DOI:
10.1039/C4RA15800H
(Paper)
RSC Adv., 2015,
5, 21925-21930
Green synthesis of monodispersed LaCO3OH microgears with novel plum blossom-like structure via a glycerol-mediated solvothermal method†
Received
4th December 2014
, Accepted 12th February 2015
First published on
13th February 2015
Abstract
Monodispersed LaCO3OH microgears with a novel plum blossom-like structure are prepared by a simple, reliable, environmentally-friendly and glycerol-mediated solvothermal method for the first time. By studying the effect of the experimental parameters on the morphology of LaCO3OH structures, we find that urea and glycerol at high concentration play a significant role in the formation of the plum blossom-like LaCO3OH microgears. In addition, we observe that the morphology evolution of the LaCO3OH microgears is from wires, spheres composed of rods, to plum blossom-like products with the increase of reaction time. More importantly, PL results demonstrate that the LaCO3OH microgears show a stronger PL than that of other structures, such as wires and spheres.
1. Introduction
The chemical and physical properties of inorganic micro-/nanostructure materials are related fundamentally to their geometrical factors such as morphology, size, and dimensionality. Accordingly, rational control over these factors allows us to observe unique properties of the materials.1–4 To date, many efforts have been made to explore excellent approaches to the fabrication of shape-controllable inorganic crystals to enhance their performance in currently existing applications.5–7 Rare-earth micro-/nanostructure materials have unique optical, catalytic, magnetic properties and many promising potential applications in various fields, such as in catalysis, up-conversion materials, high-quality phosphors, and so on.8–12 Among the large number of rare earth materials, LaCO3OH with diverse morphologies has been fabricated and investigated in recent years, such as double-deck-like microhexagrams,13 spiky balls and triangular hearts,14 apple-like nanostructures,15 microspheres,16 nanowires17 and layer-by-layer self-assembly of nanoplates.18 However, to the best of our knowledge, the preparation of monodispersed LaCO3OH microgears with novel plum blossom-like structure through a facile method has not been found in previous reports.
In order to obtain the controllable morphologies, organic additives are usually introduced into the reaction systems to adjust the anisotropic growth processes. Most recently, glycerol, as an environmentally benign organic additive, have been widely used, and received more and more attention in inorganic synthetic procedures. Various nanostructured materials, such as palladium,19 gold nanoparticles,20 flower-like In2O3,21 urchin-like α-FeOOH hollow spheres,22 Fe3O4–C nanospindles,23 hollow nest-like α-Fe2O3,24 nanostructured Co3O4 (ref. 25) and nano-Li4Ti5O12 (ref. 26) have been formed in glycerol.
In the present paper, we have successfully synthesized monodispersed and uniform LaCO3OH microgears with novel plum blossom-like structure by a simple, reliable, environmentally-friendly and glycerol-mediated solvothermal method. A systematic study of the reaction time, the concentration ratios of the starting reagents and a control experiment have been carried out to investigate the influence on the morphology of the LaCO3OH. In addition, the PL property of the LaCO3OH microgears with novel plum blossom-like structure is also investigated.
