2012, 35, 1036–1043 CrossRef CAS PubMed.
- Y. Fujii, E. Nishimura, Y. Kato, K. H. Harada, A. Koizumi and K. Haraguchi, Environ. Int., 2014, 63, 19–25 CrossRef CAS PubMed.
- R. Rodríguez-Gómez, I. Jiménez-Díaz, A. Zafra-Gómez, O. Ballesteros and A. Navalón, Talanta, 2014, 130, 561–570 CrossRef PubMed.
- Z. Shi, Y. Wang, P. Niu, J. Wang, Z. Sun, S. Zhang and Y. Wu, J. Sep. Sci., 2013, 36, 3402–3410 CrossRef CAS PubMed.
- S. Chu, G. D. Haffner and R. J. Letcher, J. Chromatogr. A, 2005, 1097, 25–32 CrossRef CAS PubMed.
- C. Sánchez-Brunete, E. Miguel and J. L. Tadeo, J. Chromatogr. A, 2009, 1216, 5497–5503 CrossRef PubMed.
- R. Zhao, X. Wang and J. Yuan, J. Sep. Sci., 2010, 33, 1652–1657 CrossRef CAS PubMed.
- S. Q. Gao, J. Y. You, X. Zheng, Y. Wang, R. B. Ren, R. Zhang, Y. P. Bai and H. Q. Zhang, Talanta, 2010, 82, 1371–1377 CrossRef CAS PubMed.
- E. Aguilera-Herrador, R. Lucena, S. Cardenas and M. Valcarcel, TrAC, Trends Anal. Chem., 2010, 29, 602–616 CrossRef CAS.
- X. X. Song, M. D. Ye, X. J. Tang and C. J. Wang, J. Sep. Sci., 2013, 36, 414–420 CrossRef CAS PubMed.
- Y. M. Ho, Y. K. Tsoi and K. Leung, J. Sep. Sci., 2013, 36, 3791–3798 CrossRef CAS PubMed.
- B. Jastorff, R. Stormann, J. Ranke, K. Molter, F. Stock, B. Oberheitmann, W. Hoffmann, J. Hoffmann, M. Nuchter, B. Ondruschka and J. Filser, Green Chem., 2003, 5, 136–142 RSC.
- Z. Q. Gao, T. F. Liu, X. J. Yan, C. Sun, H. He and S. G. Yang, J. Sep. Sci., 2013, 36, 1112–1118 CrossRef CAS PubMed.
- J. Wang, J. Xiong, G. A. Baker, R. D. JiJi and S. N. Baker, RSC Adv., 2013, 3, 17113 RSC.
- Q. Zhong, P. Su, Y. Zhang, R. Y. Wang and Y. Yang, Anal. Methods, 2012, 4, 983–988 RSC.
- Z. X. Lou, H. X. Wang, S. Zhu, S. W. Chen, M. Zhang and Z. P. Wang, Anal. Chim. Acta, 2012, 716, 28–33 CrossRef CAS PubMed.
- C. H. Ma, T. T. Liu, L. Yang, Y. G. Zu, X. Chen, L. Zhang, Y. Zhang and C. J. Zhao, J. Chromatogr. A, 2011, 1218, 8573–8580 CrossRef CAS PubMed.
- H. A. Zeng, Y. Z. Wang, J. H. Kong, C. Nie and Y. Yuan, Talanta, 2010, 83, 582–590 CrossRef CAS PubMed.
- J. H. Zhang, H. X. Gao, B. Peng, S. Q. Li and Z. Q. Zhou, J. Chromatogr. A, 2011, 1218, 6621–6629 CrossRef CAS PubMed.
- N. Deng, M. Li, L. J. Zhao, C. F. Lu, S. L. de Rooy and I. M. Warner, J. Hazard. Mater., 2011, 192, 1350–1357 CrossRef CAS PubMed.
- H. Sambe, K. Hoshina, K. Hosoya and J. Haginaka, J. Chromatogr. A, 2006, 1134, 16–23 CrossRef CAS PubMed.
- M. T. Pena, M. C. Casais, M. C. Mejuto and R. Cela, J. Chromatogr. A, 2009, 1216, 6356–6364 CrossRef CAS PubMed.
- L. Molina-García, M. L. Fernández-de Córdova and A. Ruiz-Medina, Talanta, 2012, 96, 195–201 CrossRef PubMed.
Footnote |
† Electronic supplementary information (ESI) available. See DOI: 10.1039/c4ra16098c |
|
This journal is © The Royal Society of Chemistry 2015 |