2. Experimental section
2.1 Preparation of monodispersed and uniform LaCO3OH microgears with novel plum blossom-like structure
All reagents were of analytical grade and used without further purification. Lanthanum(III) nitrate hexahydrate, glycerol and urea were analytical reagent grade and used without further purification. The sample was synthesized by glycerol-mediated solvothermal method, and the details are as follows: lanthanum(III) nitrate hexahydrate (0.25 g) and urea (0.034 mol) were dissolved in 30 mL of deionized water and glycerol (1
:
1) and stirred sufficiently. Then, the resulting solution was transferred into a Teflon-skinned autoclave and heated at 200 °C for 6 h. After the reaction system was naturally cooled to room temperature, the white precipitates was separated from solution and thoroughly washed for several times with deionized water and absolutely ethanol, and then dried in a vacuum oven at 50 °C for 12 h. In the current experiment, we have studied the effect of experimental parameters on the morphology of LaCO3OH, including the concentration ratio of lanthanum(III) nitrate hexahydrate to urea from 0.25 g/0.017 mol, 0.25 g/0.034 mol, and to 0.25 g/0.067 mol, and the volume ratio of water to glycerol from 10 mL/20 mL, 15 mL/15 mL, and to 20 mL/10 mL. To understand well, we have named this samples as Sample 1 (the concentration ratio of lanthanum(III) nitrate hexahydrate to urea 0.25 g/0.017 mol), Sample 2 (the concentration ratio of lanthanum(III) nitrate hexahydrate to urea 0.25 g/0.034 mol), Sample 3 (the concentration ratio of lanthanum(III) nitrate hexahydrate to urea 0.25 g/0.067 mol), Sample 4 (the volume ratio of water to glycerol 10 mL/20 mL), and Sample 5 (the volume ratio of water to glycerol 20 mL/10 mL).
2.2 Characterization
X-ray scattering patterns were conducted by analyzing the powder samples on a Philips X'Pert Pro X-ray diffractometer (XRD) with Cu Kα radiation (1.5418 Å). Field emission scanning electron microscope (FESEM) images were taken on a FESEM (Quanta 200 FEG) operated at an accelerating voltage of 10.0 kV. Transmission electron microscope (TEM) images were obtained on a JEOL JEM-2010 high resolution transmission electron microscope, equipped with X-ray energy dispersive spectroscopy (EDS) capabilities, working at an acceleration voltage of 200 kV. The photoluminescence (PL) spectrum was measured by a steady-state/lifetime spectrofluorometer (FLUOROLOG-3-TAU) at room temperature under ambient atmosphere. The LaCO3OH powders were pressed into the thin slices, and the excitation wavelength was 365 nm.
3. Results and discussion
3.1 Morphology of plum blossom-like LaCO3OH
The composition and phase purity of the products are examined by X-ray diffraction (XRD). As shown in Fig. 1(a), all reflections of the products obtained can be indexed to the pure LaCO3OH hexagonal phase (JCPDS no. 26-0815) with lattice constants a = b = 1.262 nm and c = 1.003 nm and no other peaks are observed, indicating that the as-synthesized product has a high phase purity. Scanning electron microscopy (SEM) image of the products shows that the products consist of many monodispersed, uniform microgear (Fig. 1(b)). The detailed morphology of the microgear is shown in Fig. 1(c) and (d), which reveal that every microgear has six teeth. Considering the morphology of the microgear is very similar to that of a plum blossom, as shown in the inset of Fig. 1(d), we address the type of structure as plum blossom-like microgear.
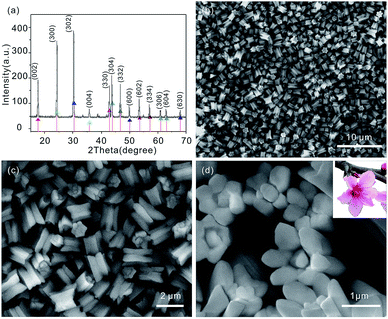 |
| Fig. 1 (a) XRD pattern and (b–d) SEM images of the LaCO3OH microgears, inset of (d): a digital photograph of a real plum blossom. The reaction parameters is including 10 mL water, 20 mL glycerol, 0.25 g lanthanum(III) nitrate hexahydrate and 0.017 mol urea and reaction time is 6 h. | |
The plum blossom-like product is further revealed by transmission electron microscopy (TEM) images in Fig. 2(a) and (b), from which we can clearly observe that the structure is assembled by some teeth. As shown in Fig. 2(b), TEM image of a single plum blossom-like microgear suggests that the length of plum blossom-like microgear is about 2 µm, which is consistent with the SEM results above (see Fig. 1(c)). The long range ordered lattice fringes are clearly viewed from high resolution transmission electron microscopy (HRTEM) image (Fig. 2(c)). The measured interplanar spacing of ∼3.66 Å is in good agreement with the d-spacing of (30
0) and (03
0) planes of the hexagonal LaCO3OH. Moreover, the angle between two planes of (30
0) and (03
0) and is observed as 119.3°, being very close to the theoretical value of 120° for hexagonal phase. The selected area electron diffraction (SAED) pattern of the plum blossom-like microgear is shown in Fig. 2(d). The diffraction points are corresponding to (0000), (1
20), (2
20), (30
0) and
planes of the hexagonal LaCO3OH. In addition, the energy-dispersive X-ray (EDX) spectrum of the products indicates that the material is composed of La, C, and O (Fig. 2(e)) without any additional elemental.
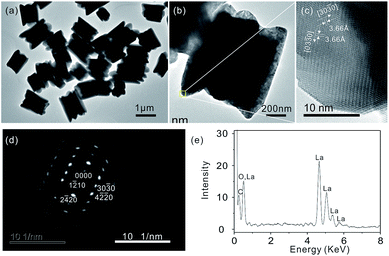 |
| Fig. 2 (a) and (b) TEM images, (c) HRTEM image, (d) SAED image and (e) EDX spectrum of the LaCO3OH microgears. | |
3.2 Effect of experimental parameters on the morphology of LaCO3OH
The reaction is mainly involved in several parameters, which affect the morphologies of the as-synthesized products, including lanthanum(III) nitrate hexahydrate, urea, water, and glycerol. Firstly, we study the effect of the concentration ratio of lanthanum(III) nitrate hexahydrate to urea on the morphology of the final products. During these experiments, the concentration ratio of lanthanum(III) nitrate hexahydrate to urea is tuned from 0.25 g/0.017 mol (named Sample 1), 0.25 g/0.034 mol (named Sample 2), and to 0.25 g/0.067 mol (named Sample 3), while the volume ratio of water to glycerol, reaction temperature and reaction time is fixed at 15 mL/15 mL, 200 °C and 6 h, respectively. As shown in Table 1 and Fig. 3(a) and (b), sphere-like products are observed when the concentration ratio of lanthanum(III) nitrate hexahydrate to urea is 0.25 g/0.017 mol. There are many small faces on every single sphere. Further increasing amount of urea to the concentration ratio of 0.25 g/0.034 mol, we find the LaCO3OH microgears are obtained successfully and the microgears are composed of several thick teeth (Fig. 1(d)). When increasing the concentration ratio to 0.25 g/0.067 mol, microgears are still obtained (Fig. 3(c) and (d)). Compared to the type of microgears synthesized under the concentration ratio of 0.25 g/0.034 mol, the products under the concentration ratio of 0.25 g/0.067 mol is microgears with glomeroplasmatic texture structures. More importantly, we find it is not beneficial for obtaining the microgears-like LaCO3OH when concentration ratio of lanthanum(III) nitrate hexahydrate to urea is 0.25 g/0.067 mol.
Table 1 Effect of experimental parameters on the morphology of LaCO3OH
Sample |
Lanthanum(III) nitrate hexahydrate (g) |
Urea (mol) |
Water (mL) |
Glycerol (mL) |
Time (h) |
Morphology |
Sample 1 |
0.25 |
0.017
|
15 |
15 |
0.5 |
Wire-like |
1 |
Rod-like |
3 |
Sphere composed by short rod |
6 |
Sphere without rod
|
Sample 2
|
0.25 |
0.034
|
15 |
15 |
0.5 |
Wire-like |
1 |
Sphere composed by short rod |
3 |
Plum blossom-like |
6 |
Plum blossom-like
|
Sample 3 |
0.25 |
0.067
|
15 |
15 |
0.5 |
Sphere composed of short rod |
1 |
Plum blossom-like |
3 |
Plum blossom-like |
6 |
Plum blossom-like
|
Sample 4 |
0.25 |
0.034 |
10 |
20
|
0.5 |
Sphere-like |
1 |
Sphere with many faces |
3 |
Plum blossom-like |
6 |
Plum blossom-like
|
Sample 2
|
0.25 |
0.034 |
15 |
15
|
0.5 |
Wire-like |
1 |
Sphere composed by short rod |
3 |
Plum blossom-like |
6 |
Plum blossom-like
|
Sample 5 |
0.25 |
0.034 |
20 |
10
|
0.5 |
Wire-like |
1 |
Wire-like |
3 |
Wire-like |
6 |
Rod-like
|
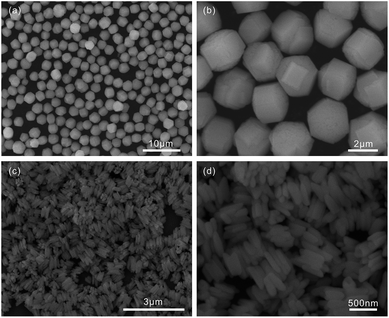 |
| Fig. 3 SEM images of products obtained using the different concentration ratio of lanthanum(III) nitrate hexahydrate to urea: (a) and (b) Sample 1 with 0.25 g/0.017 mol, (c) and (d) Sample 3 with 0.25 g/0.067 mol. Other parameters are fixed, including 15 mL glycerol and 15 mL water, T = 200 °C and t = 6 h. | |
On the basis of the above experimental results, it can be seen that the urea concentration plays a significant role in the formation of LaCO3OH with microgears-like structure. The reaction process for the formation of LaCO3OH could be described as follows:
| CO(NH2)2 + 3H2O →CO2(g) + 2NH3·H2O | (1) |
| NH3(g) + H2O NH4+ + OH− | (2) |
| La3+ + OH− + CO32− → LaCO3OH | (3) |
The hydrolyzation process of the urea provides some CO32− and OH− ions in the reaction system. According to literature reports,27 when the overall growth rate is slow, a nearly spherical morphology is favored. If the overall growth rate is fast, the growth of an anisotropic material is generally faster along an axis; and a rod-like nanoparticle is obtained. In the current work, there is only a small quantity of CO32− and OH− ions appearing when the concentration of urea is low (e.g. the concentration ratio of lanthanum(III) nitrate hexahydrate to urea is 0.25 g/0.017 mol), which can slow the growth rate of LaCO3OH crystal. Thus, the LaCO3OH crystal with sphere-like structure is obtained, as shown in Fig. 3(a) and (b). When using the urea with high concentration (e.g. the concentration ratio of lanthanum(III) nitrate hexahydrate to urea is 0.25 g/0.034 mol or 0.25 g/0.067 mol), more CO32− and OH− ions in the reaction system, can increase the growth rate of LaCO3OH crystal. As a result, the LaCO3OH crystals with plum blossom-like microgear structures are prepared, as shown in Fig. 1, Fig. 3(c) and (d).
Besides the concentration ratio of lanthanum(III) nitrate hexahydrate to urea has a strong effect on the morphology of the final products, the volume ratio of water to glycerol has also shown strong effects on the morphology of the products. In this section, the concentration ratio of lanthanum(III) nitrate hexahydrate to urea, reaction temperature and reaction time are fixed at 0.25 g/0.034 mol, 200 °C and 6 h, respectively; and at the same time the water/glycerol volume ratio is tuned from 10 mL/20 mL (named Sample 4), 15 mL/15 mL (named Sample 2), and to 20 mL/10 mL (named Sample 5). Fig. 4(a) and (b) show that the SEM images of products synthesized when the water/glycerol volume ratio is 10 mL/20 mL. We can see that there are a large number of short microgears appearing in the products. As the water/glycerol volume ratio is increased to 15 mL/15 mL, morphology of the as-prepared products is still microgears, which is longer and larger compared with products synthesized with the water/glycerol volume ratio 10 mL/20 mL. In contrast, the as-prepared products become rods of 900 nanometers in length and about 80 nm in diameter when the volume ratio of water/glycerol is changed to 20 mL/10 mL, as shown in Fig. 4(c) and (d). As a result, we find it is not beneficial for obtaining the microgears-like LaCO3OH when amount of glycerol is decreased until the volume ratio of water/glycerol is 20 mL/10 mL.
 |
| Fig. 4 SEM images of products obtained under different water/glycerol volume ratios: (a) and (b) Sample 4 with 10 mL water and 20 mL glycerol, (c) and (d) Sample 5 with 20 mL water and 10 mL glycerol. Other parameters are fixed, including 0.25 g lanthanum(III) nitrate hexahydrate and 0.034 mol urea, T = 200 °C and t = 6 h. | |
Based on the experimental results about tuning the volume ratio of water to glycerol to affect the morphology of LaCO3OH, we believe solvent density and viscosity plays a crucial role in governing the controlled growth of different self-assembled structures and shapes.28,29 According to previous reports, relative big solvent density and viscosity will show a given instantaneous driving force for the self-assembly of complicated LaCO3OH. This is due to the mobility of products in a given solvent. In the current experiment, the solvent density and viscosity for glycerol is 1.26 g mL−1 and 1.50 Pa s, respectively; and the solvent density and viscosity for water is 1.00 g mL−1 and 0.001 Pa s, respectively. At a lower molar ratio between water/glycerol volume ratio (e.g. 10 mL/20 mL), there is insufficient freedom for the crystals to agglomerate. Thus, more glycerol can drive the producing of microgears-like LaCO3OH. In contrast, at a higher molar ratio (e.g. 20 mL/10 mL), there is more freedom to promote a highly anisotropic growth in the two direction, thus extending into long rod-like structures.
3.3 Evolution process of plum blossom-like LaCO3OH
The reaction time is also critical for studying the morphology evolution process in our current experiment. As shown in Table 1, Fig. 5 and S1–4.† SEM and XRD are employed to monitor the evolution of the morphology and crystalline structure of Sample 2 collected at different reaction times. Fig. 5(a–c) shows the SEM images of Sample 2 obtained at different reaction times. As shown in Fig. 5(a), after 30 min reaction, some wires-like products are produced. When the reaction time is increased to 1 h, there are some sphere-like products appeared (Fig. 5(b)). These sphere-like products are composed of many short rods. In addition, a few wires are still remaining. Further prolonging the reaction to 3 h, the plum blossom-like microgear products are obtained (Fig. 5(c)). The morphology of the product does not change any more with longer reaction time (e.g. 6 h). For other samples, the morphology of products is also evaluated along with different reaction time. Regarding Sample 1, the shape is changed from wire to short rod, and to sphere composed of short rod when the reaction time is tuned from 0.5 h, 1 h, and 3 h. The morphology of Sample 3 is changed from sphere, to sphere with many faces, to microgears. Sample 4 evaluates from sphere, to sphere, and to microgears. For Sample 5, the morphology is changed from wire, to wire, and to rod. There are no microgears producing. Although the formation mechanism of the plum blossom-like LaCO3OH is not fully clear, we can be sure that the morphology evolution process of products is seemed from wire, to sphere composed of rod, to spheres with many faces, and to plum blossom-like products. The corresponding XRD patterns of the intermediate products obtained at different reaction times are shown in Fig. 5(d) and S1–4.† It is shown that the peaks of original products are weak in the XRD pattern for the reaction time of 30 min, which indicates that the product obtained at the early reaction stage is poorly crystallized. As the reaction time is extended to 1 h, 3 h and 6 h, it is clear that he pure LaCO3OH with hexagonal phase (JCPDS no. 26-0815) is obtained. Besides, it is also observed the crystallization become better along with the reaction time, which further implies that long reaction time is beneficial to the preparation of well-crystallized products.
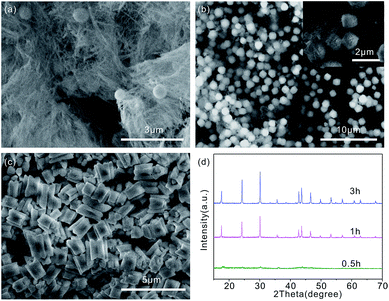 |
| Fig. 5 SEM images of Sample 2 collected at different reaction times: (a) 0.5 h, (b) 1 h, (c) 3 h. (d) XRD patterns of the products above (Sample 2: 15 mL water, 15 mL glycerol, 0.25 g lanthanum(III) nitrate hexahydrate, 0.034 mol urea and T = 200 °C). | |
3.4 Fluorescence spectrum of the as-prepared plum blossom-like microgears LaCO3OH
We also investigate the effect of morphology/crystallization on their PL intensities. Fig. 6 shows a comparison of the room temperature luminescent intensities of Samples 2 with different reaction time, including 0.5 h, 1 h, 3 h and 6 h (see Table 1). On the one hand, upon excitation with ultraviolet (365 nm) irradiation, the emission spectrum of the Sample 2 at four reaction time have only one broad band maximum at about 465 nm, and blue emission is seen in the products. The emission spectrum of the Sample 2 consists of a broad band located between 375 and 650 nm and can be attributed to the self-trapped exciton (STE) luminescence.30 A large number of the free holes and free electrons are created after the lattice is irradiated, and the STEs can be formed directly from electron–hole pairs. During the diffusion of the STEs, they can be an irradiative recombination, leading to luminescence.31 The PL property of the Sample 2 is in accordance with the previous literatures.16,32
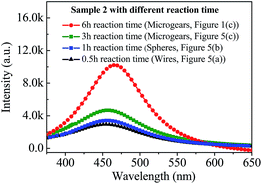 |
| Fig. 6 Room temperature photoluminescence (PL) spectra (excited by λex = 365 nm) of the LaCO3OH sample with different reaction time: 0.5 h, 1 h, 3 h and 6 h. | |
On the other hand, according to discussion above, the morphology changed from wires, to spheres composed of rods, and finally to microgears along with the reaction time from 0.5 h, 1 h, 3 h and 6 h, as shown in Fig. 5(a)–(c) and 1(d). More importantly, the crystallization of sample also becomes better gradually (Fig. 5(d)). It can be seen clearly that the emission spectra of the four samples are similar in shape and different only in the relative intensity under identical measurement condition. Namely, the relative emission intensity of the microgears (reaction time 6 h) is higher than that of the wire (reaction time 0.5 h), sphere (reaction time 1 h) and microgears (reaction time 3 h). In general, the photoluminescence emission intensity of materials has a significant correlation with their morphologies and crystallization.33,34 It is well-known that the surface area of materials increases along with the decrease of their particle size. Besides, the bad crystallization is also possible to produce more defects. In our current work, the average surface area of microgear is larger that of the wire and sphere due to their smaller particle size. The larger the surface area of materials, the more the defects, which are disadvantageous to the PL intensity as they provide non-radiative recombination routes for electrons and holes. If the surface area is greatly reduced along with fewer defects would show great improvement in the PL intensity (e.g. microgears with 6 h reaction time).
4. Conclusions
In summary, we have synthesized monodispersed and uniform LaCO3OH microgears with novel plum blossom-like structure via a simple, environmentally friendly and glycerol-mediated solvothermal method. By studying effect of experimental parameters on the morphology of LaCO3OH, including the concentration ratio of lanthanum(III) nitrate hexahydrate from 0.25 g/0.067 mol, 0.25 g/0.034 mol, and to 0.25 g/0.017 mol, and the volume ratio of water to glycerol from 10 mL/20 mL, 15 mL/15 mL, and to 20 mL/10 mL, we find the urea under higher concentration and glycerol with relative big solvent density and viscosity play a significant role in the formation of LaCO3OH with microgears. In addition, the evolution process of microgears LaCO3OH is seemed from wire, to sphere composed of rod, to sphere with many faces, and to plum blossom-like products by monitoring the evolution of the morphology and crystalline structure of the products collected at different reaction times. PL result shows one emission band centered at 465 nm (λex = 365 nm) of the LaCO3OH microgears with novel plum blossom-like structure. More importantly, compared to other structure (e.g. wire, sphere), the as-prepared LaCO3OH microgears produce stronger PL, which will bring a new opportunity to research and apply luminescence fields.
Acknowledgements
This research was supported by the National Natural Science Foundation of China (no. 51302102, 51402120, 61273066, 61104205, 51472204, and 51302101), the Natural Science Foundation of Anhui Province (no. 1408085QA19), the Huaibei Scientific Talent Development Scheme (no. 20140305) and the Natural Science Major Research Project for Colleges and Universities of Anhui Province (no. KJ2014A222). We also thank the support of Key Scientific and Technological Team from Shan Xi Province, start-up funds from NWPU and fund of State Key Laboratory of Solidification Processing no. 2014KA040098C040098.
References
- Z. Wang, D. Luan, C. M. Li, F. Su, S. Madhavi, F. Y. C. Boey and X. W. Lou, J. Am. Chem. Soc., 2010, 132, 16271–16277 CrossRef CAS PubMed.
- T. Sun, Y. S. Zhang, B. Pang, D. C. Hyun, M. Yang and Y. Xia, Angew. Chem., Int. Ed., 2014, 53, 12320–12364 CAS.
- Y. Zhao and L. Jiang, Adv. Mater., 2009, 21, 3621–3638 CrossRef CAS.
- Q. Zhang, W. Wang, J. Goebl and Y. Yin, Nano Today, 2009, 4, 494–507 CrossRef CAS PubMed.
- M.-R. Gao, Y.-F. Xu, J. Jiang and S.-H. Yu, Chem. Soc. Rev., 2013, 42, 2986–3017 RSC.
- S. Xie, S.-I. Choi, X. Xia and Y. Xia, Curr. Opin. Chem. Eng., 2013, 2, 142–150 CrossRef PubMed.
- H. Zhang, M. Jin, Y. Xiong, B. Lim and Y. Xia, Acc. Chem. Res., 2012, 46, 1783–1794 CrossRef PubMed.
- B. Pan, Q. Xie, H. Wang, J. Zhu, Y. Zhang, W. Su and X. Wang, J. Mater. Chem. A, 2013, 1, 6629–6634 CAS.
- Q. Wang, Y. Liu, B. Liu, Z. Chai, G. Xu, S. Yu and J. Zhang, CrystEngComm, 2013, 15, 8262–8272 RSC.
- H.-X. Mai, Y.-W. Zhang, R. Si, Z.-G. Yan, L.-d. Sun, L.-P. You and C.-H. Yan, J. Am. Chem. Soc., 2006, 128, 6426–6436 CrossRef CAS PubMed.
- G. Wang, Q. Peng and Y. Li, J. Am. Chem. Soc., 2009, 131, 14200–14201 CrossRef CAS PubMed.
- J. Wang, R. Deng, M. A. MacDonald, B. Chen, J. Yuan, F. Wang, D. Chi, T. S. A. Hor, P. Zhang and G. Liu, Nat. Mater., 2014, 13, 157–162 CrossRef CAS PubMed.
- G. Li, C. Peng, C. Zhang, Z. Xu, M. Shang, D. Yang, X. Kang, W. Wang, C. Li and Z. Cheng, Inorg. Chem., 2010, 49, 10522–10535 CrossRef CAS PubMed.
- X. Yang, Z. Zhai, L. Xu, M. Li, Y. Zhang and W. Hou, RSC Adv., 2013, 3, 3907–3916 RSC.
- S.-L. Zhong, L.-F. Zhang, J.-W. Jiang, Y.-H. Lv, R. Xu, A.-W. Xu and S.-P. Wang, CrystEngComm, 2011, 13, 4151–4160 RSC.
- Y. Zhang, K. Han, T. Cheng and Z. Fang, Inorg. Chem., 2007, 46, 4713–4717 CrossRef CAS PubMed.
- Z. Li, J. Zhang, J. Du, H. Gao, Y. Gao, T. Mu and B. Han, Mater. Lett., 2005, 59, 963–965 CrossRef CAS PubMed.
- J. Xie, Q. Wu, D. Zhang and Y. Ding, Cryst. Growth Des., 2009, 9, 3889–3897 CAS.
- Y.-W. Lee, S.-B. Han, A. R. Ko, H.-S. Kim and K.-W. Park, Catal. Commun., 2011, 15, 137–140 CrossRef CAS PubMed.
- R. k. Genç, G. Clergeaud, M. Ortiz and C. K. O'Sullivan, Langmuir, 2011, 27, 10894–10900 CrossRef PubMed.
- C. Wang, D. Chen and X. Jiao, J. Phys. Chem. C, 2009, 113, 7714–7718 CAS.
- B. Wang, H. Wu, L. Yu, R. Xu, T.-T. Lim and X. W. Lou, Adv. Mater., 2012, 24, 1111–1116 CrossRef CAS PubMed.
- F. Xu, W. Kang, X. Wang, R. Liu, C. Zhao and Q. Shen, CrystEngComm, 2013, 15, 4431–4437 RSC.
- Z. Wei, R. Xing, X. Zhang, S. Liu, H. Yu and P. Li, ACS Appl. Mater. Interfaces, 2012, 5, 598–604 Search PubMed.
- W. Yang, Z. Gao, J. Ma, J. Wang, B. Wang and L. Liu, Electrochim. Acta, 2013, 112, 378–385 CrossRef CAS PubMed.
- W. Zhang, J. Li, Y. Guan, Y. Jin, W. Zhu, X. Guo and X. Qiu, J. Power Sources, 2013, 243, 661–667 CrossRef CAS PubMed.
- X. Peng, L. Manna, W. Yang, J. Wickham, E. Scher, A. Kadavanich and A. P. Alivisatos, Nature, 2000, 404, 59–61 CrossRef CAS PubMed.
- X. Li, J. Wang, Y. Zhang, M. Li and J. Liu, Eur. J. Inorg. Chem., 2010, 2010, 1806–1812 CrossRef.
- M. N. Nadagouda and R. S. Varma, Green Chem., 2006, 8, 516 RSC.
- F. Chen, H. Liu, K. Wang, H. Yu, S. Dong, X. Chen, X. Jiang, Z. Ren and J. Liu, J. Phys.: Condens. Matter, 2005, 17, L467 CrossRef CAS.
- Y. Kawabe, A. Yamanaka, E. Hanamura, T. Kimura, Y. Takiguchi, H. Kan and Y. Tokura, J. Appl. Phys., 2000, 87, 7594–7596 CrossRef CAS PubMed.
- J. Wang, X. Jing, J. Wang, L. Ge, S. Jamil and M. Zhang, Solid State Sci., 2010, 12, 1934–1940 CrossRef CAS PubMed.
- I. Djerdj, G. Garnweitner, D. Sheng Su and M. Niederberger, J. Solid State Chem., 2007, 180, 2154–2165 CrossRef CAS PubMed.
- G. Li, C. Peng, C. Zhang, Z. Xu, M. Shang, D. Yang, X. Kang, W. Wang, C. Li, Z. Cheng and J. Lin, Inorg. Chem., 2010, 49, 10522–10535 CrossRef CAS PubMed.
Footnote |
† Electronic supplementary information (ESI) available. See DOI: 10.1039/c4ra15800h |
|
This journal is © The Royal Society of Chemistry 2015 |
Click here to see how this site uses Cookies. View our privacy policy here.